Peculiarities of rare-metal mineralization and genetic relationship of mineral associations in the eastern rim of Murzinsko-Aduysky anticlinorium (the Ural Emerald Belt)
- Ph.D. Associate Professor Ural State Mining University ▪ Orcid
Abstract
The paper presents features of the location and composition, as well as a generalization of data on the age of rare-metal mineralization developed at the deposits and occurrences of rare metals and gemstones in the eastern rim of Murzinsko-Aduysky anticlinorium, within the Ural Emerald Belt, which is a classic ore and mineralogical object and has been studied for almost two hundred years. With a significant number and variety of prospecting, research and scientific works devoted mainly to emerald-bearing mica complexes and beryl mineralization, as well as rare-metal pegmatites, scientific literature has so far lacked generalizations on the formation of numerous mineral associations and ore formations that represents a uniform genetic process in this ore district. The aim of the work is a comprehensive geological-mineralogical analysis of mineral associations of the eastern rim of Murzinsko-Aduysky anticlinorium and studying their age, formation conditions and characteristic features to determine the possibility of expanding and using the mineral resource base of the Urals through developing new prognostic and prospecting criteria for rare-metal and gemstone ore formations and creating the new devices for promising objects prospecting
Introduction
During the study of the Ural Emerald Belt (UEB) a lot of prospecting, research, and scientific works were carried out, mainly devoted to emerald-bearing mica complexes, beryl mineral-ization [1, 2], and rare-metal pegmatites.
Mineralogical analysis of the main mineral associations with rare-metal mineralization within UEB is given, their distinctive features are described and prognostic and estimation complex for the identification of new objects to determine the possibility of expanding and using the mineral re-source base of the Urals by developing new prognostic and prospecting criteria for rare-metal and gemstone mineralization is substantiated. The geological and structural features of the area were studied and the main mineral associations in the eastern frame of Murzinsko-Aduysky anticlinorium within UEB were identified, conditions of occurrence and features of the main UEB ore formations were determined, and prognostic and prospecting criteria for rare-metal and gemstone ore for-mations were developed.
Methodology
The microelement composition of minerals of the tantalite-columbite series was studied by ICP-MS method on ELAN 9000 mass spectrometer (D.V.Kiseleva, Zavaritsky Institute of Geology and Geochemistry, Ural Branch of RAS, Ekaterinburg). The analysis of dark micas was carried out by ICP-MS method on Element2 mass spectrometer by the analytical group of Yu.L.Ronkin (Zavaritsky Institute of Geology and Geochemistry, Ural Branch of RAS, Ekaterin-burg). The composition of beryls was studied with electron-probe microanalyzer Cameca SX100 (V.V.Hiller, Zavaritsky Institute of Geology and Geochemistry, Ural Branch of RAS, Ekaterin-burg).
Geological and structural position of the area. Murzinsko-Aduysky anticlinorium is located on the eastern slope of the Middle Urals and covers a small fragment of the conjugation zone of the East Ural uplift with the advanced structures of the East Ural Trough [3]. Altogether they represent a geological and tectonic structure, part of the East-Ural rare-metal metallogenic province, genetical-ly associated with the formation of Late Permian granite massifs. Nowadays this area occupies 3-5 km wide and about 200 km long, within its limits there are 38 deposits and occurrences of rare-metal, noble-metal, and gemstone raw materials. Territorially the area is located in the eastern frame of Murzinsko-Aduysky anticlinorium, with intensive development of magmatic formations, which differ both in composition and age.
Ordovician formations are represented by dunites, gabbro-norites, and serpentinites of the Ba-zhenovsky and Alapaevsky complexes, developed in the northeastern and eastern parts of the dis-trict. Middle Devonian rocks are represented by diorites of the Lesozavodsky massif, located in the center of the district. Late Permian granitic formations occupy the main part of the UEB area and are represented by granitoids of Murzinsky, Aduysky, and Malyshevsky massifs.
Murzinsky massif is located in the north of Murzinsko-Aduysky anticlinorium and composed of granites of three complexes: Yuzhakovsky migmatite-granite, Vatikhinsky adamellite (lower part), and Murzinsky granite (upper part of the massif), the age of the massif is 248-259 Ma [4, 5]. In the Murzinsky massif miarolitic pegmatites with beryl, topaz, and tourmaline of age 230-200 Ma are widespread [6]. Pegmatites formation, according to the authors, should correspond to the regressive stage of Murzinsko-Aduysky anticlinorium formation.
Significant part of UEB is formed by rocks of large polychronic multi-phase Aduysky granite pluton, which is a part of Murzinsko-Aduysky anticlinorium (Fig.1). Pluton belongs to the anatectic adamellite granitoid series of continental-collisional type [7], formed in abyssal facies (4-6 km).
In [8] four stages of Murzinsko-Aduysky granite complex formation based on Rb-Sr and Sm-Nd-datings are discussed: Early Permian stage (~260 Ma) with the formation of the main mass of the complex, Late Permian stage (~250 Ma) with the introduction of leucocratic granites in the eastern part of the Murzinsky and northern part of the Aduysky massifs, Middle-Late Triassic stage (~230 Ma) with the formation of pegmatitic leucogranites and pegmatites in the northern part of the Aduysky massif and, finally, Early Jurassic stage (~200 Ma) with the intrusion of rare-metal pegma-tites in the eastern part of the Aduysky massif.
Malyshevsky leucogranite massif is represented by three separate small bodies (6-7 x 1.5-2.5 km), deposited in hypabyssal facies and located in a chain in the Susansky fault zone along the eastern contact of the Aduysky granite massif. The massif is composed of post-collision leucocratic reddish-pink medium- and coarse-grained porphyritic granites. Malyshevsky granites differ from Aduysky granites by higher ferruginicity and radioactivity, the content of accessory thorite, etc. Malyshevsky granite massif has an age of 240-220 Ma [5].
Early works [9, 10] clearly distinguish the Triassic-Early Jurassic stage in the geodynamic histo-ry of the Ural folded Belt in the Southern and Middle Urals, which began with post-horogenic stretching and rifting and ended with locally manifested ancient Cimmerian folded-slip-thrust dislo-cations. The maximum of this stretching occurs at the Triassic-Permian boundary. This age stage (255-240 Ma) is very widely manifested and often found in metamorphic and intrusive complexes of the Urals.
At that time intensive block tectonics, crustal granitoid magmatism, and alternating in space and time processes of crustal stretching and compression due to different reasons of both global and re-gional nature take place in the area of the eastern rim of Murzinsko-Aduysky anticlinorium [9, 11]. All these processes have led to the formation of major post-collisional mineral associations and ore concentrations in the form of rare-metal (Ta-Nb-Be), gemstone (chrysoberyl, emerald, phenakite) mineralization in the eastern framing of Murzinsko-Aduysky anticlinorium.
Types of rare-metal mineralization
The main geological-genetic types of rare-metal minerali-zation are distinguished within the UEB ore district: vein, metasomatic ores, and hydrothermalites [12, 13]. Albite-microcline pegmatites with Be-Nb-Ta mineralization (Lipovy Log, Kvartalny depos-its, and a number of smaller ones) are confined to the conjugation zones of NW and NE structures in the areas of lateral uplift of the granite massif roof (Fig.2). The largest pegmatite bodies are local-ized in gentle cracks of NE strike-slip. Chemical Th-U-Pb dating of uraninite and monazite from rare-metal pegmatites of the Kvartalny deposit established the age of these vein rocks – 268-262 Ma [14].
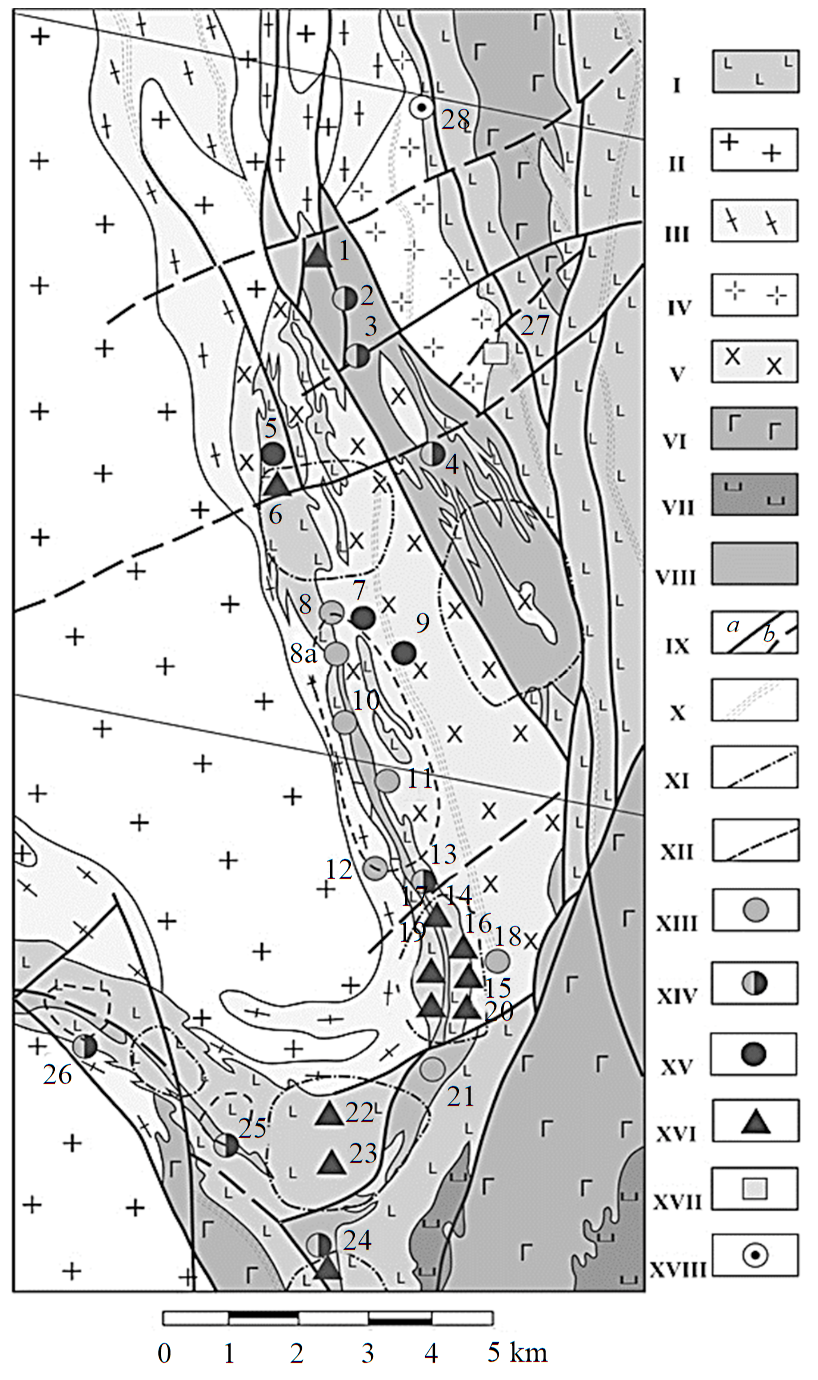
Fig.1. Schematic location of deposits and occurrences of the UEB ore district [13]: I – siliceous-basalt and rhyolite-basalt strata S1 (andesites, carbonaceous-siliceous schists, amphibolites, quartzites); II – granites; III – granite-gneiss with xenoliths of the host rocks; IV – alaskite granites; V – diorites, quartz diorites, gabbro-diorites; VI – gabbro and apogabbro amphibolites; VII – dunites, peridotites, serpentinites; VIII – calcified serpentinites, talc-carbonate, chlorite-talc rocks; IX – faults (а – established, b – supposed); X – crushing, schistose and mylonitization zones; XI – roof uplift areas; XII – roof dip areas; XIII – emerald deposits; XIV – emerald-beryllium deposits; XV – beryl deposits; XVI – rare-metal deposits; XVII – molybdenum deposits; XVIII – gold deposits; 1 – Poludensky deposit; 2 – Aulsky deposit; 3 – Malyshevky (Mariinsky) deposit; 4 – Starkovsky ore occurrence; 5 – Malinovy Klyuch ore occurrence; 6 – plot N 7; 7 – Zagrebayev point ore occurrence; 8 – Pervomaisky (Troitsky) deposit; 8а – Artem (Makarievsky) deposit; 9 – Diorite plot ore occurrence; 10 – Krupskay (Lublinsky) deposit; 11 – Sverdlovsky (Sretensky) deposit; 12 –plot N 616 ore occurrence; 13 – ore occurrence Berezovaya Roshcha; 14 – Kvartalny deposit; 15 – Lipovy Log deposit; 16 – ore occurrence Zhyla N 40; 17 – Plot N 2 deposit; 18 – ore occurrence Teply Kluch; 19 – Solnechnaya Gorka deposit; 20 – plot N 115 deposit; 21 – Cheremshansky deposit; 22 – Plot N 5 deposit; 23 – Plot N 293 deposit; 24 – Krasnoarmeysky deposit; 25 – Krasnobolotsky deposit; 26 – Ostrovnoy deposit; 27 – Yuzhno-Shammeysky deposit; 28 – Rudnichny deposit
In some cases, deposits of rare-metal granites are formed jointly (in the same ore field) with emerald-beryl ores, such as Krasnoarmeysky deposit. In pegmatites and granites, the most wide-spread process is albitization of two stages: the first stage is the formation of perthitic structures in potassium feldspars. Then albitization develops through cracks in pegmatites and mica complexes. This albitization is represented by veins composed of medium-grained albite and sometimes by a lamellar aggregate of cleavelandite. This process is associated with the formation of industrial rare-metal (Ta-Nb-Be) mineralization at UEB deposits and occurrences.
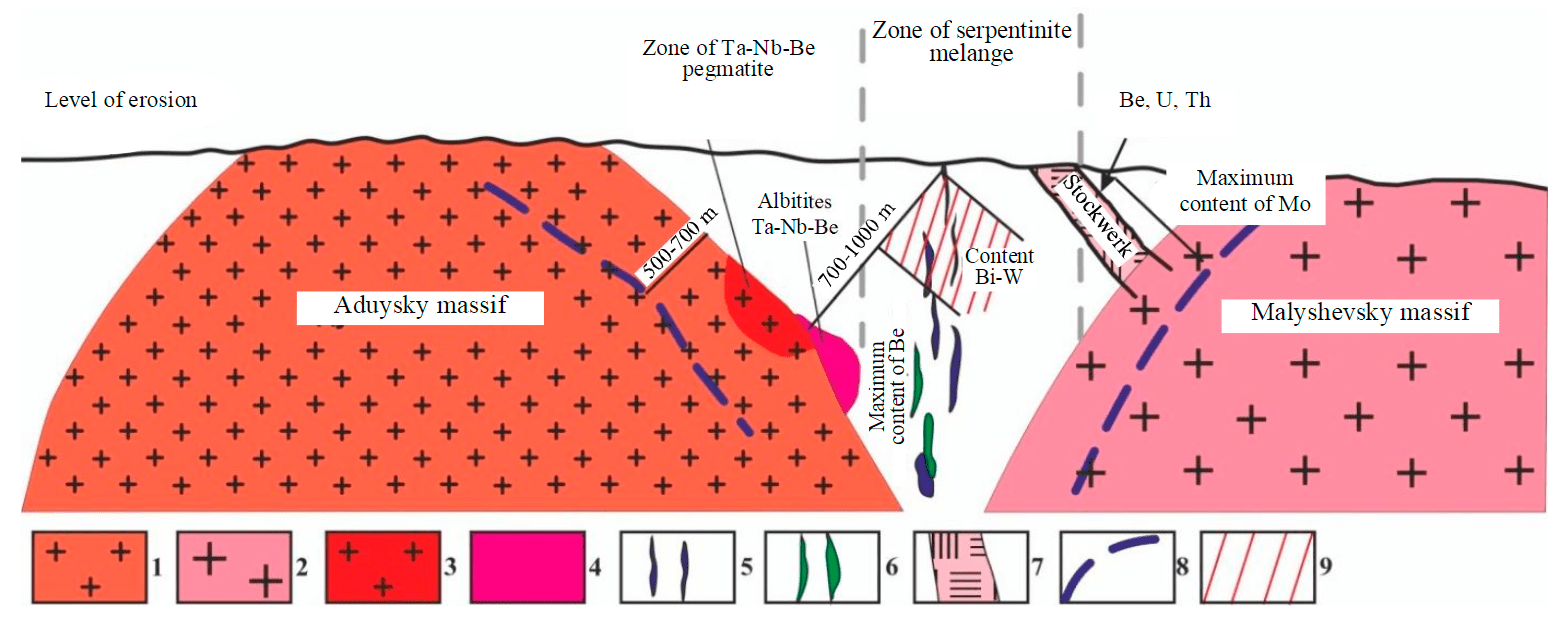
Fig.2. Scheme of mineral associations formation in the eastern exocontact of the Aduysky massif 1 – granites of the Aduysky massif; 2 – granites of the Malyshevsky massif; 3 – rare-metal pegmatites; 4 – rare-metal albitites; 5 – mica complexes; 6 – quartz-plagioclase veins; 7 – greisens; 8 – endocontact zone; 9 – Bi-W ore formation zone
The formation of rare-metal pegmatites, according to the results of early studies [15], occurred at 550-600 °C. Under the influence of high-temperature pneumatolite-hydrothermal solutions, there was the formation of vein-like bodies of albite with amphibole, which are analogs of albitite and occur in Mariinsky emerald-beryl deposit. Based on the data of mineral geothermometers [16-18] we can speak about rather high (500-550 °C) temperatures of formation of the studied vein-like bodies.
The main ore tantalum-niobium mineralization forms commercial deposits and is mainly repre-sented by minerals of the tantalite (Fe,Mn)Ta2O6 – columbite (Fe,Mn)Nb2O6 series: columbite-Fe, columbite-Mn, tantalite-Fe. The minerals occur in deposits and occurrences of rare-metal pegmatites and separate small pegmatoid bodies and quartz-plagioclase veins at emerald-beryl deposits. Crys-tals and grains of the tantalite-columbite group of minerals are often 0.1-3 mm in size, but some-times larger precipitates (up to 6-7 cm) occur. The size of short prismatic crystals is usually 0.1-0.3 mm, whereas flattened prismatic crystals are 0.2-0.4 mm. The development of late uranium-bearing hydroxycalcite-microlite [19], where UO2 content varies from 10.61 to 13.74 %, has been estab-lished for minerals of the tantalite-columbite group. Microanalysis (sample 2) showed that colum-bites are enriched in Y, Zr, Hf, Th, U, and lanthanides (La, Nd, Gd, Dy, Er, and Yb) (Table 1). At first, there is a decrease of light lanthanides with europium minimum, then quite sharp increase of heavy lanthanides (Fig.3).
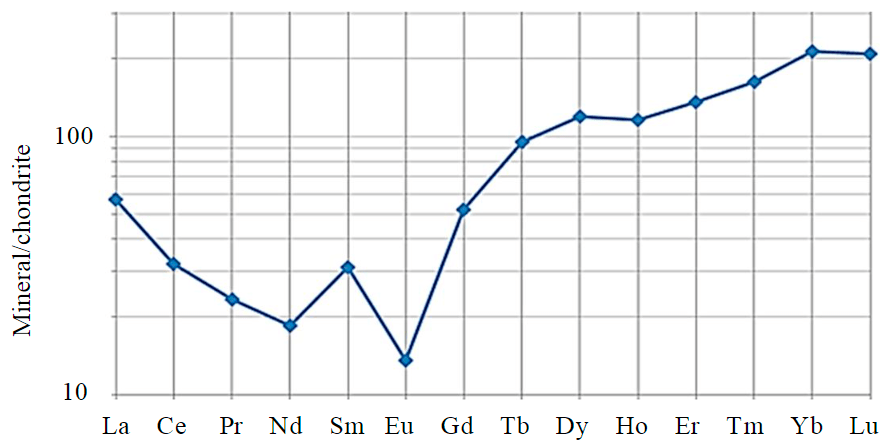
Fig.3. Distribution of rare earth elements in columbites (average of three samples, normalized on chondrite composition), Kvartalny deposit
Large emerald-beryl deposits (Mariinsky, Sverdlovsky, Krupskaya, etc.) are located above the troughs of granite massif roofs (Fig.2). Ore complex is represented by mica complexes (micaites) and beryl-quartz-plagioclase veins [20]. The most significant distribution of mica complexes with ore mineralization (rare-metal Be and gemstone) is in the eastern contact of Murzinsko-Aduysky anti-clinorium, where the world-famous emerald deposits (Mariinsky, Sverdlovsky, Krasno-bolotny) have been studied and explored. Mineral paragenesis of mica (phlogopite, fluorite, apatite, beryllium minerals, plagioclase, tourmaline, quartz, muscovite) and geochemical features (increased content of beryllium, lithium, rubidium, cesium, fluorine, boron and carbonic acid) allow refering mica complexes to greisen facies by ultrabasite of Bazhenov and Alapaev complexes (Table 2).
Table 1
Trace element composition of columbite group mineral (sample 2), ppm, Kvartalny depositElement Content Element Content Element Content
Element |
Content |
Element |
Content |
Element |
Content |
Li |
3.48 |
Zr |
4579 |
Gd |
14.4 |
Be |
1.18 |
Nb |
69612 |
Tb |
4.76 |
Sc |
23.2 |
Mo |
1.78 |
Dy |
41.0 |
Ti |
846 |
Ag |
832 |
Ho |
8.92 |
V |
3.32 |
Cd |
9.11 |
Er |
30.6 |
Cr |
2.63 |
Sn |
64.4 |
Tm |
5.70 |
Mn |
61764 |
Sb |
0.13 |
Yb |
47.1 |
Co |
0.43 |
Te |
0.45 |
Lu |
7.08 |
Ni |
0.95 |
Cs |
0.38 |
Hf |
307 |
Cu |
13.2 |
Ba |
33.7 |
Ta |
86486 |
Zn |
89.5 |
La |
18.7 |
W |
202 |
Ga |
3.75 |
Ce |
27.8 |
Tl |
0.20 |
Ge |
0.20 |
Pr |
3.03 |
Pb |
86.9 |
Rb |
6.84 |
Nd |
11.7 |
Bi |
158 |
Sr |
14.4 |
Sm |
6.30 |
Th |
176 |
Y |
249 |
Eu |
1.04 |
U |
3460 |
Table 2
Composition of dark mica from mica deposits, ppm, the Ural Emerald Belt [13]
Sampling point |
Mariinsky deposit (emerald-beryl), 4 analyses |
Krasnobolotny deposit (alexandrite-chrysoberyl) 3 analyses |
Kvartalny deposit (rare-metal pegmatites) 4 analyses |
Sverdlovsky deposit (emerald-beryl) 4 analyses |
Li |
3762 |
3974 |
5850 |
1350 |
Be |
201 |
24,8 |
40,5 |
16,4 |
Rb |
3310 |
2855 |
4580 |
2497 |
Sr |
104 |
261 |
17,7 |
10,9 |
Y |
bdl |
13,8 |
26,8 |
bdl |
Zr |
bdl |
58,2 |
97,3 |
bdl |
Nb |
21,8 |
113 |
67,7 |
41,0 |
Mo |
bdl |
bdl |
33,3 |
bdl |
Ag |
bdl |
0,09 |
0,70 |
bdl |
Sn |
20,3 |
1045 |
18,1 |
10,3 |
Cs |
457 |
464 |
249 |
314 |
Ba |
251 |
573 |
267 |
671 |
La |
0,33 |
17,1 |
15,2 |
0,06 |
Ce |
0,81 |
37,5 |
37,6 |
0,17 |
Pr |
0,03 |
4,54 |
5,01 |
bdl |
Nd |
0,24 |
18,1 |
21,4 |
bdl |
Sm |
0,04 |
3,54 |
5,34 |
0,00 |
Eu |
0,14 |
0,94 |
0,95 |
0,09 |
Gd |
0,03 |
3,45 |
5,59 |
bdl |
Tb |
bdl |
0,53 |
1,04 |
bdl |
Dy |
0,04 |
3,14 |
5,98 |
bdl |
Ho |
bdl |
0,56 |
1,13 |
bdl |
Er |
0,02 |
1,71 |
3,29 |
bdl |
Tm |
bdl |
0,22 |
1,37 |
bdl |
Yb |
0,02 |
1,47 |
2,66 |
0,00 |
Lu |
bdl |
0,20 |
0,37 |
bdl |
Ta |
16,9 |
302 |
30,8 |
9,01 |
W |
1,20 |
24,8 |
1,35 |
0,19 |
Tl |
21,4 |
18,7 |
26,8 |
17,0 |
Pb |
6,01 |
8,58 |
5,36 |
1,27 |
Th |
0,04 |
3,52 |
4,34 |
bdl |
U |
0,08 |
3,07 |
3,54 |
0,04 |
Sc |
16,7 |
10,4 |
12,2 |
8,42 |
V |
94,9 |
70,9 |
87,3 |
57,8 |
Cr |
1008 |
1257 |
34,6 |
738 |
Co |
46,8 |
3,68 |
57,6 |
55,3 |
Ni |
525 |
32,6 |
1206 |
878 |
Cu |
73,9 |
4,02 |
2,25 |
2,02 |
Zn |
608 |
252 |
664 |
555 |
Ga |
51,2 |
97,9 |
49,1 |
28,0 |
Note: bdl is below detection limit.
While the main part of granite massifs of Murzinsko-Aduysky anticlinorium is formed in the Permian period, mica complexes located in their exocontacts are of later Triassic and Jurassic ages. According to the dating data (by Rb-Sr method using the Triton thermal ionization 9-collector mass-spectrometer of VSEGEI, B.V.Belyatsky) we may distinguish three stages [21] of emerald-beryl mica formation on the UEB area: ~230 Ma (Glinsky deposit), ~205 Ma (Mariinsky deposit and Za-rechny beryl occurrence) and ~190 Ma (71 km occurrence). These studies confirm the early age de-terminations [22], where the Rb-Sr method (Triton mass spectrometer, Central Research Institute VSEGEI, B.V.Belyatsky) determined the age 206.6±1.4 Ma, which within an error agrees with the Sm-Nd age of 212±22 Ma. Based on these studies, we can conclude that the formation of mica complexes is closely related to the fluid that separated from deep melts during the Triassic-Early Jurassic stage of development (stretching and riftogenesis) of the Southern and Middle Urals.
Based on extensive factual material it has been established, that the emerald raw material is confined to the phlogopite zone and its contact with plagioclase lenses, i.e. to the central part (swells) of mica veins [23]. Mica veins over 1.2 m thick are the richest in emerald raw material, com-prising 62.5 % of the total raw material mass with a relatively low (30.1 %) frequency of occurrence. The 0.4-1.2 m thick veins are classified as moderately productive, and those less than 0.4 m thick are practically unproductive. The overwhelming (more than 95 %) amount of emerald raw material is concentrated in the mica veins; they also have the highest (13.4 ppm) content of beryl. For the main host rocks and ore complex (emerald-bearing mica and quartz-plagioclase veins with beryl) from the Mariinsky deposit new data on the magnetic susceptibility and electrical resistivity were obtained. The results of the research allowed the creation of a new express methodology for the identification of productive mica veins in boreholes and faces by simultaneous measurement of magnetic suscepti-bility and electrical resistivity of rocks during mining operations in the underground mine [24].
Table 3 shows the prognostic and prospecting criteria developed for mica veins for emeralds.
Table 3
Prognostic and prospecting criteria of mica veins for emeralds
Criteria and indicators |
Emerald (productive) veins |
Weakly mineralized and unproductive veins |
Host rocks and contacts |
Talc shales, contacts of talc shales with diorite porphyrites |
Talc shale contacts with quartzites, amphibolites and carbonaceous-siliceous shales |
Strike |
Northwest |
North East |
Dip |
Northeastern, southwest |
Northwest, southeast |
Dip angle, deg. |
> 30 |
< 30 |
Thickness, m |
0.4-1.8 |
0.1-0.4 |
Mineral composition |
Phlogopite, plagioclase, light green beryl, smoky quartz |
Phlogopite, actinolite, chlorite |
Beryllium oxide content, % |
> 0.01 |
< 0.01 |
Fluorine content, % |
> 2.0 |
< 2.0 |
Cr/Ti ratio |
> 0.3 |
< 0.3 |
Refractive index of phlogopite |
< 1.580 |
> 1.580 |
Magnetic susceptibility, SI unit |
< 2.4x10−4 |
> 2.0x10−4 |
Beryl and emerald crystals are green or bluish-green. Some crystals, along with the green color-ing (inclusions of Cr3+), have a yellowish-green coloring due to impurities Fe2+ and Fe3+. The distri-bution of coloring in crystals is uniform, zonal and spotty. The zoning is usually color – colorless green color, by light to dark green by tone, dull to bright by saturation. It is noted that the coarse zoning corresponds to different generations of emerald formation, while the fine zoning corresponds to the rhythmic fluctuations in the composition of the emerald-forming solution.
Variations in the composition of beryls, especially the Na, Li, Cs, Fe, and Mg contents, can serve as an indicator of the fractionation and evolution of the mineral and host rock [25, 26]. Mag-nesium is the main replacement cation for emeralds of almost all deposits. Emeralds from Russian (Urals), Brazilian, and Zambian deposits are referred to the so-called shale type, associated with mi-ca and pegmatites. They are characterized by high magnesium (0.70-3.10 %) and sodium (0.20-2.80 %) concentrations. In contrast, the Colombian emeralds have low contents (0.00-0.76 % Na and 0.00-0.94 % Mg) [27]. According to sources [26, 28], part of the iron in emerald can be present in trivalent form Fe3+; lithium can replace beryllium, with the charge balance being compensated by the addition of a single-valent cation in the channel. For chromium and vanadium, which determine the coloring of emerald, the following regularities are revealed – the content of Cr2O3 is usually much higher than V2O3, except for emeralds from the deposits of Muzo (Colombia) [26, 29] and Diakou (China) [30, 31].
The microprobe analysis of beryl samples from Colombia, Ural, and Brazil has shown that the main diagnostic signs of the deposit when using this method can be the following: the degree of substitution of aluminum position with impurity cations, the degree of ferruginicity and magnesiani-ty, the content of alkaline cations. Fig.4 shows the ratio of total concentration (Mg + Mn + Fe) and alkaline cations (Na+K) in emeralds from Colombia, Brazil, and the Urals. According to the results of studies [32] it was proved that the main chromophore in the Ural emeralds is Cr3+ (content 0.5-1.5 wt %).
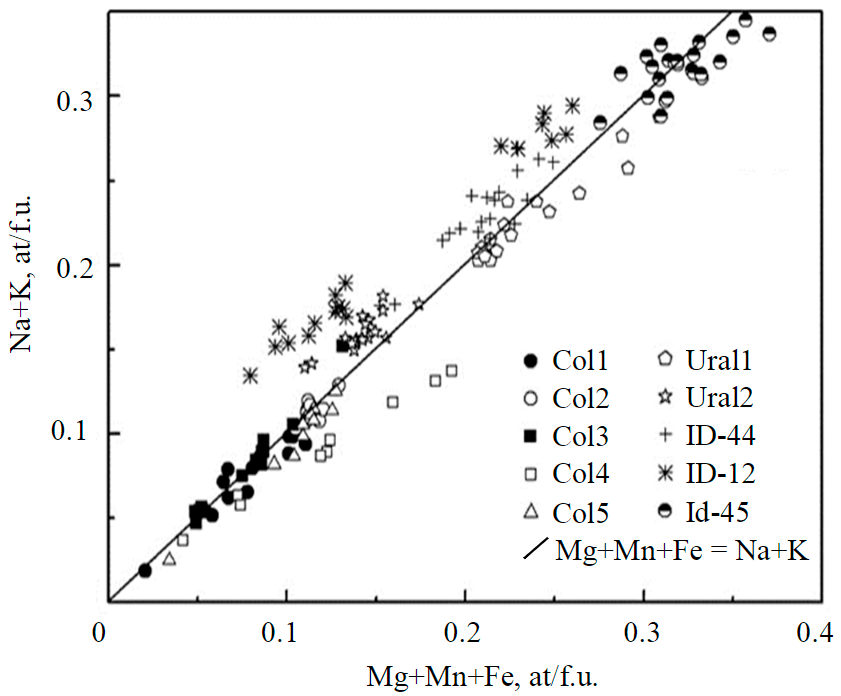
Fig.4. Ratio of total concentration of Mg+Mn+Fe and Na+K alkaline cations in investigated beryl samples Col – Colombia; Ural – Ural (Ural1 – Mariinsky, Ural2 – Sverdlovsky deposit); ID – Brazil
The coloring of medium and pale green beryls (emeralds) is also associated with high Fe2+ and Fe3+ content (0.5-0.7 wt %). Iron atoms are isomorphic in the beryl structure, replacing aluminum in the octahedral position. The main source of chromium for coloring of emeralds of the Mariinsky de-posit was chromospinelides of the Bazhenov ophiolitic complex. A change in the composition of chromospinelides from high-alumina to low-alumina is observed, which occurred during the meta-somatic processing of primary chromites under the influence of high-temperature fluorine-saturated hydrothermal solutions [32]. A similar situation with chromophores is described in emeralds from some Brazilian deposits [33, 34].
Currently, there are no identified deposits on the UEB territory, which would contain only al-exandrite-chrysoberyl mineralization in commercial concentration. Alexandrites are extracted as by-product during the extraction of emerald-beryllium middlings [1, 35]. The analysis of earlier explora-tion for beryls and emeralds in the UEB revealed that alexandrite-chrysoberyl mineralization usually crystallizes in a different geological and structural position in relation to emerald-bearing mica and has its own characteristics:
- Alexandrite-chrysoberyl mica shales with phenakite are located in the contour and often out-side the contours of emerald-bearing zones, in structures of sublatitudinal direction. This is particu-larly clear in the Sverdlovsky, Krasnobolotny, and Cheremshansky deposits.
- The localization of emerald and alexandrite mineralization varies across the deposits. Emerald-bearing mica shales bodies in blocks of commercial reserves have submeridional (strike azimuth 340-355°, dip angle 80-85°) or northwestern (strike azimuth 290-315°, dip azimuth NE, dip angle 50-80°) directions. The veins with chrysoberyl-alexandrite mineralization outside the contours of emer-ald-bearing blocks have a sublatitudinal (strike azimuth 50-80°, dip azimuth NW, dip angle 50-75°) direction.
- The composition of ore bodies with alexandrite-chrysoberyl mineralization differs from emer-ald-bearing veins with mineral diversity and forms phlogopite-chlorite veins. The chlorite content varies from 10-30 to 60-70 %.
- Chrysoberyl-alexandrite mica shales with phenakite differs from emerald-bearing mica shales in higher BeO content, according to geochemical and gamma-neutron well logging (0.05-2.00 %).
Four main mineral associations with alexandrite and chrysoberyl are observed on the UEB terri-tory: mica shale complexes; plagioclase veins in mica shales; chlorite zones with phenakite and in-termediate margarite rim from mica shale complexes; muscovite-fluorite lenses from mica shales.
In 2021, we have described a fifth, rare and unusual mineral association: alexandrite + phlogopite + emerald + allanite + Cr-spinel + fluorite.
The highest content of chrysoberyl and alexandrite crystals is recorded in mica veins, the min-eral composition of which includes, in addition to phlogopite, veins, and nodules of berylliferous margarite and elongated tourmaline crystals. The frequency of occurrence of mica veins of this para-genesis is 25.6 %, they include 37.6 % of chrysoberyl from the total volumes. Mica shale veins with phlogopite, plagioclase, beryl, and fluorite have the highest (60.1 %) specific chrysoberyl by mass [13] (Table 4).
Table 4
Prognostic and prospecting criteria of mica veins for chrysoberyl
Criteria and indicators |
Productive veins |
Weakly mineralized and unproductive veins |
Host rocks and contacts |
Talc shales, contacts of talc shales with diorite porphyrites |
Talc shale contacts with quartzites, amphibolites and carbonaceous-siliceous shales |
Strike |
North East |
Northwest |
Dip |
North-east, south-east |
Northwest |
Dip angle, deg. |
> 30 |
< 30 |
Thickness, m |
0.4-1.5 |
0.1-0.4 |
Mineral composition |
Phlogopite, Be-margarit, tourmaline, |
Phlogopite, actinolite |
Quartz-plagioclase veins were formed later than pegmatites and mica shales complexes. They are remote from the massif and confined to blocks of harder rocks (amphibolites, diorites), where they fill sublatitudinal fractures (more often gentle, less often steep) of 290-350° direction with a dip angle of 40-45°. All ore bodies are in a close genetic relationship with emerald-bearing mica shales but are located in different geological and structural settings, they seem to intersect mica veins [13]. Therefore, significant series of quartz-plagioclase veins with commercial rare-metal (Ta-Nb-Be) mineralization are found in large emerald-beryl deposits (Mariinsky, Krasnoarmeisky). In smaller occurrences, the veins are represented by single bodies. In composition they are close to rare-metal pegmatites of the area but differ by structural-textural characteristics and mineral associations of secondary minerals. Late beryllium mineralization (euclase, bavenite, bertrandite, behoite, clino-behoite, ginzburgite, bityit) reaches its maximum diversity in sublatitudinal fractures, zones of ex-tension, and tectonic crushing of quartz-plagioclase veins containing beryl, is described at the Mar-iinsky deposit. The impetus for the mass discovery of mineralization in the faces was the use of pho-toneutron sampling (gamma-neutron method, which is based on the photonuclear reaction character-ized by the absorption of γ-rays by beryllium nuclei followed by the neutron emission) both in the host rocks and in the ore bodies. Late Beryllium mineralization completes the Be-mineral formation stage in the UEB area and is an important direct mineralogical signature for rare-metal and gem-quality beryllium mineralization [36].
Discussion
The formation of the main mineral associations in the eastern frame of Murzinsko-Aduysky anticlinorium at different times is associated with the development of Triassic-Early Juras-sic riftogenesis [9, 11] and an uneven depth fluid regime. The characteristic feature of activation is also zoning of mineral associations, which is well visible not only in ore bodies but also in granites of Murzinsko-Aduysky anticlinorium. All this contributed to the formation of major post-collision min-eral associations and ore concentrations in the form of rare-metal (Ta-Nb-Be) and gemstone (chryso-beryl, emerald, and phenakite) mineralization. All of them were formed after the formation of Late Permian granite intrusions (Murzinsky, Aduysky, and Malyshevsky massifs). The earliest, with an age of 268 Ma (Late Permian), is the quartz-muscovite-feldspar mineral association, which was formed at the end of Murzinsko-Aduysky granite-gneisses complex formation. Further, the talc-actinolite-phlogopite mineral association (230-190 Ma, Triassic-Early Jurassic) was formed. The du-ration of its formation is associated with repeated exposure to fluid solutions formed after the for-mation of Late Permian granites. This fact is well manifested in the form of coarse and fine color zoning in the emerald crystal raw material. A similar pattern is observed on the known emerald-beryl ob-jects [26, 37].
The geological and structural position determines the occurrence of deposits confined to areas of uplift or dip of the roof of the Adua pluton. Complex mineralization is often observed in the are-as of kinks and transitions from one structural element to another. According to the scale and rela-tive amount of beryl and emerald reserves, quality and gem-quality of ores and raw materials, para-genetic associations, and correlation of vein and metasomatic rocks, the known objects differ signif-icantly in their geological structure, structural position, and composition of host rocks. Geological and structural features of the area, identification of the main mineral associations of promising rare-metal (Be-Nb-Ta) mineralization in the eastern framing of Murzinsko-Aduysky anticlinorium can be considered as search criteria for geological prospecting works.
Conclusions
- During the Triassic-Early Jurassic development stage of the Southern and Middle Urals (stretching and riftogenesis), various mineral associations and industrial rare-metal (Ta-Nb-Be) and gemstone (alexandrite-chrysoberyl, emerald-beryl, and phenakite) mineralization occurred at differ-ent times in the eastern rim of Murzinsko-Aduysky anticlinorium.
- The mineral formation is associated with pneumatolite-hydrothermal fluids enriched with be-ryllium, tantalum, lithium, cesium, molybdenum, and fluorine.
- Rare-metal mineralization has different types, genetically related to the formation of Late Permian granite massifs and between them, but it has also different temporal and spatial positions. The age of rare-metal pegmatites is 268-262 Ma (U-Pb dating, 28 samples); the age of mica shales and veins is 190-250 Ma (Rb-Sr method, 24 samples). Types of mineralization have different spatial position relative to granite massifs and are located in different systems of tectonic disturbances.
- Rare-metal minerals within EUB occur in pegmatite deposits and occurrences (in individual small pegmatite bodies) and quartz-plagioclase veins (in emerald-beryllium deposits).
- Important direct mineralogical indications for rare-metal and gem-quality beryllium minerali-zation are findings of secondary beryllium minerals (bavenite, bertrandite, Be-margarit, euclase). In-direct mineralogical signatures in the eastern rim of Murzinsko-Aduysky anticlinorium are findings of mica veins and complexes of predominantly phlogopite composition.
- The study of geological and structural features of the UEB area and the identification of promising rare-metal (Be-Nb-Ta) mineralization within the eastern Murzinsko-Aduysky anticlinori-um can be considered as a search criterion for exploration work aimed at finding new deposits and occurrences.
References
- Zhernakov V.I. Emerald mines: emerald, alexandrite, phenakite: ontogeny and phylogeny. Ekaterinburg: Uralskii gosudar-stvennyi gornyi universitet, 2011, p. 201 (in Russian).
- Kupriyanova I.I. On the controversial genesis of the Malyshevsky (Mariinsky) beryllium-emerald ore deposit (Middle Urals, Russia). Materialy Uralskoi letnei Mineralogicheskoi shkoly. 2003. Ekaterinburg: Uralskii gosudarstvennyi gornyi universitet, 2004, p. 37-58 (in Russian).
- Zoloev K.K., Popov B.A., Rapoport M.S. et al. Depth structure and metallogeny of mobile belts. Moscow: Nedra, 1990,
p. 191 (in Russian). - Fershtater G.B. Granitoid magmatism and continental crust formation (Uralian Orogen). Lithosrhere. 2001. N 1, p. 62-85 (in Russian).
- Fershtater G.B., Krasnobaev A.A., Beab F. et al. Geodynamic Settings and History of the Paleozoic Intrusive Magmatism of the Central and Southern Urals: Results of Zircon Dating. Geotektonics. 2007. N 6, p. 52-77 (in Russian).
- Kisin A.Yu., Koroteev V.A. Block folding and oreogenesis. Ekaterinburg: IGG UrB RAS, 2017, р. 349 (in Russian).
- Fershtater G.B., Beab F., Borodina N.S. et al. Lateral zoning, evolution and geodynamic interpretation of Ural magmatism in the light of new petrological and geochemical data. Petrologiya. 1998. Vol. 6. N 5, p. 451-477 (in Russian).
- Popov V.S., Bogatov V.I., Petrova A.Yu. et al. Age and possible sources of granites from the Murzinka – Adul block, the Central Urals: Rb-Sr and Sm-Nd isotopic evidence. Litosphere. 2003. N 4, p. 3-18 (in Russian).
- Puchkov V.N. Geology of the Urals and Cis-Urals (actual problems of stratigraphy, tectonics, geodynamics and metallogeny). Ufa: DesignPoligraphService, 2010, p. 280 (in Russian).
- Kozlov A.V., Stepanov S.Yu., Palamarchuk R.S., Minibaev A.M. Ontogenic guidelines for choice of models of the platinum ore formation in zonal clinopyroxenite-dunite massifs of the Urals. Zapiski Rossiyskogo mineralogicheskogo obshchestva (Proceedings of the Russian Mineralogical Society). 2019. Vol. 148. N 2, p. 115-130 (in Russian). DOI: 10.30695/zrmo/2019.1482.08
- Ivanov K.S. The main features of geological history (1.6-0.2 billion years) and the Urals structure: Avtoref. dis. ... d-ra
geol.-mineral. nauk. Ekaterinburg: Institut geologii i geokhimii im. akademika A.N.Zavaritskogo Uralskogo otdeleniya RAN, 1998, p. 25 (in Russian). - Kupriyanova I.I., Shpanov E.P. Beryllium deposits of Russia. Moscow: Geos, 2011, p. 353 (in Russian).
- Popov M.P. Geological and mineralogical features of rare-metal mineralization in the Eastern exocontact of the Aduysky mas-sif within the Ural Emerald Belt. Ekaterinburg: Uralskii gosudarstvennyi gornyi universitet, 2014, p. 136 (in Russian).
- Khiller V.V., Popov M.P., Erokhin Yu.V. et al. Th-U-Pb dating of granite rare-metal pegmatite in the eastern exocontact of Aduisky massif (Middle Urals). Vestnik Voronezhskogo gosudarstvennogo universiteta. Seriya: Geologiya. 2015. N 4, p. 61-65 (in Russian).
- Laskovenkov A.F. Distribution patterns of rare metals in pegmatites of Aduysky rare metal deposit and prospects for expan-sion of its raw material base: Avtoref. dis. … kand. geol.-mineral. nauk. Asbest: Iz-vo Sverdlovskogo gornogo instituta, 1982, p. 25 (in Russian).
- Otten M.T. The origin of brown hornblende in the Artfjallet gabbro and dolerites. Contributions to Mineralogy and Petrolo-gy. 1984. Vol. 86, p. 189-199.
- Vasilev E.A., Zedgenizov D.A., Klepikov I.V. The enigma of cuboid diamonds: The causes of inverse distribution of optical centers within the growth zones. Journal of Geosciences (Czech Republic). 2020. Vol. 65. Iss. 1, p. 59-70. DOI: 10.3190/jgeosci.301
- Voitekhovsky Y.L., Zakharova A.A. Petrographic structures and Hardy – Weinberg equilibrium. Journal of Mining Institute. 2020. Vol. 242, p. 133-138. DOI: 10.31897/PMI.2020.2.133
- Atencio D., Andrade M.B., Christy A.G. The pyrochlore supergroup of minerals: nomenclature. The Canadian Mineralogist. 2010. Vol. 48. N 3, p. 673-698. DOI: 10.3749/canmin.48.3.673
- Kupriyanova I.I. On the genesis of the Malyshevsk beryllium-emerald deposit (Middle Urals, Russia). Geology of Ore De-posits. 2002. Vol. 44. Iss. 4, p. 276-290.
- Bidny A.S., Baksheev I.A., Popov M.P. et al. Comparative characteristics of beryl from the Ural Emerald Belt Deposits: evi-dence from LA-ICP-MS- and IR-spectroscopy data. Moscow University Bulletin. Series 4. Geology. 2011. N 2, p. 34-41 (in Russian).
- Baksheev I.A., Kudriavtseva O.E., Beliatsky B.V. et al. Tourmaline-bearing alterations in the emerald mines, Central Urals. Part 2. Phlogopitite Uralian Geological Journal. 2003. N 4, p. 3-34.
- Zolotukhin F.F. Mariinsky (Malyshevsky) emerald deposit, Middle Urals, Asbest. St. Petersburg: Izd-vo SPbGU, 1996, p. 70 (in Russian).
- Popov M.P., Peleshko O.P., Bazhenova E.A. et al. Geophysical criteria for the separation of productive micaceous veins of the Mariinsky emerald-beryllium deposit (the Middle Urals) subprovince. News of the Ural State Mining University. 2019. Iss. 1 (53), p. 39-47. DOI: 10.21440/2307-2091-2019-1-39-47
- Uher Р., Chudík P., Bačík P., Vaculovic T. Beryl composition and evolution trends: an example from granitic pegmatites of the beryl-columbite subtype, Western Carpathians, Slovakia. Journal of Geosciences. 2010. Vol. 55. N 1, p. 69-80. DOI: 10.3190/jgeosci.060
- Groat L.A., Giuliani G., Marshall D.D. et al. Emerald deposits and occurrences: A review. Ore Geology Reviews. 2008. Vol. 34. Iss. 1-2, p. 87-112. DOI: 10.1016/J.OREGEOREV.2007.09.003
- Moroz I.I., Eliezri I.Z. Emerald chemistry from different deposits: an electron microprobe study. Australan Gemmologist. 1998. Vol. 20. N 2, p. 64-69.
- Abdel Gawad A.E., Ene A., Skublov S.G. et al. Trace Element Geochemistry and Genesis of Beryl from Wadi Nugrus, South Eastern Desert, Egypt. Minerals. 2022. Vol. 12. Iss. 2. N 206, p. 22. DOI: 10.3390/min12020206
- Banks D.A., Giuliani G., Yardley B.W.D. et al. Emerald mineralisation in Colombia: fluid chemistry and the role of brine mixing. Mineralium Deposita. 2000. Vol. 35. N 8, p. 699-713. DOI: 10.1007/s001260050273
- Zhang S.T., Feng M.G., Wang H.Q. et al. Geological features and genesis of emerald deposits in the Malipo County of Yun-nan Province, China. Geological Science Technology Information. 1999. Vol. 18, p. 50-54.
- Schwarz D., Giuliani G. Emerald deposits – A review. Australian Gemmologist. 2001. Vol. 21, p. 17-23.
- Popov M.P., Sorokina E.S., Kononkova N.N. et al. New data on genetic relationship of beryl and chrysoberyl chromophores from the Ural emerald mines with chromospinelides of the Bazhenov ophiolite complex. Doklady Akademii nauk. 2019. Vol. 486. N 6, p. 699-703 (in Russian). DOI: 10.31857/S0869-56524866699-703
- Gavrilenko E.V., Gaidamako I.M., Gavrilenko V.V. Peculiarities of redistribution of chromium in metasomatic growth of emerald crystals in metahyperbasite rocks. Zapiski Rossiiskogo mineralogicheskogo obshchestva. 1999. Vol. 128. N 2, p. 66-71
(in Russian). - Giuliani G., Silva L.J.H.D., Couto P. Origin of emerald deposits of Brazil. Mineralium Deposita. 1990. Vol. 25. Iss. 1,
p. 57-64. DOI: 10.1007/BF03326384 - Kievlenko E.Y. Geology gems. USA, CO, Littleton: Ocean Pictures Ltd., 2003, p. 468.
- Popov M.P. Mineralogical signs of rare-metal and semi-precious ore mineralization in the Murzinskaya-Aduyskaya beryllium (gemstone) subprovince. News of the Ural State Mining University. 2016. Iss. 3 (43), p. 54-58. DOI: 10.21440/2307-2091-2016-3-54-63
- Zwaan J.C., Seifert A.V., Vrána S. et al. Emeralds from the Kafubu area, Zambia. Gems and Gemology. 2005. Vol. 41. N 2, p. 2-34. DOI: 10.5741/GEMS.41.2.116