Structure maintenance experience and the need to control the soils thermal regime in permafrost areas
- 1 — Ph.D., Dr.Sci. Head of Department Lomonosov Moscow State University ▪ Orcid
- 2 — Ph.D. Head of the Center for Geocryological and Geotechnical Research Gersevanova NIIOSP, AO Research Center of Construction ▪ Orcid
- 3 — Ph.D. Researcher Lomonosov Moscow State University ▪ Orcid
- 4 — Ph.D., Dr.Sci. Deputy Director Earth Cryosphere Institute, Tyumen Scientific Centre, Siberian Branch of RAS ▪ Orcid
- 5 — Ph.D. Chief Specialist Gidrospetsgeologiya ▪ Orcid
- 6 — Ph.D., Dr.Sci. Head Technology Development Center for Fuel and Energy Complex of the Ministry of Energy of the Russian Federation ▪ Orcid
- 7 — Ph.D., Dr.Sci. Corresponding Member of the RAS, Director Melnikov Permafrost Institute, Siberian Branch of RAS ▪ Orcid
- 8 — Ph.D., Dr.Sci. Academician of the RAS, Head of Research Area Earth Cryosphere Institute, Tyumen Scientific Centre, Siberian Branch of RAS ▪ Orcid
- 9 — Ph.D. Chief Engineer ООО SPA “Foundationstroyarkos” ▪ Orcid
- 10 — Ph.D. Deputy Head of Engineering and Technical Center “Gazprom Dobycha Nadym” ▪ Orcid
- 11 — Ph.D. Leading Analytical Expert Eastern State Planning Center ▪ Orcid
- 12 — Ph.D. Leading Researcher Earth Cryosphere Institute, Tyumen Scientific Centre, Siberian Branch of RAS ▪ Orcid
- 13 — Ph.D. Head of Laboratory Институт геоэкологии им. Е.М.Сергеева РАН ▪ Orcid
- 14 — Ph.D., Dr.Sci. Chief Researcher Earth Cryosphere Institute, Tyumen Scientific Centre, Siberian Branch of RAS ▪ Orcid
- 15 — Project Manager Technology Development Center for Fuel and Energy Complex of the Ministry of Energy of the Russian Federation ▪ Orcid
Abstract
The risks of reducing the stability of buildings and structures are increasing in conditions of climate change and the active development of the territories under the influence of natural and anthropogenic factors. The main causes include: loss of the bearing capacity of frozen soils, various geocryological processes, errors at the stages of design, construction and operation of facilities. Main actual task when conducting research and industrial operations in the cryolithozone is monitoring and, if necessary, managing thermal processes in the permafrost layers interacting with facilities. In this article the obtained positive experience of various technologies applying at various stages of the life cycle of civil and industrial facilities was analyzed. It helps to eliminate or prevent the structure deformation or destruction under the influence of climate change. The methods of permafrost stabilization used in the oil and gas industry in process of industrial infrastructure development of the fields have been studied – freezing (cooling) of foundation soils during construction on heterogeneous foundations. The solution to the problems of minimizing accidents when locating production wells in the permafrost zone of the Yamal Peninsula is considered using the example of an oil and gas condensate field and restoring of the temperature regime of perennial unfrozen soils in areas of valve units of main gas pipelines. An assessment of methods used to maintain the industrial and residential infrastructure within the northern municipalities that ensure the functioning of the fuel and energy complex of the Russian Federation in the Arctic was made. The systems of thermal stabilization in the foundations of buildings and industrial facilities built and operated on permafrost soils allow to fully use the high strength and low deformability of frozen grounds. It ensures the state's long-term plans of the industrial development in the Arctic.
Introduction
The land using of permafrost areas in conditions of a changing climate is associated with the risk of reducing the stability of buildings and structures for industrial and civil purposes, as well as causing damage to the environment [1, 2]. For example, the length of pipe sections with a high risk of failure because the freezing-thawing and corrosion processes is 2.4 and 6.7 %, respectively, for the East Siberia – Pacific Ocean (ESPO) pipeline [3]. The main causes are well known: a strong, often multiple, decreasing of the bearing capacity of frozen grounds during their heating or thawing [4-7]; activation of engineering-geocryological processes; errors at all stages of the life cycle of structures (from design to operation) [8, 9].
The corresponded physicochemical factors and mechanisms are known and described separately in old and new textbooks, both qualitatively and numerically. However, their joint quantitative assessment is limited by the practical impossibility of obtaining all the numerical parameters necessary for calculations that is due for making optimal design decisions. This applies not only to difficult-to-determine parameters, but also to those that are mass-measured, due to their high spatial and temporal variability. The ice content and temperature of frozen deposits, as well as their state (frozen/thawed) are such parameters. At a distance of several meters, their values can differ by several times [10], which does not allow us to correctly identify the engineering-geological elements necessary for the design. The uncertainty of the time component of the variability significantly reduces the reliability of the permafrost forecast.
This poses an urgent problem of control and management of thermal processes in the permafrost interacting with structures. The solution is based on the typification of factual and possible combinations of natural and technical components of the natural-technical system – NTS [11]. NTS are cha-racterized by their inherent thermal and mechanical properties and the development of destructive processes, namely their qualitative description and numerical indicator – the average value and the measure of scattering (dispersion and variation limits). The taking into account of ongoing changes is necessary for adequate description of the NTS properties. The state monitoring of permafrost should be used to manage the NTS. The monitoring must be an interdepartmental and multidisciplinary system that includes the following actions: regular observations, collection, accumulation, processing and analysis of information; assessment of the dynamics of permafrost both in natural conditions (“background” monitoring) and in developed territories (geotechnical monitoring – in terms of permafrost); making forecasts of changes in the permafrost zone under the influence of natural factors and technogenesis; development of methods and standards for regulating the state of permafrost for the rational use of cryogenic resources and sustainable development [12].
Background monitoring of permafrost should be considered as an interdepartmental system of long-term observations, data collection, processing and analysis, forecasting the state of permafrost in natural conditions [12]. Background geocryological monitoring should track the natural state and spatial and temporal variability of conditions at background observation-polygons (the area tied to the management nodes) and at one or two background geocryological stations. World experience shows that such ideas of background monitoring are only a prospect both abroad and in Russia. However, already existing background networks and predictive geocryological calculations make it possible to track similar actual natural patterns of different spatial scales [13-15].
Geotechnical monitoring is a complex of regime instrumental observations of the state of capital construction objects, including soil massifs in their foundations, in order to prevent the development of unacceptable deformations of foundations, foundations and other supporting structures. In the case of sites located in the permafrost zone, the long-term instrumental observations are carried out over the thermal and humidity regimes of permafrost soils and soils of the active layer in the territories and sites involved in economic activity. Also the observation of the development of exogenous permafrost-geological processes is important to assess their dynamics and making a forecast of changes under the influence of natural and man-made factors. This ensures reliable environmentally safe operation of engineering structures throughout their life cycle.
The experience of developing the Arctic zone and the northern regions as a whole is a slow gradual transition from the approach of committing and then correcting engineering errors to technologies for monitoring, adapting, predicting and preventing non-design, including emergency, situations. That is mostly important for large investment projects [16, 17]. The gradual introduction in recent decades of the monitoring concept and the expansion of the components of the forecast for the development of geocryological conditions and conditions lead to a reduction in social, economic and environmental costs of management. The implemented engineering and technological solutions were both large and local [18, 19]. Some technical solutions are discussed in this article.
Underestimation of permafrost conditions as a systemic miscalculation in the design of capital construction projects in the permafrost zone
The experience of operating capital construction projects in the North shows that many of them built in the 1970-1980s without proper geocryological and geotechnical forecasts and in conditions of an imperfect regulatory design framework, have fallen into an unacceptable technical condition due to loss of stability of the bases and foundations. This negative process was accompanied by significant material damage.
The absence or neglect of materials from advanced engineering-geocryological research and mapping, as well as regime monitoring observations in the territories of the deposits, was a major miscalculation in justifying the safe operation of gas industry facilities in different regions of the West Siberian North.
During the development of hydrocarbon deposits in the West Siberian North from the beginning of the 1970s and approximately until the mid-late 1990s, when designing bases and foundations on the permafrost, projects declared the first principle of their construction and exploitation. However, in a significant number of cases, special solutions for preserving soils in a permafrost state and the design level of their thermal regime, with the exception of ventilated underground floors in the foundations of buildings, were not provided. The thermal regime of permafrost soils in the foundations of large industrial sites, in areas where branched pipeline systems are located, in the wellhead zones of production wells, the foundations of linear and other structures was formed under the influence of a complex of random factors, which, under conditions of direct and indirect thermal impact of engineering objects on built-up areas, in large number cases led to permafrost degradation. Design organizations used to make and continue to make obvious mistakes. The most typical example is the situation when pipelines with a positive temperature of the circulating product were laid underground next to buildings and structures built according to the first principle, or even within them. Separately, it should be noted the solutions for laying large-diameter gas pipelines (1020-1420 mm) with positive gas temperatures in permafrost soils.
Thus, at one of the site of integrated gas treatment units of the Medvezhye oil, gas and condensate field, operating since 1978, the permafrost table of the permafrost group turned out to be buried by 5-15 m for 20 years. But it was characterized by the continuous spread of permafrost soils with a temperature of –2÷–3 °С during engineering-geological surveys in 1976 (Fig.1). On the Nadym – Punga gas pipeline, not far from the main compressor station, seismic monitoring has revealed the formation of an 8 m deep thawing bowl since 1972. This indicates a complete (in some cases) ignorance of the geocryological forecast during the development of the project [20].
The results of geotechnical monitoring carried out at the Medvezhye field since 1988-1989 indicate that the deformations of the structures were massive. In the 1990s, about 20 % of foundation piles in the bases of technological buildings, equipment and pipelines experienced deformations of varying nature and magnitude. About 12 % of the observed piles were subject to deformations exceeding the permissible values accepted for the corresponding structures [21]. A similar situation with the development of deformations of gas field structures was observed at the Urengoy and Yamburg fields.
The results of the analysis indicate that at the initial stages of development of hydrocarbon fields in the Western Siberia North in the 70s of the 20 century, there was a lack of in-depth background geocryological and geotechnical monitoring, as well as geocryological forecasts at the regional and facility levels. It led to significant complications of their exploitation, as well as the operation of gas transport systems. According to available data the development of deformations of gas production facilities built in the 1970-1980s was the cause of 23 % of the failure of technical systems and 29 % of the loss of gas production.
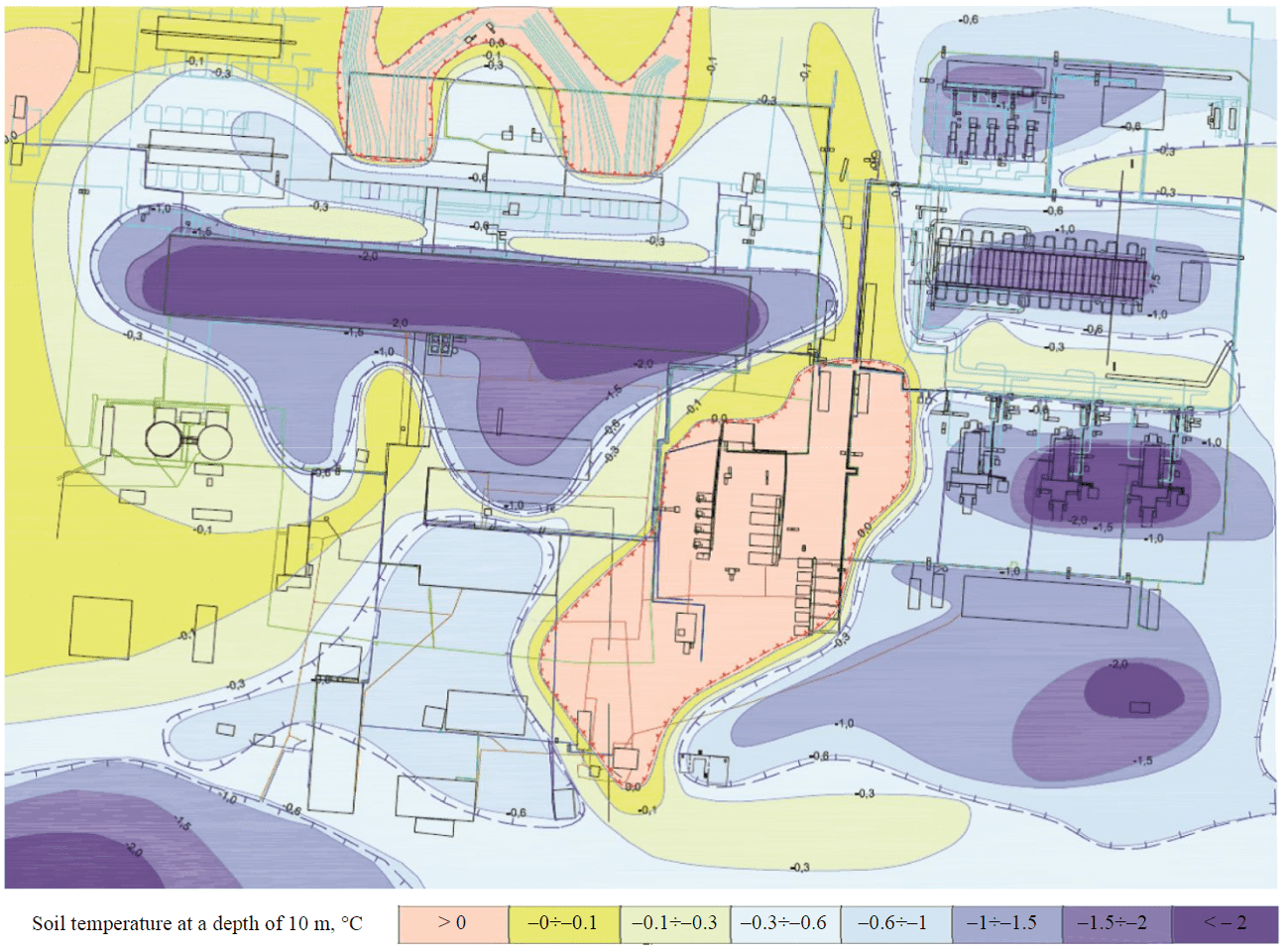
Fig.1. Scheme of geocryological zoning of the gas field site N 9 of the Medvezhye oil and gas condensate field (2008)
Methods for permafrost industrial development
The beginning of the 2000s can be attributed to the start of the II stage of northern territories of Western Siberia development. It is characterized by a more competent development of new technical solutions for the constructions on permafrost based on existing generalized experience, using of new technologies for the constructions and the improvement of calculation methods and development of the regulatory framework and the results of the departmental geotechnical monitoring systems functioning. At the same time the priority principle for selecting sites for technological objects has become the uniformity of engineering-geological (geocryological) conditions of industrial sites within the framework of project development. A successful example of the implementation of this approach is the general plan for the placement of a booster compressor station of the first stage at the Yubileiny oil and gas condensate field. The original layout of the facilities was abandoned (Fig.2, a) and significantly adjusted (Fig.2, b) during the design process. In the final version of the plan, the main objects of the booster station are located in the most favorable conditions (on a thawed foundation). The other objects are located in relatively homogeneous geocryological conditions.
The southern geocryological zone is characterized by intermittent distribution of permafrost. The most rational solution for construction within the ongoing warming and permafrost degradation is to search sites composed of thawed or predominantly thawed soils.
The experience of operating the antenna field of the Gakona Observatory (Central Alaska) is one of the examples of the need for a flexible regime for controlling the temperature regime. The basis of the antenna field is a 0.5 m thickness gravel platform. It is located within the vast intermountain depression of the river Copper, which is characterized by continuous distribution of high-temperature permafrost. The intermountain basin is filled predominantly with high-icy glaciolacustrine sediments of submarine alluvial deposits (varved clays, diamictites, sands, and lahar deposits) [22]. The average annual air temperature in the Gakona region increased from –3.5 °C in the early 1950s to –1.6 °C by the early 2000s. The permafrost has a non-gradient-temperature distribution in the depth range of 3-30 m and the temperature is –0.6 °C. Seasonal fluctuations fade away at a depth of 3 m, which is due to the fact that heat flows are spent on phase transitions in the soil. Gravel backfill shifted the phase equilibrium and led to a partial lowering of the permafrost table to 5 m and to thermal subsi-dence of the gravel base in 2004. Building of seasonal cooling devices, on the contrary, led to differential heaving on the site, which, as well as thermal subsidence, again disrupted stability of engineering structures.
Obviously, climate oscillations in the future will actively influence the stability of the foundation and require control of the soil cooling regime under each structure. The condition of it should be determined based on geotechnical monitoring data. It is also obvious that maximum homogeneity of engineering-geocryological conditions should be one of the priority requirements for the placement of major geotechnical systems in the area of permafrost [23].
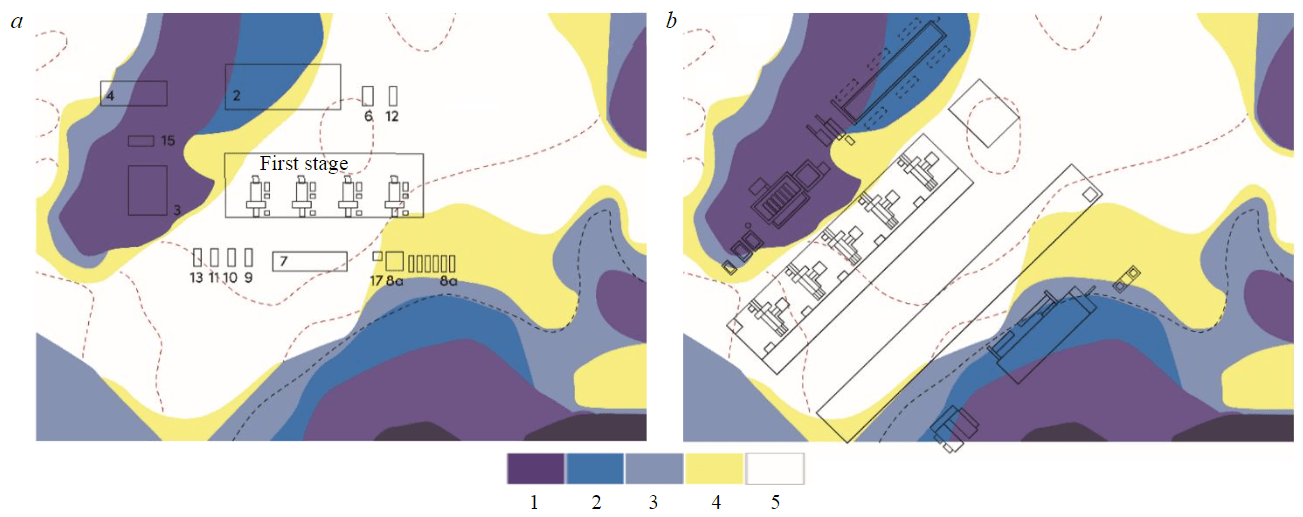
Fig.2. Distribution and temperature of frozen rocks at the booster compressor station of the first stage of the Yubileinoye oil and gas condensate field: а – initial version of the general plan; b – working draft
1, 2 – permafrost soils of the confluent type with an average annual temperature –1÷–2 °C (1) and –0.5÷–1 °C (2); 3 – frozen and thawed soils with an average annual temperature of about 0 °C; 4 – permafrost soils with a table buried 5-9 m; 5 – thawed soils
The active introduction into practice of construction and restoration work of vapor-liquid thermal stabilizers of various designs to control the thermal regime of degrading frozen foundation soils and ensure the design life of capital construction projects is the main novelty of the second period of development of the northern territories.
Objectives and situations in which thermal stabilizers are used to provide the required level of load-bearing capacity:
- freezing and lowering the temperature of thawed or plastically frozen soils during the construction of facilities in order to ensure homogeneous geocryological conditions of sites and the level of bearing capacity of permafrost, which is necessary to transfer the load to the foundations;
- ensuring the design thermal regime of permafrost in conditions of climatee changing and increased snow accumulation in built-up areas, neutralizing the heat load from engineering structures during their operation;
- restoration of the thermal regime of permafrost in the foundations of construction which disturbed due to the influence of natural and climatic factors, erroneous design decisions and mistakes during exploitation;
- ensuring the design thermal regime of permafrost in the foundations of specific objects: hydraulic structures, underground fuel-generating facilities, general industrial buildings without ventilated underground floors in the foundations, etc.
Introduction of an object-based geocryological forecast for the sites of buildings, production wells and other engineering structures, including those characterized by the presence of heat sources, predetermined possibility of influencing the thermal state of frozen foundation soils.
Preliminary freezing (cooling) of foundation soils during construction on heterogeneous foundations
When large industrial and civil facilities are located in the fields of the Nadym-Purovsky interfluve, where the distribution of permafrost is intermittent, the development areas often have sharply heterogeneous permafrost-geological conditions (merging and non-merging permafrost, thawed soils). Structures are located in relatively homogeneous permafrost conditions if it possible. However, it is not always possible. As exemplified by the construction sites of a gas treatment facility at the Yubileiny oil and gas condensate field, a booster compressor station at the gas field N 6 of the Medvezhye oil and gas condensate field and a number of other facilities. This required the development of technical solutions for freezing talik zones in the contours of a number of structures in various geological and permafrost conditions.
Thus, at gas field N 6 of the Medvezhye field in the zone of discontinuous permafrost there were loamy plastic-frozen soils of the confluent type, frozen soils with a buried permafrost table and thawed soils at the base of the compressor of the booster compressor station. Modeling of the freezing process of the foundation soils of the designed compressor shop showed that the necessary thermal stabilization of the thermal field can be achieved during three winter periods by immersing three gas pumping units and their piping into the foundation soils of 1300 cooling columns of vertical natural temperature stabilization systems (Fig.3). The results of this decision was confirmed by the validity of subsequent monitoring.
A complicating factor in such freezing is heaving. The temperature within the newly formed frozen soil mass decrease in winter to –8÷–11 °C (significantly lower than –1.5 °C required to ensure the required level of bearing capacity of the soil at the base of the booster compressor station). However, uneven heaving and unacceptable deformations were avoided due to the fact that the heaving of soil masses with a distributed cooling pulse from the vertical natural temperature stabilization systems occurred relatively evenly. The capacity of the cooling system turned out to be excessive and was reduced through a calculated periodic shutdown, which eliminated “freezing” of the soil mass during the operation of the facility.
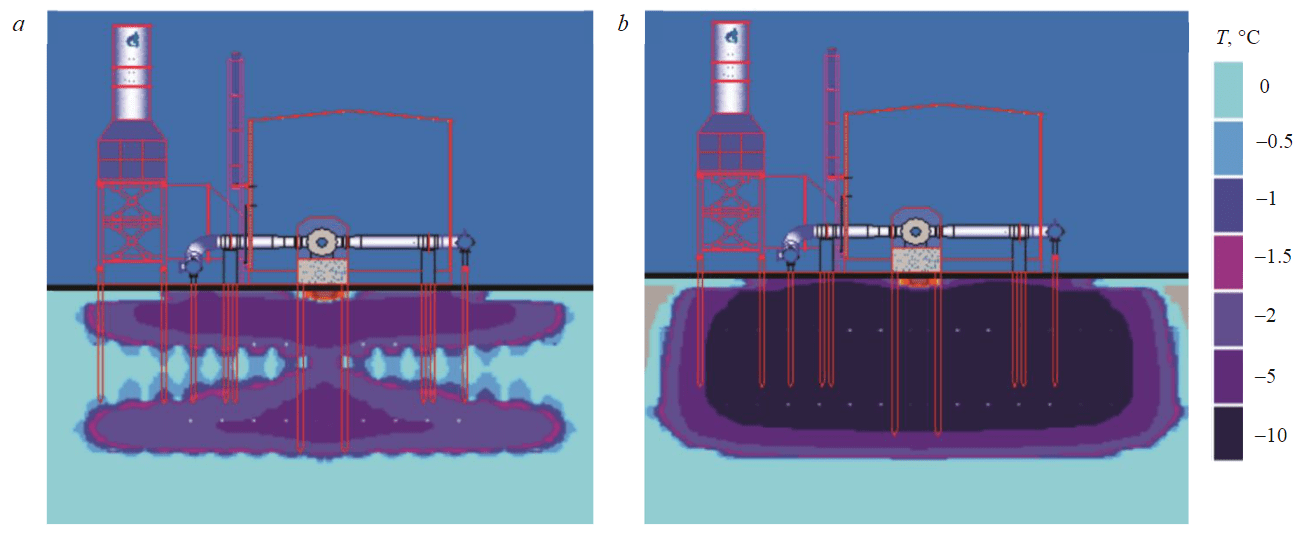
Fig.3. Results of the soil the thermal regime modeling under the compressor place at gas field N 6 of the Medvezhye field: a – april 2013; b – april 2015
On the territory of the Bovanenkovskoye gas condensate field in the common floodplain of the large Se-Yakha and Mordy-Yakha rivers in Central Yamal the freezing foundation soils during the construction of engineering structures was used. Here the permafrost is continuous and this is optimal for construction. However, since the 1980s, the background average annual permafrost temperatures have increased by ~2-2.5 °C. As a result, the calculated level of bearing capacity of pile foundations is far from corresponding to the real situation due to the low strength and deformation properties of high-temperature and saline sandy-loamy frozen deposits.
During the construction of new objects, the thermal stabilizers are immersed simultaneously with the piles. The transfer of a payload to the foundation is allowed after successful testing of piles with loads and reaching the design soil temperatures. Thermal stabilizers remain in the ground for the period of operation of objects in conditions of increased snow accumulation and densely built industrial sites. They ensure the stability of buildings in the presence of thermal influences in conditions of long-term climate warming. In this case, the duration of construction increases by several months, waiting for the soil to cool to the calculated values. To reduce the construction time, the forced soil cooling is sometimes used during the warm season.
Ensuring the design thermal regime around wellhead zones
The intense thermal effect of gas production wells on permafrost leads to the formation of a thawed ring zone around the wellbore. The consequence is surface subsidence, longitudinal bends of casing strings, violation of their tightness, loss of stability of pile foundations and the development of unacceptable deformations of auxiliary pipelines and the appearance of gas in the annulus [24, 25].
There are two main stages to overcome complications when locate production wells in the permafrost zone of the Yamal Peninsula – the well locations are optimized and the thermal state of permafrost is managed in the wellhead zones [26, 27].
Thus, at the Bovanenkovskoye gas condensate field, the clusters of gas production wells were placed according to specialized geocryological survey data and zoning based on the magnitude of soil subsidence after permafrost thawing. On such a map, the areas are shown in different colors based on the traffic light principle. Red color corresponds to unfavorable areas of watershed terraces of marine and ice-marine origin with ice deposits. Green color corresponds to relatively favorable areas.
Using such a map, 8 out of 56 cluster pads of gas wells in the field (every seventh well) were moved to areas with more favorable geocryological conditions within the reservoir management operating plan limits.
This made it possible to avoid the thick layers of high-ice deposits and sheet ice presence. This procedure has been recognized as effective and is used in the regulatory document of the Gazprom company – “Methodology for conducting geocryological studies during exploration and development of fields” (STO Gazprom 2-3.1-233-2008).
However, there are very few favorable territories. The sites of gas well pads fall into conditions that are conditionally favorable for the construction and operation of wells. Therefore, the projects provide for temperature stabilization of permafrost in the wellhead zones of production wells. This happened for the first time in Russia [28]. Thermally insulated lift pipes with vacuum thermal insulation are used as technical columns for production wells instead of conventional pump and compressor pipes in the depth range of 0-50 m. This limits the thermal impact on icy sedimentary rocks [29, 30]. In the wellhead zones of wells in the depth range of 0-15 m, the construction of vertical thermal stabilization systems is provided to prevent thawing of the most icy and subsidence layer of permafrost (Fig.4) [31].
Ten years of experience in thermal stabilization in the wellhead zones of wells at the Bovanenkovskoye field has paid off. Complications associated with loss of stability of well support, foundations of their sites and wellhead piping foundations in the well stock where complex solutions for thermal stabilization are installed have not been identified.
At the Kharasaveyskoye gas condensate field, located north of the Bovanenkovskoye field in even less favorable geocryological conditions, including a large thickness of a richly icy layer, a wider range of thermal stabilization measures is provided for production wells for their safety:
- thermally insulated casing pipes for boreholes (thermocases) with polyurethane foam thermal insulation in the depth range of 0-40 m;
- vapor-liquid seasonally operating cooling systems in subsurface zones with an increased descent depth relative to the Bovanenkovskoye field (up to 40 m);
- heat-insulated elevator pipes with increased descent depth (up to 150 m).
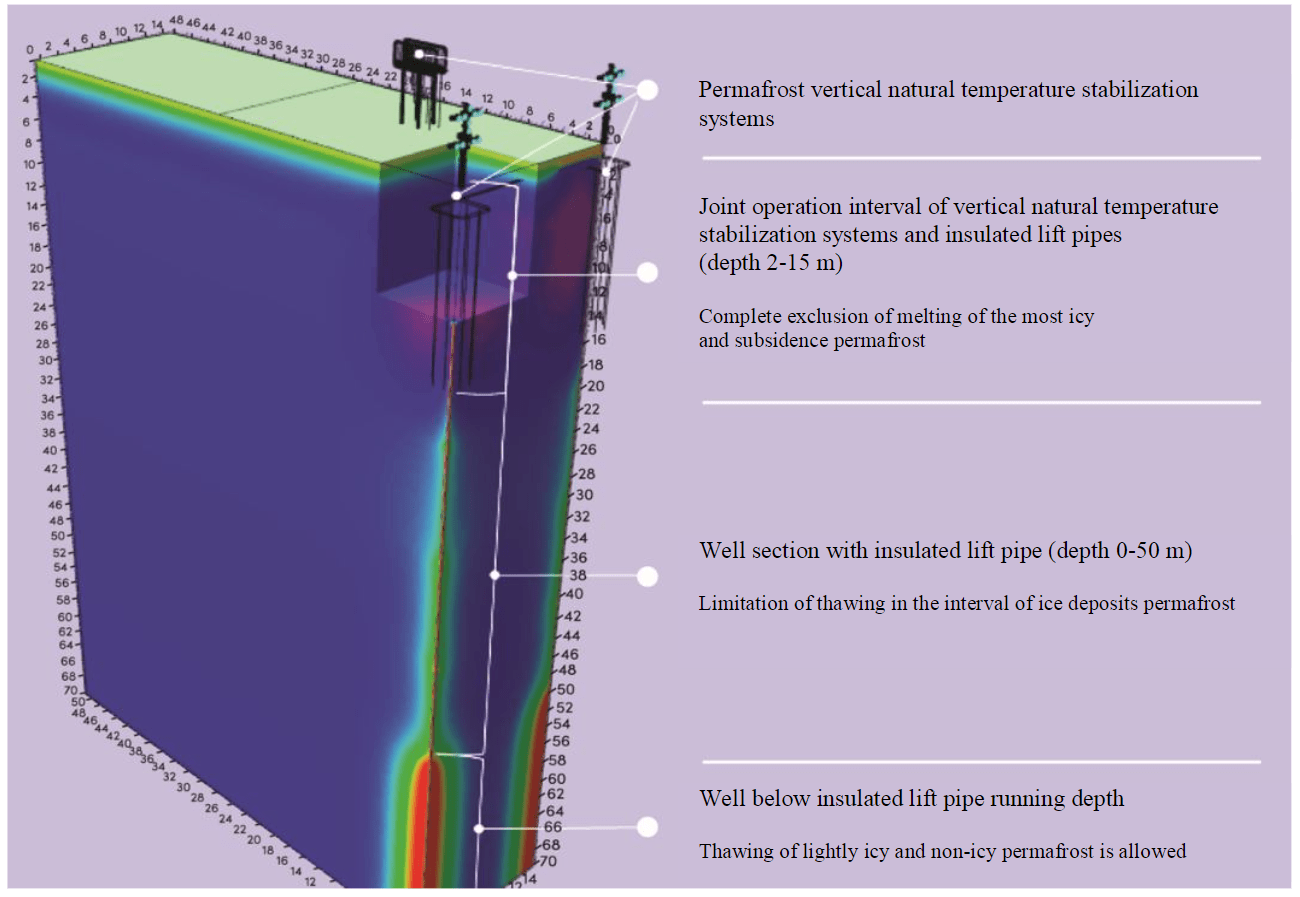
Fig.4. Temperature stabilization systems in wellhead zones of gas wells (according to thermal engineering modeling data of VNIPIgazdobycha Company)
This combination of solutions was chosen based on the results of thermal engineering calculations and assessment of the stability of the well supports according to Standard STO Gazprom 16-2005.
Restoration of the temperature regime of frozen ground in the vicinity of crane nodes of main gas pipelines
Deformations of crane nodes of connection pipelines are typical for the gas complex of the Nadym-Purovsky interfluve region. The region is dominated by discontinuous permafrost of mainly clay and sandy composition and a low ice content in the near-surface layer. At the sites of the crane nodes, when there is no permafrost or Isolated frozen patches are presented, the so called II principle of construction was used – with thawing of the permafrost. As a result of the degradation of frozen ground under the pile foundations of cranes nodes the multidirectional deformations were started. They were caused by both sediment during thawing and pile heaving. In some cases, this required repair work in order to eliminate the stress-strain state of the pipelines and restore their design spatial position.
Thermal stabilization was used to preserve frozen soils in areas of permafrost with a temperature close to 0 or with a lowered permafrost table. It gave a positive effect already in the first year of its operation (Fig.5) by preventing or suspending the development of deformations caused by the thawing of frozen ground and the frost heaving. To stabilize the crane nodes, the pipelines were insulated with 100 mm thick foam polystyrene shells.
In general, the experience of operating crane units of main gas pipelines show that the implementation of the thermal stabilization of the foundation ground indicates the cessation of deformations during one or two winters after the completion of work and ensuring the stability of structures for a long period (up to 20 years).
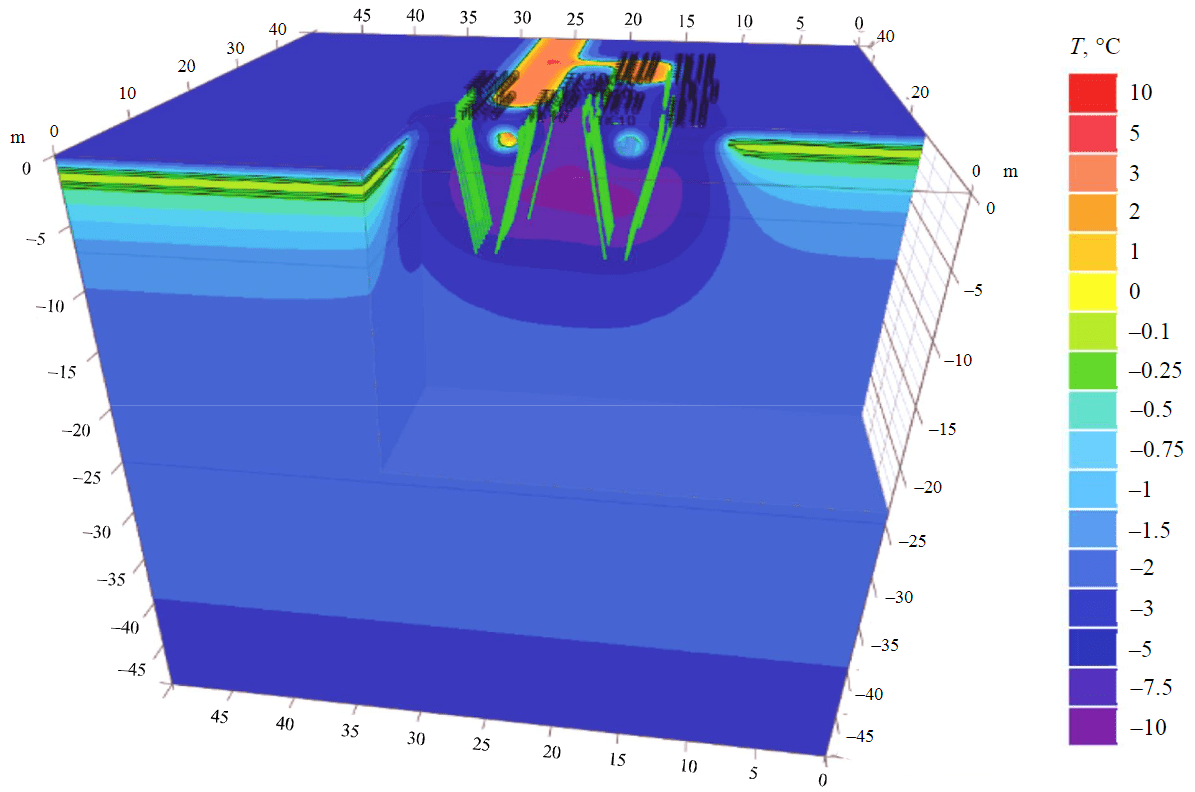
Fig.5. Results of thermal engineering calculations to justify decisions on restoring frozen soils strength of the base of the connection point on the main gas pipeline
Ensuring the stability of main gas pipelines in the Yamal Peninsula
Special attention should be paid to the solutions provided for ensuring the stability of large-diameter gas pipelines (1420 mm) in particularly complete geocryological conditions of the Yamal Peninsula. The negative experience of transporting gas with a positive temperature from the Medvezhye, Urengoy and Yamburgskoye fields in the Nadym-Pur-Taz region through main gas pipelines built in the 1970s and 1980s on areas of continuous and intermittent permafrost was taken into account. When designing gas pipelines from the Bovanenkovskoye and Kharasaveyskoye fields on the Yamal Peninsula, decisions were made providing for the transportation of gas through the peninsula exclusively with negative temperatures. For this purpose, low-temperature separation using turbodetander units has been adopted for gas preparation for long-distance transport as the main drying technology. This solution allows year-round gas supply to the Bovanenkovo – Ukhta main gas pipeline system with a temperature no higher than –2 °C. This decision fully justified itself. For more than a decade of operation of the Bovanenkovskoye field, there has been no loss of bearing capacity of foundation of the main gas pipelines. There has been no pipeline ascent, accompanied by the development of deformations.
Thermal conditions and deformations of industrial and residential buildings
In built-up areas, as a result of natural and anthropogenic changes, the accident rate of geotechnical systems is growing. This situation is mostly caused by unjustified economy at the stage of engineering surveys, outdated standards, violation of the operating regime. Deformations are often so significant that structures cannot correspond to operational requirements anymore, and in some cases they must be dismantled. This happens in all regions of the Arctic zone of Russian Federation, but is most resonantly reflected in multi-storey urban buildings (Vorkuta, Salekhard, Nadym, Novy Urengoy, Norilsk, Yakutsk, Magadan, Anadyr). The reason of this situation in most cases is the loss of the bearing capacity of the frozen grounds due to the thawing under the thermal effect of buildings and structures, as a result of errors and violation of the rules for operating facilities. A decrease in the bearing capacity of grounds and the development of deformations occurs and at negative temperatures, in cases where the soils are saline, as example in Norilsk. At the same time, temperature monitoring of the thermal state of the soils is not sufficient. Geophysical studies, in particular engineering seismic survey, are required to establish the physical state of the soils.
The problem of “disappearance” of permafrost under buildings was encountered in the cities of Yakutia, where the effect of warming was significantly supplemented by technogenesis. The closure of the foundations makes it almost impossible to carry out control mining and drilling operations under the facilities, therefore, the use of geophysical work and well drilling around the perimeter of buildings comes to the forefront of engineering-geological surveys.
Seismic methods provide unambiguous identification of frozen rock in the conditions of sand-clay section [32]. Their use is effective in built-up areas under residential buildings and within opera-ting enterprises with any lithological composition of dispersed soils. Depending on the specific conditions, refracted or reflected, preferably transverse SH waves are used.
In the works [32, 33] there is a comparison of the results of thermal and seismic surveys performed in Mirny (Republic of Sakha (Yakutia), where they were used to determine the configuration of man-made taliks, the formation of which is the cause of deformation and emergency state of a group of buildings. Seismic surveys were conducted directly under the houses, and thermal surveys were conducted in 10 wells located on the outside of the houses. There is a significant difference (up to 4 m) in determining the depth of the permafrost ground by various methods. It should be emphasized that seismic profiling has provided continuous information about the thickness of the talik directly under the buildings, while thermometry to characterize their base is less reliable due to the geometry of the location and thermal imperfection of the wells.
Engineering seismic survey also allows to understand the nature of deformations of structures when they are mistakenly associated with temperature changes in the soil thickness. Figure 6 shows the results of studying the situated above the permafrost talik under a house built on alluvial soils with a thickness of 8-13.5 m. The house is subject to deformations that appear in the form of cracks in the middle of the building.
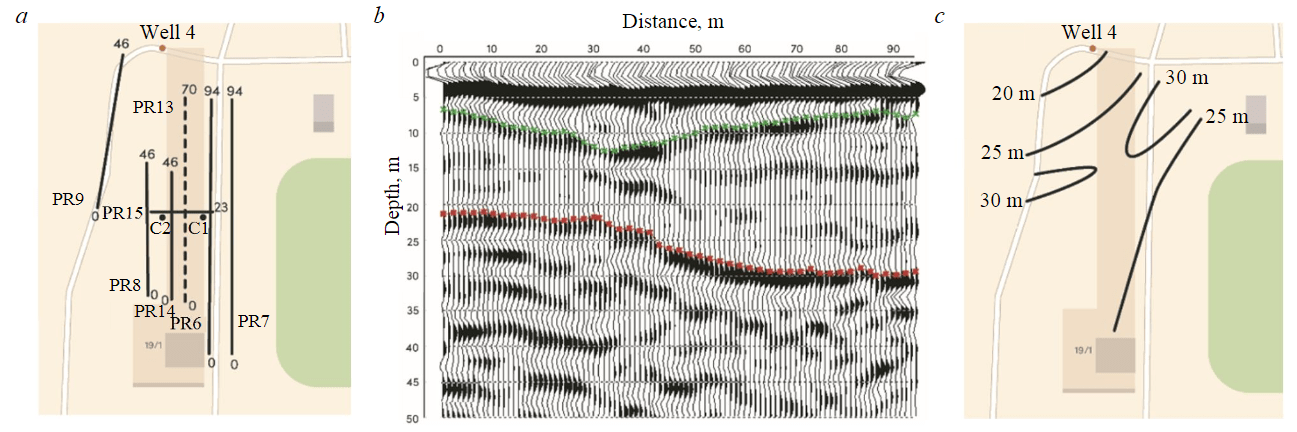
Fig.6. Results of seismic surveys near of the house N 19 (quarter N 202, Yakutsk): а – seismic observation network diagram (dotted line – non-longitudinal profiling); b – depth section along profile 6 (PR6); c – permafrost table isolines
Measurements were carried out using reflected SH-waves along a network of profiles located both outside the building and directly below it, in a ventilated underground, as well as using non-linear profiling.
The boundary corresponding to the top layer of permafrost is confidently distinguished in the deep section. A boundary (green line) is fixed above, which is probably associated with the bottom of the alluvial soils. The immersion of the permafrost top layer up to 30 m is due to the presence of a channel filled with alluvial soils. The configuration of this duct is well manifested on the plan of the isolines of the top layer of permafrost. The depth of the top layer of permafrost determined by seismic exploration is well consistent with the drilling data of well 4, which made it possible to unambiguously identify and contour the top layer in plan. The interval in which the deformations of the building appear practically corresponds to the interval in which the top layer of permafrost decreases on profile 6 and differs in the maximum thickness of the alluvial soils. This suggests that one of the reasons for the deformation of the building may be the unevenness of soil conditions within the thaw zone.
In the cities of the Yamalo-Nenets autonomous okrug, some of the buildings received critical damage and were decommissioned and dismantled ahead of schedule, including buildings built quite recently. The cause of unacceptable deformations is degradation of permafrost soils at the base [34], most often as a result of errors during survey and design work. A positive experience of the construction of previous years could also cause underrating of all existing risks. So, in Nadym, for the first five-story series of residential buildings, a special site was determined, characterized by the spread from the surface of mainly melted sandy soils with thin lenses of slightly icy permafrost. Basically, five-story large-panel buildings on ribbon and pile foundations were built here (II principle of construction). This practice has proven itself well, there were no significant uneven deformations. However, over time, the number of storeys of construction was gradually increased, and new construction sites shifted to zones where geocryological conditions turned out to be more difficult: thaw and low-icy frozen alluvial sands on a number of sites were underlain by permafrost highly icy loams of coastal-marine genesis, not identified due to insufficient depth of engineering and geological surveys. Icy loams in the foundations of buildings fell into the heat zone of buildings built with warm basements, and uncontrolled deformations began. By this time even under the old five-story houses, the heating zone in places reached icy loams, where uneven deformations also began. As a result, since the mid-90s of the 20th century, 12 residential buildings and a number of industrial buildings were dismantled in Nadym (and Novy Urengoy), and cement injection was used to prevent further destruction, compensating for the filtration consolidation of thawing soils.
Municipal services of Nadym and Salekhard have a positive experience of restoring the bearing capacity of permafrost soils of foundations degraded under the thermal influence of buildings using forced freezing (restoration of permafrost co-standing) of soils and lowering their temperature by using vapor-liquid termostabilizers in combination with thermal insulation of the floor surface in basements (Fig.7).
In Nadym, similar solutions were implemented in 2003-2004 in the foundations of two buildings subject to uneven sags. After the recovery of the solid-frozen state of the soils, the deformations of the buildings ceased, the stable state of the bearing structures has been ensured for almost 20 years.
At the same time, taking into account the changing geopolitical conditions, it is important to understand the capabilities of companies specializing in the production of thermal soil stabilizers and the provision of related services in Russia. This issue is especially relevant in the context of the need for import substitution and the likely growth in demand in the near future due to the increased impact of climate change on the state of permafrost.
At present, about ten key companies produce soil thermal stabilizers in Russia. Among them, there are both narrow-profile, specializing exclusively in the production of thermal stabilization systems, and companies with a much-diversified type of activity related to the production of a wide range of construction nomenclature. According to open sources, most companies produce about 2.5-3.5 thousand soil thermal stabilizers per month, and more highly specialized companies produce up to 10 thousand units. On average, according to “Foundationstroyarkos”, the specific cost of stabilizing a square meter of frozen soil in a building plan is from 10,000 to 80,000 rub./m2. Such a spread in prices is associated with technical solutions and prices depending on the category of soil and the depth of drilling [35].
Most organizations – manufacturers of technical solutions for soil thermal are mainly focused on the consumer from the enterprises of the fuel and energy complex, primarily the oil and gas industry, energy facilities. In the social sphere, one can cite the example of Norilsk city, where a large-scale program for the renovation of the housing stock, social and logistics infrastructure (including main gas pipelines, airports, etc.) has been started. This program includes, among other things, measures for the thermal stabilization of the soils of the buildings and structures foundations (inclu-ding major pipelines, airports etc.).
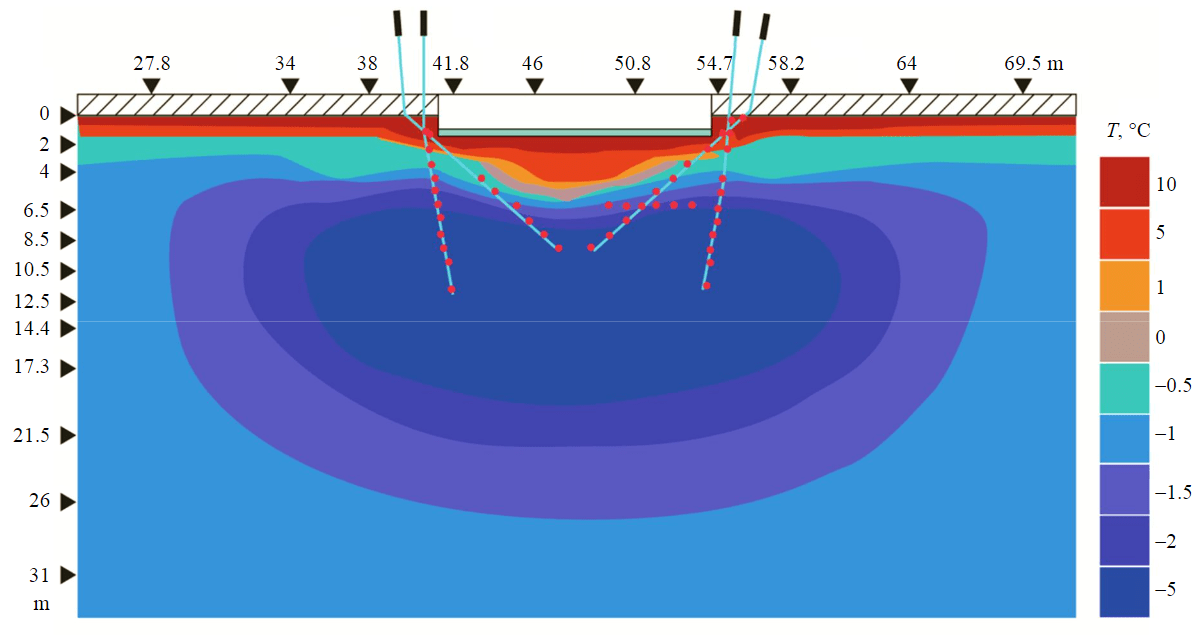
Fig.7. Results of calculations of freezing of a thawing bowl at the base of a residential building in Nadym at the end of the third year of operation of the thermal stabilization system
The main customers of works related to the soil thermal stabilization are localized in the most economically developed regions of the permafrost zone, specializing in the extraction of hydrocarbon raw materials: Yamalo-Nenets autonomous okrug, Krasnoyarsk krai, Irkutsk oblast, Republic of Sakha (Yakutia), Tyumen oblast, Nenets autonomous okrug. Individual auctions are held by organizations from the Komi Republic, Zabaykalsky krai, Amur, Murmansk, Arkhangelsk and Sverdlovsk oblasts.
Conclusion
The main problems and risks of the economic development of the Arctic are due to the complexity and mosaic nature of the geocryological structure and the thermal state of the permafrost zone, the insufficient knowledge of the developed regions and the unsatisfactory quality of surveys, design and construction, as well as the lack of a unified system of background and geotechnical monitoring of changes occurring in the permafrost zone.
Climate change and related permafrost degradation processes have significantly complicated the construction of new industrial and civil buildings and structures in the permafrost zone as well as the operating conditions of existing ones.
The development of oil and gas condensate fields in the North of Western Siberia and related technological problems, in essence, predetermined the practical development of the foundations of geotechnical monitoring based on geocryology science. The departmental level of geotechnical mo-nitoring solves the problems of ensuring accident-free operation of production facilities, but it seems insufficient to solve the strategic tasks of reducing the risks of economic development in new areas of the permafrost zone, which is far from being fully provided with regional geocryological surveys and monitoring observations.
Significant progress has been made over the past two decades in the application and provision of a regulatory framework for modern sustainable green technologies. This allow to solve a wide range of geotechnical tasks in the permafrost zone in the design and construction of buildings and structures, in the arrangement of production well systems and various types of transport systems that ensure long-term reliability of bases and foundations on permafrost soils.
The creation of thermal stabilization systems in the foundations of buildings and structures on permafrost makes it possible to use fully one of the main resources of the cryolithozone – the high strength and low deformability of frozen soil massifs, which, under a controlled design thermal regime, provide the design level of their bearing capacity.
References
- Zhdaneev O.V. Technological sovereignty of the Russian Federation fuel and energy complex. Journal of Mining Institute. Vol. 258, p. 1061-1078. DOI: 10.31897/PMI.2022.107
- Litvinenko V.S., Petrov E.I., Vasilevskaya D.V. et al. Assessment of the role of the state in the management of mineral resources. Journal of Mining Institute. Vol. 259, p. 95-111. DOI: 10.31897/PMI.2022.100
- Mingtang Chai, Guoyu Li, Wei Ma et al. Assessment of Freeze – Thaw Hazards and Water Features along the China – Russia Crude Oil Pipeline in Permafrost Regions. Remote Sensing. 2020. Vol. 12. Iss. 21. N 3576. DOI: 10.3390/rs12213576
- Suter L., Streletskiy D., Shiklomanov N. Assessment of the cost of climate change impacts on critical infrastructure in the circumpolar Arctic. Polar Geography. 2019. Vol. 42. Iss. 4, p. 267-286. DOI: 10.1080/1088937X.2019.1686082
- Streletskiy D.A., Suter L.J., Shiklomanov N.I. et al. Assessment of climate change impacts on buildings, structures and infrastructure in the Russian regions on permafrost. Environmental Research Letters. 2019. Vol. 14. N 2. N 025003. DOI: 10.1088/1748-9326/aaf5e6
- Timofeev A.V., Piirainen V.Y., Bazhin V.Y., Titov A.B. Operational Analysis and Medium-Term Forecasting of the Greenhouse Gas Generation Intensity in the Cryolithozone. Atmosphere. 2021. Vol. 12. Iss. 11. N 1466. DOI: 10.3390/atmos12111466
- Post E., Alley R.B., Christensen T.R. et al. The polar regions in a 2 °C warmer world. Science Advances. 2019. Vol. 5. Iss. 12. N DOI: 10.1126/sciadv.aaw9883
- Raynolds M.K., Walker D.A., Ambrosius K.J. et al. Cumulative geoecological effects of 62 years of infrastructure and climate change in ice-rich permafrost landscapes, Prudhoe Bay Oilfield, Alaska. Global Change Biology. 2014. Vol. 20. Iss. 4, p. 1211-1224. DOI: 10.1111/gcb.12500
- Melnikov V.P., Osipov V.I., Brushkov A.V. et al. Past and Future of Permafrost Monitoring: Stability of Russian Energetic Infrastructure. Energies. 2022. Vol. 15. Iss. 9. N 3190. DOI: 10.3390/en15093190
- Min Liew, Xiaohang Ji, Ming Xiao et al. Synthesis of physical processes of permafrost degradation and geophysical and geomechanical properties of permafrost. Cold Regions Science and Technology. 2022. Vol. 198. N 103522. DOI: 10.1016/j.coldregions.2022.103522
- Ze Zhang, Wei Ma, Zhongqiong Zhang. Scientific concept and application of frozen soil engineering system. Cold Regions Science and Technology. 2018. Vol. 146, p. 127-132. DOI: 10.1016/j.coldregions.2017.11.017
- Melnikov V.P., Osipov V.I., Brushkov A.V. et al. Development of geocryological monitoring of natural and technical facilities in the regions of the Russian Federation based on geotechnical monitoring systems of fuel and energy sector. Earth's Cryosphere. Vol. 26. N 4, p. 3-18 (in Russian). DOI: 10.15372/KZ20220401
- Hjort J., Karjalainen O., Aalto J. et al. Degrading permafrost puts Arctic infrastructure at risk by mid-century. Nature Communications. 2018. Vol. 9. N 5147. DOI: 10.1038/s41467-018-07557-4
- Flynn D., Kurz D., Alfaro M. et al. Forecasting Ground Temperatures under a Highway Embankment on Degrading Permafrost. Journal of Cold Regions Engineering. 2016. Vol. 30. Iss. 4. N 04016002. DOI: 10.1061/(asce)cr.1943-5495.0000106
- Karjalainen O., Aalto J., Luoto M. et al. Circumpolar permafrost maps and geohazard indices for near-future infrastructure risk assessments. Science Data. 2019. Vol. 6. N 190037. DOI: 10.1038/sdata.2019.37
- Melvin A.M., Larsen P., Boehlert B. et al. Climate change damages to Alaska public infrastructure and the economics of proactive adaptation. The Proceedings of the National Academy of Sciences. 2017. Vol. 114. N 2, p. E122-E131. DOI: 10.1073/pnas.1611056113
- Buslaev G., Tsvetkov P., Lavrik A. et al. Ensuring the Sustainability of Arctic Industrial Facilities under Conditions of Global Climate Change. Resources. 2021. Vol. 10. Iss. 12. N 128. DOI: 10.3390/resources10120128
- Lin Chen, Fortier D., McKenzie J.M., Sliger M. Impact of heat advection on the thermal regime of roads built on permafrost. Hydrological Processes. 2019. Vol. 34. Iss. 7, p. 1647-1664. DOI: 10.1002/hyp.13688
- Loktionov E.Y., Sharaborova E.S., Shepitko T.V. A sustainable concept for permafrost thermal stabilization. Sustainable Energy Technologies and Assessments. 2022. Vol. 52. Part A. N 102003. DOI: 10.1016/j.seta.2022.102003
- Shammazov I.A., Batyrov A.M., Sidorkin, D.I., Van Nguyen T. Study of the Effect of Cutting Frozen Soils on the Supports of Above-Ground Trunk Pipelines. Applied Sciences. 2023. Vol. 13. Iss. 5. N 3139. DOI: 10.3390/app13053139
- Vasiliev G.G., Dzhaljabov A.A., Leonovich I.A. Analysis of the causes of engineering structures deformations at gas industry facilities in the permafrost zone. Journal of Mining Institute. Vol. 249, p. 377-385. DOI: 10.31897/PMI.2021.3.6
- Putikov O., Kholmyanski M., Ivanov G., Senchina N. Application of geoelectrochemical method for exploration of petroleum fields on the Arctic shelf. Geochemistry. 2020. Vol. 80. Iss. 3. N 125498. DOI: 10.1016/j.geoch.2019.02.001
- Syasko V., Shikhov A. Assessing the State of Structural Foundations in Permafrost Regions by Means of Acoustic Testing. Applied Sciences. 2022. Vol. 12. Iss. 5. N 2364. DOI: 10.3390/app12052364
- Eppelbaum L.V., Kutasov I.M. Well drilling in permafrost regions: dynamics of the thawed zone. Polar Research. 2019. Vol. 38. N 3351. DOI: 10.33265/polar.v38.3351
- Chuvilin E., Ekimova V., Davletshina D. et al. Evidence of Gas Emissions from Permafrost in the Russian Arctic. Geosciences. Vol. 10. Iss. 10. N 383. DOI: 10.3390/geosciences10100383
- Zhdaneev O., Frolov K., Petrakov Y. Predictive Systems for the Well Drilling Operations. Cyber-Physical Systems: Design and Application for Industry 4.0. Springer, 2021. Vol. 342, p. 347-368. DOI: 10.1007/978-3-030-66081-9_28
- Gromadsky A.N., Arefiev S.V., Volkov N.G. et al. Remote temperature regime monitoring of permafrost soils under the buildings in Salekhard. Scientific Bulletin of the Yamal-Nenets Autonomous District. N 3, p. 17-21 (in Russian). DOI: 10.26110/ARCTIC.2019.104.3.003
- Melnikov I.V., Nersesov S.V., Osokin A.B. et al. Geotechnical design solutions for gas well construction in extremely severe permafrost conditions of the Yamal Peninsula. Gas Industry. N 12 (794), p. 64-71 (in Russian).
- Chikalov S.G., Pyshmintsev I.Yu., Zaselsky E.M. et al. Experience of application of insulated lift pipes in the conditions of gas fields in the North of Western Siberia. Gas Industry. N 12 (778), p. 38-42 (in Russian).
- Georgiyadi V.G., Agapov A.A., Poverenniy U.S. et al. The use of ultra-thin thermal insulation in the development of deposits in areas of permafrost distribution. Oil Industry. N1, p. 52-57 (in Russian). DOI: 10.24887/0028-2448-2023-1-52-57
- Sharaborova E.S., Shepitko T.V., Loktionov E.Y. Experimental Proof of a Solar-Powered Heat Pump System for Soil Thermal Stabilization. Energies. 2022. Vol. 15. Iss. 6. N 2118. DOI: 10.3390/en15062118
- Sudakova M.S., Brushkov A.V., Velikin S.A. et al. Geophysical methods in permafrost monitoring. Moscow University Bulletin. Series 4. Geology. N 6, p. 141-151 (in Russian). DOI: 10.33623/0579-9406-2022-6-141-151
- Mulev S.N., Rukavishnikov G.D., Moroz D.I. et al. Monitoring of the stress state by seismic numerical methods at the mines of JSC “Vorkutaugol”. 2022. N 12, p. 88-93 (in Russian). DOI: 10.18796/0041-5790-2022-12-88-93
- Kamnev Ya.K., Filimonov M.Yu., Shein A.N., Vaganova N.A. Automated Monitoring The Temperature Under Buildings With Pile Foundations In Salekhard (Preliminary Results). Environment. Sustainability. 2021. Vol. 14. N 4, p. 75-82. DOI: 10.24057/2071-9388-2021-021
- Shammazov I.A., Sidorkin D.I., Batyrov A.M. Ensuring the stability of aboveground trunk pipelines in areas of continuous permafrost distribution. Bulletin of the Tomsk Polytechnic University. Geo Assets Engineering. Vol. 333. N 12, p. 200-207. DOI: 10.18799/24131830/2022/12/3832