Current state of above-ground and underground structures of the Alexander Column: an integral basis for its stability
Abstract
The Alexander Column as a compositional center of the architectural ensemble of Palace Square in Saint Petersburg, Russia, has always been a matter of concern for both the public and specialists due to progressive deterioration of its granite shaft caused by crack formation. The article examines previous studies related to the inspection and restoration of the column's shaft and other parts above ground level, as well as reasons for crack initiation and propagation in the column. An analysis was performed on the anomalies in the Fennoscandian Shield and the structural-tectonic conditions at the Montferrand quarry site, revealing the presence of faults and circular features within the studied area. The research considers N.Hast's measurements of excess tectonic stresses in anomaly zones (southeastern Finland), which acted horizontally and resulted in the development of tensile cracks within the granite massif and later in the column’s shaft after its installation. The most dangerous type of deformation for the Alexander Column is its tilt in the northeast direction, recorded in 1937 and 2000. The article analyzes the construction features of the column's foundations and additional underground elements, as well as soil and groundwater characteristics based on archival data. The contamination history of the underground space is taken into account, and an analogy-based method is used to assess the engineering-geological and hydrogeological conditions of the underground load-bearing structures within the placement zone of the Alexander Column and the New Hermitage buildings. The results of visual observations on the nature of deterioration and deformation of the pavement around the monument, as well as its pedestal, indicating the development of uneven settlement of the foundation, are presented. The article concludes with general recommendations for organizing and implementing comprehensive monitoring to forecast the deformation dynamics of the Alexander Column.
Introduction
Preserving the cultural heritage masterpieces of various countries, including architectural and historical monuments in cities, is considered one of the top priorities and essential tasks of our time [1, 2]. Addressing this global challenge involves engaging specialists from various fields: architects, restorers, sculptors, builders, biologists, microbiologists, geotechnical engineers, hydrogeologists, and experts in engineering geology [3, 4].
J.Kerisel (1908-2005), a prominent figure in the French geotechnical school, since 1975, played a crucial role in establishing and developing the geotechnical approach to studying the long-term stability and preservation of architectural-historical monuments. In memory of J.Kerisel, re-gular “Kerisel’s lectures” are organized at almost all international conferences (e.g., International Conference on Soil Mechanics and Geotechnical Engineering (ISSMGE), 2013, 2017, 2021) [5, 6]. Collaborating with A.Croce, J.Kerisel proposed and chaired the technical committee for preserving historical landmarks within the International Society for Soil Mechanics, Geotechnical Engineering, and Foundation Engineering (ISSMGE) [6]. Inspired by J.Kerisel’s initiative, the first international symposium on engineering geotechnics was organized in Naples in 1996 in honor of A.Croce. Italian specialists such as R.Jappelli, G.Viggiani, and others have actively contributed to the preservation of architectural treasures. Currently, G.Viggiani holds the Chair of the Italian Geotechnical Association, which promotes integrating modern geotechnical achievements into the theory and practice of reconstructing and restoring historical monuments. In Greece, Ch.Tsatsanifos and N.Psarropoulos have been involved in similar endeavors, including programs for saving the Leaning Tower of Pisa and a cathedral in Mexico City based on detailed studies of foundation characteristics and changes in soil-bearing capacity over time.
In Leningrad (now St. Petersburg), efforts to preserve architectural-historical monuments were led by Professor B.D.Vasiliev, Corresponding Member of the USSR Academy of Sciences V.A.Florin, and other scientists. Today, this noble tradition continues with Professor V.M.Ulitsky, General Director of the design institute “Georeconstruction” A.G.Shashkin, and others [7, 8]. Notably, researchers from the Department of Hydrogeology and Engineering Geology at St. Petersburg Mining University have contributed to preserving the architectural heritage of 18th-19th-century St. Petersburg. In 2013, at J.Viggiani’s invitation, a report was prepared for a symposium in Naples on ensuring the long-term stability of certain cathedrals in Naples [9].
One of the most significant architectural-historical monuments, listed as part of the world heritage, is the Alexander Column located on Palace Square. This monument stands in the historical center of St. Petersburg, characterized by complex engineering-geological and hydrogeological conditions. These conditions arise from the presence of a deep buried valley, the development of weak water-saturated and water-gas-bearing soils, and natural and natural-technogenic processes such as quick-sand formation, biochemical gas generation, and high corrosion activity of underground components to construction materials. Such conditions require non-trivial approaches to assessing the long-term stability of the Alexander Column.

Fig.1. The general appearance of monumental columns from various epochs 1 – Alexander; 2 – Vendôme; 3 – Trajan's; 4 – Pompey's; 5 – Antoninus [10]
The monument was designed by Auguste de Montferrand to commemorate the victory of the Russian army led by Emperor Alexander I in the War with Napoleon’s France of 1812. According to one of the main researchers of Montferrand's work, N.P.Nikitin, the Alexander Column is considered his finest creation [10]. When designing and implementing the project (1829-1834), Montferrand drew inspiration from four world masterpieces: Trajan's Column (113 AD) and Antoninus Column (161 AD) in Rome, Pompey's Column (298-303 AD) in Alexandria, and Vendôme Column (1806-1810) in Paris (Fig.1). The architect introduced new proportions between the diameters of the granite shaft along its height, creating an impression of lightness and elegance for the entire Alexander Column [10].
Despite compositional similarities with its prototypes, the Alexander Column is grander and taller – the total height of the column, including its pedestal and statue, reaches 47.5 m.
Structural features of the above-ground part of the Alexander Column
Visual analysis of this architectural-historical monument is presented in Fig.2. A detailed description of each fragment is provided in Table 1.
During the construction of the Alexander Column, Montferrand demonstrated not only his talent as an architect and builder but also his exceptional organizational skills. Successful installation of the monolithic shaft onto the lower pedestal occurred in 1832. Drawing on engineering solutions developed by A.Betancourt for assembling columns at St. Isaac's Cathedral, Montferrand improved the system of capstans and blocks. He first erected scaffolding on an above-ground brick structure since the mass of the granite shaft was comparable to that of six columns at St. Isaac's Cathedral. Thanks to these innovations, lifting and installing the column's shaft took only 100 min. The event was so extraordinary and grand for St. Petersburg at that time that not only ordinary residents filled the square and roof of the General Staff Building but also members of the imperial family. Upon completing the installation of the granite shaft, Emperor Nicholas I, addressing Montferrand, remarked: “Montferrand, you have immortalized yourself”.
Underground space components at the base of the Alexander Column
Until now, research on operational reliability of the Alexander Column has been conducted only for its above-ground part. Evaluating the stability of this architectural monument requires thorough consideration of its underground load-bearing structures, which include the foundations designed and implemented by A.Montferrand. Additionally, it is crucial to take into account the unique engineering-geological and hydrogeological conditions at the monument's base.
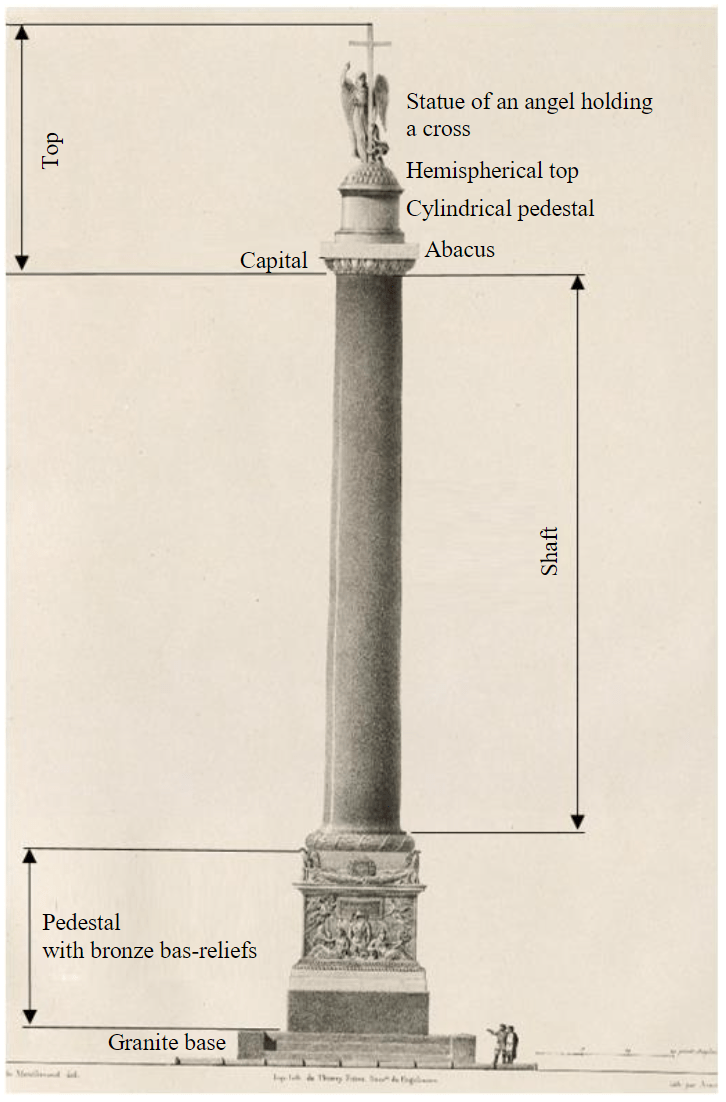
Fig.2. Fragments of the above-ground part of the Alexander Column (from albums by A.Montferrand with additions by the authors) [10]
Table 1
Characteristics of the fragments of the above-ground part of the Alexander Column
Fragment name |
Material |
Height, m |
Mass, t |
Top part: |
|||
Cross in the angel’s hands |
Bronze |
6.40 |
37 |
Statue of the angle |
Bronze |
4.26 |
|
Semispherical top |
Multilayer masonry (granite, brick, and 2 layers of granite), |
~1.34 |
|
Cylindrical pedestal (upper part) |
Brick masonry with bronze facing |
||
Abacus |
Brick masonry with bronze facing |
||
Capital |
Bronze |
||
Column’s shaft |
Monolith of granite rapakivi |
25.50 |
612 |
Pedestal (lower part) |
8 blocks of granite rapakivi on a lime mortar |
7.62 |
704 |
Base (plinth) |
Monolith of granite rapakivi |
2.38 |
|
TOTAL |
47.50 |
1353 |
When justifying place for the construction of the Alexander Column, the architect conducted a site survey using probing equipment (crowbar). As a result of this survey, a sandy “landmass”, according to the terminology of A.Montferrand, was discovered. The authors propose the hypothesis that Montferrand might have been aware of the original location of the monument to Peter I (designed by B.C.Rastrelli), which was intended to be installed facing the Winter Palace. This assumption was confirmed during the foundation construction work of the Alexander Column: workers discovered 99 piles and a stone foundation, which was later dismantled. A pit with a depth of 2 Russian fathoms (4.26 m) and dimensions of nearly 30×30 m was excavated for the monument [11]. At the bottom of the pit 1250 pine piles were driven in, each measuring 3 Russian fathoms (6.4 m) in length and ranging from 26 to 31 cm in diameter. The distance between the centers of adjacent piles reached 70 cm. Once the piles were driven in, the pit was filled with water, and the tops of the piles were cut to the level established by the water. Following this, a grillage was laid, consisting of individual large granite blocks, each 0.5 m thick and 1.5 m long, arranged in 11 rows with a slight offset in the shape of a “rigid core”, resembling a truncated pyramid with a top base area of 12×12 m (Fig.3). To reinforce the granite grillage, smaller granite blocks were laid on all sides using limestone rubble from the Putylov’s Quarry on a lime mortar. After the foundation for the Alexander Column was constructed, temporary piles were driven into the ground surface around the excavation to facilitate the installation of scaffolding and brickwork. These elements were necessary for the subsequent lifting and assembly of the granite shaft (Fig.3) [11]. These piles were not removed, as they provided additional stability to the underground part of the monument by reinforcing the soil around the foundation framework.
The territory of the Alexander Column is located on the slope of a deep buried valley of the pre-Neva, where a significant thickness of Quaternary deposits, reaching 70 m or more, is observed. In terms of structural-tectonic features, according to the latest tectonic map of St. Petersburg compiled by V.A.Yaduta, the territory of Palace Square is located within the zone of development of modern block-forming faults [12].
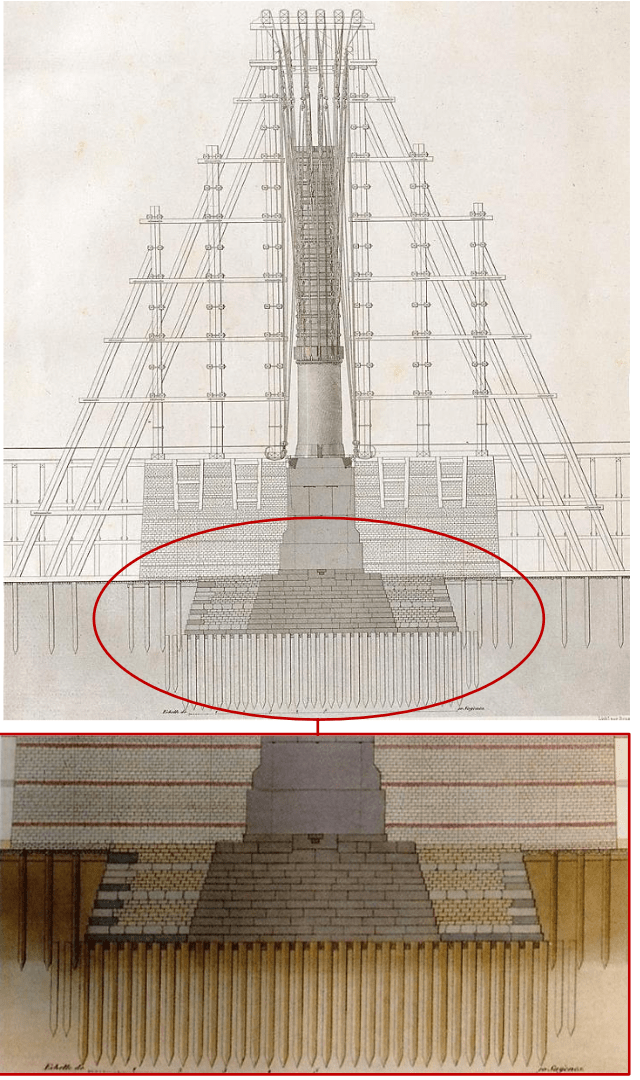
Fig.3. The view of the Alexander Column with underground structures (from albums of A.Montferrand)
The geological-lithological section of the monument's foundation is first shown based on the data from the borehole N 20, drilled in 1929 by the Trust “Lenkanalizatsiya” near the northwest part of the Alexander Column fence (Fig.4)*.
Borehole N 20 was drilled to a depth of 25.18 meters, revealing a characteristic section for the historical center of St. Petersburg composed of Quaternary-age sediments (from bottom to top): glacial, lacustrine-glacial, lacustrine-marine, and technogenic (Fig.5).
From an engineering-geological perspective, the exposed soils are characterized as weak, water- and water-gas-saturated deposits. Sand deposits exhibit fluid properties (quick-sand behavior), while clay formations are in a quasi-plastic state and tend to thixotropy (similar to the soils previously studied in the foundation of the General Staff building and the Winter Palace complex) [13, 14].
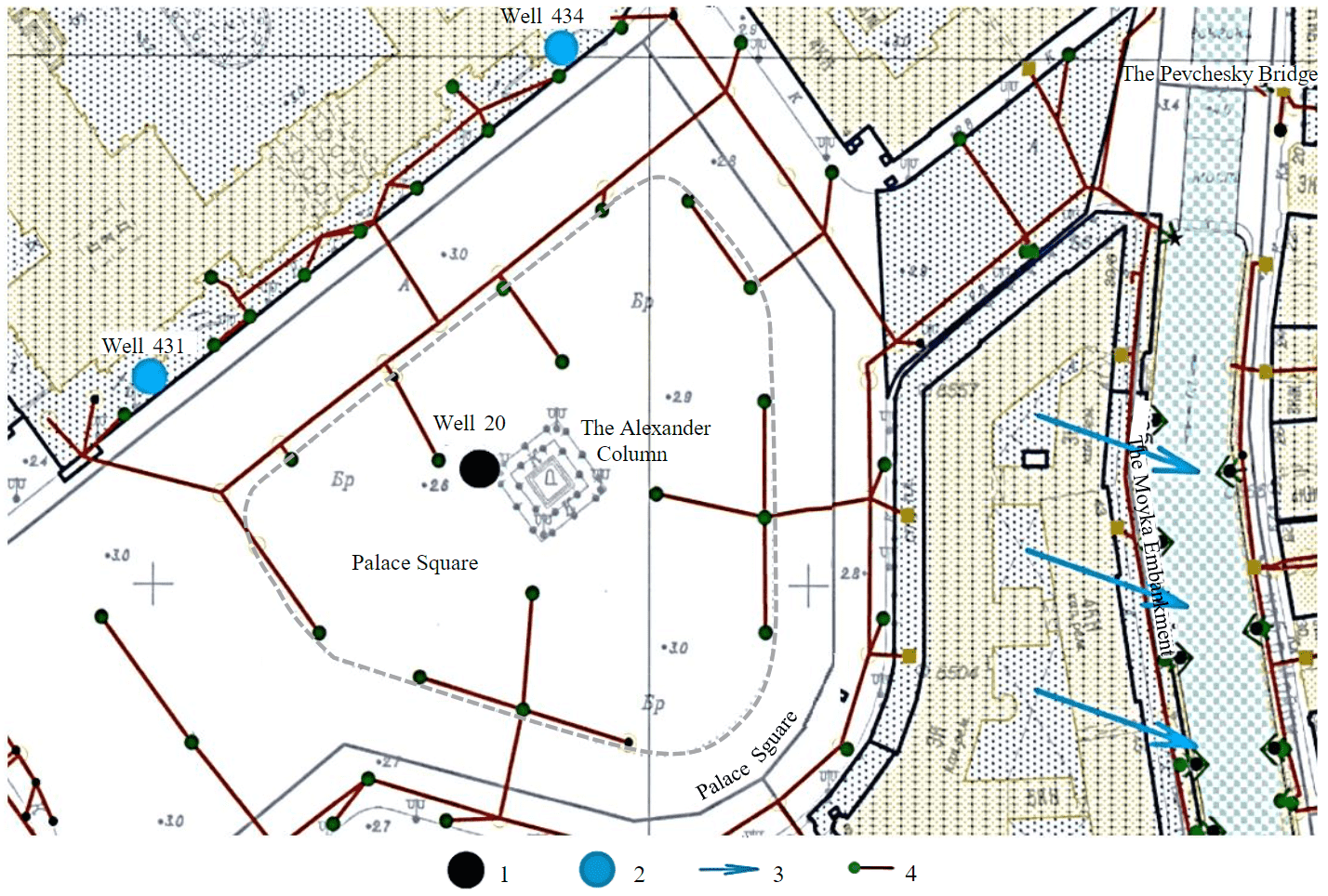
Fig. 4. Layout plan of local sewerage system and geological-hydrogeological wells 1 – reference geological well; 2 – hydrogeological well; 3 – groundwater discharge direction; 4 – position of shallowly laid local sewerage system
From Fig.5, it can be seen that the bearing horizon of the pile foundation of the Alexander’s Column is water-saturated fine-grained gray sands of lacustrine-marine genesis. Below, weak clayey layers of lacustrine-glacial origin are traced. Studies of the physical and mechanical properties of soils in 1929 were not carried out, since there were no laboratories for engineering-geological study of soils in Russia.
Numerous studies of the historical center of St. Petersburg, conducted by the Department of Hydrogeology and Engineering Geology at St. Petersburg Mining University, provide evidence of the formation of a natural-technogenic groundwater regime in the conditions where active drainage zones are absent within its territory due to the presence of impermeable structures along the embankments of rivers and canals. Additionally, localized areas of inundation are formed due to losses from the drainage systems, particularly in the vicinity of the Alexander Column, where aged local sewage networks are present (see Fig.4). Such specifics of the natural-technogenic environment led to the establishment of a stagnant hydrodynamic groundwater regime and the activation of their contamination by organic and inorganic compounds of various origins [15, 16].
When assessing the hydrogeological conditions and analyzing the formation of the contemporary engineering-geological and geotechnical specifics of the underground space within the studied area, it is essential to consider the historical aspect of its contamination from the 17th to the 21st centuries. During the pre-Petrine period (prior to 1703), according to cartographic materials from the late 17th to early 18th centuries, the future Palace Square area was covered by marshes. Their influence in terms of depth penetration reached up to 30 m or more due to the enrichment of the underlying water-saturated soils with abiotic and biotic organic matter, including marsh microorganisms of anaerobic and facultative forms, as well as the byproducts of their metabolism (enzymes, acids, biochemical gases) [15]. An additional source of contamination to the underground environment in the surveyed area was the agricultural activities of existing settlements, such as Gavgueva Village, which contributed to an increase in the concentration of both organic and inorganic pollutants in the subsurface environment [16].
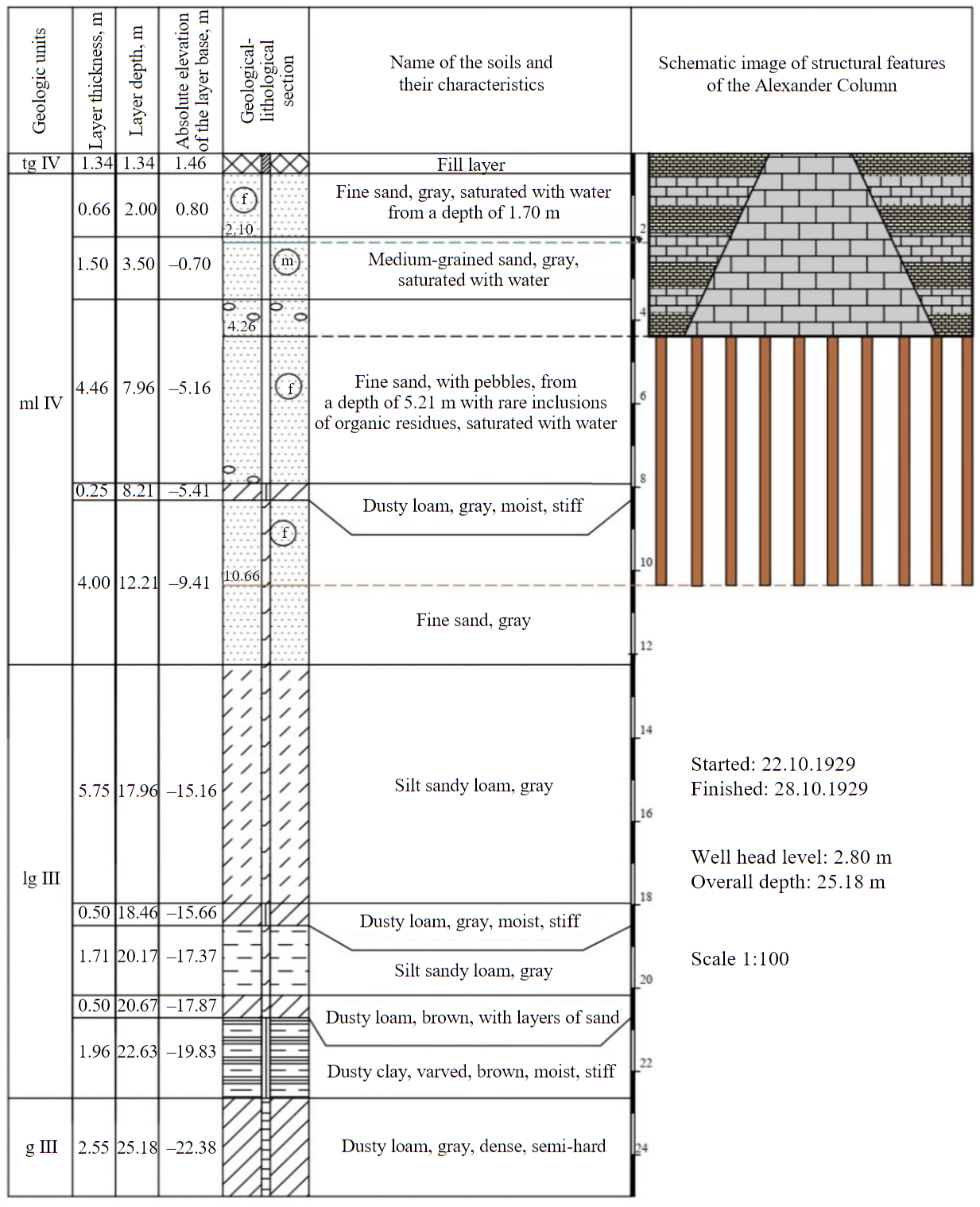
Fig.5. Geological-lithological section of the base of the Alexander Column (archive data of the Committee for Urban Development and Architecture of St. Petersburg)
Our research experience within the historical center of St. Petersburg demonstrates that sewage system leaks during the city's operational period lead to an increase in the number and diversity of microorganism taxa (with 107-108 CFU per 1 ml of wastewater). Additionally, there is an augmentation in the concentration of proteins, lipids, and carbohydrates, which serve as a nutritive and energetic environment for indigenous microbiota. The inorganic fraction of the wastewater is enriched with nitrogen, sulfur, and phosphorus compounds [15].
Groundwater sampling within the island part of St. Petersburg, conducted at the Department of Hydrogeology and Engineering Geology of St. Petersburg Mining University, revealed that a high content of organic compounds leads to a reduction in the redox potential (Eh) of groundwater to negative values. This observation is supported by measurements of this parameter in field conditions from monitoring wells [15].
The chemical composition of groundwater at Palace Square reflects the intensity, nature, and duration of subsurface contamination according to the results of well sampling conducted in close proximity to the Alexander Column (see Fig.4, Table 2).
Table 2
Chemical composition of groundwater within the Palace Square (archive data of the Trust of Geodetic Works and Engineering Surveys, 2002)
Components |
Quantity, mg/dm3 |
|
Well 431 |
Well 434 |
|
Ca2+ |
160.3 |
168.3 |
Mg2+ |
26.8 |
55.9 |
(K+Na)+ |
2194.5 |
2750.8 |
Sum of cations |
2381.5 |
2975.1 |
SO42 |
297.8 |
345.8 |
Cl |
3309.7 |
4174.7 |
HCO3 |
311.2 |
396.6 |
CO32 |
30.0 |
36.0 |
NO3 |
1.50 |
1.00 |
Sum of anions |
3950.2 |
4954.2 |
Mineral residue |
6176.1 |
7730.9 |
Total hardness, degree |
28.6 |
36.4 |
Carbonate |
14.3 |
18.2 |
Non-carbonate |
14.3 |
18.2 |
Fe2++Fe3+ |
Traces |
Traces |
рН |
8.67 |
8.60 |
Permanganate oxidizability, mgO2/dm3 |
50 |
60 |
Humus |
46.0 |
53.3 |
Note. Organoleptic properties: opaque, light-yellow color, odorless.
According to the chemical composition, groundwater within the Palace Square is chloride sodium. High values of mineralization are noted due to the content of potassium and sodium ions, chlorides and sulfates, which indicates contamination of groundwater by leaks from operating sewer systems (see Fig.4). A large amount of calcium and magnesium ions is associated with the dissolution and leaching of alkaline earth elements from underground bearing and enclosing structures of buildings of the Palace complex.
Many years of experience in scientific research of the Department of Hydrogeology and Engineering Geology at St. Petersburg Mining University shows that the groundwater composition and their contamination determine soils state and properties, as well as the formation of dangerous processes, mainly due to activity of underground microorganisms that contribute to the formation of biofilms on the surface of mineral soil particles, including sands and clay deposits. Such films consist of living and dead cells of microbiota and products of their metabolism, which leads to a decrease in the angle of internal friction in water-saturated sands, transferring them to a quick-sand state [15]. In addition, due to the activity of microorganisms, biochemical gas formation of hydrogen sulfide (H2S), methane (CH4), molecular nitrogen (N2), hydrogen (H2), ammonia (NH3) and carbon dioxide (CO2) – the product of respiration of microorganisms – is observed, occurring mainly in anaerobic conditions.
To predict the development and activation of natural and natural-technogenic processes, one should consider the solubility of gases in water and their aggressiveness towards construction materials. The deposition of poorly soluble gases, such as CH4, N2 and H2, in the underground environment leads to the loosening of soils and the formation of gas-dynamic pressure with a change in the stress-strain state of the entire soil mass [15]. Well-soluble gases include H2S, NH3, and CO2, among which hydrogen sulfide is of the greatest importance, encountered within the section of the historical center of St. Petersburg to a depth of 25 m, sometimes lower. It poses a danger for various construction materials – natural stone (primarily carbonate rocks), concrete, reinforced concrete and metals. In addition, CO2 is aggressive, forming carbonic acid aggressiveness. NH3 in the aquatic environment exists as an ammonium ion (NH4+), which contributes to the dispersion of clay soils, as well as has a weak aggressive effect on concrete.
The specificity of the underground space conditions leads to the development of not only corrosive, but also biocorrosive processes for underground structures and building materials. The analysis of the nature and progress of biocorrosion should be based on evaluating the effects of metabolites from microbiota (enzymes, organic acids, gases), as well as the activity of microorganisms that create biofilms on the surface of structures and extract missing elements from the crystal lattice of minerals in building materials by breaking it down [15].
Methodology
A unified methodology, methods and techniques for analyzing the long-term stability of architectural and historical monuments, their survey, as well as designing reconstruction and restoration projects for cultural heritage objects, especially in historical areas of megacities have not been developed yet [7, 14].
The basis for our methodology of assessing the long-term stability of architectural and historical monuments in St. Petersburg is a conceptual approach to studying underground space as a multi-component system: dispersed soils that contain underground water, microorganisms, biochemical and deep-earth gases, as well as underground load-bearing structures. We analyzed the condition and causes of deformation development in the complex structure of aboveground part of the Alexander Column, which has been undergoing preservation work since its first years of operation due to cra-cking in its shaft made of granite-rapakivi. To identify the reasons for its disintegration, we evaluated the structural-tectonic conditions in Montferrand’s quarries in southeastern Finland, where monoliths for both the Alexander Column and St. Isaac’s Cathedral columns were extracted. Such an evaluation has not been done before. For this purpose, we examined the works by Swedish rock mechanic N.Hast who measured excess tectonic stresses in the upper part of this area’s section on the Fennoscandian Shield. These stresses caused microcracking development in granites horizontally along the length of each extracted monolith. We compared tensile strength values of granite-rapakivi samples with existing tectonic stresses according to N.Hast’s data. It is clear that placing the column vertically led to gradual opening up tension cracks that were visually observed as vertical or subvertical ones.
We also performed an analysis for the first time on condition of underground structures of the Alexander Column – its foundation combined with retaining elements under their interaction with a multi-component underground environment: water-saturated sandy-clay soils, heavily contaminated underground waters, microorganisms and biochemical gases. We applied an analogy method to assess the condition of the Alexander Column foundations by comparing it with how underground load-bearing structures were destroyed at the New Hermitage located nearby in similar engineering-geological, hydrogeological and geoecological conditions. R.E.Dashko supervised studies on condition of underground and aboveground structures of the New Hermitage at the end of the 20th and the beginning of the 21st centuries. The methodology for analyzing causes of load-bearing structures destruction under their interaction with multi-component underground space proved to be effective for predicting long-term stability of various structures, including architectural-historical monuments, their reconstruction and restoration, as well as for creating a comprehensive monitoring system [17].
Discussion of results
Analysis of aboveground part condition of the Alexander Column
The Alexander Column was consecrated and officially opened in 1834, but lime runs were already noticed on the surface of its granite shaft in 1836. After inspecting the shaft of the column, A.Montferrand concluded that rainwater seeped through holes in the cylindrical (upper) pedestal and washed the lime solution from brickwork out [18].
In 1838, the first cracks in the granite monolith of the column were recorded. The Commission for the Construction of St. Isaac’s Cathedral concluded that these cracks resulted from an “optical illusion” caused by granite-rapakivi’s structure – dark mica clusters forming “stripes”. By 1841, there was a need for a repeated inspection of the column, based on which the commission recognized the existence of real cracks, and studied the condition of the granite insert (dimensions 0.31×0.23×0.13 m), installed at the end of one of the cracks during the preparation of the monolith for the column shaft [19]. Table 3 describes restoration work done from 1836 to 1841. Despite clear visual signs of cracks forming and developing on the surface of the shaft, St. Petersburg Gazette N 240 published an article in 1841 with a semi-official report by the commission that “proved” that “the dark stripes seen on the column … are an optical illusion” [19].
Unfortunately, no reliable information exists about research done in the following 20 years, but restoration work is well documented for eliminating defects in the lower pedestal from 1840 to 1850 (Table 3).
In 1861, Alexander II established a Committee for Investigating the Damage to the Alexander Column, which included: from Institute of the Corps of Transport Engineers (now – Emperor Alexander I St. Petersburg State Transport University) – V.D.Evreinov, S.V.Kerbedis and V.P.Sobolevsky; from Institute of the Corps of Mining Engineers (now – St. Petersburg Mining University) – G.P.Helmersen; from the Academy of Sciences – E.I.Eikhvald, A.Ya.Kupffer; from the Imperial
St. Petersburg University (now – St. Petersburg State University) – A.A.Voskresensky; architects of the highest court – K.A.Thon, A.P.Brullov, A.I.Stackenschneider, Y.A.Bosse, as well as A.Montferrand’s former assistant – A.A.Poiraux, who personally participated in the inspection of the column in 1841. The new commission’s members concluded after inspecting the column that the destruction of its shaft occurred due to cracks forming on its surface, once again contradicting the 1841 research results [20].
The Mining Institute’s director (1856-1872) – G.P.Helmersen [19] presented a detailed report on the inspection of the column in 1861. He personally conducted research on the shaft’s fracturing and assessed its development features: thin hairline cracks ran through the entire shaft’s surface from the capital to the pedestal’s edge; three crack systems were identified: south-southeastern, north-northeastern and eastern; besides, on the south-southeastern side where the granite insert was located, cracks of different lengths and openings were observed; the west-southwestern, western, north-western and northern sides of the column had fewer cracks and damages than the south-eas-tern and north-northeastern sides. The most serious defects were on the southern side where the granite insert was located [19].
Another committee member – Major General V.D.Evreinov noted when inspecting the shaft that “the set of cracks on both the south-western and north-eastern sides of the column formed a kind of strip crossing the column from top to bottom”, which could eventually lead to the column’s collapse due to the displacement of its upper part [20].
The committee members agreed that the shaft’s cracks existed before installing it on the lower pedestal and were not detected in the first years of monument’s operation because they were skillfully “plastered”. G.P.Helmersen also suggested that hairline cracks might have existed in granite-rapakivi’s massif before monolith processing [19]. We will elaborate on this statement further.
Montferrand’s quarries are located in the southeastern part of Finland. The considered area is associated with anomalous sections of the Fennoscandian Shield (submersion zones) and is characterized by complex structural-tectonic conditions [21], as indicated by the data of the cosmotectonic map (Fig.6) [22].
The presence of ring structures in combination with faults of different directions within the quarry’s location determines the level of tectonic stresses [23], which is confirmed by studies carried out on the Kola Peninsula [24].
As is known, tectonic stresses are most actively manifested in crystalline rocks of the foundations of ancient platforms and folded belts. In the middle of the 20th century, N.Hast conducted field measurements of horizontal tectonic stresses on the Fennoscandian Shield, which allowed him to establish the nature of their change with depth z according to the dependence [25]
Table 3
Restoration work on the Alexander Column in 19-21 centuries [18]
Name of elements |
Change in element condition |
Time and result of inspection |
Figure of angel with cross |
Integrity damage of figure |
1963 Figure of angel restored |
Hemispherical top |
Leaching out lime solution from brickwork by rainwater |
1836 Fixed holes sealed; lime runs on column removed |
Cylindrical pedestal (upper) |
Absence of several bolts in the |
1950 Bolts replaced, damage from shell splinters sealed, seams on abacus soldered |
Column’s shaft |
Inspecting column for cracks and assessing hazard of their further development |
1838 Causes of cracks formation on surface of granite monolith analyzed |
Presence of cracks already existing during column erection established |
1861-1862 Causes of cracks appearance identified; map of cracks drawn at a scale 1:14; existing cracks cleared from weathered granite then filled with Portland cement |
|
New plan of restoration work mapped out based on inspection |
1911-1912 Old cracks cleared, filled with Portland cement, |
|
Increase in number, length and opening of cracks on the column shaft noted. Column tilt |
1935-1937 Geodetic research carried out; displacement from the column axis in north-east direction reaches 31 mm |
|
Defects in seams, runs on shaft |
1950 Seam between shaft and column base filled, traces of runs from under abacus removed |
|
Appearance of new cracks |
1954 Quality of Portland cement in seams around the inserts – “dropouts” – checked |
|
– |
1963 Scheme of cracks location on the shaft drawn; old granite inserts strengthened |
|
Column tilt |
2000 Geodetic measurements of column vertical position carried out; deviation from the axis by 65 mm towards the General Staff building and the Moyka River embankment – Pevchesky Bridge |
|
– |
2001-2003 Some granite inserts in the column replaced; cracks filled with mastic; column shaft polished |
|
Pedestal (lower) |
– |
1840-1850 Damaged parts corrected, spalling restored, diverged seams filled with lead, polishing done 1963 Pedestal reliefs and granite elements with lost parts restored 2001-2003 Granite base and pedestal restored; cracks between blocks of steps and pedestal base eliminated |
Base (plinth) |
– |
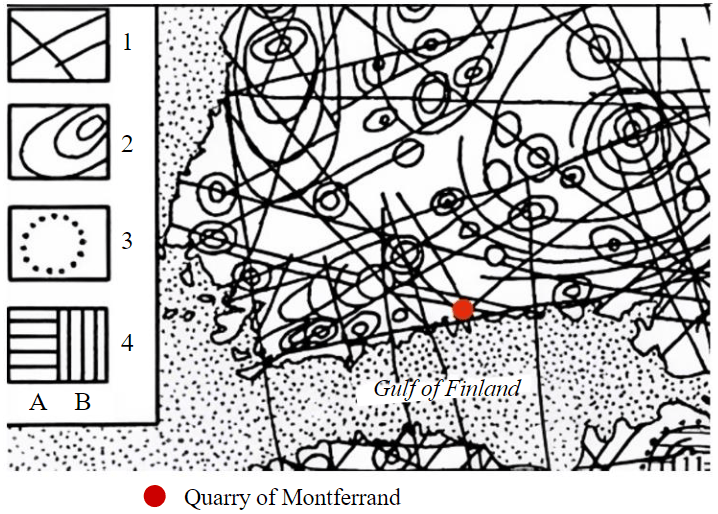
Fig.6. Fragment of the cosmotectonic map of the north-west of the Russian Plain [22] 1 – transgressive, regional and local faults; 2 – ring structures; 3 – island elevations (remnants of the Neogene lacustrine-marine terrace) 4 – island elevations: basement (A) and overlain by N-Q deposits
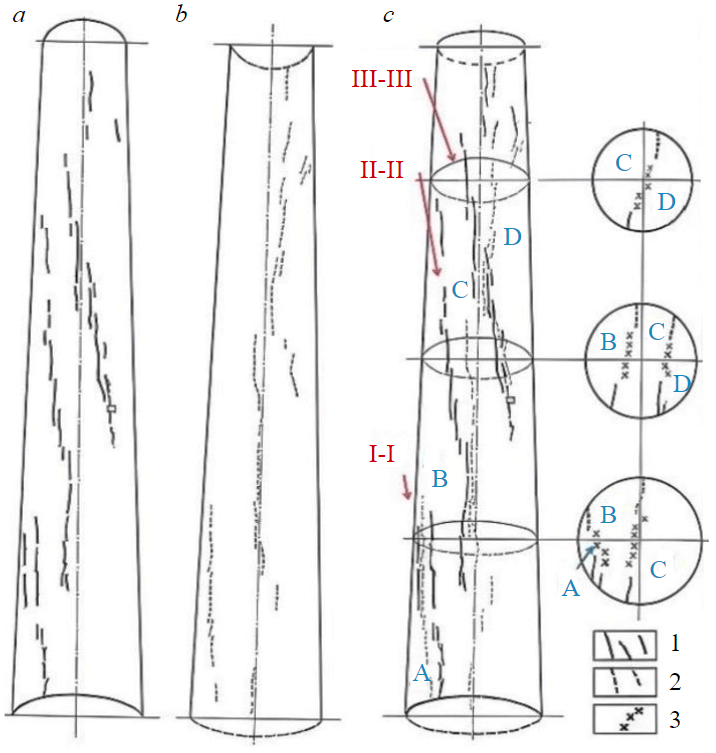
Fig.7. Scheme of the fracture network of the granite core of the Alexander Column (data from M.A.Ivanov): а – fractures of the south side of the column (1); b – fractures of the north side of the column (2); c – combined projections of a and b. Cross-sections illustrate the location of the assumed fractures inside the column (3). I-I, II-II, III-III – selected zones of fracturing; A, B, C, D – blocks that divide the column by fractures [26]
Since a monolith for the column was extracted at a depth of 7 m, it experienced almost 20 MPa of horizontal stress that caused subhorizontal tensile microcracks in its rock mass. These microcracks were hard to detect visually. The choice of this place for sampling granite-rapakivi by A.Montferrand was explained by the location of the required monolith between horizontal macrocracks, which predetermined the initial diameter of the column [11]. Such cracks divide the massif into layers, forming the so-called structure of a “layered pie”, according to the termino-logy of M.A.Ivanov [26].
Granite-rapakivi samples were tested for compressive strength in mid-19th century at the mechanical laboratory of Institute of the Corps of Transport Engineers. The maximum value of compressive strength did not exceed 58 MPa [27]. As is known, the tensile strength of rock masses is on average 1/10 of compression, i.e., for the studied granite-rapakivi it does not exceed 6 MPa.
After the column’s monolith was transported from the quarry to Palace Square where it was processed, polished and mounted on a pedestal, the microcracks in its granite shaft took a subvertical direction. Under its own weight, these microcracks transformed into macrodefects. This process was accelerated by additional pressure due to the fragments of the column installed above (see Table 1). Temperature stresses in the polymineral rock, as well as wind loads contribute to the growth of existing and appearance of new cracks in the shaft. The effect of these natural factors on the degradation of granite-rapakivi was described by G.P.Helmersen [19].
In 2021, Professor M.A.Ivanov from St. Petersburg Mining University studied the granite shaft using a binocular and digital imaging. He created a scheme of fracturing for northern and southern parts of the Aleksander column and their combined projection (Fig.7) [26].
M.A.Ivanov’s research showed that only vertical and subvertical tensile cracks that existed in situ in granite-rapakivi massif before extraction continued to develop (Fig.7).
It is necessary to mention the research of St. Petersburg microbiologists who are engaged in the problem of biogenic weathering of granite-rapakivi architectural monuments in urban conditions [28, 29]. Scientists have found that microbial colonization is confined to moist cracks in rocks, while biomass growth contributes to their opening [30]. The most active biodestructors of granite-rapakivi are actinomycetes, micromycetes, silicate bacteria, photoautotrophic and diatom algae [31]. However, clarification is required for presence of these taxa of microorganisms in cracks in the granite shaft and their activity aimed at accelerating destruction process. Microbiological studies were only done for monuments the granite of which was in contact with ground or close to it. Microflora reached these monuments through contaminated paving, precipitation, dust and capillary rise of groundwater that was shallow (less than 2 m) in the historical center.
Crack formation is not the only problem that specialists face when studying the condition of the aboveground parts of the Alexander Column. The problem of leaching of lime solution from the brickwork was solved only at the beginning of the 21st century. In 188 years since the opening of the Alexander Column, numerous surveys of its elements have been conducted by specialists and, at least, nine cycles of complex restoration works have been performed (Table 3).
As can be seen from Table 3, the last comprehensive studies and restoration works of all aboveground structures of the monument were carried out in 2001-2003, including acoustic study of the column’s shaft along its height to determine its condition and resource of the monolithic part [18].
During the visual inspection in 2022, the authors noted the following defects in the granite pedestal and its base: spalling, cracks, divergence of the granite blocks forming steps in the base, salt efflorescence on the surface, which was recorded as early as the 19th century (Table 3).
Despite the importance of the crack formation problem in the monolithic shaft of the column, the most alarming, in our opinion, is the tilt of the monument, first recorded in 1937 and amounting to 31 mm. Repeated geodetic measurements were made more than 60 years later, according to which the deviation of the column from its vertical axis reached 65 mm (Table 3). According to the measurements, the tilt is directed to the northeast towards Pevchesky Bridge [18]. Based on the results of observations, the tilt rate was calculated, which was 0.5 mm/year. According to experts dealing with safety of operation of buildings, including architectural and historical monuments, their reconstruction and restoration, such a value of tilt rate is considered dangerous. In an interview dedicated to the condition of the Alexander Column, Honored Architect of Russia R.M.Dayanov noted that in 2003 a calculation was made of the maximum permissible tilt of the column, which was 60 mm. After 2000, geodetic observations of the column were not carried out.
To assess the permissible value of tilt, we will conduct the following analysis. The Alexander Column belongs to absolutely rigid structures, whose construction is analogous to smokestacks, cooling towers and other similar objects. According to the instruction on erection of such structures (VSN 430-82), deviation of the axis of a pipe from vertical line is allowed for pipes with height H ≤ 100 m not more than 0.002 times its height provided that this deviation does not exceed 150 mm. The height of the Alexander Column is 47.5 m, accordingly for it the limit value of deviation is 95 mm. The calculated value requires adjustment – it is necessary to take into account the uniqueness of this architectural monument and a significant period of its operation. Taking into account the measured value of deviation of the column from its vertical axis, equal to 65 mm, we can calculate the existing relative deformation, which is 0.0014 (the ratio of tilt value to height of entire monument). For ensuring stability of the monument under consideration, the excess of this value needs to be clarified when conducting comprehensive monitoring, including geodetic observations, as well as performing special calculations based on provisions of structural mechanics [32-34].
The current state of the underground part of the Alexander Column
As noted earlier, due to the lack of complete information on the engineering and geological conditions of the underground space of the Alexander Column, the method of analogies can be used to assess the current state of its underground structures. This method compares the conditions in the foundation zone of the New Hermitage, which is in close proximity to the Alexander Column (200 m to the northwest), has identical engineering and geological and hydrogeological conditions, as well as a similar history of contamination of the underground space. R.E.Dashko studied how the foundations of this building were destroyed at the end of the 20th century. Within the New Hermitage placement zone, there is a presence of contaminated groundwater related to long-term contamination by sewage; presence in the foundation section of quick clays, clayey soils characterized by extreme instability, as well as a large amount of hydrotroilite, which indicates a reducing environment in the underground space and formation of H2S. In the course of studies conducted in the courtyards of the New Hermitage, low-soluble gases were detected, the exhalation was observed in the form of bubbles of different sizes [15]. Such a state of soils and gas generation are determined by high microbial infection of underground space components. It is not considered when surveying architectural and historical monuments and evaluating corrosion of building materials.
It is especially necessary to dwell on the state of the New Hermitage underground structures, since its foundation was arranged as a distribution plate made of limestone from the Putilov’s Quarry and pine wooden sleepers. The same materials were used when arranging the underground part of the Alexander Column. During inspection, patchy destruction of limestone to a state of dolomite powder was found. Sleepers were affected by bacteria and micromycetes. On some wooden piles serving as foundation for bridge crossings near Palace Square, main damage was caused by lignin-degrading bacteria characteristic for swamp microflora [15]. Most often biocorrosion of wood is expressed in form of change in its color and structure, easy separation into individual fibers and release of water when pressed. When dried, such wood takes on a “pseudo-stone-like” appearance due to proteins (enzymes) that glue fibers [35, 36]. With an average density of pine 0.52 g/cm3, density of wood affected by lignin-degrading bacteria did not exceed 0.28 g/cm3, in some cases – lower, which indicates loss of pile function as a bearing structure. It is quite understandable that leaching out carbonate rocks and lime solution from masonry foundations, as well as destruction of wooden sleepers creates conditions for development of additional and uneven deformations of underground bearing and enclosing structures, which together with settlement of foundation soils lead to formation of cracks in bearing walls of New Hermitage continuing to this day. Obviously, there is a need to study underground bearing structures of the Alexander Column in order to assess their condition and the influence of foundation destruction on the development of tilt [8, 37].
The possibility of developing a tilt will be determined by the total pressure under the base of the “rigid core”, taking into account that the pine piles are destroyed by active biocorrosion of wood within the considered area. In addition, it is necessary to note the eccentricity of the acting load due to the displacement of the angel figure relative to the center of the hemispherical top in the northeast direction (see Fig.2), within which the tilt of the Alexander Column is observed. According to the provisions of soil mechanics, when a load with an eccentricity e < 0.25b (where b is the width of the foundation) acts, a concentration of contact stresses occurs in the edge zone under the base of the foundation in the direction of tilt. Stress values exceed more than twice the value of the total pressure from the aboveground and underground parts of the monument.
The total load from the aboveground part of the Alexander Column is 1353 t (see Table 1). The mass of the underground part, which is a truncated pyramid (“rigid core”), can be calculated by the formula
where V is the volume of a truncated prism, m3; ρ is the density of granite masonry from separate slabs, assumed to be equal to 2.2 t/m3;
H is the height, m (corresponds to the depth of the pit); S1 is the area of the pyramid’s upper base, S1 = 12×12 m2; S2 is the area of the pyramid’s lower base, S2 = 20×20 m2;
The pressure from the aboveground part of the Alexander Column and the “rigid core” will be equal to
where mtot is the total mass of the aboveground part of the Alexander Column (see Table 1) and “rigid core”;
Thus, the value of contact stresses under the base of the foundation in its edge part exceeds 0.19 MPa and for weak water-saturated soils will be considered as a force factor that contributes to the growth of tilt over time.
The development of uneven deformations of underground bearing structures, as well as their destruction due to physicochemical and biochemical processes, leads to a damage of the paving integrity on Palace Square in the zone of the Alexander Column influence. According to visual inspection, both deformations of lifting masonry and its subsidence are observed on the south side of the monument. At the same time, on the north side, deformations of the pedestal and the staircase facing are traced in form of divergence and displacement of the steps, which is associated with unevenness of deformation processes of different signs.
Recommendations for conducting comprehensive monitoring of the Alexander Column
To conduct a comprehensive monitoring of the Alexander Column, we need to split the concept into two parts, each addressing a common challenge: how to ensure the long-term stability of the monument and how to develop effective and preventive actions to avoid negative outcomes that could put the architectural heritage at risk.
The first block should include a comprehensive inspection and instrumental observations of the condition of the aboveground part of the monument. The second block involves the use of low-impact technologies to study the condition of the underground bearing structures, whose deformation will largely affect the safety and functioning of the aboveground part, especially the tilt.
The first block should include geophysical and modern geodetic methods of observation. It seems possible to apply acoustic technologies that are related to “illuminating” the state of all elements of the column along its height. For the aboveground part, the main attention should be paid to the shaft, especially the causes of crack formation in it. One of the priority research directions for assessing the stability of the Alexander Column is to determine the patterns of its tilt development, which can be done by using 3D observations with terrestrial laser scanning, along with measuring the deformation of the column and the surrounding area using surface and deep benchmarks [33, 38-40].
The second block of observations is complicated by the impossibility of conducting standard engineering-geological and geotechnical studies – drilling and static sounding, as well as using equipment with dynamic impact [7, 8, 41]. The main information can be obtained from the results of electrical tomography, which will reveal some negative aspects of the state of the “rigid core” and underground structures [42-44]. The existing standards for reconstructing and restoring architectural and historical monuments deem it safe for the object if the works are done at least 30 m away from it on weak soils [45, 46]. Thus, it is possible to examine the state of piles, which were driven from ground surface for brick masonry of temporary structures with purpose of installing lifting mechanisms on them for subsequent installation of the shaft (see Fig.3). Examining such a pile along its full length using low-impact methods will provide information about the state of wood – pine below level of contaminated sewage groundwater, as well as identify main taxa of microorganisms from host soils and water. This will enable us to determine the aggressiveness of microbiota towards the construction materials of the monument underground part, their role in transforming dispersed soils and the biochemical composition of groundwater, as well as the development processes of gas formation due to the activity of microorganisms. Developing the concept and content of the monitoring should be considered as a separate topic of research.
Conclusions
- Ensuring the safety of operation and long-term stability of the Alexander Column should be regarded as one of the priority tasks for preserving the architectural and historical monuments of St. Petersburg.
- The greatest concern for the preservation of the aboveground part of the Alexander Column is its granite shaft. The authors believe that the cause of cracking, recorded in the first years after its installation, is related to the specificity and complexity of the structural-tectonic conditions of the quarries, located in the areas of the Fennoscandian Shield (southeastern part of Finland), characterized by high tectonic stresses in the rocks, that began to be studied by geologists, geophysicists and rock mechanics of Finland, Sweden, and the USSR only in the 60-70s of the last century. The concentration of tectonic stresses is observed on the earth’s surface and increases with depth according to the regularity obtained by N. Hast. Tectonic stresses lead to the development of tensile stresses in the massif of granite-rapakivi in situ. The analysis of the factors that induce the cracking of granites in the body of the Alexander Column is presented.
- For the first time, the structural features of the underground part of the Alexander Column are considered and analyzed; a geological-lithological section of the monument base to a depth of 25 m is given (archive data of the Committee for Urban Development and Architecture of St. Petersburg). A qualitative analysis of groundwater contamination is given and the history of their pollution over time is presented. The aggressiveness of groundwater towards construction materials is emphasized, which was confirmed by the research on the state of underground structures of the Winter Palace (New Hermitage) complex.
- Based on the analysis of long-term surveys, including data acquired in the 20th and 21st centuries, a conclusion is drawn about the danger of developing a tilt of the monument in the northeast direction. The deviation rate of the Alexander Column from the vertical axis is a serious concern, and the importance of studying its tilt is emphasized. By applying the analogy method with the foundations of the New Hermitage, the direction and causes of degradation processes of underground bearing structures of the Alexander Column are assessed. The tilt of monument is attributed to the eccentric position of the angel figure relative to the top axis, which is supported by calculations of contact stress concentration in the edge zone under the rigid base grillage.
- The authors propose recommendations for the content, organization and implementation of a comprehensive monitoring program for the Alexander Column: inspection and instrumental observation of the aboveground part of the monument, selection and application of low-impact technologies for studying underground bearing structures, as well as deformation monitoring, especially the tilt, which will largely affect stability of the Alexander Column.
References
- Valore C., Ziccarelli M. The preservation of Agrigento Cathedral. Proceedings of the 18th International Conference on Soil Mechanics and Geotechnical Engineering. Paris. September 2-6. 2013, p. 3141-3148.
- Yeon-Soo Jang. Geotechnical Issues and Preservation of Korean Heritage Sites. Proceedings of the 19th International Conference on Soil Mechanics and Geotechnical Engineering. Seoul. September 17-21. 2017, p. 231-244.
- Sesov V., Cvetanovska J., Edip K. Geotechnical aspects in sustainable protection of cultural and historical monuments. Proceedings of the 18th International Conference on Soil Mechanics and Geotechnical Engineering. Paris. September 2-6. 2013, p. 3129-3132.
- Margottini C. Engineering Geology in Shaping and Preserving the Historic Urban Landscapes and Cultural Heritage: Achievements in UNESCO World Heritage Sites. Engineering Geology for Society and Territory. 2015. Vol. 8, p. 1-28. DOI: 10.1007/978-3-319-09408-3_1
- Calabresi G. Kerisel Lecture: The role of Geotechnical Engineers in saving monuments and historic sites. Proceedings of the 18th International Conference on Soil Mechanics and Geotechnical Engineering. Paris. September 2-6. 2013, p. 71-83.
- Viggiani C. 2nd Kerisel lecture: Geotechnics and Heritage. Proceedings of the 19th International Conference on Soil Mechanics and Geotechnical Engineering. Seoul. September 17-21. 2017, p. 119-140.
- Ulitsky V.M., Shashkin A.G. Preservation of Architectural Monuments and Provision of their Mechanical Safety. Industrial and Civil Engineering. 2017. N 7. p. 31-39 (in Russian).
- Shashkin A.G., Shashkin V.A. Is it possible to preserve historical monuments on the basis of standards for new construction? Geotechnics. 2021. Vol. 13. N 2, p. 20-30 (in Russian). DOI: 10.25296/2221-5514-2021-13-2-20-30
- Dashko R.E., Shidlovskaya A.V. Long-term stability of old cathedrals in St. Petersburg. Geotechnical Engineering for the Preservation of Monuments and Historic Sites. London: CRC Press, 2013, p. 299-306. DOI: 10.1201/b14895-35
- Nikitin N.P. Auguste Montferrand: Design and construction of St. Isaac’s Cathedral and Alexander Column. Leningrad: Leningradskoe otdelenie Soyuza sovetskikh arkhitektorov, 1939, p. 348 (in Russian).
- Lyubin D.V. Alexander Column. St. Petersburg: Izd-vo Gosudarstvennogo Ermitazha, 2013, p. 104 (in Russian).
- Yaduta V.A. Modern tectonics of St. Petersburg and Leningrad region. Mineral. 2006. N 1 (5), p. 28-35 (in Russian).
- Shashkin A.G., Paramonov V.N., Ulitsky V.M. The geotechnical aspect of underground space development of St. Petersburg. Geotechnics. 2018. Vol. 10. N 3, p. 8-23.
- Mangushev R.A., Osokin A.I., Sotnikov S.N. Geotechnics of St. Petersburg. Moscow: Izd-vo Assotsiatsii stroitelnykh vuzov, 2018, p. 386 (in Russian).
- Dashko R.E., Vlasov D.Yu., Shidlovskaya A.V. Geotechnics and subsurface microbiota. St. Petersburg: Izd-vo instituta PI “Georekonstruktsiya”, 2014, p. 279 (in Russian).
- Lebedeva Y., Kotiukov P., Lange I. Study of the Geo-Ecological State of Groundwater of Metropolitan Areas under the Conditions of Intensive Contamination Thereof. Journal of Ecological Engineering. 2020. Vol. 21. Iss. 2, p. 157-165. DOI: 10.12911/22998993/116322
- Dashko R.E., Salnikov P.M. Main principles of an integral monitoring for St. Isaac’s Cathedral in Saint-Petersburg. Topical Issues of Rational Use of Natural Resources: Proceedings of the International Forum-Contest of Young Researchers, April 18-20, 2018, Russia. St. Petersburg: Saint Petersburg Mining University, 2019, p. 17-22.
- Lyubin D.V., Makeeva E.I. Alexander Column. History of restoration. Preventive care. Muzei pod otkrytym nebom. Strategiya sokhraneniya skulptury v gorodskoi srede. St. Petersburg: Znak, 2018, p. 66-72 (in Russian).
- Gelmersen G.P. Alexander Column in St. Petersburg. Gornyi zhurnal. 1862. N 5, p. 219-231 (in Russian).
- Aspidov A.P. Arabesques of Petersburg. Moscow: Tsentrpoligraf, 2007, p. 463 (in Russian).
- Girin R.E. Tectono-geodynamic analysis of the earth’s crust thickness in the west of the East European Platform. Minsk: Belaruskaya navuka, 2022, p. 110 (in Russian).
- Kuzin I.L. Myths and realities of the doctrine of continental glaciations. St. Petersburg: Izd-vo SZNII “Nasledie”, 2013, p. 178 (in Russian).
- Morozov K.V., Demekhin D.N., Bakhtin E.V. Multicomponent strain gauges for assessing the stress-strain state of a rock mass. Mining informational and analytical bulletin. 2022. N 6-2, p. 80-97 (in Russian). DOI: 10.25018/0236_1493_2022_62_0_80
- Kasparyan E.V., Fedotova Yu.V. Stress-strain state in rock mass of the Khibiny deposits and tasks of geomechanical zoning. Mining informational and analytical bulletin. 2015. N 5, p. 97-107 (in Russian).
- Stress state of the earth’s crust (based on measurements in rock masses). Otv. red. P.N. Kropotkin. Moscow: Nauka, 1973, p. 185 (in Russian).
- Ivanov M.A., Popov G.N. Nature of granite fracturing of the Alexander Column – geological aspect. Muzei pod otkrytym nebom. Problemy sokhraneniya pamyatnikov v gorodskoi srede. St. Petersburg: MediaKomfort, 2021, p. 22-25 (in Russian).
- Lyamin N.N. Natural stone building materials of St. Petersburg. Zodchii. 1903. N 45, p. 507-511; N 47, p. 531-535 (in Russian).
- Panova Ye.G., Vlasov A.D., Popova T.A. et al. Biological weathering of granite in urban environments. Biosfera. 2015. Vol. 7. N 1, p. 61-79 (in Russian). DOI: 10.24855/biosfera.v7i1.47
- Sazanova K.V., Zelenskaya M.S., Bobir S.Yu., Vlasovа D.Yu. Micromycetes in the Biofilms on Stone Monuments of Saint Petersburg. Mycology and Phytopathology. 2020. Vol. 54. N 5, p. 329-339 (in Russian). DOI: 10.31857/S0026364820050104
- Vlasov A.D., Nesterov E.M., Rodina O.A., Vlasov D.Yu. Microbial biofilms on rapakivi granite in the Monferran historic quarry. Regional environmental issues. 2020. N 5, p. 6-11 (in Russian). DOI: 10.24412/1728-323X-2020-5-6-11
- Zhao F., Qiu G., Huang Z. et al. Characterization of Rhizobium sp. Q32 isolated from Weathered Rocks and its Role in Silicate Mineral Weathering. Geomicrobiology Journal. 2013. Vol. 30. Iss. 7, p. 616-622. DOI: 10.1080/01490451.2012.746406
- Mustafin M.G., Viet Huu Viet. The Estimation of the Building's Vertical Shifts and Construction Groundworks on the Basis of Deformation Network Element Analysis. Geodesy and Cartography. 2019. Vol. 80. N 3, p. 11-19 (in Russian).
DOI: 10.22389/0016-7126-2019-945-3-11-19 - Zubov A.V., Eliseeva N.N. Software suite for determining the tilts of tower-type structures according to terrestrial laser scanning. Geodesy and Cartography. 2020. Vol. 81. N 7, p. 2-7 (in Russian). DOI: 10.22389/0016-7126-2020-961-7-2-7
- Kornilov Yu.N., Tsareva O.S. Perfecting the methods of monitoring the buildings and structures deformation. Geodesy and Cartography. 2020. Vol. 81. N 4, p. 9-18 (in Russian). DOI: 10.22389/0016-7126-2020-958-4-9-18
- Dashko R.E., Alekseev I.V. On the issue of the role of biocorrosion processes in the underground environment of megalopolises. Engineering Geology World. 2016. N 1. С. 22-29 (in Russian).
- Pokrovskaya E.N., Agapov D.V., Kovalchuk Yu.L. Mycological Investigation of a Wood Substance of Historic Cultural Heritage. Lesnoy zhurnal. 2019. N 4, p. 212-220 (in Russian). DOI: 10.17238/issn0536-1036.2019.4.212
- Trushko V.L., Protosenya A.G. Prospects of Geomechanics Development in the Context of New Technological Paradigm. Journal of Mining Institute. 2019. Vol. 236, p. 162-166. DOI: 10.31897/PMI.2019.2.162
- Kornilov Yu.N., Tsareva O.S., Shevchenko A.S. Optimization of deformation marks location at building a network in the form of a linear spatial intersection. Geodesy and Cartography. 2021. Vol. 82. N 12, p. 2-11 (in Russian). DOI: 10.22389/0016-7126-2021-978-12-2-11
- Hatoum H.M., Mustafin M.G. Optimization of locating robotic total stations for determining the deformations of buildings and structures. Geodesy and Cartography. 2020. Iss. 81. Vol. 9, p. 2-13 (in Russian). DOI: 10.22389/0016-7126-2020-963-9-2-13
- Kuzin A.A., Palkin P.O. Coordinate method for determining position in geodetic monitoring of cracks. Journal of Physics: Conference Series. 2021. Vol. 1728, p 1-6. DOI: 10.1088/1742-6596/1728/1/012010
- Shashkin A.G., Shashkin K.G., Bogov S.G. et al. Monitoring of buildings and structures during construction and operation. St. Petersburg: Izd-vo instituta PI “Georekonstruktsiya”, 2021, p. 640 (in Russian).
- Volynin A.F. Survey of Soils Condition at the Site of Two Deep Sewer Collectors in St. Petersburg using Electrical Prospecting with Capacitively-Coupled Electrodes. Inzhenernaya i rudnaya geofizika: Materialy 17-i nauchno-prakticheskoi konferentsii i vystavki, 26-30 aprelya, 2021, Gelen-dzhik, Rossiya, p. 131-132 (in Russian). DOI: 10.3997/2214-4609.202152150
- Kulikova N.V., Danilev S.M., Efimova N.N., Kulikov A.I. Simulation of seismotomography and electrotomography data for a sand-clay section with the presence of subsurface gas accumulations. Monitoring. Science and Technologies. 2020. N 2 (44), p. 26-30 (in Russian). DOI: 10.25714/MNT.2020.44.004
- Glazunov V.V., Ageev A.S., Gorelik G.D., Sarapulkina T.V. Results of comprehensive geophysical studies on the search for crypts on the territory of suburban necropolis of Tauric Chersonese in the Karantinnaya balka. Journal of Mining Institute. 2021. Vol. 247, p. 12-19. DOI: 10.31897/PMI.2021.1.2
- Potapov A., Pavlov I., Verkhovskaia I. Non-destructive monitoring and technical evaluation conditions of the monument Alexander III. Architecture and Engineering. 2019. Vol. 4. Iss. 1, p. 38-46. DOI: 10.23968/2500-0055-2019-4-1-38-46
- Pashkin E.M. Engineering-geological diagnosis of architectural monuments deformations. Moscow: ANO “Traditsiya”, 2022, p. 368 (in Russian).