Microbiological remediation of oil-contaminated soils
- 1 — Environmental Engineer AO Novaya Aviatsiya ▪ Orcid
- 2 — Ph.D. Senior Lecturer Saint Petersburg Mining University ▪ Orcid
Abstract
Microbiological remediation is a promising technology for the elimination of environmental contamination by oil and petroleum products, based on the use of the metabolic potential of microorganisms. The issue of environmental contamination by crude oil and its refined products is relevant in the Russian Federation since the oil industry is one of the leading sectors of the country. Mechanical and physico-chemical methods of treatment are widely used to clean oil-contaminated soils. However, the methods belonging to these groups have a number of significant drawbacks, which actualizes the development of new methods (mainly biological), since they are more environmentally friendly, cost-effective, less labor-intensive, and do not require the use of technical capacities. Various bio-based products based on strains and consortia of microorganisms have been developed that have proven effectiveness. They include certain genera of bacteria, microscopic fungi, and microalgae, substances or materials acting as sorbents of biological agents and designed to retain them in the soil and increase the efficiency of bioremediation, as well as some nutrients. Statistical data, the most effective methods, and technologies, as well as cases of using microorganisms to restore oil-contaminated soils in various climatic conditions are presented.
Introduction
The oil-producing and refining industries can be attributed to one of the leading industries in terms of the amount of waste generated [1]. According to Rosstat, in 2020 in Russia, the mass of waste after the extraction of crude oil and natural gas, as well as after the production of coke and petroleum products amounted to 8127.1 and 928.6 thousand tons, respectively. Most of the waste generated falls on oil-producing companies (more than 1 million tons of oil sludge and oil-contaminated soils), oil refineries (0.7 million tons), oil depots (0.3 million tons), railways, airports, and seaports (0.5 million tons) [2].
Oil is a valuable energy resource, providing, according to various estimates, 30-35 % of global energy consumption [3, 4].
According to the Federal Classificatory Catalogue of Wastes, oil-containing waste belongs to the third (moderately hazardous waste, oil content of 15 % or more) or fourth hazard class (low-hazard substances, oil content less than 15 %). Oil in its pure form, depending on the fractional composition and impurities, is classified as a second or third-hazard-class substance in accordance with the current regulatory documentation (GOST R 51858-2020). The presence of volatile aromatic hydrocarbons, naphthalene, and sometimes sulfur compounds and other impurities in the composition of oil causes its high environmental hazard as a waste of human activity.
Environmental contamination occurs during the construction and operation of oil-producing wells, transportation and processing of hydrocarbon raw materials, and other processes associated with an increase in oil production and transportation [5]. According to some data, more than a third of the total number of oil contamination cases is associated with transportation [6]. Due to the low solubility and nonpolar and hydrophobic nature of the oil components, the physical and chemical removal of contaminants is difficult [7]. The solubility of certain hydrocarbon fractions in water depends on their structure, molecular weight, and ambient temperature. At a temperature of 25 °C and an atmospheric pressure of 0.1 MPa, the lower hydrocarbons have the greatest solubility (Table 1). Solubility among hydrocarbons with the same number of carbon atoms declines in a row: arenes, naphthenes, and alkanes [8].
Table 1
Solubility of petroleum hydrocarbons in water at 25 °C and an atmospheric pressure of 0.1 MPa
Compound |
Solubility, mg/ml |
Compound |
Solubility, mg/ml |
Alkanes |
|||
Methane |
24.4 |
2-Methylpentane |
13.8 |
Ethane |
60.4 |
2,2-Dimethylpentane |
18.4 |
Propane |
62.4 |
2,4-Dimethylpentane |
4.06 |
n-Butane |
61.4 |
n-Heptane |
2.93 |
Isobutane |
48.9 |
n-Octane |
0.66 |
n-Pentane |
38.5 |
n-Nonan |
0.122 |
Isopentane |
47.8 |
n-Decane |
0.022 |
n-Hexane |
9.5 |
|
|
Cyclic compounds |
|||
Cyclopentane |
156.0 |
Methylcyclopentane |
42.0 |
Cyclohexane |
55.0 |
Methylcyclohexane |
14.0 |
Aromatic compounds |
|||
Benzene |
1780.0 |
Ethylbenzene |
152.0 |
Toluene |
515.0 |
1,2,4-Trimethylbenzene |
57.0 |
o-Xylene |
175.0 |
Isopropylbenzene |
50.0 |
Polycyclic aromatic compounds |
|||
Indane |
109.1 |
Phenantrene |
1.29 |
Naphthalene |
31.7 |
Pyrene |
0.135 (2.4) |
Diphenyl |
7.0 |
Anthracene |
0.073 (3.2) |
Fluorene |
1.98 |
Chrysen |
0.002 |
As a result of contamination, irreversible changes occur: disruption of natural biocenoses, changes in their species diversity; decrease in the respiratory and productive capacity of organisms; changes in the enzymatic activity of soils; deterioration of the quality of life and human health [9]. In world history, there have been many accidental oil spills, many of which have caused environmental disasters. Thus, when the Maltese tanker Erika broke up and sank in 1999 in the Bay of Biscay (about 20 thousand tons of fuel oil), about 400 km of the coast was flooded with an oil-water mixture. On the coast, 65 thousand oil-contaminated seabirds were collected, of which 50 thousand were already dead. Important coastal marine fisheries, areas of mariculture, and tourist areas in the provinces of Brittany and Vendée were affected [10].
On April 20, 2010, an explosion occurred on the Deepwater Horizon offshore drilling rig, 80 km off the coast of Louisiana (USA) in the Gulf of Mexico. The oil spill was precisely the main cause of the ecological disaster – almost 1800 km of coasts were contaminated, and tens of thousands of marine animals and birds died [10].
On November 28, 2015, the oil tanker Nadezhda crashed on Sakhalin Island near the port of Nevelsk. As a result of damage to the hull, oil products sank into the waters of the Tatar Strait. Contamination occurred over the water area of more than 20 thousand m2, the length of the contaminated coastal strip was about 7 km, and birds and fish died en masse.
There are various methods of eliminating oil contamination: mechanical, physico-chemical, and biological [11]. Mechanical methods are most widely used, but their impact often causes no less damage to the environment than oil contamination [12, 13]. Some physico-chemical methods are also used, in particular sorption [14], the disadvantage of which is the need to recycle spent sorbents. The physico-chemical method – the introduction of ameliorants [15, 16], for example, based on sewage sludge [17] – is not always applicable and effective, and also requires additional research of each specific sediment and specifics of treated territories.
Inorganic sorbents, i.e., diatomite rocks, various clays, sand, pumice, zeolites, etc., among which diatomites and clays are used most widely due to low cost and large available volumes. These inorganic sorbents as well as sand (used for filling small areas of oil and petroleum product spills), have a low sorption capacity of 70-150 % for oil and do not retain gasoline, diesel fuel, and kerosene. When eliminating the consequences of oil spills in the aquatic environment, inorganic sorbents sink together with oil, thus contributing to the contamination of reservoirs. Most often, sorbents are disposed of by burning (that emits greenhouse gases), washing by water with surfactants or extractants (which can lead to eutrophication of reservoirs where wastewater is discharged), or by storing in dumps (becoming a source of a complex of environmental problems).
Synthetic sorbents have the lowest cost and can be produced from industrial waste. They are characterized by high volume, light weight, buoyancy after oil absorption, high sorption rate, and the possibility of regeneration and reuse. Molded polyethylene with polymer fillers, polyurethane in granular or spongy form, and other types of plastics, pressed basalt or polypropylene fibers are widely used (oil absorption rates: 6.1-7.2 g/g for basalt fibers; 3.8-4.5 g/g for polypropylene fibers). The use of carbon fiber for oil sorption increases the cost of filter elements, but shows a high cleaning effect, 10.2-10.6 g/g. Synthetic sorbents are not bio-decomposable and require incineration, which entails significant environmental issues associated with the emission of greenhouse gases and toxic combustion products of synthetic materials, and additional fuel and electricity costs.
Peat, waste paper, sawdust, wool, dried cereals, etc. are more often used as organic sorbents. Such sorbents have a small sorption capacity (no more than 15 kg/kg) and a low adsorption rate, so they are used with an oil film thickness of no more than 0.1 mm [18]. For this reason, there is a need to develop new environmentally friendly, inexpensive, simple, sustainable, and effective methods for removing oil products from contaminated soils [19]. Biological methods (bioremediation and phytoremediation) have shown promising results for the restoration of soils contaminated with crude
oil [20] and the products of its processing [21].
Microbiological remediation is a method based on the ability of microorganisms to destruct organic contaminants by including such substances in its metabolic cycle, is one of the most effective methods of eliminating oil and petroleum product spills at the stage of post-treatment of contaminated sites [22, 23].
A review of the existing statistical data, Russian and foreign developments, and research directions devoted to microbiological bioremediation of oil-contaminated soils is presented.
Discussion
Basic information about oil contamination
Crude oil is the main resource for the production of petroleum fractions, fuels, oils, and various materials. It includes mainly such petroleum hydrocarbons as aliphatic and aromatic compounds, resins, and asphaltenes [24].
According to Rosstat data for 2019 in Russia, the area of disturbed and used land due to accidents during the transportation of oil, gas, and refined products amounted to 3120 ha, and the area of reclaimed land from this category for the same period was 806 ha (Table 2).
Table 2
The area of reclaimed and used land disturbed due to oil contamination in Russia, 2015-2019
Cause of land degradation |
Year |
||||
2015 |
2016 |
2017 |
2018 |
2019 |
|
Disturbed, ha: |
|||||
Total |
136232 |
444503 |
25642 |
119481 |
194225 |
Due to leakage during the transit of oil, gas, and oil products |
821 |
514 |
995 |
131 |
3120 |
Used, ha: |
|
|
|
|
|
Due to leakage during the transit of oil, gas, and oil products |
382 |
463 |
709 |
188 |
2892 |
Reclaimed, ha: |
|||||
Total |
86552 |
92052 |
98673 |
59397 |
102225 |
Due to leakage during the transit of oil, gas, and oil products |
492 |
547 |
639 |
178 |
806 |
The area of disturbed and used lands in Russia remains significant, and the growing rates of reclamation are not able to fully compensate for the damage caused to the environment due to oil spills. The main sources of soil contamination with oil are leaks during accidents on transport and pipelines, drilling, and oil production; places of storage of oil refining waste; natural disasters; household waste, etc. [25]. In 2021, about 32 accidents with oil or petroleum product spills occurred in Russia; up to 10 thousand cases of field pipeline depressurization happen annually. The biggest accident in 2021 was an oil spill at the offshore terminal of the Caspian Pipeline Consortium near Novorossiysk. The environmental damage is estimated at more than 4 billion rubles.
Microbiological remediation
The development of bioremediation technologies for the restoration of contaminated soils began in the 1970s precisely for the purification of lands adjacent to the areas of oil production. Bioremediation is mainly applied to matrices, such as soil or some types of wastewater, and can be carried out in-situ (on site) or ex-situ (in bioreactors) [26]. Soils are the second most studied object of bioremediation after water, accounting for 46 % of articles and 36 % of patents from their total number worldwide. This indicates that this problem remains relevant [27].
The advantages of microbiological bioremediation include the relative cost-effectiveness of the technology, ease of use, availability of materials, and environmental friendliness of the method. The advantages are provided by the work of enzymatic systems of microorganisms. Their metabolism allows splitting oil and using oil fractions as a source of carbon and energy [28]. Bioremediation technologies are diverse, but a number of basic methods can be distinguished (see Figure).
In-situ bioremediation and the main methods belonging to this group are the most attractive since recovery takes place without the removal and transportation of contaminants. However, in-situ restoration is more suitable for the elimination of surface contamination and is not always effective when the contaminant penetrates into the deep layers of the soil [29, 30]. The ex-situ bioremediation method consists of the removal or extraction and restoration of contaminated soil [31].
There are also combined methods, the essence of which is the simultaneous or sequential application of a combination of methods. For example, in microbiological remediation, the combined method may consist of the removal of native microorganisms from contaminated soils, followed by introduction into the contaminated site after the cultivation of microorganisms in the reactor. The essence of the methods used for microbiological bioremediation and their main advantages and disadvantages are presented in Table 3.
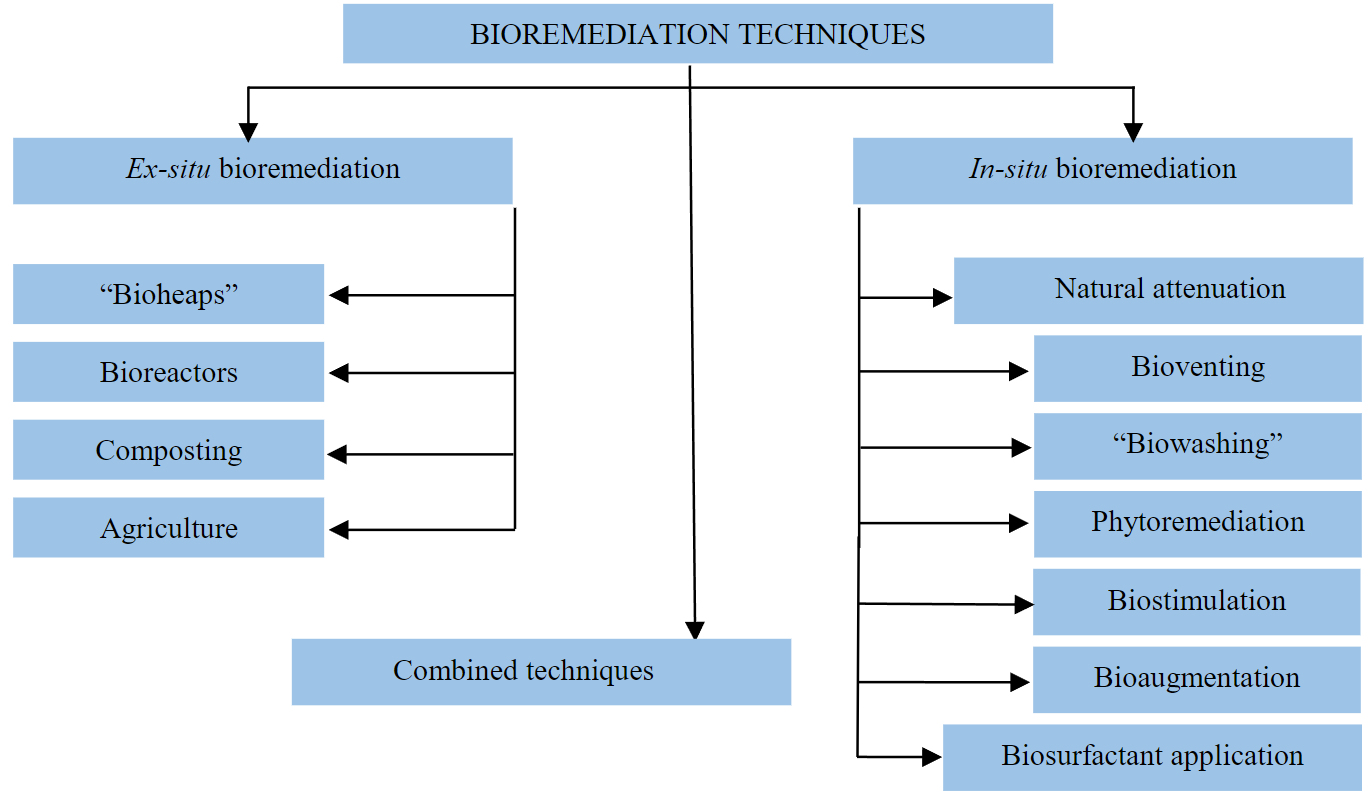
Basic techniques of bioremediation
Table 3
Basic techniques of bioremediation, their advantages and disadvantages [29, 32]
Name |
The essence of the technique |
Advantages |
Disadvantages |
Ex-situ bioremediation |
|||
“Bioheaps” |
Contaminated soil is stored above the ground in a processing area equipped with devices for aeration, temperature, and humidity control and nutrient application [32, 33] |
Prevents the spread of contaminants; requires relatively small areas; economically less costly |
Causes soil drying and volatilization rather than degradation of hydrocarbons; requires a continuous power supply |
Bioreactors |
Bioremediation technology uses a reactor to adjust the parameters of the process environment [34] |
High efficiency in both aerobic and anaerobic conditions; the ability to control all parameters of the bioprocess; genetically modified microorganisms can be used |
High price; laborious technique; different bioreactor designs may be required depending on the contaminant |
Composting |
The transformation of organic substances into humus under the action of microbial catabolic activity at elevated ambient temperatures [35] |
High biological efficiency; mature compost can be used for agricultural needs; temperature rises during the process |
Requires large areas and frequent mixing; the likelihood of leaching toxic intermediates in an event of a failed operation |
Agriculture |
Contaminated soil is evenly distributed over the surface to increase the bioavailability of contaminants [36] |
Simple, less laborious, and inexpensive technique; uses a minimum amount of equipment and technologies |
Requires large areas; reduced microbial activity (not suitable for toxic volatile contaminants); laborious technique; the likelihood of washing out contaminants |
In-situbioremediation |
|||
Bioventing |
A bioremediation strategy that uses controlled airflow to stimulate the destructive activity of local microorganisms against a contaminant [32, 37] |
Cost-effective; enhances microbial activity |
The process can be long; requires regulation of the airflow rate |
Natural attenuation |
A variety of physical, chemical, or biolo-gical processes that, under favorable |
Inexpensive and less labor intensive |
Long process; the likelihood of washing out and spreading contaminants |
“Biowashing” |
Similar to the bioventing technique, however, a controlled fluid flow is used to equalize the concentration of contaminants in the environment [32] |
Inexpensive; fewer operational dangers |
The likelihood of the spread of volatile components into the atmosphere; requires controlled water flow |
Phytoremediation |
An integrated technique that includes the use of plants for the extraction, accumulation, degradation, filtration, stabilization, and volatilization of contaminants from soil and water environments [39] |
Less expensive; environment- friendly; used for large-scale contamination; improves soil |
Long process; the likelihood of spreading toxic contaminants through the food chain; not suitable for heavily contaminated soil |
Biostimulation |
Deliberate stimulation of native microorganisms by the addition of electron acceptors and/or donors, water, or nutrients in the form of fertilizers to accelerate the |
Increases soil nutrient content and bioavailability of contaminants; promotes the development of natural microflora |
High content of nutrients (nitrogen, phosphorus) can cause algal blooms in the water; the use of surfactants can create a toxic solution in the soil |
Bioaugmentation |
Addition of highly concentrated and specialized populations of specific microorganisms to a contaminated site increases the biodegradation rate of the |
Cometabolic processes take place; high bioremediation efficiency |
Environmental parameters affect microbial growth; a number of international agreements restrict the use of introduced microorganisms and genetically modified organisms; the possibility of mutual suppression of the vital activity of microorga-nisms of various types |
Biosurfactant application |
Bioaugmentation strategy that involves |
Increased bioavailability of contaminants; biosurfactants are |
High cost and low yield of substances during synthesis; a small number of microorganisms are producers of biosurfactants |
The bacteria that carry out the biodegradation of oil in the soil belong mainly to the genera Pseudomonas, Flavobacterium, Acinetobacter, Aeromonas, Arthrobacter, and Rhodococcus [42, 43]. Fungi of the genera Alternaria, Aspergillus, Candida, Cephalosporium, Cladosporium, Fusarium, Geotrichum, Gliocladium, Mucor, Paecilomyces, Penicillium, Rhizopus, Rhodotolura, Saccharomyces, Talaromyces, Torulopsis, etc. [44-46], and microalgae Chlorella vulgaris [47, 48] also possess biodestructive ability in relation to petroleum hydrocarbons.
Bacteria are the most effective microorganisms for bioremediation due to their ability to break down almost any hydrocarbons up to the heaviest paraffins (asphalt residues) [49]. The patents describe more than 70 types of bacteria capable of metabolizing various contaminants. Pseudomonas spp., Rhodococcus spp.,and Acinetobacter spp. are the most common and universal. Most of the patents belong to authors from China, the USA, Japan, the UK, India, and Korea [27]. However, bacteria are very demanding to ambient conditions, so the most favorable for the reproduction of most of them are the temperature of 30-40 °C; the presence of oxygen (aerobic conditions) and nutrients (nitrogen, phosphorus, and iron); neutral pH. The experimental data confirm that no microorganism leads to the complete decomposition of oil. Bioremediation is more effective when carried out by complex microbial consortia, in particular, developing independently in historically contaminated territories [50, 51].
Microscopic fungi are widely used for microbiological bioremediation of soils. Fungal mycelia with many branching hyphae have a large adsorption surface [52], due to which they are able to adsorb xenobiotics (phenol, phenolic compounds, and polycyclic aromatic hydrocarbons). Unlike fungi, bacteria cannot efficiently decompose polycyclic aromatic hydrocarbons with more than four aromatic rings [53, 54]. Due to the presence of hyphae, fungi can penetrate into solid particles and contribute to loosening the soil [55]. Important factors determining the effectiveness of the use of micromycetes as oil utilizers are their active enzymatic system and high viability in conditions of oil contamination [56]. Micromycetes-destructors of petroleum hydrocarbons include at least 100 species of microscopic fungi [57]. Patent documents for bioremediation techniques (most of them from China, the USA, Japan, and Taiwan [27]) mention more than 30 species of fungi. Chrysosporium spp., Phanerochaete spp., Aspergillus spp., Acremonium spp., and Penicillium spp. are the most common.
The total number of patents in the field of microbiological bioremediation for algae is low. More than 40 species of algae are mentioned; Chlorella spp., Scenedesmus spp., and Chlamydomonas spp. are the most frequent [58]. Almost all supported patents belong to patent holders from China and the USA [27].
Bio-based products. Microbiological composition.
When developing bio-based products, special attention is paid to the following aspects: microbiological composition (active strains of bacteria, fungi, and microalgae are selected); selection of a carrier sorbent and auxiliary nutrients. Bio-based products are often developed for use in specific climatic conditions since the biodestructive activity in relation to petroleum hydrocarbons and other contaminants directly depends on the humidity and temperature characteristics when using microbiological bioremediation in-situ.
Native and introduced microorganisms
A number of modern studies demonstrate the high efficiency of the oil-destructive ability of microorganisms isolated from the soils of historically oil-contaminated territories [59]. Biostimulation is the key research method.
When using bio-based products for bioremediation, microorganisms are introduced into the soil. Bio-based products can have different compositions – microorganisms of the same genus, species, and strain, as well as consortia of microorganisms, can serve as active agents.
Monocultures
Bio-based products based on monocultures (Pseudomonas putida 36; Acinetronacter sp. НВ-1; and Pseudomonas alcaligenes Е7) were developed earlier and are significantly inferior in efficiency to bio-based products based on consortia of microorganisms.
The disadvantage of the strains is that they recycle oil at high temperatures: Rhodococcus erythropolis VKPM As-1668 (the strain is used to purify water and soil from oil contamination); Rhodococcus globerulus Н-42; Rhodococcus spp. [43]; Micrococcus luteus LER-4 [60]; and
Bacillus subtilis [61], capable of reducing the concentration of oil to 0.48 % during the three months of the summer period.
These strains degrade oil in the soil effectively under the conditions of a model experiment, are able to purify the environment from oil, and dispose of petroleum products (diesel fuel) in a wide temperature range of 8-37 °C.
Consortia
Bio-based products based on consortia of microorganisms have a higher proven
effectiveness since different types of microorganisms have biodestructive activity against different classes of organic compounds. A large number of such bio-based products have been developed:
- “Devoroil” – five types of oil-oxidizing bacteria and yeast.
- “Ecoil” – aerobic oil-oxidizing bacteria Mycobacterium, Rhodococcus, and Acinetobacter;
sucrose, polyethylene glycol, oil, and water. - “Bak-Verad” – a bacterial product from a consortium of bacterial strains of the genera Bacillus, Atherobacter, Rhodococcus, and Pseudomonas. Research results have shown that the introduction of bio-based products significantly reduces the content of petroleum products in the soil and accelerates natural destruction by a factor of 3-4 [62].
- In the study [63] strains of P. putida TPHK-1, P. aeruginosa TPHK-4, Acenitobacter sp. TPHK-3, and S. maltophilia TPHK-2, capable of growing on petroleum hydrocarbons, were isolated from areas contaminated with oil. The introduction of these hydrocarbon-decomposing strains into samples of oil-contaminated soil led to pronounced bioaugmentation [64].
- A consortium of microorganisms composed of bacterial strains belonging to the genera Pseudomonas and Microbacterium, as well as microscopic fungi of the genus Penicillium, was used in [65] to evaluate the effectiveness of oil-contaminated soil purification in the Kola North. The most effective purification was achieved in experimental variants using a consortium made up of bacteria and fungi. So, the volume of petroleum products in the soil decreased by 57 % from the initial level in 30 days, by 82 % in 120 days, and by 83 % in 370 days.
Bio-based products. Sorbents-carriers.
For effective bioremediation of oil-contaminated soils, it is necessary to develop mechanisms for introducing microorganisms. To this end, various sorbents have been tested and their impact on the purification of soils from petroleum products has been assessed. The introduction of sorbents into the soil during bioremediation has a positive effect on the activation of the oil-destructive ability of local or introduced microorganisms and on the growth of plants planted at the final stage of land reclamation. Due to sorbents, a decrease in soil hydrophobicity and an increase in their moisture capacity and porosity can be observed [66].
The cost of biological remediation of oil-contaminated soils using sorbents is 12-200 thousand euros/ha (as of 01.04.2022), depending on the type and amount of sorbent applied. The cost of mechanical methods of reclamation is several times higher [67].
Activated carbon
Activated carbon can serve as a sorbent for introducing microorganism-based products into the soil. This carrier type has been tested in the course of many modern studies [21, 68].
Treatment of oil-contaminated soil with the addition of 1-3 % of granular activated carbon during the experiment reduced the volume of oil hydrocarbons by 78-91 % in four months, which significantly exceeds the result of bioremediation in the control sample without additives – 55-65 % [21].
Activated carbon is a fairly cheap, effective, environmentally friendly sorbent that prevents the leaching of hydrocarbons, heavy metals, and other toxic mobile products into groundwater and surface water bodies [5].
Sapropel
In [69], the ability of six bacterial strains to destroy a number of petroleum products and oil on a solid mineral medium was studied: Pseudomonas sp., Pseudomonas oryzihabitans, Rhodococcus erythropolis, Pseudomonas sp., Acinetobacter guillouiae 1, and Acinetobacter guillouiae 2. Various modifications of sapropel have been selected to introduce bacterial cultures into the soil as a sorbent. It has been established that bacteria are able to attach, effectively retain on sapropel substrates, and persist during drying. According to the research results, the destruction of oil occurred more efficiently in the variant with the addition of crushed sapropel to the conta-minated soil – in seven days the oil content in the soil decreased by 65 %, which significantly exceeds the purification rates when adding pure bacterial strains. The advantage of such a carrier is its organic nature and ecological purity.
Glauconite
It is a mineral that is an aqueous aluminosilicate of iron, silica, and potassium oxide unstable. The ion-exchange properties of the mineral and the layered structure determine its high sorption activity against petroleum products. Since glauconite, when applied to the soil as part of a bio-based product, can serve not only as a sorbent carrier but also as a mineral fertilizer, its extraction and disposal are not required. As a glauconite-containing carrier, both pure glauconite and glauconite rock containing 30-80 % of glauconite can be used.
A bio-based product for biodegradation of petroleum products has been developed on the basis of glauconite [70]. It includes the association of bacteria Bacillus megaterium VKM В-396, Bacillus subtilis VKPM В-5328, Pseudomonas putida VKM В-1301, Pseudomonas putida VKPM В-5624, and Rhodococcus erythropolis VKPM AS-1269, immobilized on a glauconite-containing carrier in the amount of 108-1010 cells/g.
Zeolites
Zeolites are another group of minerals that can be used as sorbents. The fixation of microorganisms inside zeolite crystals allows them to safely tolerate exposure to direct sunlight and sudden changes in ambient temperatures, and the sorption and ion-exchange properties of the mineral provide microorganisms with additional sources of nutrition (petroleum hydrocarbons and mineral nutrition elements sorbed from the soil) necessary for the metabolism of microbial cells. This ensures high efficiency and an increase in the duration of the process of destruction of petroleum products.
The bio-based product for bioremediation of oil-contaminated soils for the climatic conditions of the Far North [71] in its composition contains a solid carrier substrate – crushed zeolitized tuff with a fraction of 1-3 mm with a zeolite content of 60-95 % and a consortium
of hydrocarbon-oxidizing microorganisms immobilized on its surface, including strains of Bacillus vallismoris VKPM В-11017, Exguobacterium mexicanum VKPM B-11011, Serratia plymuthica VKM B-2819D, and Rhodococcus sp. VKM As-2626D. The advantage of the invention is a high degree of purification of soils and soils from oil and petroleum products (87-88 %) in the cold climate of the Far North in a short period of 60 days.
Other types of carriers
Synthetic materials can be used as sorbents-carriers. Thus, polyurethane foam is a high-quality carrier for cell immobilization thanks to its chemical and physical resistance and stability [72, 73]. However, it is necessary to extract and dispose of such sorbents after the end of bioremediation.
A patented Russian invention is a composition that contains a porous carrier – foamed glassy metaphosphates and strains of microorganisms-destructors Serratia marcescens PL-1, Pseudomonas fluorescens biovar II10-1, and Acidovorax delafieldii 3-1 at a concentration of 1012 cells/g, immobilized in the pores of the carrier.
In order to optimize the removal of oil, a biological carrier has been developed for the immobilization of native hydrocarbon-destroying bacteria using peanut shell powder. Biodegradation rates after powder application increased from 26 to 61 % after 12 weeks of treatment. The use of peanut husk powder accelerated the mass transfer of water, oxygen, nutrients, and hydrocarbons and provided nutrition for microorganisms [74]. The introduction of wood chips (sawdust) into the soil with oil-destructing microorganisms significantly reduced the concentration of contaminants in the soil [75]. Studies have also been conducted with chitin or chitosan, vermiculite, etc. used as sorbents.
Bio-based products. Nutrients.
There is another aspect that needs to be taken into account when using bioremediation methods to achieve the most successful result [30]. In order to stimulate the development and support of microorganisms in the soil in concentrations sufficient for bioremediation, the presence of nutrients – nitrogen, phosphorus, potassium, and sulfur – is necessary. The biostimulation method is based on the artificial introduction of these substances into the natural environment, in which nutrients are introduced into the soil and provide aeration, which allows local microorganisms to multiply. The biostimulation method is based on the artificial introduction of these substances into the natural environment, in which nutrients are introduced into the soil and provide aeration, which allows local microorganisms to multiply. Microorganisms also use these substances to produce enzymes that break down petroleum hydrocarbons and other contaminants [76].
Complexes of mineral fertilizers are used as a source of nutrients to stimulate the vital processes of microorganisms: “Azofoska” [21], urea, etc. Bioremediation using fertilizers to increase the concentration of nutrients – nitrogen and phosphorus, necessary for the growth of microorganisms and decomposing hydrocarbons – was used to clean the coastline from oil after the Exxon Valdez spill [77].
Bio-based products. Temperature regime.
Temperature is one of the main factors influencing the intensity of biochemical transformations of organic substances under the action of the processes of vital activity of microorganisms [78]. For this reason, when selecting strains for microbiological bioremediation of soils in certain climatic conditions, it is worth considering their belonging to a group depending on the temperature optimum: psychrophiles (Pseudomonas spp., Microbacterium spp., Rhodococcus spp., Arthrobacter spp., Brevibacterium spp., and Bacillus spp.) are used most often, even in northern latitudes (topt = 10-20 °C) [65, 71, 79]; psychrotrophs (Acinetobacter spp., Pseudomonas spp.,and Rhodococcus spp., topt = 20-28 °C); mesophylls (Staphylococcus spp.,and Acinetobacter spp.) are used in mid-latitudes with other microorganisms in laboratory and field experiments (topt = 37 °C) [59]; thermophiles (some representatives of Bacillus spp., Methanobacterium spp.,and Chlamydomonas spp.) are rarely used, mainly in model or laboratory experiments (topt = 42 °C).
The study of the effectiveness of the use of psychrophilic microorganisms for cleaning soils from oil contamination in conditions of low ambient temperatures is given in the materials of many scientific studies and dissertations [80].
The results of the work [81] show the ability to utilize oil and petroleum products with a mixed culture of psychrophilic and psychrotolerant strains that actively oxidize petroleum hydrocarbons (Rhodococcus sp. As-2626D + Serratia plymuthica As-2819D + Bacillus vallismortis B-11017 + Exiguobacterium mexicanum B-l 1011), at low positive temperatures (4-10 °C). It was found that these strains, when introduced into permafrost soil at –45...–50 °C, retained viability and after thawing retained UV-oxidizing activity, which showed the possibility of their use for cleaning soils from oil contamination not only within the growing season. The use of the obtained bio-based product in winter before and after the formation of snow cover ensured the 44-62 % degree of soil purification at a 1 % level of oil contamination and 34-46 % at 5 % of contamination.
According to the results of the study [80], on the basis of a consortium of bacteria of the genera Rhodococcus and Pseudomonas, the “MicroBak” bio-based product was developed and patented for bioremediation of soils with an oil content of up to 15 % at low and moderate temperatures of 4-32 °C in the presence of up to 5 % of salt at pH of 6-8. A microbial association “ViO” was created, consisting of strains-destructors of the genera Rhodococcus, Pseudomonas, and Acinetobacter. Consortium bacteria are capable of degradation of petroleum hydrocarbons at concentrations up to 30 % in the temperature range of 4-42 °C in the presence of up to 5 % of salt at pH 4-10. The effectiveness of the prototype of the “ViO” bio-based product in field tests for cleaning the soil from oil on the territory of the Pogranichnoye field of Yamalo-Nenets Autonomous Okrug was 80 %.
The study [82] proved the high efficiency of biotechnological techniques based on the use of the psychrotolerant consortium IB ND 1 for the remediation of contaminated objects. Table 4 provides basic information about the results of a number of studies of the oil-destructive ability of strains and consortia of microorganisms.
Table 4
Results of studies of the oil-degrading ability of strains and consortia of microorganisms
Microbiological agent |
Ambient conditions, duration of an experiment |
Initial concentration of oil or oil products in the soil, g/kg |
Concentration of oil or oil products in the soil at the end of the experiment |
Pseudomonas putida 36 [20] |
20-28 °C, 5 days |
– |
20 °C: oil – decrease in concentration by 22.0 %; fuel oil М-40 – 10.2 %; kerosene – 75.0 % |
Pseudomonas alcaligenes Е7 [21] |
20-28 °C, 5 days |
– |
20 °C: oil – decrease in concentration by 63.8 %; fuel oil М-40 – by 34.2 %; kerosene – by 81.0 % |
Rhodococcus erythropolis VKPM As-1668 [22] |
28-30 °C, 1 month (laboratory experiment) |
18.8 |
In 1 month – 6.5 g/kg (degree of purification – 65.4 %) |
Micrococcus luteus LER-4 [60] |
10; 20; 30; 37 °C, |
Oil – 0.91 |
10 °C – 0.86 g/kg (5.6 %); 20 °C – 0.402 g/kg (55.8 %); |
Bacillus subtilis |
Yakutsk city, three-months field experiment (summer period) |
135.14 |
0.645 g/kg (residual oil content of 0.48 %) |
Biological product based on the “Devoroil” consortium of microorganisms [23] |
28-30 °C, 1 month |
18.8 |
1 month – 9.6 g/kg (degree of purification – 51.1 %) |
P. putida TPHK-1, |
25 °C, 10 days |
0.073-0.365 |
Initial diesel fuel concentration of 1 %: |
Rhodococcus erythropolis + |
7 days |
– |
Soil + Rhodococcus erythropolis – degree of oil |
End of Table.4 |
|||
Microbiological agent |
Ambient conditions, duration of an experiment |
Initial concentration of oil or oil products in the soil, g/kg |
Concentration of oil or oil products in the soil at the end of the experiment |
“BIOIONIT” biological pro-duct for biodegradation of oil products (Bacillus megaterium VKM В-396, Bacillus subtilis VKPM В-5328, Pseudomonas putida VKM В-1301, Pseudomonas putida VKPM В-5624, and Rhodococcus erythropolis VKPM AS-1269, immobilized |
100 ml of distilled water |
30 |
Decrease in oil concentration at different pH values: |
Consortium of hydrocarbon-oxidizing microorganisms, |
18-22 °C, 60 days
|
Cryogenic bog soil – 108.34 |
12.3 g/kg – 88.64 % |
“AVALON” biological pro-duct (foamed glassy metaphosphates and strains of microorganisms-destructors Serratia marcescens PL-1, Pseudomonas fluorescens biovar II10-1, and Acidovorax delafieldii 3-1 at a concentration of |
28 days, fuel oil |
– |
Biodestruction degree of 86.5-88.2 % |
Sorbent for immobilization of microorganisms: 0.5-1.5 mm peanut shell powder, microorganisms – native bacterial consortium [74] |
Soil contaminated with crude oil from Liaohe Oilfield, |
– |
After 12 weeks of biodegradation, the concentration |
Rhodococcus sp. As-2626D, Serratia plymuthica |
4-10 °C |
105 |
Biodegradation degree of 44-62 and 34-46 % |
“MikroBak” biological pro-duct (a consortium of bacteria of the genera Rhodococcus |
June-August, oil- |
– |
Purification degree of 80 % |
Conclusion
Contamination by crude oil and petroleum products is a global environmental problem. Various methods have been developed aimed at restoring contaminated environments. Biological ones (bioremediation) are the most promising but they are used rarely and mainly for post-treatment processes.
The main methods of bioremediation, most often used, include ex-situ methods (use of bioreactors, composting, etc.), in-situ (biostimulation, bioaugmentation, bioventing, phytoremediation, etc.). When working with oil-destructing microorganisms (microbiological remediation), combinations of these methods are most often used.
During in-situ bioremediation, various types of sorbent materials, both natural and synthetic, are used to introduce strains of microorganisms to contaminated areas: wood chips (sawdust), husks, activated carbon, sapropel, glauconite, and zeolite. The greatest efficiency is shown in works using activated carbon. Natural carriers are also promising, since, in addition to performing the main function (sorption of microorganisms), they improve the physico-mechanical and physico-chemical pro-perties of the soil.
An increase in the efficiency of microbiological remediation of contaminated soils is also achieved by introducing nutrients (nitrogen, phosphorus, sulfur, and oxygen) into the soil as part of a combined bio-based product or separately. Complex mineral fertilizers are applied to stimulate the vital processes of native soil microorganisms.
The strains of microorganisms used in microbiological remediation of oil-contaminated soils can be divided into three groups depending on the ambient temperature optimal for their vital activity: psychrophiles (topt = 10-20 °C); psychrotrophs (topt = 20-28 °C); mesophiles (topt = 37 °C); and thermophiles (topt = 42 °C). This feature of the strains must be taken into account, since highly effective thermophilic microorganisms may be unsuitable for bioremediation in the conditions of the Far North.
A number of bio-based products have been developed for the restoration of oil-contaminated soils with proven effectiveness (“Putidoil”, “Ecoil”, “Valentis”, “Avalon”, etc.). They include consortia of oil-destructing microorganisms and auxiliary substances (nitrogen, phosphorus, etc.). Strains can be placed on a sorbing material (substrates, granules, and chips). More than 70 species of bacteria (Pseudomonas spp., Rhodococcus spp., Acinetobacter spp., Microbacterium spp., etc.), at least 100 species of microscopic fungi (Chrysosporium spp., Phanerochaete spp., Aspergillus spp., Acremonium spp., Penicillium spp., etc.), and some types of microscopic algae (Chlorella spp., Scenedesmus spp., Chlamydomonas spp., etc.) are capable of oil destruction. Modern research is devoted to the study of the prospects for the use of individual strains, genera, and types of microorganisms in various conditions with different concentrations of petroleum products in the soil. Strains isolated from historically contaminated territories and prospects for their use are also being investigated. Particular attention is paid to the problem of cleaning oil-contaminated soils in the Far North, where climatic conditions limit the use of bioremediation methods. However, strains have been found capable of performing their functions with sufficient efficiency under these conditions.
A detailed study, improvement of the technology of microbiological bioremediation and the search for effective natural strains or the creation using genetic methods of new, more efficient, and less demanding to ambient conditions, strains of hydrocarbon-oxidizing microorganisms will fully realize the potential of this technology.
1. Key indicators of environmental protection. Statistical bulletin. Moscow: Federal State Statistics Service, 2021, p. 41.
2. Environmental Protection in Russia. Moscow: Rosstat, 2020, p. 113.
3. Zhirkova N.A., Kobelev B.C., Kholodenko V.P. Patent N RU2077579. Strain of bacterium Acinetobacter species used for water and soil treatment from oil and petroleum products. Publ. 20.04.1997.
4. Ermolenko Z.M., Kholodenko V.P., Chugunov V.A. Patent N RU2134723. Strain of bacterium Pseudomonas alcaligenes Е7 used for treatment of water and soil from oil and petroleum products. Publ. 20.08.1999.
5. Vlasov S.A., Safarova V.I., Safarov A.M., Krasheninnikova T.K., Krasnopevtseva N.V. Patent N RU2257409. Strain
Rhodococcus erythropolis for decomposition of petroleum and petroleum products. Publ. 27.07.2005. Bul. N 21.
6. Vlasov S.A., Krasnopevtseva N.V., Krasheninnikova T.K., Sinitsyn A.N., Ukraintsev A.D. Patent N RU2300561. Strain
Rhodococcus globerulus Н-42 for decomposition of petroleum and petroleum products. Publ. 10.06.2007. Bul. N 16.
7. Rogozina E.A., Andreeva O.A., Zharkova S.I. et. al.Comparative characteristics of domestic biological products offered for cleaning soils and soils from contamination by oil and oil products. Oil and Gas Geology. Theory and Practice. 2010. Vol. 5. N 3, p.10-28.
8. Limbakh I.Yu., Karapetyan G.O., Karapetyan K.G., Novikova I.I., Boikova I.V., Pisarev I.N., Lednev V.A. Patent N RU2181701. Biological preparation “Avalon” for cleaning environmental objects to remove petroleum and petroleum derivatives, and method of preparation thereof. Publ. 27.04.2002. Bul. N 12.
9. Moorthy K., Lavanya V., Malarvizhi A. et al.Isolation of soil bacteria for bioremediation of hydrocarbon contamination.
Biosciences, Biotechnology Research Asia. 2010. Vol. 7 (2), p. 901-906.
10. Maamar R.M., Mogadami F.S.Bioremediation of Libyan Crude Oil-Contaminated Soil under Mesophilic and Thermophilic Conditions. APCBEE Procedia. 2013. Vol. 5, p. 82-87. DOI: 10.1016/j.apcbee.2013.05.015
References
- Nikonov A.N., Potapova S.O. The Oil Industry is one of the Major Environmental Contaminants. Pozharnaya bezopasnost: problemy i perspektivy. 2018. Vol. 1. N 9, p. 666-673 (in Russian).
- Korshunova T.Yu., Loginov O.N. Oil Sludge: Conditions of the Problem in the Russian Federation and Methods to Reduce Their Negative Influence on the Environment. Ecobiotech. 2019. Vol. 2. N 1, p. 75-85 (in Russian). DOI: 10.31163/2618-964X-2019-2-1-75-85
- Pashkevich M.A., Bykova M.V. Methodology for thermal desorption treatment of local soil pollution by oil products at the facilities of the mineral resource industry. Journal of Mining Institute. 2022. Vol. 253, p. 49-60. DOI: 10.31897/PMI.2022.6
- TumanyanF., Tyutyuma N.V., Bondarenko A.N., Shcherbakova N.A. Influence of oil pollution on various types of soil. Chemistry and Technology of fuels and oils. 2017. Vol. 53. N 3, p. 369-376. DOI: 10.1007/s10553-017-0813-7
- Vasilyeva G., Kondrashina V., Ortega-Calvo J.-J.Adsorptive bioremediation of soil highly contaminated with crude oil. Science of the Total Environment. 2020. Vol. 706. N DOI: 10.1016/j.scitotenv.2019.135739
- Ushakov I.E. Detection of floods of oil products in the sea radar-tracking means. Journal of Mining Institute. 2016. 219, p. 421-427. DOI: 10.18454/PMI.2016.3.421
- Lianwen Liu, Wei Li, Weiping Song, Mingxin Guo. Remediation techniques for heavy metalcontaminated soils: principles and applicability. Science of the Total Environment. 2018. Vol. 633, p. 206-219. DOI: 1016/j.scitotenv.2018.03.161
- Khaustov A.P., Redina M.M. Transformations of Oil Damages in Geological Environment under the Effect of Liquid Substance. Gaz. Novatsii. 2013. N 10, p. 22-30 (in Russian).
- Lamichhane S., Krishna K.C.B., Sarukkalige R. Surfactant-enhanced remediation of polycyclic aromatic hydrocarbons: a review. Journal of Environ. Manag. 2017. Vol. 199, p. 46-61. DOI: 1016/j.jenvman.2017.05.037
- Sarkova O.M., Krasnopolskii V.G. Practical guide for oil spill response volunteers. URL publications/booklets/prakticheskoe-posobie-dlya-dobrovoltsev-po-likvidatsii-razlivov-nefti/ (accessed04.2022) (in Russian).
- Sagitov R.F., Shabanova S.V., Vasilewskaya S.P. et al. Lassification of Methods Localization and Liquidation of Oil-Contaminated Soil and Oil Products. Nauka i Sovremennost. 2016. N 1, p. 202-207 (in Russian). DOI: 17117/ns.2016.01.202
- Shamak D.N. Methods for eliminating oil contamination. Tezisy dokladov IV Mezhdunarodnoi nauchno-prakticheskoi konferentsii “Ekologiya i zashchita okruzhayushchei sredy”, 16-17 maya 2018, Minsk, Belarus. Belorusskii gosudarstvennyi universitet, 2018, p. 226-228 (in Russian).
- Gaur N., Flora G., Yadav M., Tiwari A. A review with recent advancements on bioremediation-based abolition of heavy metals. EnvironmentalScience: Processes & Impacts. 2014. Vol. 16. Iss. 2, p.180-193. DOI: 1039/C3EM00491K
- Kogan V.E. Inorganic and organic vitreous foam materials and prospect of environmental cleaning from oil and oil products pollutions. Journal of Mining Institute. 2016. 218, p. 331-338 (in Russian).
- Petrova T.A., Rudzish E. Types of Soil Improvers for Reclamation of Mining-Disturbed Lands. Mining Informational and Analytical Bulletin. 2021. N 4, p. 100-112. DOI: 10.25018/0236_1493_2021_4_0_100
- Petrova T.A., Rudzisha E., Alekseenko A.V. et al. Rehabilitation of Disturbed Lands with Industrial Wastewater Sludge. Minerals. 2022. Vol. 12. Iss. 3. N DOI: 10.3390/ min12030376
- Petrova T.A., Rudzisha E. Utilization of sewage sludge as an ameliorant for reclamation of technogenically disturbed lands. Journal of Mining Institute. 2021. 251, p. 767-776. DOI: 10.31897/PMI.2021.5.16
- Sobgayda N. Sorption materials for waste and natural waters clearing from oil products. Bulletin of the Kharkiv National Automobile and Road University. 2011. N 52, p. 120-124 (in Russian).
- Lekan Taofeek Popoola, Adeyinka Sikiru Yusuff.Optimization and characterization of crude oil contaminated soil bioremediation using bacteria isolates: Plant growth effect. South African Journal of Chemical Engineering. 2021. Vol. 37, p. 206-213.
DOI: 1016/j.sajce.2021.06.004 - Farag S., Soliman N.A., Abdel-Fattah Y.R. Statistical optimization of crude oil biodegradation by a local marine bacterium isolate Pseudomonas sp. sp48. Journal of Genetic Engineering and Biotechnology. 2018. Vol. 16. Iss. 2, p. 409-420.
DOI: 1016/j.jgeb.2018.01.001 - Myazin V.A., Isakova E.A., Vasilyeva G.K. The Effect of Activated Carbon on the Bioremediation Rate of the Soils Historically Contaminated With Oil Products in the Murmansk Region. Regional Environmental Issues. 2020. N 2, p. 20-26
(in Russian). DOI: 10.24411/1728-323X-2020-12020 - Finley S.D., Broadbelt L.J., Hatzimanikatis V. In silico feasibility of novel biodegradation pathways for 1, 2, 4-trichlorobenzene. BMC Systems Biology. 2010. Vol. 4. N 7. DOI: 10.1186/1752-0509-4-7
- Kumar, Kaur A. Oil spill removal by mycoremediation. Microbial Action on Hydrocarbons. 2018, p. 505-526.
DOI: 10.1007/978-981-13-1840-5_20 - Steliga T. Role of fungi in biodegradation of petroleum hydrocarbons in drill waste. Polish Journal of Environmental Studies. 2012. Vol. 2. Iss. 2, p. 471-479.
- Bulatov V., Igenbaeva N., Nanishvili O. Oil and Gas Complex Waste Products as Technological Indicator of Geoecological Condition of Russia Regions. Bulletin of Science and Practice. 2021. Vol. 7. N 8, p. 46-55 (in Russian). DOI: 10.33619/2414-2948/69/05
- Zhang, Gedalanga P.B., Mahendra S. Advances in bioremediation of 1,4-dioxane-contaminated waters. Journal of Environmental Management. 2017. Vol. 204. Part 2, p. 765-774. DOI: 10.1016/j.jenvman.2017.05.033
- Quintella C.M., Mata A.M.T., Lima L.C.P. Overview of bioremediation with technology assessment and emphasis on fungal bioremediation of oil contaminated soils. Journal of Environmental Management. Vol. 241, p. 156-166.
DOI: 1016/j.jenvman.2019.04.019 - Taki G., Islam M.N., Park Seong-Jae, Park Jeong-Hun. Optimization of operating parameters to remove and recover crude oil from contaminated soil using subcritical water extraction process. Environmental Engineering Research. 2018. Vol. 23. Iss. 2, p. 175-180. DOI: 10.4491/eer.2017.145
- Dhiraj Kumar Chaudhary, Jaisoo Kim. New insights into bioremediation strategies for oil-contaminated soil in cold environments. International Biodeterioration & Biodegradation. 2019. Vol. 142, p. 58-72. DOI: 1016/j.ibiod.2019.05.001
- Ajona, Vasanthi P. Bioremediation of petroleum contaminated soils – A review. Materials Today: Proceedings. 2021. Vol. 45. Part 7, p. 7117-7122. DOI: 10.1016/j.matpr.2021.01.949
- Sivaraman C., Ganguly A., Nikolausz M., Mutnuri S. Isolation of hydrocarbonoclastic bacteria from bilge oil contaminated water. International Journal of Environmental Science & Technology. 2011. Vol. 8, p. 461-470. DOI: 10.1007/BF03326232
- Azubuike C.C., Chikere C.B., Okpokwasili G.C. Bioremediation techniques-classification based on site of application: principles, advantages, limitations and prospects. International Biodeterioration & Biodegradation. 2016. Vol. 32, p. 58-72.
DOI: 10.1007/s11274-016-2137-x - Dias L., Ruberto L., Calabró A. et al. Hydrocarbon removal and bacterial community structure in on-site biostimulated biopile systems designed for bioremediation of diesel-contaminated Antarctic soil. Polar Biology. 2014. Vol. 38, p. 677-687.
DOI: 10.1007/s00300-014-1630-7 - Firmino P.I.M., Farias R.S., Barros A.N. et al. Understanding the anaerobic BTEX removal in continuous-flow bioreactors for ex situ bioremediation purposes. Chemical Engineering Journal. 2015. Vol. 281, p. 272-280. DOI: 10.1016/j.cej.2015.06.106
- Saum L., Jiménez M.B., Crowley D. Influence of biochar and compost on phytoremediation of oil-contaminated soil. International Journal of Phytoremediation. 2017. Vol. 20. Iss. 1, p. 54-60. DOI: 10.1080/15226514.2017.1337063
- Seung-Woo Jeong, Jongshin Jeong, Jaisoo Kim. Simple surface foam application enhances bioremediation of oil-contaminated soil in cold conditions. Journal of Hazardous Materials. 2015. Vol. 286, p. 164-170. DOI: 10.1016/j.jhazmat.2014.12.058
- Höhener P., Ponsin V. In situ vadose zone bioremediation. Current opinion in biotechnology. 2014. Vol. 27, p. 1-7.
DOI: 1016/j.copbio.2013.08.018 - Guarino C., Spada V., Sciarrillo R. Assessment of three approaches of bioremediation (Natural Attenuation, Landfarming and Bioagumentation – Assistited Landfarming) for a petroleum hydrocarbons contaminated soil. Chemosphere. 2017. Vol. 170,
10-16. DOI: 10.1016/j.chemosphere.2016.11 - Ikeura H., Kawasaki Y., Kaimi E. et al. Screening of plants for phytoremediation of oil-contaminated soil. International Journal of Phytoremediation. 2015. Vol. 18. Iss. 5, p. 460-466. DOI: 10.1080/15226514.2015.1115957
- Adams G.O., Tawari-Fufeyin P., Okoro S.E. et al. Bioremediation, biostimulation and bioaugmentation: a review. International Journal of Environmental Bioremediation & Biodegradation. 2015. Vol. 3. N 1, p. 28-39. DOI: 10.12691/ijebb-3-1-5
- Malavenda R., Rizzo C., Michaud L. et al. Biosurfactant production by Arctic and Antarctic bacteria growing on hydrocarbons. Polar Biology. 2015. Vol. 38, 1565-1574. DOI: 10.1007/s00300-015-1717-9
- Xingjian Xu, Wenming Liu, Shuhua Tian et al. Petroleum hydrocarbon-degrading bacteria for the remediation of
oil pollution under aerobic conditions: a perspective analysis. Frontiers in microbiology. 2018. Vol. 9. N 2885.
DOI: 3389/fmicb.2018.02885 - Kuyukina S., Ivshina I.B. Bioremediation of contaminated environments using Rhodococcus. Biology of Rhodococcus. Cham: Springer, 2019, p. 231-270. DOI: 10.1007/978-3-030-11461-9_9
- Al-Nasrawi H. Biodegradation of crude oil by fungi isolated from Gulf of Mexico. Journal of Bioremediation and Biodegradation. 2012. Vol. 3. Iss. 4. N 1000147. DOI: 10.4172/2155-6199.1000147
- Chaporgina A.A., Korneykova M.V., Fokina N.V. The Destructive Activity of Hydrocarbon-Oxidizing Micromycetes Isolatedfrom Soils of the Kola Peninsula. Mycology and Phytopathology. 2019. Vol. 53. N 1, p. 36-45 (in Russian).
DOI: 10.1134/s0026364819010057 - Isakova E.A., Korneikova M.V. Destructive activity of hydrocarbon-oxidizing micromycetes isolated from substrates of the coastal territories of the Barents and White Seas. Vestnik Murmanskogo gosudarstvennogo tekhnicheskogo universiteta. Vol. 24. N 2, p. 178-189 (in Russian). DOI: 10.21443/1560-9278-2021-24-2-178-189
- Korchagina Yu.S., Shchemelinina T.N. Patent N RU2764305. Method for purifying soils from petroleum contamination by the method of hydroseeding a biological mixture using microalgae Chlorella vulgaris globosa IPPAS C-2024.17.01.2022. Bul. N 2 (in Russian).
- Shchemelinina T.N., Anchugova E.M. Patent N Oil-oxidizing biopreparation, biosorbent based on it and method for its preparation. Publ.17.10.2019. Bul. N 29 (in Russian).
- Hwanhwi Lee, Seo Yeong Yun, Seokyoon Jang et al. Bioremediation of polycyclic aromatic hydrocarbons in creosote-contaminated soil by Peniophora incarnata Bioremediation Journal. 2015. Vol. 19. Iss. 1, p. 1-8. DOI: 10.1080/10889868.2014.939136
- Hesnawi M., Adbeib M.M. Effect of nutrient source on indigenous biodegradation of diesel fuel contaminated soil. APCBEE Procedia. 2013. Vol. 5, p. 557-561. DOI: 10.1016/j.apcbee.2013.05.093
- Santos H.F., Carmo F.L., Paes J.E.S. et al. Bioremediation of mangroves impacted by petroleum. Water Air Soil Pollut. 2011. Vol. 216, p. 329-350. DOI: 10.1007/s11270-010-0536-4
- Doneryan L.G., Vodyanova M.A., Tarasova Zh.E. Microscopic Soil Fungi – Bioindicators Organisms Contaminated Soil. Hygiene and Sanitation, Russian Journal. Vol. 95. N 9, p. 891-894 (in Russian). DOI: 10.1882/0016-9900-2016-9-891-894
- Leitão A. Potential of Penicillium species in the bioremediation field. International Journal of Environmental Research and Public Health. 2009. Vol. 6. Iss. 4, p. 1393-1417. DOI: 10.3390/ijerph6041393
- Maamar A., Lucchesi M.-E., Debaets S., Nguyen van Long N. et al. Highlighting the crude oil bioremediation potential of marine fungi isolated from the port of Oran (Algeria). Diversity. 2020. Vol. 12. Iss. 5. N 196. DOI: 10.3390/d12050196
- Raghukumar S. Fungi in coastal and oceanic marine ecosystems. Cham: Springer, 2017, p. 378. DOI: 10.1007/978-3-319-54304-8
- Zvolinskii V.P., Batovskaya E.K., Tumanyan A.F. The influence of oil contamination on the microbiological activity of soils. Teoreticheskie i prikladnye problemy agropromyshlennogo kompleksa. 2010. N 4, p. 39-44 (in Russian).
- Shapiro T.N., Dolnikova G.A., Nemtseva N.V. Identification and Physiological Characterization of a Consortium of Hydrocarbon-Oxidizing Bacteria of Oil and Oil Products. Journal of microbiology, epidemiology and immunobiology. N 4, p. 107-113. DOI: 10.36233/0372-9311-2018-4-107-113
- Bukharin O.V., Gogoleva O.A., Nemtseva N.V. Patent N RU Gordona terrae VKPM As-1741 strain for decomposing petroleum and petroleum products. Publ. 27.02.2009 (in Russian).
- Frank Yu.A., Nikitchuk K.L., Sapega A.A. et al. Improvement of the Efficiency of Oil-Contamined Soils Remediation in the Natural Conditions of the North Tomsk Region and the Nearby Regions by Indigenous Microorganisms Application. Bulletin of the Tomsk polytechnic university. Geo assets engineering. 2020. Vol. 331. N 9, p. 130-139 (in Russian). DOI: 10.18799/24131830/2020/9/2815
- Erofeevskaya L.A. Patent N RU Strain of the Micrococcus luteus VKM As-2627d bacteria – oil and oil products decomposer. Publ. 07.05.2019. Bul. N 13 (in Russian).
- Neustroev M.P., Tarabukina N.P., Neustroev M.M. et al. Patent N RU Method of cleaning out of frozen soil from crude oil by spore-forming bacteria Bacillus subtilis. Publ. 10.04.2012 (in Russian).
- Gamzaeva R.S. Application of the Bak-Verad biodestructor on soddy-podzolic soil contaminated with oil products. Izvestiya Sankt-Peterburgskogo gosudarstvennogo agrarnogo universiteta. 2019. N 55, p. 38-45 (in Russian).
DOI: 10.24411/2078-1318-2019-12038 - Ramadass , Megharaj M., Venkateswarlu K., Naidu R. Soil bacterial strains with heavy metal resistance and high potential in degrading diesel oil and n-alkanes. International Journal of Environmental Science and Technology. 2016. Vol. 13, p. 2863-2874. DOI: 10.1007/s13762-016-1113-1
- Ramadass K., Megharaj M., Venkateswarlu K., Naidu R. Bioavailability of weathered hydrocarbons in engine oil-contaminated soil: Impact of bioaugmentation mediated by Pseudomonas spp. on bioremediation. Science of the Total Environment. 2018. Vol. 636, p. 968-974. DOI: 10.1016/j.scitotenv.2018.04.379
- Chaporgina A.A., Korneykova M.V. Evaluation of the Microorganisms Consortium Efficiency to Cleaning Soils Contaminated by Oil Products in the Kola North Conditions. Theoretical and Applied Ecology. 2020. N 2, p. 136-142 (in Russian). DOI: 10.25750/1995-4301-2020-2-136-142
- Zinnatshina L.V., Strizhakova E.R., Danshina A.V., Vasileva G.K. Influence of sorbents on the rate of bioremediation and properties of soil contaminated with a mixture of petroleum products. Natural and EngineeringSciences. 2018. N 9 (123), p. 24-30 (in Russian).
- Slyusarevskiy A.V., Zinnatshina L.V., Vasilyeva G.K. Comparative environmental and economic analysis of methods for the remediation of oil-contaminated soils by in situ bioremediation and mechanical soil replacement. Ecology and Industry of Russia. 2018. Vol. 22. N 11, p. 40-45 (in Russian). DOI: 10.18412/1816-0395-2018-11-40-45
- Igun O.T., Meynet P., Davenport R.J., Werner D. Impacts of activated carbon amendments, added from the start or after five months, on the microbiology and outcomes of crude oil bioremediation in soil. International Biodeterioration & Biodegradation. 2019. Vol. 142, p. 1-10. DOI: 1016/j.ibiod.2019.04.008
- Nikulina A.R. Research of the Ability of Oil Destructive Bacteria to Recover Oil-Polluted Soil. Nauka bez granits. 2019. N 7 (35), p. 125-128.
- Volkov M.Yu., Ilin A.A., Kalilets A.A. Patent N RU Preparation for biodegradation of petroleum products
“Bioionit” and method of obtaining thereof. Publ. 20.12.2015. Bul. N 35 (in Russian). - Erofeevskaya L.A., Glyaznetsova Yu.S. Patent N RU25 Biopreparation for bioremediation of oil-contaminated soils for climatic conditions of Far North. Publ. 20.10.2015. Bul. N 29 (in Russian).
- Partovinia A., Rasekh B. Review of the immobilized microbial cell systems for bioremediation of petroleum hydrocarbons polluted environments. Critical Reviews in Environmental Science and Technology. 2018. Vol. 48. Iss. 7-9, p. 723-771.
DOI: 1080/10643389.2018.1439652 - Majul L., Wirth S., Levin L. High dye removal capacity of Peniophora laxitexta immobilized in a combined support based on polyurethane foam and lignocellulosic substrates. Environmental Technology. 2022. Vol. 43. Iss. 5, p. 684-695.
DOI: 1080/09593330.2020.1801851 - Yaohui Xu, Mang Lu. Bioremediation of crude oil-contaminated soil: Comparison of different biostimulation and bioaugmentation treatments. Journal of Hazardous Materials. 2010. Vol. 183. Iss. 1-3, p. 395-401. DOI: 10.1016/j.jhazmat.2010.07.038
- Alvim G.M., Pontes P.P. Aeration and sawdust application effects as structural material in the bioremediation of clayey acid soils contaminated with diesel oil. International Soil and Water Conservation Research. 2018. Vol. 6. Iss. 3, p. 253-260.
DOI: 1016/j.iswcr.2018.04.002 - Das, Chandran P. Microbial Degradation of Petroleum Hydrocarbon Contaminants: An Overview. Biotechnology Research International. 2011. Vol. 2011. N 941810. DOI: 10.4061/2011/941810
- Ivshina I.B., Kuyukina M., Krivoruchko A.V. et al. Oil spill problems and sustainable response strategies through new technologies. Environmental Science: Processes & Impacts. 2015. Vol. 17. Iss. 7, p. 1201-1219. DOI: 10.1039/c5em00070j
- Alkorta I., Epelde L., Garbisu C. Environmental parameters altered by climate change affect the activity of soil microorga-nisms involved in bioremediation. FEMS microbiology letters. 2017. Vol. 364. Iss. 19. DOI: 1093/femsle/fnx200
- Sivtsev S.I., Erofeevskaya L.A. Sanation and reclamation of oil-contaminated lands in the soil and climatic conditions of the Republic of Sakha (Yakutia). Vysshaya shkola: nauchnye issledovaniya. Part 2. Ufa: Infinity, 2019, p. 125-131 (in Russian).
- Filonov A.Е. Microbial biopreparations for cleaning the environment from oil contamination in temperate and cold climates: dis. … d-ra biol. nauk. Pushchino: Institut biokhimii i fiziologii mikroorganizmov im. G.K.Skryabina RAN, 2016, p.46 (in Russian).
- Erofeevskaya L.A. Development of a method for cleaning permafrost soils and soils from oil contamination in the natural climatic conditions of Yakutia: dis. … kand. biol. nauk. Yakutsk: Institut problem nefti i gaza Yakutskogo nauchnogo tsentra Sibirskogo otdeleniya RAN, 2018, p. 28 (in Russian).
- Smolova O.S. Bioremediation of soils contaminated with hydrocarbons using psychrotolerant microorganisms with mycostatic activity: dis. … kand. biol. nauk. Ufa: Vserossiiskii nauchno-issledovatel'skii i tekhnologicheskii institut biologicheskoi promyshlennosti, 2015, p. 24 (in Russian).