Manifestation of incompatibility of marine residual fuels: a method for determining compatibility, studying composition of fuels and sediment
- 1 — Ph.D. Assistant Lecturer Saint Petersburg Mining University ▪ Orcid
- 2 — Ph.D., Dr.Sci. Head of Department Saint Petersburg Mining University ▪ Orcid
Abstract
The results of studying the problem of active sediment formation when mixing residual fuels, caused by manifestation of incompatibility, are presented. A laboratory method has been developed for determining the compatibility and stability of fuels allowing identification of a quantitative characteristic of sediment formation activity. Laboratory studies were performed, and incompatible fuel components were identified. Tests were made to determine the quality indicators of samples and group individual composition of fuels. Results on the content of total and inorganic carbon in the obtained sediments were determined using Shimadzu TOC-V SSM 5000A. Chemical composition was determined and calculated on LECO CHN-628 analyser. Group composition of hydrocarbon fuels contained in the sediment was studied by gas chromato-mass spectrometry on GCMS-QP2010 Ultra Shimadzu. To obtain additional information on the structural group composition of fuel sediment, IR spectrometry was performed on IR-Fourier spectrometer IRAffinity-1. X-ray diffraction analysis of sediment samples was made using X-ray diffractometer XRD-7000 Shimadzu; interplanar distances d002 and d100 as well as Lс and Lа crystallite sizes served as the evaluation criteria. Microstructural analysis of total sediment was performed by scanning electron microscopy. The results of the research confirmed that the content of normal alkanes in the fuel mixture mainly affects sediment formation. Recommendations were drawn on preserving the quality of fuels and reducing sediment formation during storage and transportation.
Introduction
A sharp increase in the share of blended fuels for shipboard installations is due to toughening of sulphur content constraints following the new requirements by the International Maritime Organization (IMO). According to the new convention of the International Maritime Organization, in Annex VI MARPOL 73/78, from January 1, 2020, in all areas of the World Ocean, it is regulated that sulphur content in marine fuels should not exceed 0.5 wt.%. As a result, the allowable sulphur content in marine fuels decreased seven-fold – from 3.5 to 0.5 wt.% [1, 2]. Oil refineries in Russia are currently not able to fully provide consumers with the new type of fuel with sulphur content to 0.5 %, since this requires significant changes in the refining process and major capital investments [3, 4]. To meet the demand for the new type of marine fuels, bunkering companies actively engage in fuel mixing operations to reach the required quality indicators [5, 6].
Therefore, during storage and transportation, especially marine transportation, the problem of active sediment formation in residual and blended fuels caused by the loss of stability is relevant [7, 8]. According to requirements of the international standard ISO 8217 for fuel quality, the content of total sediment should not exceed 0.1 wt.%. It should be noted that there are risks of incompatibility even when mixing the same brand of fuels due to differences in composition. The incompatibility of residual fuels is manifested due to strong intermolecular interactions caused by a change in the structural group individual composition as well as the ratio of concentrations of high-molecular compounds of residual fuels. All this contributes to the formation of molecule associations, bulk colloidal particles of various shapes and structures [9, 10]. This problem is relevant in the production of high-paraffinic crude oil [11, 12] and its preparation for transportation at the fields, as there is a change in technological para-meters, primarily temperature and pressure [13, 14]. More detailed theoretical and experimental studies of this process are required, which will allow determining the main mechanisms for the manifestation of sediment formation and, on this basis, drawing-up efficient solutions.
There are several laboratory procedures and methods for determining the compatibility and stability of residual fuels before actual mixing in tanks and storage facilities, namely the spot test GOST 33365 and ASTM D 4740, determination of xylene and toluene equivalents after GOST 33288 and GOST 33296, patent N 2444005 RF [15] and international application N WO2018185520. However, these methods and procedures cannot determine the quantitative characteristic of sediment formation and have a high error and low accuracy; therefore, they have not been widely used in practice [16].
According to colloidal chemical concepts, asphaltenes are in a stable state suspended in the fuel system due to a solvate shell which prevents the formation of associations and sediment. However, with increasing proportion of normal paraffins, heavy components coagulate and precipitate [17, 18].
When mixing residual fuels containing paraffin compounds with a low C/H ratio and a low molecular weight, the solvate shell of the so-called protective rings is dissolved. Paraffin compounds play the role of a solvent, which leads to enlargement of asphaltene associations, and as a result, active sediment formation [6, 19]. State of knowledge on the sediment formation process in fuel systems in comparison with lighter hydrocarbons is insufficient [20]. This is due to the fact that standard procedures and approaches to the analysis of light hydrocarbons are not applicable here. Therefore, it is necessary to study the group individual composition of fuel samples as well as the sediment forming due to the loss of stability of oil dispersed system for a more detailed knowledge of the effect of fuel composition on sediment formation.
Sediment formation caused by the loss of fuel stability entails several urgent problems, namely deterioration of fuel quality, a decrease in usable volume of tanks, wear and clogging of technological systems as well as the associated economic losses [21-23]. Additionally, it should be noted that the sediment negatively affects the corrosion activity of metal, especially considering tank bottom water and water-oil emulsions [24, 25]. The process of sediment formation caused by the loss of stability has not been sufficiently studied: there are no dependences on the influence of composition, methods for the analytical determination of manifestation of incompatibility of fuel mixtures, and studies of the sediment proper.
Methodology
To perform the research, a new laboratory method for determining the compatibility and stability of fuels has been developed [26]. This method imitates the process of long-term storage of fuels due to a new testing algorithm based on GOST 33360-2015. Initially, the content of total sediment of each mixture component is determined. The components are mixed in the required proportions, then the value of total sediment of the fuel mixture is determined, from which the compatibility index is calculated. Samples with petroleum products should be mixed and heated before testing. It is necessary to determine the value of total sediment of each sample and use the equipment in accordance with GOST 33360-2015. Next, mix the required number of petroleum product samples with the known values of total sediment in a chemically clean 100 ml glass beaker in the required proportion and blend thoroughly to a homogeneous state. The resulting homogeneous mixture of pet-roleum products should be placed in a conical flask in the amount of 25.0 ± 1.0 g of the sample.
To conduct the laboratory tests with thermal aging of the sample, it is necessary to attach a condenser tube to the conical flask with a mixture of petroleum products (to prevent evaporation of light fractions) and place it in an oil bath heated to a temperature 100.0 ± 0.5 °C for 24.00 ± 0.25 h [26, 27]. Shake the flask after holding to ensure that the sediment is evenly distributed. Then the contents of the flask are transferred to the filtering unit in the centre of the heated filter. The transferred amount of the sample should be 10.0 ± 0.5 g.
After filtration is completed and the filter is dried, heating, and vacuum blowing continue for another 5 mintes. The filter is thoroughly washed with a mixture of toluene (15 %) and heptane (85 %). The filters are then dried in a drying cabinet at 110°C, cooled to room temperature in a desiccator, weighed, and total sediment content of the mixture (Sc) is calculated.
Mass fraction of the sediment is recorded as the arithmetic mean of the results of two parallel determinations with an accuracy to 0.01%. The compatibility index of the fuel mixture is defined as the sum of the average value of total sediment of mixture components and convergence value:
where Sav is the average value of total sediment of fuel mixture components, wt.%; r is the value of convergence (repeatability), r=0,123√X; X is the arithmetic mean of two determinations of total sediment content.
If Sc ≥ CI, fuel mixture is incompatible; if Sc < CI, fuel mixture is compatible and stable.
The advantage of the method is that it becomes possible to determine the compatibility and stability of the fuel mixture before actual mixing in tanks, containers, and storage tanks as well as to quantitatively characterize sediment formation in fuels and take samples of the forming sediment for further research.
Previously, studies of different types of fuels for compatibility were performed and incompatible mixtures of residual fuels were determined [27, 28]. As objects of study, fuels of TSU-380 brand (sample 1) and a compound of lubricating oils, brand A, type 1 (sample 2) were chosen, since these samples of residual marine fuels contain all the main groups of hydrocarbons, namely: normal alkanes, isoalkanes, alkenes, naphthenic, aromatic, and asphaltene-resinous substances.
Determination of group and individual compositions of hydrocarbons was performed by gas chromato-mass spectrometry on GCMS-QP2010 Ultra Shimadzu and by SARA-analysis on “Gradient-M” laboratory gas chromatograph with a thermal conductivity detector by liquid adsorption chromatography on glass columns 30 cm high and 1.2-1.4 mm in diameter [20, 29].
When studying the chemical composition of fuel sediment, both organic and inorganic micro-impurities, modern methods and equipment were used to obtain reliable results that determine the effect of hydrocarbon composition on incompatibility.
The contents of organic and inorganic carbon were measured by Shimadzu TOC-V with an attachment for the analysis of solid samples SSM 5000A [30, 31]. For this purpose, the subsample is completely burned, and the content of exhaust carbon dioxide is determined using IR cells. Elemental composition of fuel sediment was determined using LECO CHN-628 analyser, which allows establishing the content of carbon, nitrogen, and hydrogen [32-34]. Ash content of fuel sediments was also determined according to GOST 1461. Oxygen content in the resulting sediment was calculated following ISO 17247:2013. To find the composition of elements forming inorganic compounds in the sediment, X-ray fluorescence analysis was performed using X-act detector to Tescan Vega 3.0 LMH scanning electron microscope [17, 35]. Determination of sulphur content in the sediment was carried out on Spectroscan S energy-dispersive X-ray fluorescence analyser. Sediment was previously dissolved in toluene, after determining mass fraction of sulphur, sulphur content in fuel sediment was calculated.
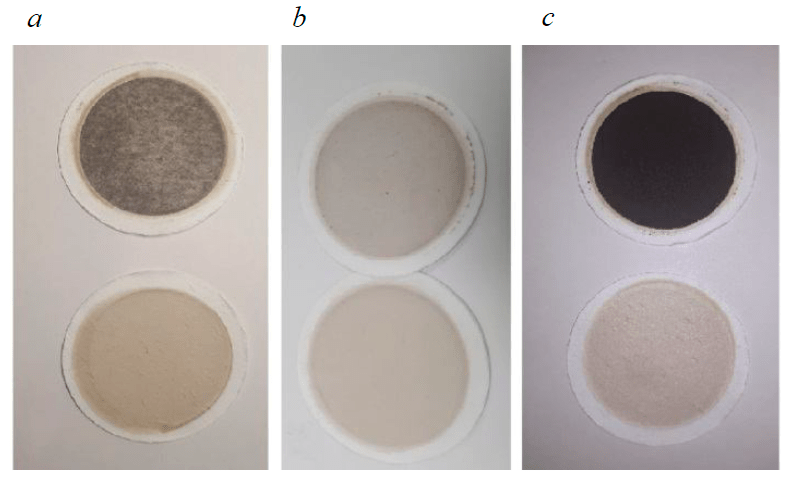
Fig.1. Filters after filtration and determination of total sediment content: a – sample 1; b – sample 2; c – mixture of samples 1 and 2 [30]
For X-ray diffraction analysis of sediment samples, X-ray diffractometer XRD-7000 Shimadzu (CuKα radiation, 2.7 kW) was used according to the polycrystal method, X-ray exposure was performed at room temperature with long accumulation times (2 s) and a sampling interval 0.02° [36, 37].
To obtain additional information on the structural group composition of fuel sediment, studies were carried out by IR spectrometry method on IR-Fourier spectrometer IRAffinity-1 [38]. A solution of sediment was prepared with concentration 1 % in carbon tetrachloride.
Discussion
Quality indicators of fuels (samples 1 and 2) were obtained by laboratory tests (Table 1).
Table 1
Quality indicators of investigated fuel samples
Quality indicators |
Regulatory documents |
Sample 1 |
Sample 2 |
Density (at 15 °С), kg/m3 |
ISO 12185 |
956.0 |
833.5 |
Kinematic viscosity (at 50 °С), mm2/s |
GOST 33 |
321.5 |
12.10 |
Closed cup flash point, °С |
ISO 2719 |
98.0 |
181.0 |
Sulphur content, % |
GOST Р 51947 |
1.21 |
0.004 |
Pour point, °С |
ASTM D 6749 |
16.0 |
26.0 |
Water content, % |
GOST Р 51946 |
0.1 |
0.05 |
Total sediment (TSA), % |
GOST 33360 |
0.02 |
0.01 |
Total sediment (TSP), % |
GOST 33360 |
0.02 |
0.01 |
According to ISO 8217 sample 1 corresponds to brand RMG 380 and sample 2 to brand RMD-80.
According to the developed method, samples 1 and 2 are mixed in a ratio 1: 1. Incompatibility of fuels contributes to a sharp increase in the amount of total sediment, TSP = 0.17 wt.% (Fig.1).
Group hydrocarbon composition of samples was analysed using SARA analysis and chromato-mass spectrometry. The results are presented in Table 2.
Table 2
Analysis of group hydrocarbon composition of fuel samples, wt.%
Fuel samples |
Asphaltenes |
N-paraffins |
Isoparaffins |
Naphthenes |
Alkenes |
Aromatic HC |
Resins |
1 |
2.53 |
54.14 |
22.74 |
5.43 |
0.57 |
14.12 |
0.47 |
2 |
– |
71.93 |
18.35 |
2.62 |
5.55 |
1.55 |
– |
Based on results of determining group hydrocarbon composition of the studied marine residual fuel samples, it can be concluded that sample 1 contains residual oil refining products, since it contains asphaltenes and resins and products of secondary oil refining processes, which is clear due to the presence of alkenes. Sample 2 has a high content of normal alkanes, which contribute to precipitation of asphaltene agglomerates.
Studies were carried out to identify the composition of sediment obtained from the mixture of residual fuels that are insoluble in n-heptane (heptane-insoluble – HI), but soluble in toluene (toluene-soluble – TS), the sample was dissolved in toluene in a ratio of 1:100. Hydrocarbon composition of the resulting liquid-phase mixture was determined using gas chromato-mass spectrometer GCMS-QP2010 Ultra. At the same time, substances were found that boil off at temperature to 300 °C in the chromatograph column (Fig.2).
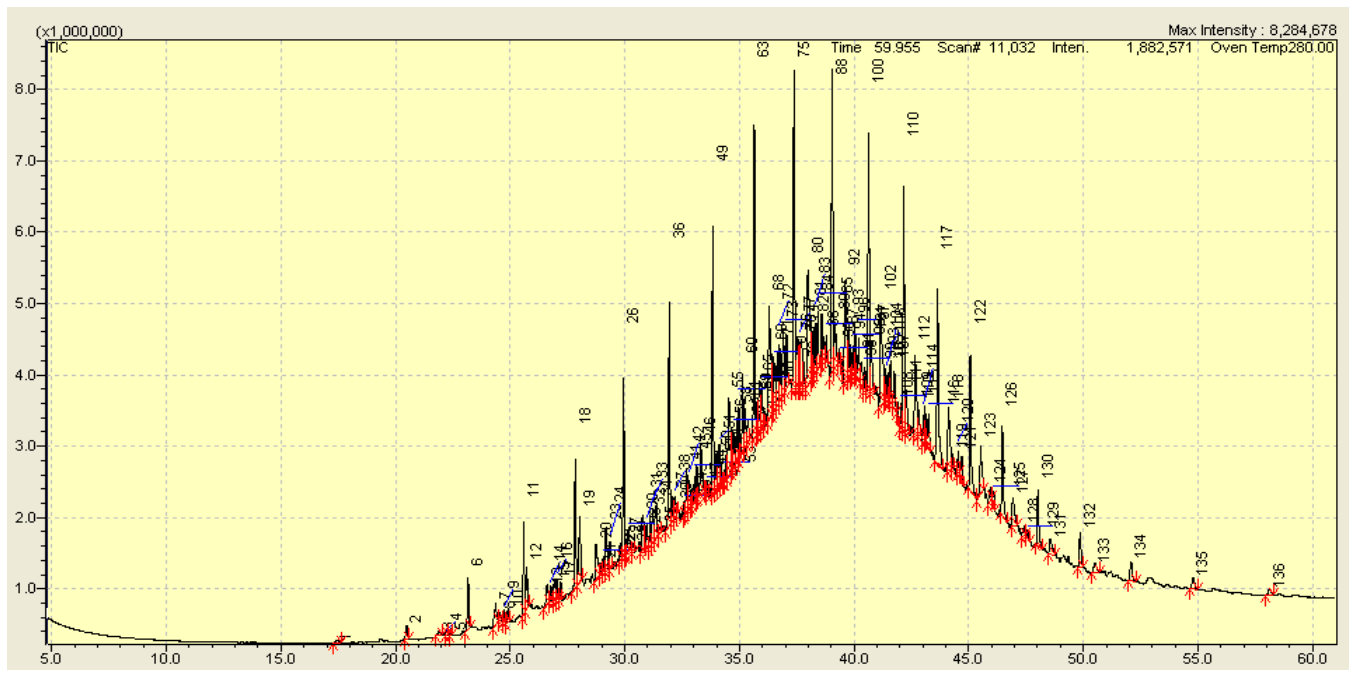
Fig.2. Chromatogram of sediment obtained from residual fuel
As a result of gas chromato-mass spectrometry of HI-TS fraction obtained from residual fuel, the structural group composition was determined, %: normal paraffins – 39.01; aromatic hydrocarbons – 15.63; isoparaffins – 15.29; naphthenes – 3.01; unidentified – 28.16.
Thus, the main share of HI-TS fraction of residual fuel from the identified compounds belongs to normal paraffins, and unidentified components, presumably, to asphaltenes and resins, since the chromatogram (Fig.2) is defined as an undivided peak in the form of a Gaussian. Therefore, we presume a presence of a complex mixture of macromolecular compounds boiling up to 300 °C, which got into the column, but was not considered when calculating the hydrocarbon composition.
According to the test results on TOC-V SSM 5000A, the content of organic carbon is 83.50 ± 1.0 %, inorganic carbon was not detected.
Studies were performed to determine the elements contained in inorganic substances, in fuel sediment by X-ray fluorescence analysis using an X-act detector to a scanning electron microscope. Scanning electron microscopy was applied to obtain electron microscopic images of sediment particles in secondary electrons in the Resolution scanning mode. Scanning of sediment obtained after thermal aging, filtration and drying was carried out on the top filter. Spectra were processed for all elements based on the standards, no peaks were missed, the number of iterations performed was 3, which indicates a high reliability of the obtained results.
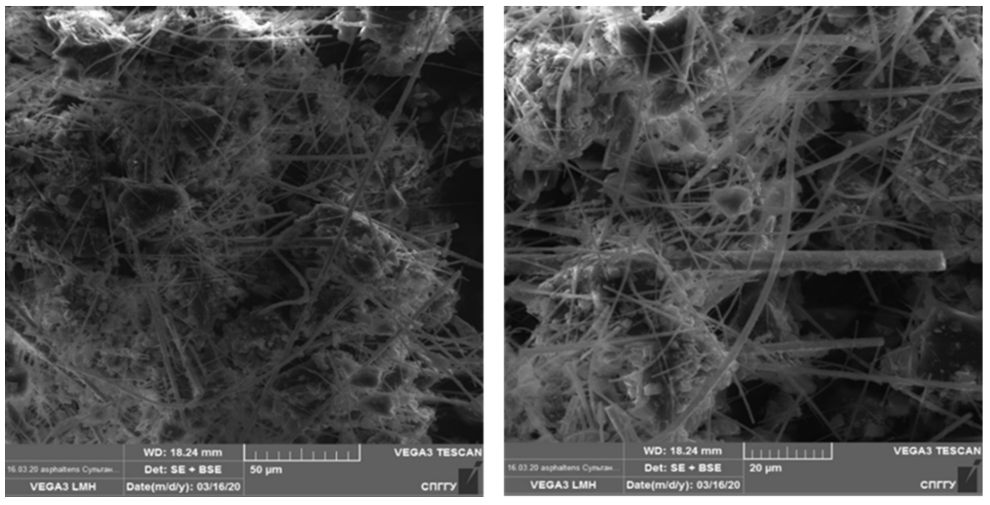
Fig.3. Electronic image of filter with sediment particles
The research results are presented as SEM images (Fig.3). By determining the spectrum of the filter fiber, elemental composition of the filter proper was established to exclude a possible influence when measuring the spectrum of se-diment elements (Fig.4).
It was recorded that inorganic impurities in the sediment are represented only by sulphur derivatives. There are traces of zinc that are not part of fuel sediment but are defined as a component of the filter fibre. Howe-ver, the method of scanning electron microscopy can only determine the presence and ratio of inorganic substances in the sample. To determine the a mount of sulphur in total composition of the studied sediment, X-ray energy dispersive analysis of sulphur was applied. A sediment sample was dissolved in toluene in a ratio of 1:10, after which sulphur was detected in the mixture and a calculation was performed, which showed that sulphur content in fuel sediment was 2.61 %.
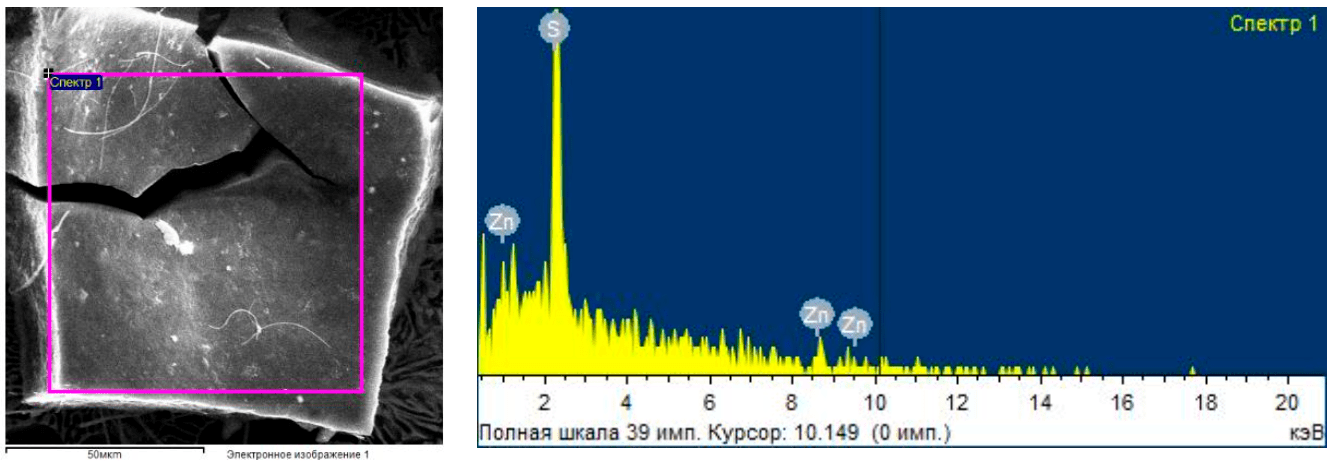
Fig.4. Electronic image and spectrum of elements of carbon material of sediment
The results of determining elemental analysis (Fig.5) using LECO CHN-628 analyser and ash content values by the laboratory method were obtained, and oxygen content was calculated. Chemical composition of sediment, %: carbon – 84.936; hydrogen – 10.709; nitrogen – 0.639; sulphur – 2.610; oxygen – 1.076; ash content – 0.03.
It should be noted that total carbon content obtained on LECO CHN-628 element analyser (84.94 ± 1.0 %) is higher compared to that determined on Shimadzu TOC-V (83.50 ± 1.0 %). Perhaps, this is due to the fact that the latter has a higher temperature in the oxidizing chamber, so complete oxidation of carbon does not occur, and the values are underestimated. Thus, the results of carbon determination by the two methods are close and allow determining with high accuracy the amount of carbon in total sediment of residual fuels. The result obtained on LECO CHN-628 element analyser (84.94 ± 1.0 %) was accepted as final.
According to the results of determining the chemical composition of sediment, it can be stated that, in terms of C:H = 8.5:1 it has an intermediate value between liquid oil fuels and oil carbon materials (for example, petroleum coke). The increase in C:H occurs due to a decrease in H concentration and an increase in C, which can be attributed to the so-called condensation. Polyaromatic hydrocarbons coagulate during formation of sediment with an increase in molecular weight and represent amorphous carbon.
The resulting IR spectrum of fuel sediment solution in carbon tetrachloride is shown in Fig.6.
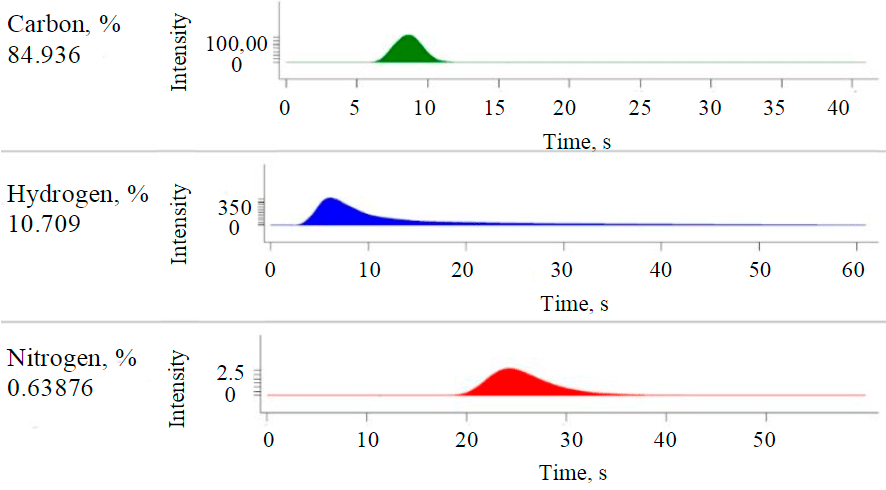
Fig.5. Result of determining elemental composition of sediments on LECO CHN-628 analyser
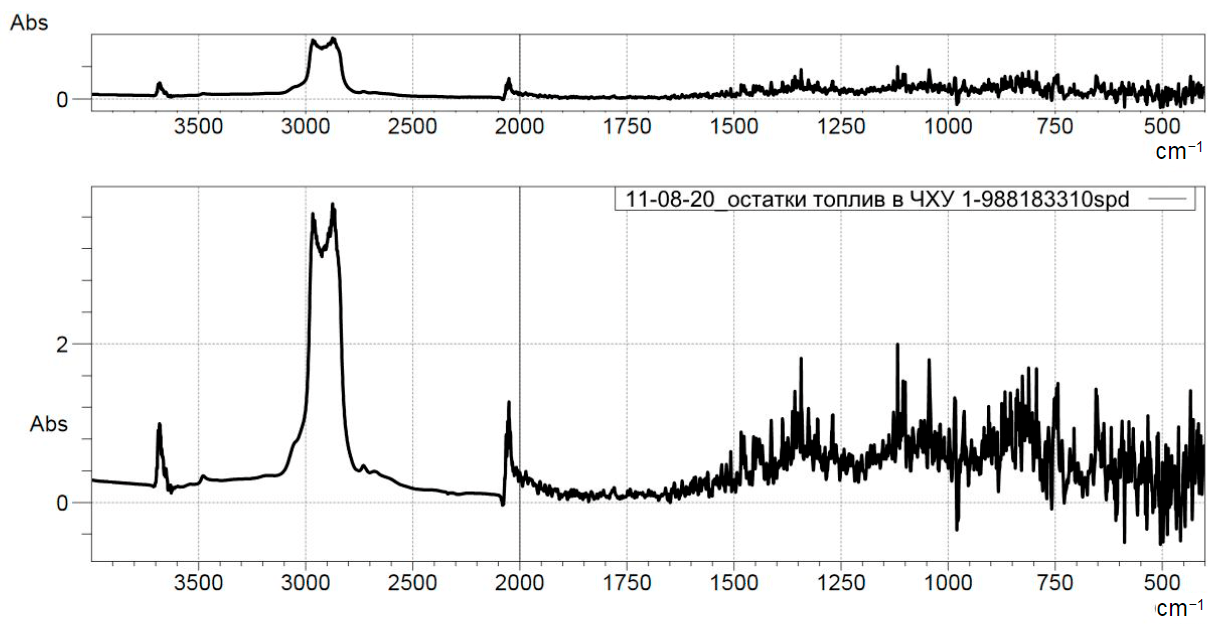
Fig.6. IR-spectrum of fuel sediment in carbon tetrachloride
Wave numbers of about 3,000 cm–1 correspond to normal paraffinic hydrocarbons, which confirms the results obtained by chromatography. Using the results of the authors of papers [1, 39] and applying their calculation procedure the absorption bands were estimated and calculations were made on the basis of the obtained data on IR spectrum of sediment. Unbranched paraffin structures are defined by strong absorption bands corresponding to 720 and 1,300 cm–1. Evaluation of the absorption bands in the region of 1,376 cm–1 and 1,464 cm–1 makes it possible to establish the branching degree of hydrocarbon paraffin chains, the characteristic of which is determined using β coefficient. To determine β coefficient, it is necessary to calculate the ratio of intensities of the most characteristic absorption bands for СН3 and СН2 groups:
The greater the value of this coefficient β7201464, the higher the branching degree of paraffin structures in hydrocarbons. Ranges 812-816 cm–1 as well as 1,600 cm–1 are the absorption bands corresponding to aromatic structures. The degree of aromaticity can be characterized by the ratio of intensities of the most characteristic absorption bands for aromatic structures relative to methylene groups of paraffinic structures determined by the formula:
For aromatic structures, relative to methylene groups of paraffin structures, a ratio is determined that shows the degree of aromatization of fuels:
Based on results of determining the values of aromatization coefficients (β7201600=0.69 and β14641600=0.85), it can be concluded that the studied fuel sediment is estimated as methane, or methane-naphthenic, and this is consistent with the results of gas chromato-mass spectrometry.β7201464=0.82 coefficient confirms the presence of a considerable number of branched structures in the composition of the studied fuel sediment.
Based on results of using the X-ray diffraction method, it was determined that total sediment is amorphous carbon (Fig.7) with crystallinity degree much lower than in the ideal structure of graphite [40]. Amorphous carbon matrix is formed by crystallites which have a turbostratic structure [8]. The main parameters of the structures of such crystallites are interplanar distances – d002, d110 and the sizes of coherent scattering regions in the direction of crystallographic axes с and а, Lc and La, respectively. For graphite, d002 and d110 are 3.354 and 1.232 Å, respectively, while for the turbostratic structure, d002 ranges from 3.37 to 3.60 Å and d110 from 1.215 to 1.230 Å [41].
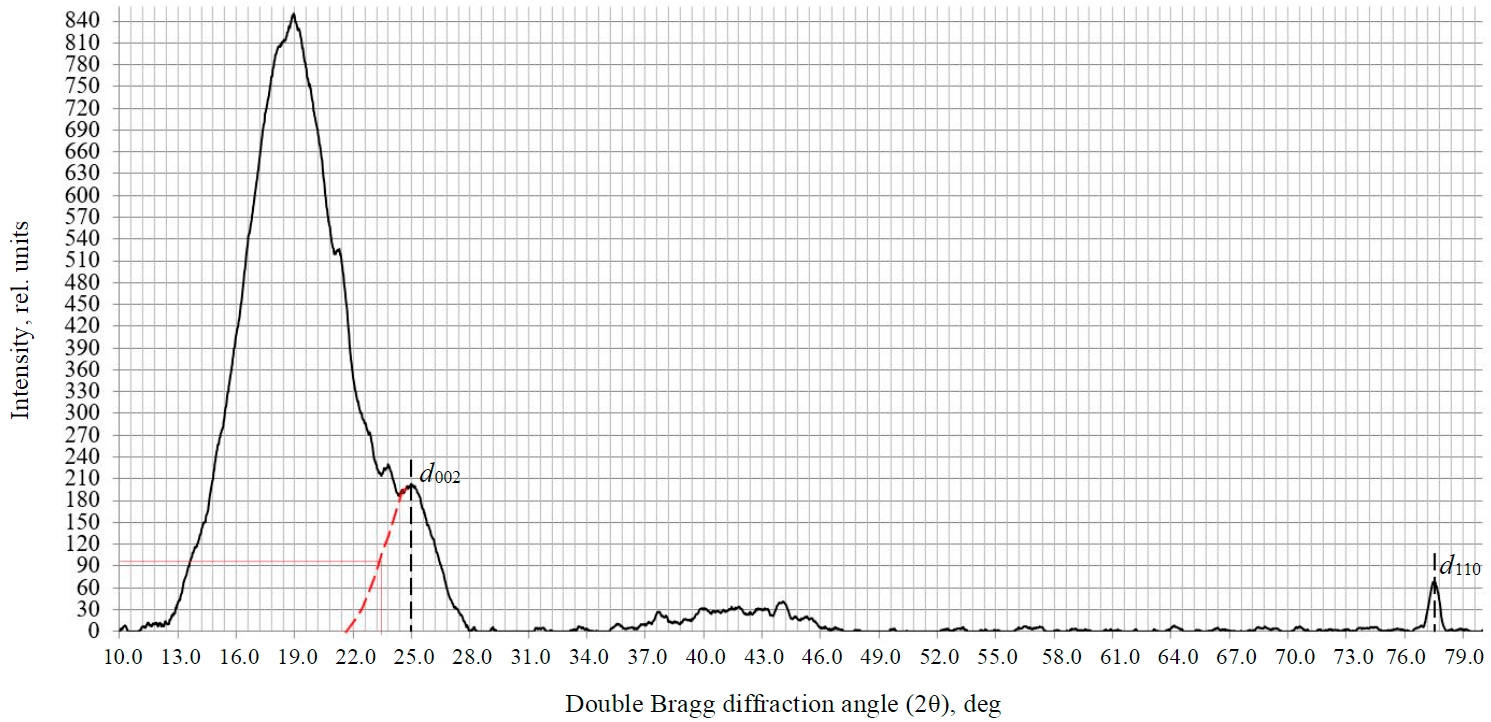
Fig.7. Diffractogram of obtained sediment
The highest intensity (850 rel. units) in the diffraction pattern has an amorphous peak with the maximum value of doubled Bragg diffraction angle 2θ of about 19°. This peak can characterize the liquid-phase heavy hydrocarbon components of residual fuels remaining after heat treatment at 100°C and filtration and be a γ-peak characteristic of asphaltenes contained in residual marine fuel and show the presence of alkyl and naphthenic rings in the structure of the asphaltene shield. It should be noted that the ratio of 002 peak and γ-peak intensities is approximately the same.
Structure of crystal lattice of sediment after thermal aging is controlled by pronounced peaks with maximum 2θ angle values of about 25 and 77° corresponding to reflexes 2θ002 and 2θ110. Angular position of reflexes (2θ002 and 2θ110) on X-ray pattern is determined by the corresponding interplanar spacing (d002 and d110) [41]. Results of diffraction analysis and calculated values for the sediment after aging: reflex (002) – 2θ = 24.9800°, half-width – 2.8400°, d002 – 3.5618 Å, Lc – 27.7648 Å; reflex (110) – 2θ = 77.5000°, half-width – 0.6000°, d110 – 1.2307 Å, La – 174.0710 Å.
Interplanar distance d002 is 3.5618 Å, and d110 – 1.2307 Å. According to the results of X-ray phase analysis, the nature of microstructure of carbon materials can be presumed from the ratio of the average height Lc and average diameter La of crystallites [41, 42]. In this case, Lc to La ratio is approximately 1 to 16, which indicates an oblate structure of crystallites pointing to the start of transition from the liquid phase to the amorphous solid state due to physical filtration process after heat treatment at 100 °C for 24 h.
Results
The developed laboratory method for determining the compatibility and stability of fuels allows determining the compatibility of fuel mixtures before actual mixing in tanks and storage facilities, which reduces the risks of incompatibility and loss of fuel quality during storage and technological operations at fuel terminals and tank farms. At the same time, the method allows evaluating the activity of sediment formation and determining its quantitative characteristics, which means that it is possible to select fuel components and their ratio in the fuel mixture to obtain optimal quality indicators.
The studies carried out to identify the problem of active sediment formation which is caused by the manifestation of incompatibility of residual fuels during mixing, give a clear idea of this negative process. The manifestations of incompatibility are caused by a change in structural group composition of fuel oil dispersed system. An increase in the share of normal paraffins and, at the same time, a decreasing proportion of asphaltenes, reduces the stability of the dispersed system of fuel mixture, which leads to active sediment formation. With increasing proportion of n-paraffins, the solvate shell of asphaltenes, which are in suspension in a stable system, is destroyed, thereby n-paraffin acts as a solvent. As a result, the growth of asphaltene nanoaggregates occurs, the inner part of molecules, which has a high energy, is surrounded by alkanes. This is confirmed by the studies presented in this paper: in sample 2, the content of n-paraffins is almost 72 %, which increases their share in fuel during mixing and promotes active sedimentation of asphaltenes. It was recorded that a considerable proportion (about 39 %) in total carbon of sediment is accounted for by normal paraffins.
X-ray phase analysis allowed determining the microstructure of carbon materials. Total sediment is amorphous carbon with crystallinity degree much lower than in the ideal graphite structure. The amorphous carbon matrix is formed by crystallites having a turbostratic structure. Lc to La ratio is approximately 1 to 16, which indicates an oblate structure of crystallites pointing to the beginning of transition from the liquid phase to the amorphous solid state.
According to the results of determining the chemical composition of sediment, ratio C:H = 8.5:1 is an intermediate value between liquid petroleum fuels and petroleum carbon materials. The increase in C:H occurs due to a decreasing H concentration and increasing C concentration, which can be attributed to the so-called condensation. Polyaromatic hydrocarbons coagulate in the process of sediment formation with an increase in molecular weight and represent amorphous carbon. Sulphur content in the studied sediment is 2.61 wt.%, in fuel mixture – 0.6 wt.%, and in sediment – 4.35 times higher than in the fuel mixture. Therefore, when preparing mixed fuels, it is necessary, first, to consider the influence of fuel composition, namely, the content of n-alkanes, with increasing share of which the risks of sediment formation increase sharply and, therefore, the quality of the fuel mixture decreases.
References
- Demidova N.P., Marchenko A.A., Onishchenko O.A. Assessment of compatibility of bunker fuel oils. Vestnik KamchatGTU. 2016. N 35, p. 15-20.
- Korshunov G.I., Eremeeva A.M., Drebenstedt P. Justification of the use of a vegetal additive to diesel fuel as a method of protecting underground personnel of coal mines from the impact of harmful emissions of diesel-hydraulic locomotives. Journal of Mining Institute. 2021. Vol. 247, p. 39-47. DOI: 10.31897/PMI.2021.1.5
- Konoplin R.R., Kondrasheva N.K. Difficulties in the industrial introduction of new effective hydrodesulphurization catalysts in the Russian Federation. Topical Issues of Rational Use of Natural Resources 2021, 31 May – 6 June 2021, St. Petersburg, Russia. E3S Web Conference. 2021. Vol. 266. N 02016. DOI: 10.1051/e3sconf/202126602016
- Pyagay I.N., Shaidulina A.A., Konoplin R.R. et al. Production of Amorphous Silicon Dioxide Derived from Aluminum Fluoride Industrial Waste and Consideration of the Possibility of Its Use as Al2O3-SiO2Catalyst Supports. Catalysts. 2022. Vol. 12. Iss. 2. N 162. DOI: 10.3390/catal12020162
- Dubovoy E.S., Shmatkov А.А., Shtonda N.V., Lyapin A.Y. On the Approach to Performance Evaluation Oil Blending Stations. Science and Technologies: Oil and Oil Products Pipeline Transportation. 2018. Vol.8. N 5, p. 540-546 (in Russian). DOI: 10.28999/2541-9595-2018-8-5-540-546
- Legkokonets V.A., Islamov S.R., Mardashov D.V. Multifactor analysis of well killing operations on oil and gas condensate field with a fractured reservoir. Proceedings of the International Forum-Contest of Young Researchers: Topical Issues of Rational Use of Mineral Resources, 13-17 May 2019, St. Petersburg, Russia. CRC Press, 2019, р. 111-118.
- Beloglazov I.I., Morenov V.A., Leusheva E.L. Flow modeling of high-viscosity fluids in pipeline infrastructure of oil and gas enterprises. Egyptian Journal of Petroleum. 2021. Vol. 30. Iss. 4, р. 43-51. DOI: 10.1016/j.ejpe.2021.11.001
- Gabdulkhakov R.R., Rudko V.A., Povarov V.G. et al. Technology of petroleum needle coke production in processing of decantoil with the use of polystyrene as a polymeric mesogen additive. ACS Omega. 2021. Vol. 6. Iss.30, p. 19995-20005. DOI: 10.1021/acsomega.1c02985
- Karimov R.M., Mastobaev B.N. Effect of paraffins, resins and asphaltenes on commodity quality of oil. Bashkir chemical journal. 2012. Vol. 19. N 1, p. 97-102 (in Russian).
- Mozhayskaya M.V. Influence of composition and structure of high-molecular hydrocarbons and resinous asphaltene substances on sediment formation in oils: Avtoref. dis. … kand. tekhn. nauk. Tomsk: Institut khimii nefti, Sibirskoe otdelenie RAN, 2011, p. 24.
- Mardashov D.V., Bondarenko А.V., Raupov I.R. Technique for calculating technological parameters of non-Newtonian liquids injection into oil well during workover. Journal of Mining Institute. 2022, p. 1-14 (Online first). DOI: 10.31897/PMI.2022.16
- Islamov S., Grigoriev А., Beloglazov I. et al. Research risk factors in monitoring well drilling – A case study using machine learning methods. Symmetry. 2021. Vol. 13. Iss. 7. N 1293. DOI: 10.3390/sym13071293
- Islamov S.R., Bondarenko A.V., Mardashov D.V. Substantiation of a well killing technology for fractured carbonate reservoirs. Youth Technical Sessions Proceedings: VI Youth Forum of the World Petroleum Council – Future Leaders Forum, 23-28 June 2019, Saint Petersburg, Russia. CRC Press, 2019, р. 256-264. DOI: 10.1201/9780429327070
- Khaibullina K.S., Sagirova L.R., Sandyga M.S. Substantiation and selection of an inhibitor for preventing the formation of asphaltresin-paraffin deposits. Periódico Tchê Química. 2020. Vol. 17, р. 541-551. DOI: 10.52571/ptq.v17.n34.2020.565_p34_pgs_541_551.pdf
- Deryugina L.A., Yarosh V.I. Patent N 2444005 RF. Method for determining the compatibility of fuel mixture components. Publ. 27.02.2012. Bull. N 6.
- Pilschikov V.A., Yeremina Yu.V., Tsvetkov V.S. et al. Study of Bottom Sediments in the Oil Storage Tanks. Vestnik of Samara State Technical University (Technical Sciences Series). 2014. N 1 (41), p. 173-179 (in Russian).
- Gabdulkhakov R.R., Rudko V.A., Pyagay I.N. Methods for modifying needle coke raw materials by introducing additives of various origin. Fuel. 2022. Vol. 310. Part A. N 122265. DOI: 10.1016/j.fuel.2021.122265
- Nurgalieva K.S., Saychenko L.A., Riazi M. Improving the Efficiency of Oil and Gas Wells Complicated by the Formation of Asphalt–Resin–Paraffin Deposits. Energies. 2021. Vol. 14. Iss. 20. N 6673. DOI: 10.3390/en14206673
- Kirbizhekova E.V., Prozorova I.V., Yudina N.V. The Study of Asphaltene-Resin-Paraffin Deposits Composition from Water-In-Oil Emulsions. Tomsk State University Journal. 2014. Vol. 388, p. 257-262 (in Russian).
- Sunagatullin R.Z., Karimov R.M., Dmitriev M.E., Baykova M.I. Experimental Studies of the Operational Properties of Asphaltene-Resin-Paraffin Deposits Formed in Main Oil Pipelines. Science and Technologies: Oil and Oil Products Pipeline Transportation. 2018. Vol. 8. N 4, p. 398-406 (in Russian). DOI: 10.28999/2541-9595-2018-8-4-398-406
- Van Thang Nguyen, Rogachev M.K., Aleksandrov A.N. A new approach to improving efficiency of gas-lift wells in the conditions of the formation of organic wax deposits in the Dragon field. Journal of Petroleum Exploration and Production Technology. 2020. Vol. 10, p. 3663-3672. DOI: 10.1007/s13202-020-00976-4
- Palaev A.G., Dzhemilev E.R. Research of efficiency of influence of ultrasonic treatment on asphalt and paraffin oil deposits. IOP Conference Series: Materials Science and Engineering. 2020. Vol. 862. Iss. 3. N 032081. DOI: 10.1088/1757-899X/862/3/032081
- Palaev A.G., Shammazov I.A., Dzhemilev E.R. Research of the impact of ultrasonic and thermal effects on oil to reduce its viscosity. Journal of Physics: Conference Series. 2020. Vol. 1679. Iss. 5. DOI: 10.1088/1742-6596/1679/5/052073
- Bolobov V.I., Popov G.G. Methodology for testing pipeline steels for resistance to grooving corrosion. Journal of Mining Institute. 2021. Vol. 252, p. 854-860. DOI: 10.31897/PMI.2021.6.7
- Kasyanov A.V., Belousov A.E., Popov G.G., Bolobov V.I. Determination of factors affecting on grooving corrosion. Topical Issues of Rational Use of Natural Resources, 13-17 May 2019, St. Petersburg, Russia. CRC Press, 2019. DOI: 10.1201/9781003014577-49
- Sultanbekov R.R., Nazarova M.N., Shchipachev A.M. Patent N 2733748 RF. Method for determining the compatibility and stability of fuel mixture components. Publ. 06.10.2020. Bull. N 28.
- Sultanbekov R.R., Shammazov I.A., Schipachev A.M. Determination of Compatibility and Stability of Residual Fuels before Mixing in Tanks. Petroleum Engineering. 2021. Vol. 19. N 3, p. 128-137. DOI: 10.17122/ngdelo-2021-3-128-137
- Sultanbekov R.R., Nazarova M.N. Studies of mixing high viscosity petroleum and pyrolysis resin to improve quality indicators. Scientific and Practical Studies of Raw Material Issues. London: CRC Press, 2019, p.97-102. DOI: 10.1201/9781003017226-14
- Pashkevich M.A., Bykova M.V. Methodology for thermal desorption treatment of local soil pollution by oil products at the facilities of the mineral resource industry. Journal of Mining Institute. 2022. Vol. 253, p. 49-60. DOI: 10.31897/PMI.2022.6
- 3Carter S., Clough R., Fisher A. et al. Atomic spectrometry update: review of advances in the analysis of metals, chemicals and materials. Journal of Analytical Atomic Spectrometry. 2019. Vol. 34, р. 2159-2216. DOI: 10.1039/C9JA90058F
- Makarov I.S., Golova L.K., Mironova M.V. et al. Structural and Morphological Features of Carbon–Silicon-Carbide Fibers Based on Cellulose and Triethoxyvinylsilane. Chemistry and technology of chemical fibers. 2018. Vol. 50, p. 79-84. DOI: 10.1007/s10692-018-9935-x
- Sánchez R., Lefevreb Johan, Todolí José-Luis. Direct elemental analysis of petroleum heavy fractions by means of ICP-OES equipped with a high temperature torch integrated sample introduction system. Journal of Analytical Atomic Spectrometry. 2019. N 34, р. 664. DOI: 10.1039/C8JA00320C
- Savchenkov S.A., Bazhin V.Y., Povarov V.G. Research on the process of gadolinium recovery from the melt of salts on formation of Mg – Zn – Gd master alloys for manufacturing of magnesium and aluminium special-purpose alloys. Non-ferrous Metals. 2020. Vol. 48. Iss.1, p. 35-40. DOI: 10.17580/nfm.2020.01.06
- Tcvetkov P., Cherepovitsyn A., Fedoseev S. The Changing Role of CO2 in the Transition to a Circular Economy: Review of Carbon Sequestration Projects. Sustainability. 2019. Vol. 11. Iss. 20. N 5834. DOI: 10.3390/su11205834
- Beloglazov I.I., Morenov V.A., Leusheva E.L., Gudmestad O.T. Modeling of Heavy-Oil Flow with Regard to Their Rheological Properties. Energies. 2021. Vol. 14. Iss. 2. N 359. DOI: 10.3390/en14020359
- Ivanova L.V., Safieva R.Z., Koshelev V.N. IR-spectrometry in the analysis of oil and petroleum products. Vestnik Bashkirskogo universiteta. 2008. Vol. 13. N 4, p. 869-874.
- Popova A.N., Sukhomlinov V.S., Mustafaev A.S. Accounting for Interelement Interferences in Atomic Emission Spectroscopy: A Nonlinear Theory. Applied Sciences. 2021. Vol. 11. Iss. 23. N 11237. DOI: 10.3390/app112311237
- Bykova M.V., Pashkevich M.A., Matveeva V.A., Sverchkov I.P. Assessment and abatement of the soil oil-contamination level in industrial areas. Proceedings of the International Forum-Contest of Young Researchers: Topical Issues of Rational Use of Natural Resources 18-20 April 2018, St. Petersburg, Russia. CRC Press, 2019, р. 347-359.
- Rudko V.A., Derkunskii I.O., Gabdulkhakov R.R. et al. Kinetics of various hydrocarbon groups formation in distillates obtained during the production of needle coke via the delayed coking of decantoil. Egyptian Journal of Petroleum. 2022. N 31 (1), р. 33-38. DOI: 10.1016/j.ejpe.2022.02.002
- Rogachev M.K., Thang Nguyen Van, Aleksandrov A.N. Technology for Preventing the Wax Deposit Formation in Gas-Lift Wells at Offshore Oil and Gas Fields in Vietnam. Energies. 2021. Vol. 14. Iss. 16. N 5016. DOI: 10.3390/en14165016
- Smyshlyaeva K.I., Rudko V.A., Povarov V.G. et al. Influence of Asphaltenes on the Low-Sulphur Residual Marine Fuels Stability. Journal of Marine Science and Engineering. 2021. Vol. 9. Iss. 11. N 1235. DOI: 10.3390/jmse9111235
- Sultanbekov R.R., Nazarova M.N. Preserving the quality of petroleum products when mixed in tanks. Topical Issues of Rational Use of Natural Resources 2019. London: CRC Press, 2019, p. 914-922. DOI: 10.1201/9781003014638-57