Electric steelmaking dust as a raw material for coagulant production
- 1 — Ph.D., Dr.Sci. Head of Department Belgorod State Technological University named after V.G.Shukhov ▪ Orcid
- 2 — Ph.D., Dr.Sci. Professor Belgorod State Technological University named after V.G.Shukhov ▪ Orcid
- 3 — Ph.D. Researcher Saint Petersburg Mining University ▪ Orcid
- 4 — Senior Lecturer Belgorod State Technological University named after V.G.Shukhov ▪ Orcid
- 5 — Postgraduate Student Kazan National Research Technological University ▪ Orcid
- 6 — Postgraduate Student Belgorod State Technological University named after V.G.Shukhov ▪ Orcid
Abstract
The paper describes the issues associated with waste generated during steel production and processing, in particular the dust from electric arc furnaces (EAF). An effective solution for the disposal of such waste is its involvement in processing to obtain valuable products. This paper studies the physical and chemical properties of EAF dust produced during the smelting of metallized pellets and captured by the dust and gas cleaning system of the steel-smelting shop at the Oskol Electrometallurgical Combine, Belgorod Region. The results obtained in the study of the chemical and disperse compositions of dust, the microstructure of the surface made it possible to propose the use of dust as a raw material for coagulant production. The conditions of acid-thermal treatment of dust are determined, contributing to the partial dissolution of iron (II), (III), and aluminium compounds, which ensure the coagulation processes during wastewater treatment. Model solutions show high efficiency (> 95 %) of water treatment from heavy metal ions by modified EAF dust.
Introduction
Steel production and processing are associated with a large amount of industrial waste [1-3]. Most part of this waste does not find proper use and for this reason is stored in industrial landfills and temporary storage sites. The accumulation of a large amount of industrial waste and the need for its temporary storage cause a number of serious environmental and economic problems [4, 5]. An effective solution to these problems can be the involvement of this waste in the resource circulation as a feed for the production of various materials, which is a prerequisite for low-waste and waste-free technologies. Such an approach to the disposal and processing of man-made industrial waste contributes to solving the issues of resource and energy saving, makes it possible to expand the range of manufactured products, increase the raw material base and its diversity [6, 7]. In addition, the involvement in the production cycles of abandoned large-tonnage industrial waste will make it possible to release many hectares of land now under dumps and tailings and begin their reclamation and fertility recovery [8-10]. The importance of the indicated problem can be judged by the scale of mining and processing of iron ore. In 2013, Russia produced 102 Mt of iron ore, making it the fifth largest iron ore producer after China, Australia, Brazil, and India. According to Rosgeolfond, the country’s iron ore reserves for 2020 are estimated at about 112 Bt, with more than 60 % of the reserves in the Kursk Magnetic Anomaly (KMA) in the European part of Russia [11].
The KMA region is one of the largest iron ore (mainly magnetite and hematite) deposits on Earth. The area of iron ore deposits within the KMA is 120 thousand km2. They are located in three regions of Russia (Kursk, Belgorod, and Orel) [12-14]. Rich ores were discovered in 1931. Mineralization is represented by ferruginous quartzites (banded iron formation) occurring together with various Early Precambrian metamorphic and igneous rocks [15, 16]. High iron zones were formed by hypergene enrichment of the upper horizons of the banded iron formation. The reserves of ferruginous quartzites with iron content of 32-37 % are more than 25 Bt, and the amount of enriched ores with iron content of 52-66 % exceeds 30 Bt. The deposits are developed by open-pit (Stoilensky, Lebedinsky, Mikhailovsky) and underground (Korobkovsky) mines [17].
Iron ore mining is sometimes viewed as mere mineral extraction, but the industry is fiercely competitive internationally and is currently under increased pressure to reduce costs due to the recent significant drop in iron ore prices. Despite modern advances, the international iron ore industry will have to develop alternative types of ores in the coming decades as the reserves of high iron ore around the world continue to dwindle. Magnetite and polymetallic ores, which are now mined in China, can act as a replacement. They have a complex mineral composition and fine grains.
More than 30 % of world steel is produced in electric arc furnaces [18]. During the steelmaking in such furnaces at temperatures above 1600 °C, many volatile components of the feedstock evaporate. This gas is cooled, and large particles are collected in cyclones, while smaller particles are captured in bag filters or electrostatic precipitators. Production of 1 ton of raw steel generated approximately 10-20 kg of EAF dust. It was estimated that 6 Mt of EAF dust is generated worldwide every year, while only 2.5 Mt of dust is recycled. EAF dust contains various metal elements, mainly depending on the type of scrap steel, iron ore, and smelting conditions. In general, the dust consists of 7-40 wt. % Zn and 30-45 wt. % Fe in the forms ZnFe2О4, ZnO, Fe2О3, and Fe3О4. Other minor metallic elements such as Mn, Al, and Cr are also present in EAF dust and therefore the dust is classified as a hazardous waste that must be properly disposed of or treated in accordance with the European Waste Catalogue and the List of Hazardous Waste. Obviously, the processing and disposal of EAF dust is an urgent task [18, 19].
The seriousness of the recycling management issue arises from the fact that EAF dust is generated in significant volumes and the amount is constantly increasing. The chemical composition of the dust varies depending on the type of raw material and steel produced, and these differences can be significant [20, 21]. Efforts were made around the world to stabilize EAF dust. There are studies on the use of dust with a high FeO content for asphalt modification, in cement mortars, to produce sintered bodies with promising mechanical properties [22-24]. Studies evaluating the use of EAF dust in ceramic and cement compositions show that although the dust properties may vary depending on the physical and chemical properties of the raw materials and process parameters, it is possible to develop process circuits and modify the waste (i.e., by hydrometallurgy, pyrometallurgy, etc.) considering the needs of the target application area [25-27].
Another well-known direction of management and processing of waste containing metals is leaching, or extraction, of valuable components with various reactants [28]. Selective extractants are used to separate certain metals from undesirable components and from each other or in groups [29-31]. The metal-containing filtrate is separated individually by solvent extraction using cationic, anionic, and neutral extractants. The hydrometallurgical method makes it possible to extract and purify metals in multi-element solutions [32-34]. Due to the specific chemical composition and high dispersion, such dust can be used to obtain coagulants suitable for wastewater treatment [35, 36].
The purpose of this work was a comprehensive study of the mineral composition and physical and chemical properties of electric steelmaking dust in the production of steel in electric arc furnaces at the Oskol Electrometallurgical Plant (Stary Oskol, Belgorod Region). When achieving the goal, we had to solve the following tasks:
- conduct comprehensive studies of the physical and chemical properties of EAF dust;
- determine the influence of conditions of acid treatment of dust on leaching of iron, zinc, aluminum from EAF dust;
- assess the possibility of using modified EAF dust as a coagulant in wastewater treatment.
Materials and methods
The studies used EAF dust formed during the melting of metallized pellets and captured in the dust and gas cleaning system of the steel-smelting shop at the Oskol Electrometallurgical Plant. EAF dust consists of a light fraction of metal-containing compounds and is a finely dispersed system of a multi-component composition. For dust treatment, concentrated hydrochloric acid was used. Hydrochloric acid was chosen as one of the well-known strong mineral acids, which does not release dangerous substances such as SO2 or NxOy into the air.
To increase the reactivity of the dust, it was treated with hydrochloric acid at a temperature of 23 °C and boiled. Boiling was carried out in HCl solutions diluted 1:3 and 1:5 for 0.5; 2, and 3 h. After the end of boiling, the contents of the reaction vessels were quantitatively transferred into flasks with a capacity of 1 dm3, filtered; the remaining precipitate was analysed using X-ray diffraction (XRD), and the concentrations of Fetot, Al, and Zn were determined in the solution.
Microstructural studies were carried out using a TESCAN MIRA 3 LMU electron microscope. This microscope has the possibility of high resolution (to 1 nm) and energy dispersive microanalysis, magnification from 4 to 1000000. The investigation was carried out at an accelerating voltage from 200 V to 30 kV with continuous adjustment. The maximum value of the probe current is 200 nA. The SE secondary electron detector of the Everhart – Thornley type was used to obtain topographic contrast images. We also used the AdvancedAztecEnergy (IE350)/ X-max80 energy-dispersive microanalysis system with a guaranteed spectral resolution on the Mn Ka line of 127 eV. The electron source is a high brightness Schottky cathode. Resolution was 1 nm at 30 kV.
X-ray phase analysis of EAF dust ground in an agate mortar and pressed into a tablet was carried out using an ARL 9900 WorkStation X-ray fluorescence spectrometer with a built-in diffraction system.
Experimental studies on the treatment of model solutions were conducted out as follows. Model solutions were prepared containing Fe3+, Fe2+, Al3+, Zn2+, Cu2+ ions at concentrations of 10 mg/dm3. To 100 cm3 of model solutions, a dust suspension in an HCl solution was added in an amount of 0.5 to 4 cm3, mixed for 30 min, and filtered. In the filtrate after treatment of the model solution, the residual concentration of the pollutant was determined by the atomic absorption method on the KVANT-Z.ETA-T device according to the M-MVI 539-03 method.
The particle size distribution of the dust was measured using a Micro Sizer 201 laser particle sizer, which makes it possible to study particles in the range from 0.2 to 600 μm. The bulk density was found by weighing a known volume of dust and dividing the mass found by the volume. To determine the true density, the pycnometric method was used in an alcohol solution. In order to measure the pH of the aqueous extract, 10 g of dust was added to 100 cm3 of distilled water with a pH of 7; the contents of the laboratory container were boiled for 30 minutes, filtered through a paper filter, the pH of the medium was determined in the filtrate using an Akvilon I-500 device.
Statistical processing was carried out for the experimental data, Student's t-test was calculated for independent samples. The critical values of the t-test for df = 8 are 2.31 (p = 0.05), 3.36 (p = 0.01), and 5.04 (p = 0.001). Student's t-test for independent samples was calculated using the formula
where and are the sample means; σ12 and σ22 are sample variance of samples; n1 and n2 are the number of experiments in each sample.
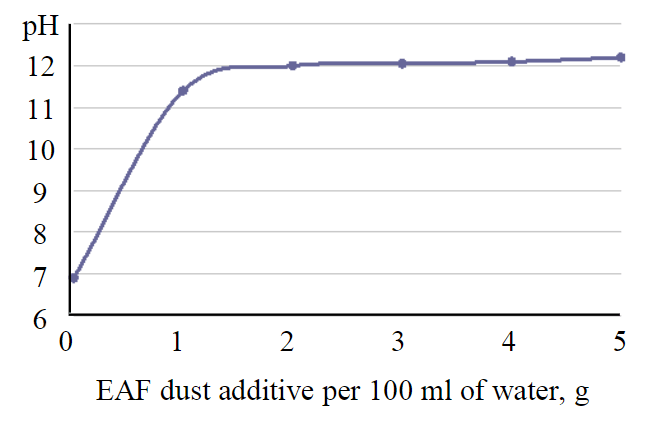
Fig.1. Change in the pH of the aqueous medium upon the addition of EAF dust
Discussion of the results
EAF dust of the current electric steelmaking is a finely dispersed system of complex composition of dark brown colour with a moisture content to 2.5 % and a bulk particle size (> 60 %) to 0.1 mm. Processing properties of EAF dust: bulk density ρbulk = 0.94 g/cm3; true density ρtrue = 3.64 g/cm3; pH of water extract 11.2; solubility in water 6.4 %; solubility in 1 n HCl is greater than 82.6 %. The graph of changes in the pH of the aqueous medium when the dust is added is shown in Fig.1. When the values of hydrate formation in the solution are reached, the heavy metal ions existing in the solution (and released from the dust) form poorly soluble hydroxides and are removed from the solution together with coagulation particles [37].
The mineral composition of the dust, according to XRF estimates, is represented by such compounds as Fe3O4 (FeO·Fe2O3), Fe2O3, MgO, Al2O3, SiO2, Ca(OH)2. Compounds of di- and trivalent iron are mainly oxides, compounds that are poorly soluble in water and are not able to participate in exchange reactions in normal conditions [38].
Figure 2 shows X-ray diffraction patterns of initial dust and dust treated with acid diluted 1:3 for 0.5; 2, and 3 h, the ratio of dust to acid solution is 1:5.
Iron peaks in Fig.2, b, c are somewhat lower compared to Fig.2, a, which confirms the fact of dust dissolution and the transition of metals to a dissolved state. As expected, the lowest peaks are noted in Fig.2, d, which indicates the highest level of leaching of the slag components.
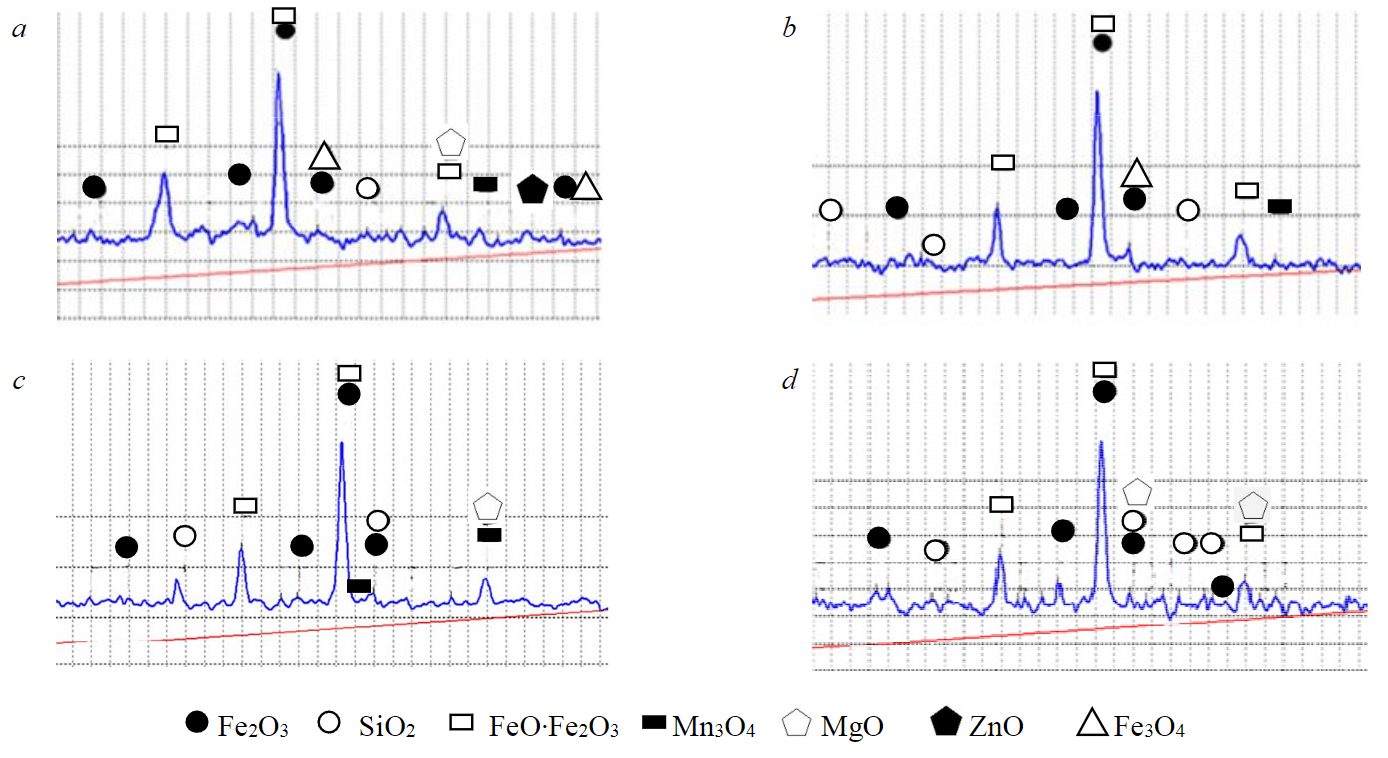
Fig.2. X-ray patterns of initial EAF dust (a) and dust treated with acid diluted 1:3 for 0.5 (b), 2 (c), 3 h (d)
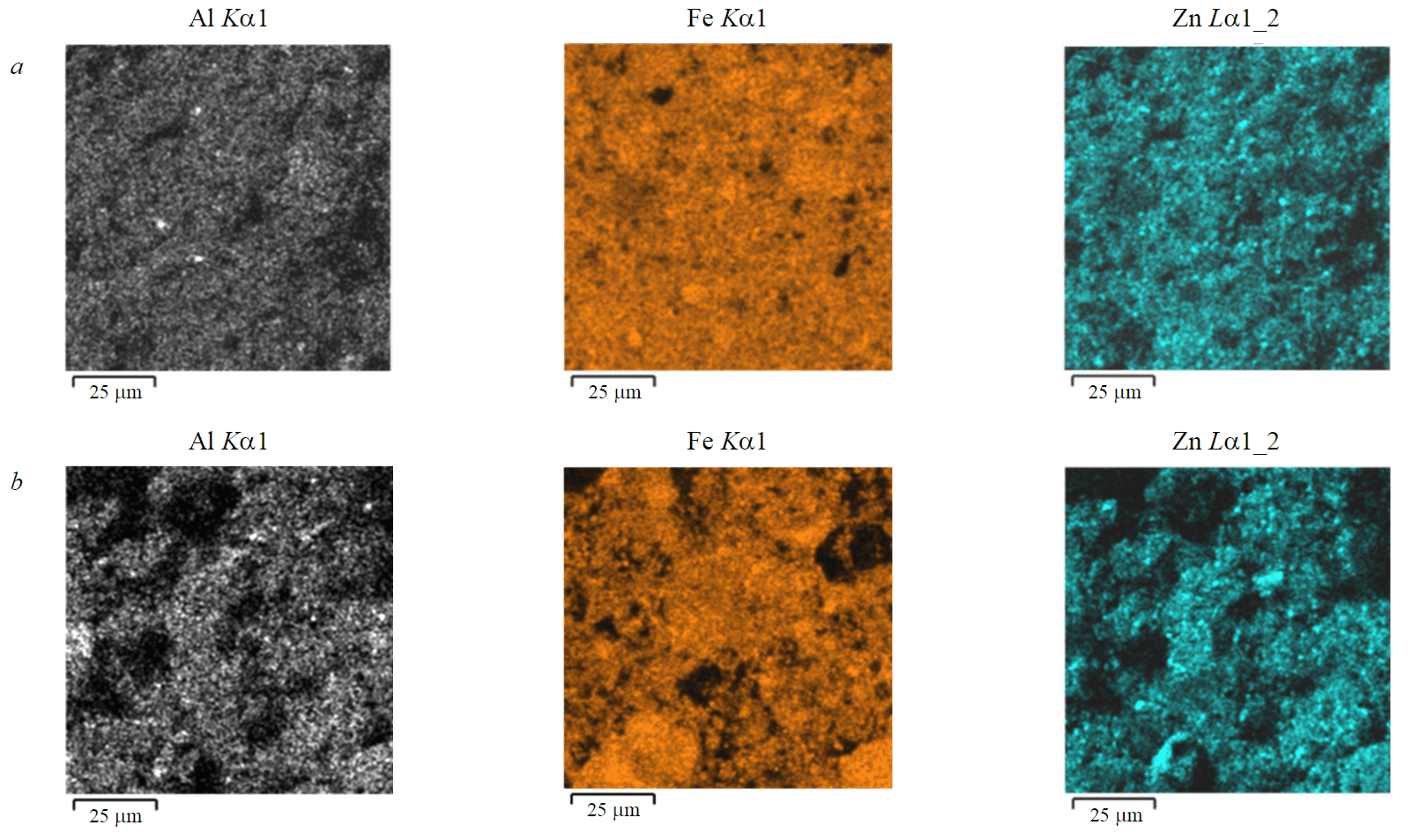
Fig.3. Multilayer maps of the energy-dispersive spectra of the dust surface after 180 min of boiling in HCl diluted 1:3 (a); 1:5 (b) and indicating sections of Fe, Al, and Zn
Multilayer maps of the energy dispersive spectra of the dust surface are shown in Fig.3.
Figure 4 shows micrographs of the dust particles surface and energy dispersive spectra for samples treated with HCl.
The average values of the elemental composition of EAF dust samples are presented in Table 1.
Table 1
Averaged values of the elemental composition of dust after acid treatment
Treatment temperature, °C |
Treatment conditions |
Initial сontent of elements, wt.% |
|||||||||||||
Acid dilution |
Treatment time, min |
21.5С |
33.98О |
0.71Na |
1.99Mg |
0.29Al |
8.91Si |
1.72S |
1.75Cl |
1.14K |
1.1Ca |
2.51Mn |
40.12Fe |
4.87Zn |
|
23 |
1:5 |
30 |
17.13 |
30.82 |
0.68 |
1.62 |
0.22 |
8.02 |
0.65 |
1.55 |
0.29 |
0.22 |
2.31 |
34.42 |
3.42 |
1:5 |
120 |
19.12 |
31.14 |
0.27 |
1.50 |
0.18 |
6.84 |
0.48 |
0.96 |
0.15 |
0.22 |
1.60 |
36.75 |
1.99 |
|
1:5 |
180 |
18.04 |
31.08 |
0.09 |
1.25 |
0.06 |
4.88 |
0.13 |
0.78 |
0.09 |
0.06 |
1.13 |
35.72 |
1.87 |
|
1:3 |
30 |
16.73 |
31.06 |
0.33 |
1.59 |
0.18 |
8.52 |
1.53 |
0.49 |
1.00 |
0.98 |
1.14 |
39.97 |
3.52 |
|
1:3 |
120 |
11.93 |
31.78 |
0.27 |
1.44 |
0.16 |
6.67 |
0.94 |
0.43 |
0.66 |
0.46 |
1.79 |
36.19 |
2.50 |
|
1:3 |
180 |
16.58 |
33.82 |
0.25 |
1.07 |
0.07 |
4.85 |
0.81 |
0.32 |
0.48 |
0.37 |
0.85 |
33.92 |
2.04 |
|
100 |
1:5 |
30 |
16.91 |
30.42 |
0.67 |
1.6 |
0.22 |
7.92 |
0.64 |
1.53 |
0.283 |
0.22 |
2.28 |
33.98 |
3.38 |
1:5 |
120 |
18.87 |
30.74 |
0.263 |
1.48 |
0.18 |
6.75 |
0.47 |
0.95 |
0.15 |
0.22 |
1.58 |
36.28 |
1.96 |
|
1:5 |
180 |
17.81 |
30.68 |
0.09 |
1.23 |
0.06 |
4.82 |
0.13 |
0.77 |
0.09 |
0.06 |
1.12 |
35.26 |
1.85 |
|
1:3 |
30 |
16.52 |
30.66 |
0.33 |
1.57 |
0.18 |
8.41 |
1.51 |
0.48 |
0.99 |
0.97 |
1.13 |
39.46 |
3.47 |
|
1:3 |
120 |
11.78 |
31.37 |
0.27 |
1.42 |
0.16 |
6.58 |
0.93 |
0.42 |
0.65 |
0.45 |
1.77 |
35.73 |
2.47 |
|
1:3 |
180 |
16.37 |
33.39 |
0.25 |
1.06 |
0.07 |
4.79 |
0.80 |
0.32 |
0.47 |
0.37 |
0.84 |
33.48 |
2.01 |
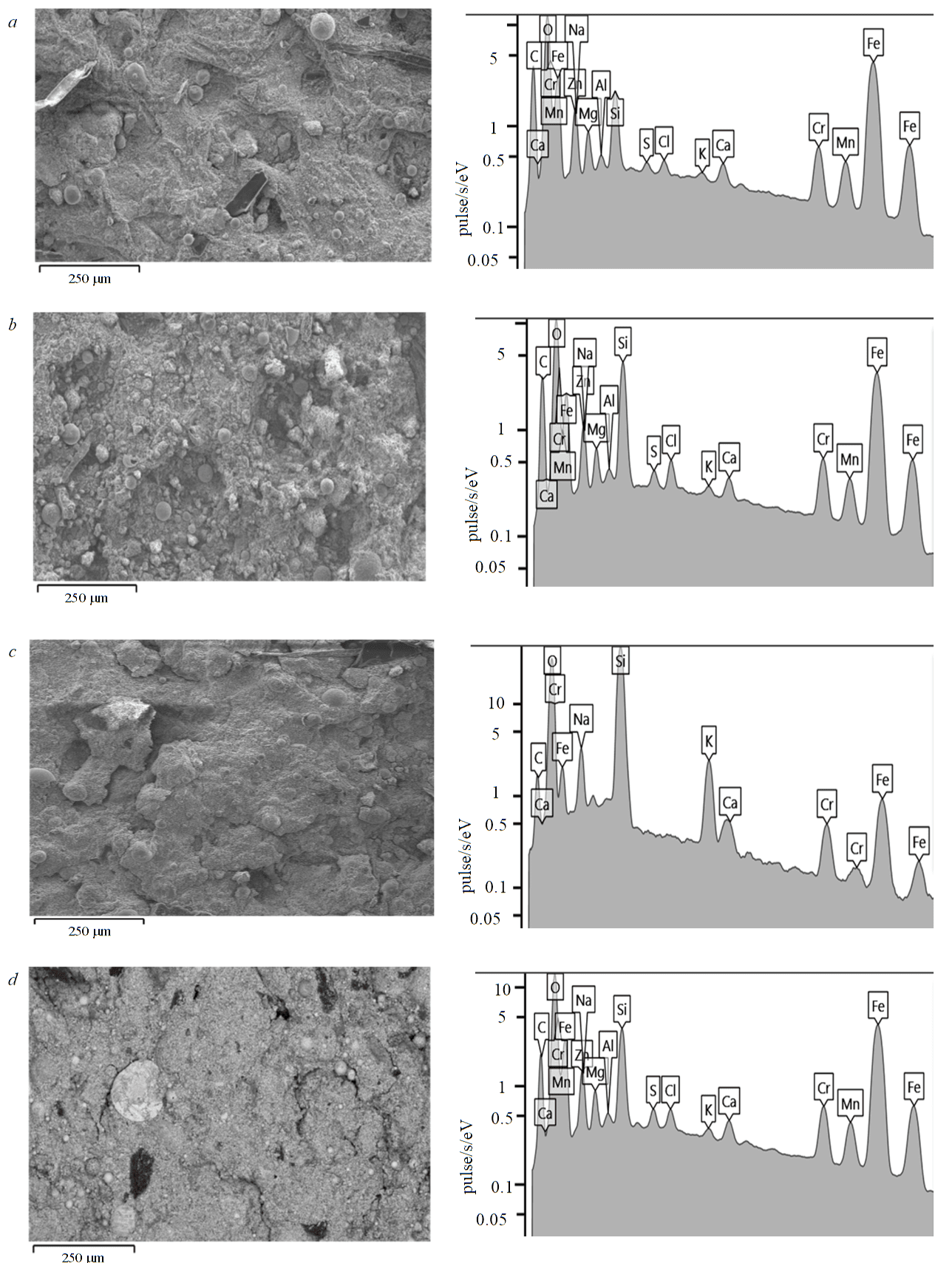
Fig.4. Dust surface in reflected electrons after boiling in HCl and energy dispersive spectrum: after 30 min of boiling with dilution 1:5 (a), 1:3 (c); after 180 min of boiling with dilution 1:5 (b), 1:3 (d)
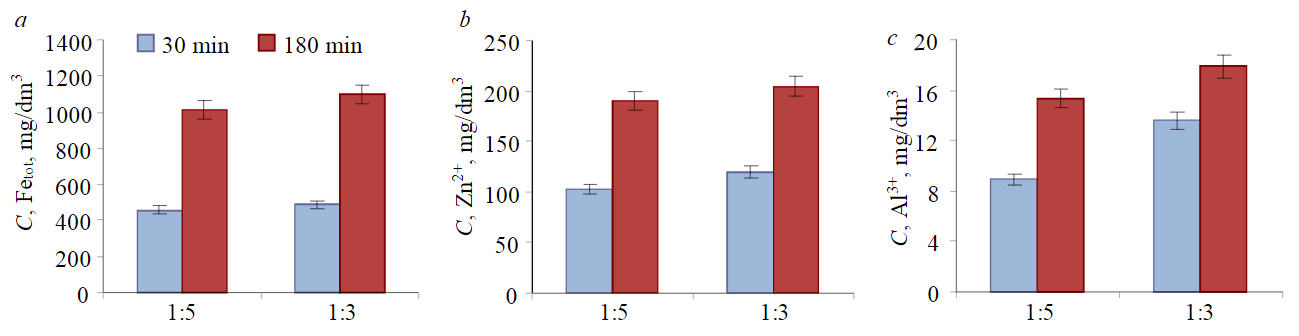
Fig.5. Leaching of iron (a), zinc (b), and aluminum (c) from EAF dust at different HCl concentrations
It follows from Table 1 that as the acid concentration and the treatment duration increase, the dust contains an ever smaller amount of elements, Mg, Al, Fe, Zn. Zn content in the dust also decreases as the treatment duration and the acid concentration increase. The preliminary experiments determined that 3 hours of EAF dust treatment is sufficient. A further increase in the duration of boiling leads to unjustified energy costs. Thus, at a 1:5 dilution, as the treatment time increases from 30 to 180 min, the Zn content in the dust decreases from 3.38 to 1.85 %. At a 1:3 dilution, as the treatment time increases from 30 to 180 min, the Zn content decreases from 3.47 to 2.01 %. Figure 5 shows the dependence of the intensity of leaching of Fetot, Al3+, and Zn2+ ions on the acid solution concentration.
Table 2
Student's t-test values
Treatment time, min | Acid dilution | t-test | ||
Fe | Zn | Al | ||
30 | 1:5 | 5.09923.86 | 4.103 | 15.503 |
30 | 1:3 | |||
180 | 1:5 | 23.86 | 14.3564.103 | 18.721 |
180 | 1:3 |
The resulting difference in the concentration of metal ions in solutions at different acid concentrations is statistically significant (Table 2). Thus, within the framework of this study, hydrochloric acid diluted 1:3; heat treatment temperature 100 °C; duration of boiling 180 min can be considered the best conditions for transferring Fe2+, Fe3+, Al3+, Zn2+ ions into solution.
Modified dust can be used as a coagulation material for wastewater treatment [36].
In an aqueous medium, Fe2+, Fe3+, Al3+ cations undergo hydrolysis according to the following tentative reactions:
The Al3+ ions are hydrolysed similarly to the Fe3+ ion:
Zn2+ ions are hydrolysed similarly to Fe2+.
As it is known, hydrolysis is the interaction of salt dissociation products with water to form large flakes, which can then be separated from the aqueous medium by decantation or filtration.
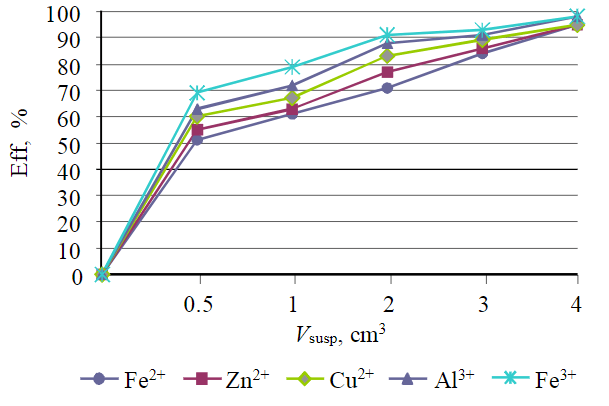
Fig.6. Dependence of treatment efficiency on suspension volume
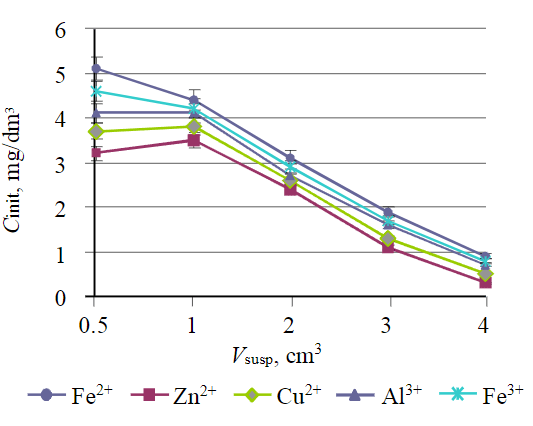
Fig.7. Influence of suspension volume on the content of metal ions in solution
In the practice of water treatment, salts Al2(SO4)3, AlCl3, FeCl3, Fe2(SO4)3, FeSO4, FeCl2 and their mixtures in various combinations are widely used as coagulants [36].
Wastewater from various industrial productions often simultaneously contain ions of many heavy metals, Ni2+, Cu2+, Cd2+, Co2+, Cr3+ etc. With an increase in the pH of the aqueous medium, these ions form poorly soluble hydroxides, Ni(OH)2, Cu(OH)2, Cd(OH)2, Co(OH)2, Cr(OH)3, which, due to their high dispersion, form a stable hard-to-remove suspension. In this case, the use of coagulants makes it possible to extract suspended particles from a dispersed medium using coagulation and purify the solution to the specified requirements.
Due to the fact that the dust composition includes oxides such as CaO, Na2O, K2O, BaO, MgO, etc., when dust is added to the aqueous medium, they partially dissolve, which invariably leads to an increase in the pH of the medium. During acid treatment of dust, the pH of the medium also gradually increases over time due to the dissolution of alkaline components.
The results of studies presented in Fig.6 and 7 show that the treatment efficiency for all ions is at least 95 %. If it is necessary to achieve a lower residual concentration of metal ions, it is possible to use the second treatment stage.
Thus, EAF dust can be successfully used to obtain a coagulant suitable for use in water treatment. This conclusion is confirmed by experimental data. In the course of observations, large flakes of coagulated particles were noted after adding a coagulating suspension to a finely dispersed system. After coagulation, a rapid, even sedimentation was observed.
Conclusion
The prospects for the use of steel industry waste as a raw material for coagulant production are considered. According to the analysis of literary sources, the coagulation material obtained on the base of EAF dust can be used in wastewater treatment. It was determined that the recommended conditions for obtaining a coagulation mixture are HCl acid diluted 1:3, boiling duration is 180 min. These conditions lead to partial dissolution of dust and the transition of Fe3+, Fe2+, Al3+ ions into solution, causing a coagulation effect in the aqueous medium with respect to suspended fine particles. EAF dust after acid treatment is used as a coagulation mixture, which leads to wastewater treatment with a high efficiency of 95-98 %.
References
- Sizyakov V.M., Kozyrev B.A. Processing of formate solutions obtained from red mud leaching. iPolytech Journal. 2021. Vol. 25. N 5, p. 633-642 (in Russian). DOI: 10.21285/1814-3520-2021-5-633-642
- Lebedev A.B., Utkov V.A., Bazhin V.Yu. Use of alumina production waste red mud during molten sulfur-containing slag granulation. Metallurgist. 2019. Vol. 63, p. 727-732. DOI: 10.1007/s11015-019-00882-z
- Urbanovich N.I., Korneev S.V., Volosatikov V.I., Komarov D.O. Analysis of the composition and processing technologies of dispersed iron-containing waste. Foundry Production and Metallurgy. 2021. N 4, p. 66-69 (in Russian). DOI: 10.21122/1683-6065-2021-4-66-69
- Aryngazin K.Sh., Stanevich V.T., Tleulesov A.K. et al. Research of Production Processes of Concrete Products on the Steelmaking Slags Basis. Science and Technology of Kazakhstan. 2018. N 4, p. 43-49 (in Russian).
- Ruzavin A.A. Disposal of Steelmaking Slags by Accelerated Carbonation. Bulletin of the South Ural State University.Seriya “Construction Engineering and Architecture”. 2018. Vol. 18. N 3, p. 68-72 (in Russian). DOI: 10.14529/build180310
- Duisekenov R.K., Mazdubai A.V. Dust and Sludge of Gas Cleaning Plants and Analysis of Ways of Their Utilization. Science and Technology of Kazakhstan. 2020. N 3, p. 29-37 (in Russian).
- Belskii S.S., Zaitseva A.A., Tyutrin A.A. et al. Current state of steelmaking slag processing. iPolytech Journal. 2021. Vol. 25. N 6, p. 782-794 (in Russian). DOI: 10.21285/1814-3520-2021-6-782-794
- Chukaeva M.A., Povarov V.G., Sverchkov I.P. Iron-Containing Metalworking Wastes as a Chemosorbent for Wastewater Treatment from Molybdenum Ions. Moscow University Chemistry Bulletin. 2020. Vol. 75. N 1, p. 36-42. DOI: 10.3103/S0027131420010058
- Novichikhin A.V., Shorokhova A.V. Systematic processing of iron-ore waste in mining regions. Steel in Translation. 2017. Vol. 47, p. 456-462. DOI: 10.3103/S0967091217070105
- Svergusova S.V., Sapronova Zh.A., Svyatchenko A.V. Iron-Containing Modeled Waste as Raw Material for Coagulant Receiving. IOP Conference Series: Earth and Environmental Science. 2019. Vol. 272. Iss. 3. N 032007. DOI: 10.1088/1755-1315/272/3/032007
- 11. Holmes R.J., Yifan Lu, Liming Lu. Introduction: overview of the global iron ore industry. Iron Ore. Mineralogy, Processing and Environmental Sustainability. Amsterdam: Elsevier, 2022, p. 1-56. DOI: 10.1016/B978-0-12-820226-5.00023-9
- Taylor P.T., Kis K.I., Wittmann G. Satellite-altitude horizontal magnetic gradient anomalies used to define the Kursk Magnetic Anomaly. Journal of Applied Geophysics. 2014. Vol. 109, p. 133-139. DOI: 10.1016/j.jappgeo.2014.07.018
- Gzogyan T.N., Gzogyan S.R. Material Composition of Rich Iron Ore Deposits of KMA. Nauchnye vedomosti Belgorodskogo gosudarstvennogo universiteta. Seriya Estestvennye nauki. 2018. Vol. 42. N 2, p. 131-141 (in Russian). DOI: 10.18413/2075-4671-2018-42-2-131-141
- Khramtsov B.A., Bakaras M.V., Kravchenko A.S., Korneychuk M.A. Loose Dump Stability Control at Open Pit Iron Ore Mines of the Kursk Magnetic Anomaly. Mining informational and analytical bulletin. 2018. N 2, p. 66-72 (in Russian). DOI: 10.25018/0236-1493-2018-2-0-66-72
- Oreshkin D., Proshlyakov A. Assessment of Ecological Parameters of Iron Ore Deposit Open-Cast Mining in Time Based on the Resource Approach. Proceedings of the Siberian department of the section of earth sciences of the Russian academy of natural sciences. Geology, exploration and development of mineral deposits. 2017. Vol. 40. N 4, p. 98-111 (in Russian). DOI: 10.21285/2541-9455-2017-40-4-98-111
- Egger F. Implementation of a Dynamic Mining Strategy Following Changing Conditions in a Multipit, Multielement Blending Iron Ore Mining Operation. BHM Berg- und Hüttenmännische Monatshefte. 2011. Vol. 156, p. 325-329. DOI: 10.1007/ s00501-011-0014-3
- Savko A.D., Ovchinnikova M.Yu. The Main Types and Mineral Composition of Bauxites of the Kursk Magnetic Anomaly. Uchenye Zapiski Kazanskogo Universiteta. Seriya estestvennye nauki. 2020. Vol. 162. N 2, p. 274-289 (in Russian). DOI: 10.26907/2542-064X.2020.2.274-289
- Huimin Tang, Zhiwei Peng, Liancheng Wang et al. Facile synthesis of zinc ferrite as adsorbent from high-zinc electric arc furnace dust. Powder Technology. 2022. Vol. 405. N 117479. DOI: 10.1016/j.powtec.2022.117479
- Karahan B.D. Carbon coated electric arc furnace dust prepared by one-pot pyrolysis: An effcient, low carbon footprint electrode material for lithium-ion batteries. Materials chemistry and physics. 2022. Vol. 287. N 126178. DOI: 10.1016/j.matchemphys.2022.126178
- Mamdouh O., Timo F., Eetu-Pekka H. Selective Zinc Removal from Electric Arc Furnace (EAF) Dust by Using Microwave Heating. Journal of Sustainable Metallurgy. 2019. Vol. 5, p. 331-340. DOI: 10.1007/s40831-019-00222-0
- Toporkova Yu.I., Bludova D., Mamyachenkov S.V., Anisimova O.S. A review of processing methods for electric arc furnace dust. iPolytech Journal. 2021. Vol. 25. N 5, p. 643-680 (in Russian). DOI: 10.21285/1814-3520-2021-5-643-680
- Loaiza A., Colorado H.A. Marshall stability and flow tests for asphalt concrete containing electric arc furnace dust waste with high ZnO contents from the steel making process. Construction and Building Materials. 2018. Vol. 166, p. 769-778. DOI: 10.1016/j.conbuildmat.2018.02.012
- Aryngazin K., Abisheva A. Use of recycled waste in the production of building materials. Technobius. 2022. Vol. 2. N 2. N 0020. DOI: 10.54355/tbus/2.2.2022.0020
- Atroshchenko Yu.M., Nikishina M.B., Simonov R.V. Study of the strength characteristics of building composites using converter slags. Endless light in science. 2022. N 5-5, p. 161-165 (in Russian). DOI: 10.24412/2709-1201-2022-161-165
- Korneeva E.V., Berdov G.I. Use of Electric Furnace Slag in Production of Clinkerless Cement. Bulletin of the South Ural State University. Seriya “Construction Engineering and Architecture”. 2018. Vol. 18. N 3, p. 35-40. (in Russian). DOI: 10.14529/build180305
- Kauchur А.S., Sheleh V.K., Kauchur S.G. et al. Development of Terracot Ceramic Tiles Manufacturing Technology with use of Technogenic Products of Energy Complex. Vestnik Vitebskogo gosudarstvennogo tekhnologicheskogo universiteta. 2017. N 2 (33), p. 86-94 (in Russian).
- 27. Singh M., Saini B., Chalak H.D. An Overview on Waste Materials Used in Engineered Cementitious Composite. Smart Technologies for Sustainable Development. Cham: Springer, 2021. LNCE. Vol. 78, p. 213-222. DOI: 10.1007/978-981-15-5001-0_17
- Montoya D.A., Pistofidis N., Giannakopoulos G. et al. Revisiting the iron-rich “ordinary Portland cement” towards valorisation of wastes: study of Fe-to-Al ratio on the clinker production and the hydration reaction. Materials and Structures. 2021. Vol. 54. N 30. DOI: 10.1617/s11527-020-01601-w
- Shahbaz A. A systematic review on leaching of rare earth metals from primary and secondary sources. Minerals Engineering. 2022. Vol. 184. N 107632. DOI: 10.1016/j.mineng.2022.107632
- Martins Th.A.G., Calda M.P.K., Tavares de Moraes V. et al. Recovering metals from motherboard and memory board waste through sulfuric leaching. Journal of Environmental Chemical Engineering. 2021. Vol. 9. Iss. 6. N 106789. DOI: 10.1016/j.jece.2021.106789
- Fomina D.D., Danilov N.F. Leaching of Vanadium Compounds in the Processing of Vanadium Pentoxide Production Chemical Waste Using Sulfuric and Nitric Acids. Vestnik Permskogo natsionalnogo issledovatel'skogo politekhnicheskogo universiteta. Khimicheskaya tekhnologiya i biotekhnologiya. 2021. N 2, p. 77-93 (in Russian). DOI: 10.15593/2224-9400/2021.2.07
- Voropanova L.A., Pukhova V.P. Extraction of Copper, Cobalt and Nickel Ions from Aqueous Solutions by Extractant Cyanex 272. Journal of Mining Institute. 2018. Vol. 233, p. 498-505. DOI: 10.31897/PMI.2018.5.498
- Kioresku A.V. Intensification of Bacterial-Chemical Leaching of Nickel, Copper and Cobalt from Sulfide Ores Using Microwave Radiation. Journal of Mining Institute. 2019. Vol. 239, p. 528-535. DOI: 10.31897/PMI.2019.5.528
- Awasthi A.K., Jinhui Li. An overview of the potential of eco-friendly hybrid strategy for metal recycling from WEEE. Resources, Conservation & Recycling. 2017. Vol. 126, p. 228-239. DOI: 10.1016/j.resconrec.2017.07.014
- Svergusova S.V., Sapronova Zh.A., Svyatchenko A.V. et al. Coagulant on the base of industrial ferriferous waste for the preliminary treatment of multi-component sewage. III International Scientific and Technical Conference Energy Systems (ICES 2018), 29-30 November 2018, Belorgod, Russian Federation. IOP Conference Series Materials Science and Engineering, 2019. Vol. 552. N 012026. DOI: 10.1088/1757-899X/552/1/012026
- Sverguzova S.V., Sapronova Zh.A., Svyatchenko A.V. Technology to produce iron-containing coagulant of steel production wastes for treatment rainwater. Bulletin of Belgorod state technological university named after V.G.Shukhov. 2016. N 12, p. 160-164 (in Russian). DOI: 10.12737/22652
- Sverguzova S.V., Shaikhiev I.G., Porozhnyuk L.A. et al. Possible uses of solid waste from electric steel smelting – dust from electric arc furnaces. Vestnik Kazanskogo tekhnologicheskogo universiteta. 2014. Vol. 17. N 6, p. 199-201 (in Russian).
- Ipanov D.Yu. Treatment of wastewater from phosphates and heavy metals by dust from electric arc furnaces: Avtoref. dis. … kand. tekhn. nauk. Penza: Penzenskaya gosudarstvennaya tekhnologicheskaya akademiya, 2015, p. 19 (in Russian).