On the presence of the postmagmatic stage of diamond formation in kimberlites
Abstract
On nowadays multiphase and the facies heterogeneity of the formations are distinguished at the study of kimberlite pipes. Most researchers associate the formation of diamonds only with the mantle source. To date, satellite minerals with specific compositions associated with kimberlite diamonds have been identified as deep mantle diamond association. They are extracted from the concentrate of the kimberlites heavy fraction and may reflect the diamond grade of the pipe. For some minerals in the diamond association, however, they can not be reliable. Some researchers also revealed shallow diamond associations, related to the formation of serpentine, calcite, apatite, and phlogopite. There is recent data on the formation of diamonds in rocks of the oceanic crust. In the last years microdiamonds were identified in chromites of the oceanic crust in association with antigorite formed at 350-650 °C and 0.1-1.6 GPa. As a result, the authors established a postmagmatic kimberlitic stage of diamond formation associated with secondary mineral associations based on the experimental and mineralogical data for the conditions of the shallow upper mantle and crust. Mineralogical and petrographic studies of Angolan kimberlite pipe show that antigorite is the indicator mineral of this stage.
Introduction
Currently, most diamonds are found in volcanogenic rocks – kimberlites and lamproites, containing inclusions of the deep mantle and crustal rocks. Half a century of studying kimberlites and the diamonds themselves enabled to obtain a wide range of their petrographic, mineralogical, and geochemical characteristics. However, despite the long period of kimberlites' systematic study, many aspects of their geology, genesis, and composition remain unresolved, poorly understood, or controversial. Currently, most researchers attribute diamondformation only to the mantle. In recent years there has been evidence of diamond formation in volcanoes [1, 2], serpentinites, and chromites, being the rocks of the oceanic crust [3-5]. The author's previous work [5] showed the possibility of nano-diamond formation in serpentinites in equilibrium with lizardite at temperatures of 150-300 °C and a pressure of about 2 kbar. Micro-diamonds have been discovered in chromite in equilibrium with antigorite at temperatures of 350-650 °C and pressures of 0.1-1.6 GPa [3, 4]. At the same time, high-pressure minerals were not found in these rocks. As a result, E.M.Galimov and F.V.Kaminsky concluded that diamonds could be synthesized as a metastable phase at relatively shallow depths at low temperatures (500-700 °C) and pressures [1].
Methodology. The study of kimberlite bodies revealed the multiphase nature of kimberlite volcanism and the facies heterogeneity of formations that make up kimberlite pipes [6]. For kimberlites, there are currently two main deep diamond-forming environments: peridotite (~65 %) and eclogite (~33 %), and, to a lesser extent, an intermediate one associated with the mantle [7, 8]. Satellite minerals with specific compositions associated with kimberlite diamonds have been identified and grouped in a so-called diamond association, which includes garnet, chromospinelide, picroilmenite, olivine, and pyroxenes. They are extracted from the concentrate of the kimberlites' heavy fraction and may reflect the degree of their potential diamond content. These criteria are empirical and based on large statistical samples. For some minerals in the diamond association, however, they can not be reliable. However, several researchers in addition to deep diamond associations also revealed shallow diamond ones associated with the formation of Ti-bearing chromite, serpentine, calcite, apatite, and phlogopite from kimberlite [9]. It is confirmed by the fact that the diamonds absolute age varies from the ancient mantle to the kimberlite age [6, 10]. At the same time, diamonds with eclogite paragenesis are younger than those with peridotite [11]. Studies of the physical properties of diamonds suggest a zonal structure consisting of core, rim, and intermediate regions [12].
Discussion
Organic and carbon formation in ultramafic serpentinites and kimberlites
The widespread occurrence of injection-metasomatic and superimposed post-magmatic processes in the pipes causes the spread of epigenetic structures that mask the original image of the rocks. Epigenetic inclusions have been found in many diamonds as fracture filling and primary phase replacement products [13]. They include serpentine, calcite, dolomite, amphibole, acmite, graphite, hematite, magnetite, kaolinite, perovskite, Mn-ilmenite, sulfides, xenotime, goethite, apatite, mica (phlogopite, biotite), microcline, sellaite, spinel, and talc [14-16]. Serpentine has been identified on more than one occasion in diamonds as an epigenetic mineral. S.B.Talnikova [17] described a mineral association of serpentine-chromite-calcite-sulfides in Yakutsk diamond from Udachnaya pipe in the absence of fractures on the diamond surface. The author believes that this association is protogenetic and was captured by a diamond from kimberlite during its growth in the Earth's crust. Three main modifications of serpentine are known: lizardite, chrysotile, and antigorite [18]. Experimental studies show that antigorite can be stable up to pressures of 60 kbar [19]. Serpentinization processes can produce reduced hydrogen and hydrocarbon fluids with organic matter. This is possible by the reaction of olivine hydration with the formation of serpentine, magnetite, and hydrogen [5]:
3Fe2SiO4 + 3Mg2SiO4+ 6H2O → 2Mg3Si2O5[OH]4 + 2Fe3O4+ 2H2 + 2SiO2.
The products of this reaction (serpentine and magnetite) have been noted in epigenetic inclusions in diamonds [20]. The reaction can lead to the formation of hydrocarbons according to the well-known Fischer – Tropsch (FTT) reactions:
nCO2 + [3n + 1]H2 → CnH[2n+2] + 2nH2O,
where 1 < = n < + ∞.
As a result of these reactions, the formation of various organic substances is possible [21]. Carbon dioxide reacts with H2, giving rise to CO, which binds to the catalyst surface to form carbonyl groups (C=O). Carbonyl groups are reduced to carbide and then to methylene groups (=CH2), which in some cases become methyl groups (−CH3). These processes can produce methanol and alcohol. Methanol synthesis is possible at 320-400 °C and 100-300 atm according to the reaction
CO2 + 3H2 → CH3OH + H2O.
Further, ethyl alcohol can be synthesized from methanol by the following reaction:
CH3OH + CO + 2H2 → C2H5OH + H2.
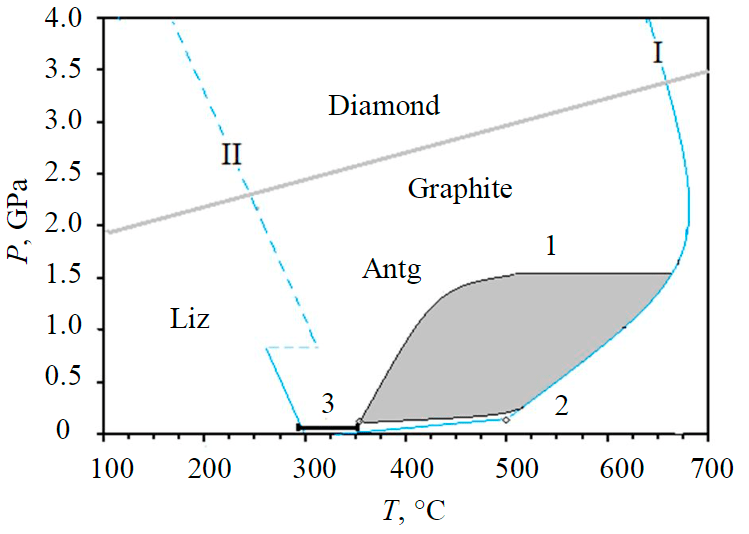
Fig.1. Temperature and pressure range of antigorite stability I – the upper limit of antigorite stability; II – limits of antigorite-lizardite equilibrium according to [18, 19]; 1 – the region of micro-diamond formation in chromites [3, 4]; 2, 3 – PT parameters of synthesis and growth of diamonds from organic mixtures [12, 22]
The formation of nano- and micro-diamonds from alcohol at 500 °C and 0.1 GPa [22], as well as the growth of diamonds on seeds at temperatures of 100–350 °C and pressures of 100-400 atm, from organics, are known [23] (Fig.1). Serpentinization produces nano- and micron-sized diamonds in the temperature range of 150-650 °C and pressures of 0.1-1.6 GPa [3-5]. Epigenetic graphite, found in diamond fractures, can also be formed by the serpentinization of olivine in kimberlites under reduced conditions [24]. Thus, serpentinization and other types of secondary alterations in kimberlites can lead to the formation of nano- and micro-diamonds, as well as to diamond growth on nucleated mantle crystals in conditions of shallow upper mantle and crust by reactions like [4, 5]:
CH4 + CO2 → 2C + 2H2O.
On the other hand, these processes can also lead to the dissolution of diamonds, for example, upon contact of reduced hydrogen fluids with crystals. Serpentinite diamonds have lighter carbon isotopes than mantle diamonds, so nano-diamonds from the serpentinites of Sicily have δ13C = −29 ‰ [5].
Organic matter is noted in diamonds from kimberlites. A wide range of organics has been found in the fluid inclusions of diamonds from the northeastern Siberian platform and Ural placers, including various hydrocarbons, alcohols, carboxylic acids, ketones, etc. [25, 26]. For these diamonds with eclogite and uncertain paragenesis types, carbon isotopic values with δ13 less than −20 ‰ have been noted. In [27] studied the organic matter impurities, nitrogen concentrations, and its aggregated form were studied by infrared spectroscopy in diamond crystals of the Yubileinaya (Yakutia) and Juína (Brazil) pipes. The crystals of both sites contain graphite impurities in the rims. For the Yakutian diamond, there is a trend towards a decrease in the aggregated form of nitrogen (% Ng) from the core to the rim regardless of the total nitrogen concentration, which corresponds to a decrease in the crystallization temperature. Infrared spectroscopy shows the presence of organic substances in the form of methylene groups CH2 and methyl CH3 saturated hydrocarbons in the rim. The diamond from the Juína area contains high concentrations of fully aggregated nitrogen in the core (about 1000 ppm) and relicts of organic matter in the rim. Organic matter is found in both nitrogen-rich and nitrogen-poor areas of the crystal. These data, combined with the relatively light carbon isotope composition of the studied diamonds (−9.6 and −22.0 ‰ δ13C, respectively), mainly show an increase in organic matter content in the late stage of diamond formation with decreasing temperature.
Mineralogical and petrographic features of the Catoca kimberlite pipe
A detailed mineralogical, petrographic and structural study of the Catoca pipe (Angola) has shown a correlation between serpentinization processes and the diamond grade of the rocks [28]. In this kimberlite pipe, the diamond association minerals, secondary mineralization, and the horizontal distribution of diamond concentrations were investigated. The main minerals of the heavy fraction of the pipe kimberlites are pyrope, chromium diopside, chromium spinel, and picroilmenite. Pyrope is constantly present in samples, but inferior in quantity to ilmenite. It is recorded both as individual grains of a predominantly isometric, less often angular shape, sometimes fragments, and as aggregates with pyroxene. The size ranges from a tenth of a millimeter to 3-5 mm, rarely more. The surface is mostly matt, sometimes with relics of a kelyphite rim. A smooth surface is more common for garnet grains from volcanogenic-sedimentary and epiclastic rocks. and epiclastic rocks. The composition of the pyrope varies significantly, with lherzolitic associations being the most widespread (Fig.2).
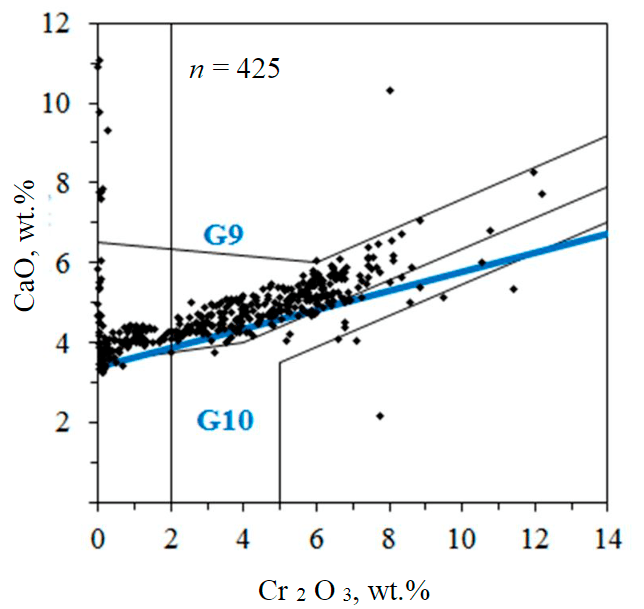
Fig.2. Composition of pyrope from the kimberlites of the Catoca pipe and combined diagram by N.V.Sobolev [7] and J.J.Gurney [29-31]; G9 – lherzolitic garnets; G10 – harzburgitic garnets
Monoclinic pyroxenes, mainly chrome diopside, are found in significant quantities in the kimberlitic rocks of the Catoca pipe. Most often they have prismatic, sometimes angular forms. The size is 3-5 mm or more along the long axis, with occasional individuals of 10-12 mm in length. Chrome diopside with a chromium content of more than 2 wt.% has been observed, which with increased Na2O content characterizes the presence of kosmochlor component (NaCrSi2O6). Similar pyroxenes are characteristic of pyrope-chrome diopside-chrome spinel intergrowths from diamond-bearing pipes and correspond to the coesite depth facies. For other varieties
of monoclinic pyroxenes, characterized by the absence of chromium oxide, the increased Al2O3 and Na2O concentrations with a decrease of calcium and magnesium oxides share characterizes the presence of jadeite component, which brings them close to omphacite from magnesian-ferrous eclogites. Clinopyroxene intergrowths with biotite and hornblende have also been recorded. In addition to the noted indicator minerals of kimberlites, chrome spinels occur occasionally. They are usually part of the matrix, morphologically represented by isometric grains and octahedral crystals with flattened tops. The surface is mostly matt, sometimes with clear signs of magmatic corrosion. A characteristic feature of chromium spinelides is their low alumina content (Al2O3 less than 6 wt.%). Their composition is similar to that of high-chromium spinelides of the lherzolitic paragenesis, with a slightly higher titanium content. Most of the studied spinels from kimberlites of the Сatoca pipe belong to the group of intergrowths with garnets and clinopyroxenes.
The most characteristic mineral in the kimberlitic rocks of the Catoca pipe is picroilmenite, which in some cases exceeds 5 vol.%, corresponding to the level of the rock-forming mineral. It is represented by grains of isometric and isometric-angular shape with smoothed edges. In some cases crystals are elongated. The size of the picroilmenite aggregates varies from a tenth to 5-8 mm, sometimes up to 12-15 mm. The surface in kimberlite breccias and tuff breccias is matted and leucoxenized, sometimes cavernous. In contrast, in volcanogenic-sedimentary and epiclastic formations, the surface is usually shiny, smooth, and pitch-black colored.
Picroilmenite is one of the minerals that react sensitively to changes in the composition of the crystallization environment. The authors [32] point out four stages of Ilm macro-, megacrysts formation: asthenospheric, lithospheric, diatremic, and subsurface. The final, fourth crystallization stage is represented by the Ilm of the groundmass. Its composition in the Mir pipe is characterized by a high MgO content (up to 15 %) and a low Al2O3 content (generally less than 0.3 %). The researchers [32] do not exclude that this stage coincides with the beginning of the late magmatic serpentinization of kimberlites. The diagnostic diagrams (Fig.3, a-c) show that the Catoca picroilmenites with an Al2O3 content not exceeding 0.3 % belong mainly to the kimberlite type and provide good preservation of diamond crystals. The calculated temperatures of their formation mainly range from 330 to 650 °C (Fig.3, d).
Secondary mineral formation in the kimberlites of the Catoca pipe is mainly represented by serpentine replacing olivine and clinopyroxene, as well as by minerals formed mainly in post-magmatic and hydrothermal conditions (smectite, mica, carbonates, and saponite). Mica is confined to kimberlite composed of serpentine group minerals. In comparison to other rock-forming minerals, mica is the is the most stable in a low-thermal process. Under weathering conditions, they are transformed into other layered silicates. According to the available results, the following transformations take place during mica destruction in this tube: phlogopite → vermiculite → saponite. Smectites are characterized by a variable composition, and some of them, in addition to magnesium, iron, and aluminum, also contain chromium, the presence of which has been detected and confirmed by X-ray diffraction.
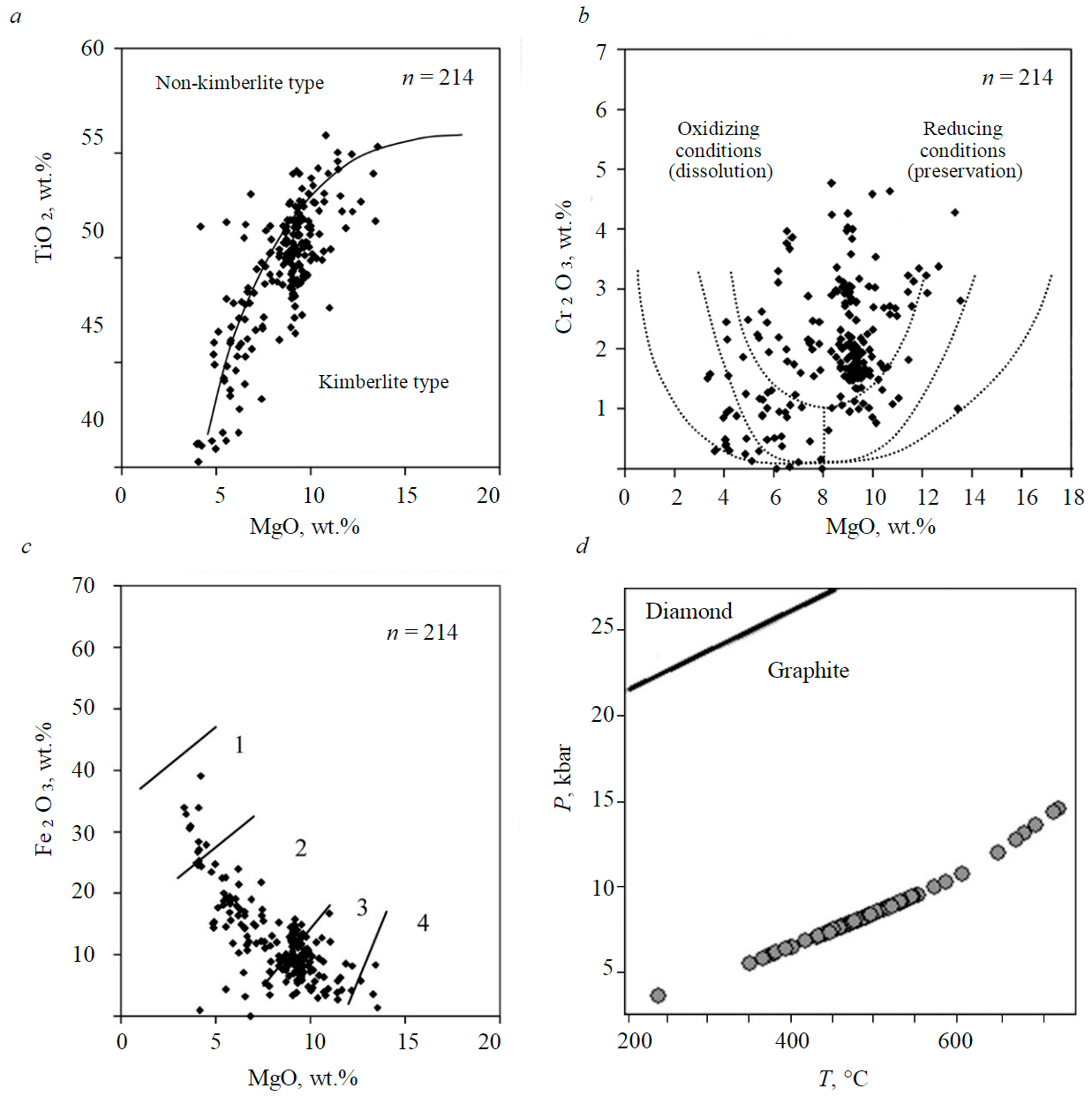
Fig.3. Characteristics of composition and PT-parameters of picroilmenites from the Catoca kimberlite pipe in the diagrams: a – Vaganova et al. [33], Wyatt et al. [34]; b – Moore [35], Schultze et al. [36] with Haggerty parabola [37]; c – Gurney et al. [31] with diamond preservation index fields (1 – diamonds were not preserved; 2 – poorly preserved; 3 – moderately preserved; 4 – good preserved); d – calculation of temperatures according to the Ashchepkov ilmenite thermometer [38]; pressure is assumed in accordance with the paleogeotherm of 50 MW/m2 according to Hasterok and Chapman [39]
The distribution of serpentine group minerals has been studied in reference boreholes in the Catoca pipe [28]. The main variety here is pseudo-isometric lamellar lizardite (1T). This mineral is contained in rocks either as an individual component, or its layers are included in an ordered lizardite-saponite mixed-layer formation [40] formed under certain petrophysical properties of rocks and hydrogeochemical features of the mineral formation medium. Antigorite and chrysotile are also recorded in certain quantities. Fibrous serpentine was not visually detected. In the lower horizons of the geological sections of the pipe, kimberlite rocks are almost completely composed of serpentine minerals. Serpentine refers to minerals of the 1:1 type. Its crystal structure consists of idealized Si-O-tetrahedral and Mg-OH-octahedral layers with possible partial replacement of Si by Fe3+ and especially Mg by Fe2+ and Fe3+, including also some other elements. These layers are interconnected by hydrogen bonding with a c-axis period of ~7.30 Å during transformation with almost no or only small amounts of mica in the kimberlite.
At the early stages of serpentine transformation, mainly sequentially transforming phases of saponite from ferro to oxyferri forms appear, through intermediate ones enriched first in Mg(Fe-Mg) and then in Fe(Mg-Fe). In some cases, the serpentine alteration, present in the rocks under study mainly in the form of lizardite and sometimes clinochrysotile, is accompanied by the emergence of a metastable ordered mixed-layered lizardite-saponite formation. Subsequently, the general trend of serpentine transformation products is the formation of nontronite-like phases. Judging by the ratio of reflections with a value of ~ 7.30 Å for serpentine and ~ 12-15 Å or ~ 18 Å for saponite on the diffraction curves of both air-dry rock samples, and at saturation of samples with organic fillers, in particular glycerol, the content of swelling mineral at this stage of kimberlite changes is minor. This shows that only partial generation of saponite occurs due to serpentine decomposition products in the kimberlites of the Catoca pipe.
A significant amount of Mg is removed from the altering rock due to the lack of Si in the mineral formation system of considered kimberlites, capable to bind Mg in silicate layered saponite structure.
At the analyzing the distribution model of serpentine group minerals in the volume of the pipe, its clear association with kimberlites of the diatreme facies and the complete absence of crater facies in the rocks are noted. At the upper horizons, serpentine is contained in kimberlite breccias of a ring structure with maximum values in the western and southwestern parts of the pipe. Its content decreases slightly with depth and is characterized by an almost uniform distribution in the groundmass, except for the 650 m horizon, where in the western part of the deposit the amount of serpentine does not exceed 10 %. The distribution of diamond content across the explored horizons of the Catoca pipe shows a similar trend: significant concentrations are also confined to rocks of the ring structure and diatreme facies.
Conclusions
The analysis of the secondary mineralogical features of the Catoca pipe showed correlations between minerals of the serpentine group and the diamond content in the rocks. Significant Spearman correlations (r[s] = 0,33) were obtained only for antigorite, which exceeds the correlation degree for pyrope (see table). The observed correlations are significant at p < 0.05000. The relationship between diamond content and serpentine could be explained by the fact that serpentine is a product of the alteration of olivine, a typical diamond satellite mineral. Zinchuk et al. [40] have shown that serpentinization of olivine-bearing rocks occurs mainly at temperatures not higher than 500 °C. At maximum temperatures olivine is replaced by antigorite, at lower temperatures by lizardite, and at the end, chrysotile may also appear. In the case of the association of diamonds with olivine, the diamond content should have correlated as closely as possible with the total serpentine content.
Spearman's rank correlations
Correlation parameters |
Number of observations |
Spearman Method – R |
t(N-2) |
p |
Diamond content and serpentine |
59 |
0.04 |
0.33 |
0.75 |
Diamond content and antigorite |
59 |
0.33 |
2.63 |
0.01 |
Diamond content and lizardite |
59 |
−0.09 |
−0.67 |
0.51 |
Diamond content and Crsp |
32 |
0.10 |
0.52 |
0.60 |
Diamond Content and Prp |
32 |
0.27 |
1.53 |
0.14 |
Diamond content and Plim |
32 |
−0.24 |
−1.35 |
0.19 |
Diamond Content and Crdi |
32 |
−0.03 |
−0.18 |
0.86 |
However, the maximum correlation has been established with antigorite, the highest temperature, pressure serpentine phase, indicating that diamonds can form with it. Based on the serpentinite phase stability diagram and experiments on the formation and build-up of diamonds from organic mixtures (see Fig.1), we can conclude that diamond growth associated with secondary processes of antigorite formation in the temperature range 300-650 °С. It took place on the late stages of kimberlite formation. At this stage, the composition of fluids varies from reduced to oxidized, and various organic substances were formed. Diamond growth here occurred mainly on existing crystals from the fluid phase, with the participation of hydrocarbons, alcohols, and carboxylic acids formed under these conditions and fixed in diamond inclusions. The evidence of this is the increased content of organic matter at a later stage of diamond formation [27] and the presence of hydrocarbons, alcohols, and other organics in the gas-liquid inclusions of diamonds from the north-eastern Siberian platform, the Urals, and Africa. The range of temperatures and pressures of antigorite stability corresponds to the formation conditions of organics (alcohols and HC) for diamond synthesis, conditions of synthesis and build-up of diamonds from organic mixtures [22, 23], and formation of micro-diamonds during serpentinization [3, 4].
Conclusions
Based on these facts, a post-magmatic hydrothermal kimberlite stage of diamond formation can be distinguished. It is possible at the conditions of shallow upper mantle and crust and associated with the secondary mineral formation on the primary minerals of kimberlites and xenoliths. For this stage, antigorite is identified as an indicator mineral.
References
- Galimov E.M., Kaminsky F.V. Diamond in the Oceanic Lithosphere. Volcanic Diamonds And Diamonds In Ophiolites. Geochemistry International. 2021. Vol. 59. N 1. DOI: 10.1134/S0016702921010043
- Galimov E.M., Sevast'yanov V.S., Karpov G.A. et al. Microcrystalline diamonds in the oceanic lithosphere and their possible nature. Doklady Akademii nauk. 2016. Vol. 469. N 1, p. 61-64 (in Russian).
- Farre-de-Pablo J., Proenza J.A., Gonzalez-Jimenez J.M. et al. A shallow origin for diamonds in ophiolitic chromitites. Geology. 2018. Vol. 47. N 1, p. 75-78. DOI: 10.1130/G45640.1
- Pujol-Sola N., Garcia-Casco A., Proenza J.A. et al. Diamond forms during low pressure serpentinisation of oceanic lithosphere. Geochemical Perspective Letters. 2020. Vol. 15, p. 19-24. DOI: 10.7185/geochemlet.2029
- Simakov S.K., Kouchi A., Mel’nik N.N. et al. Nano-diamond finding in the Hyblean shallow mantle xenoliths. Scientific Reports. 2015. Vol. 5. N 10765. DOI: 10.1038/srep10765
- Gress M.U., Timmerman S., Chinn I.L. et al. Two billion years of episodic and simultaneous websteritic and eclogitic diamond formation beneath the Orapa kimberlite cluster, Botswana. Contributions to Mineralogy and Petrology. 2021. Vol. 176. N 54.
DOI: 10.1007/s00410-021-01802-8 - Sobolev N.V. Deep inclusions in kimberlites and the problem of upper mantle composition. Novosibirsk: Nauka, 1974. p. 264 (in Russian).
- Stachel T., Harris J.W. Formation of diamond in the Earth’s mantle. Journal of Physics: Condensed Matter. 2009. Vol. 21. N 364206. DOI: 10.1088/0953-8984
- Garanin V.K., Kudryavtseva G.P., Marfunin A.S., Mikhaylichenko O.A. Inclusions in diamond and diamond-bearing rocks. Мoscow: MGU, 1991. p. 240 (in Russian).
- Burgess R., Layzelle E., Turner G. et al. Constraints on the age and halogen composition of mantle fluids in siberian coated diamonds. Earth and Planetary Science Letters. 2002. Vol. 197, p. 193-203. DOI: 10.1016/S0012-821X(02)00480-6
- Shirey S.B., Richardson S.H. Start of the Wilson Cycle at 3 Ga Shown by Diamonds from Subcontinental Mantle. Science. 2011. Vol. 333. Iss. 6041, p. 434-436. DOI: 10.1126/science.1206275
- Beskrovanov V.V. Ontogeny of diamond. Novosibirsk: Nauka, 2000. p. 263 (in Russian).
- Taylor L.A., Anand M. Diamonds: time capsules from the Siberian Mantle. Geochemistry. 2004. Vol. 64. Iss. 1, p. 1-74.
DOI: 10.1016/j.chemer.2003.11.006 - Banas A., Stachel T., Muehlenbachs K., McCandless T.E. Diamonds from the Buffalo Head Hills, Alberta: Formation in a non-conventional setting. Lithos. 2007. Vol. 93. Iss. 1-2, p. 199-213. DOI: 10.1016/j.lithos.2006.07.001
- De Stefano A., Kopylova M.G., Cartigny P. et al. Diamonds and eclogites of the Jericho kimberlite (Northern Canada). Contributions to Mineralogy and Petrology. 2009. Vol. 158, p. 295-315. DOI: 10.1007/s00410-009-0384-7
- Korolev N.M., Kopylova M., Bussweiler Y. et al. The uniquely high-temperature character of Cullinan diamonds: A signature of the Bushveld mantle plume? Lithos. 2018. Vol. 304-307, p. 362-373. DOI: 10.1016/j.lithos.2018.02.031
- Tal'nikova S.B. Inclusions in natural diamonds of different habits // Sixth International Kimberlite Conference, Novosibirsk, Russia, Extended Abstracts. 1995. Vol. 6(1), p. 603-605. DOI: 10.29173/ikc1982
- Schwartz S., Guillot S., Reynard B. et al. Pressure-temperature estimates of the lizardite/antigorite transition in high-pressure serpentinites. Lithos. 2013. Vol. 178, p. 197-210. DOI: 10.1016/j.lithos.2012.11.023
- Ulmer P., Trommsdorff V. Phase relations of hydrous mantle subducting to 300 km. Mantle Petrology: Field Observations and High Pressure Experimentation. Geochemical Society. 1999. Vol. 6, p. 259-281.
- Meyer H.O.A., McCallum M.E. Mineral Inclusions in Diamonds from the Sloan Kimberlites, Colorado. The Journal of Geology. 1986. Vol. 94. N 4, p. 600-612. DOI: 10.1086/629062
- Manuella F.C., Scribano V., Carbone S. Abyssal serpentinites as gigantic factories of marine salts and oil. Marine and Petroleum Geology. 2018. Vol. 92, p. 1041-1055. DOI: 10.1016/j.marpetgeo.2018.03.026
- Simakov S.K., Dubinchuk V.T., Novikov M.P., Drozdova I.A. The formation of diamond and diamond-like phases from carbon-containing fluid at PT-parameters corresponding to processes in the Earth's crust. Doklady Akademii nauk. 2008. Vol. 421. N 1, p. 98-100 (in Russian).
- Borshchevskiy Yu.A. Patent N 2042748 RU. Method of diamond synthesis. Publ. 27.08.1995 (in Russian).
- Pasteris J.D. Occurrence of graphite in serpentinizedolivines in kimberlite. Geology. 1981. Vol. 9. N 8, p. 356-359.
DOI: 10.1130/0091-7613(1981)9<356:OOGISO>2.0.CO;2 - Sobolev N.V., Sobolev A.V., Tomilenko A.A. et. al. Prospects of Search for Diamondiferous Kimberlites in the Northeastern Siberian Platform. Russian Geology and Geophysics. 2018. N 10, p. 1701-1719 (in Russian). DOI: 10.15372/GiG20181012
- Sobolev N.V., Tomilenko A.A., Bul’bak T.A., Logvinova A. Composition of hydrocarbons in diamonds, garnet, and olivine from diamondiferous peridotites from the Udachnaya pipe in Yakutia, Russia. Engineering. 2019. Vol. 5. Iss. 3, p. 471-478.
DOI: 10.1016/j.eng.2019.03.002 - Khachatryan G.K. Organic Matter In Diamonds from Kimberlite Sources: Genetic Information Content. Rudy i metally. 2017. N 3, p. 77-84 (in Russian).
- Stegnitskiy Yu.B. Indication parameters of kimberlites and their use in exploration and exploitation of deposits (on the example of the Nyurbinskaya and Katoka pipes): Author's dissertation. ... Candidate of Geological and Mineralogical Sciences. Мoscow: Tsentral'nyy nauchno-issledovatel'skiy geologorazvedochnyy institut tsvetnykh i blagorodnykh metallov, 2006. p. 28 (in Russian).
- Gurney J.J. A correlation between garnets and diamonds. Kimberlite Occurrence and origin: A Basis for Conceptual Models in Exploration. University of Western Australia. 1984. Vol. 8, p. 143-166.
- Gurney J.J. Diamonds. International Kimberlite Conference, Perth, Australia, 1986. Geological Society of Australia, 1989. Vol. 4, p. 363-367.
- Gurney J., Helmstaedt J.H., Moore R.O. A review of the use and application of mantle geochemistry in diamond exploration. Pure and Applied Chemistry. 1993. Vol. 65. N 12, p. 2423-2442. DOI: 10.1351/pac199365122423
- Kostrovitskiy S.I., Suvorova L.F., Yakovlev D.A. Compositional evolution of picroilmenite from kimberlite pipes of Yakutia. All-Russian conference “Modern problems of geochemistry”. Irkutsk: Institut geokhimii Sibirskogo otdeleniya Rossiyskoy akademii nauk, 2012. Vol. 2, p. 90-93 (in Russian).
- Vaganov V.I., Ilupin I.P., Prokopchuk B.I. Kimberlites. Мoscow: Nedra, 1990, p. 248 (in Russian).
- Wyatt B.A., Baumgartner M., Anckar E., Grutter H. Compositional classification of “kimberlitic” and “non-kimberlitic” ilmenite. Lithos. 2004. Vol. 77. Iss. 1-4, p. 819-840. DOI: 10.1016/j.lithos.2004.04.025
- Moore A.E. A model for the origin of ilmenite in kimberlite and diamond: implications for genesis of the discrete nodule (magacryst) suite. Contributions to Mineralogy and Petrology. 1987. Vol. 95, p. 245-253. DOI: 10.1007/BF00381274
- Schulze D.J., Anderson P.F.N., Hearn C.J.B. Origin and significance of ilmenite magacrysts from kimberlite. International Geology Review. 1995. Vol. 37. Iss. 9, p. 780-812. DOI: 10.1080/00206819509465427
- Haggerty S.E. The chemistry and genesis of opaque minerals in kimberlite. Physics and Chemistry of the Earth. 1975. Vol. 9, p. 295-308. DOI: 10.1016/B978-0-08-018017-5.50027-4
- Ashchepkov I.V., Pokhilenko N.P., Vladykin N.V. et al. Reconstruction of mantle sections beneath Yakutian kimberlite pipes using monomineral thermobarometry. Metasomatism in Oceanic and Continental Lithospheric Mantle. Geological Society. 2008. Vol. 293, p. 335-352. DOI: 10.1144/SP293.15
- Hasterok D.S., Chapman D. Heat production and geotherms for the continental lithosphere. Earth and Planetary Science Letters. 2011. Vol. 307. Iss. 1-2, p. 59-70. DOI: 10.1016/j.epsl.2011.04.034
- 40. Zinchuk N.N., Gorshkov A.I., Rotman A.Ya. et al. The first discovery of a new ordered mixed-layer lizardite-saponite mineral in kimberlites of the Katoka pipe. Problems of forecasting, prospecting and exploration of mineral deposits at the threshold of the XXI century. Voronezh: Izd-vo Voronezhskogo gosudarstvennogo universiteta, 2003, p. 206-210 (in Russian).