Environmental damage from the storage of sulfide ore tailings
- 1 — Ph.D., Dr.Sci. Head of Department Saint Petersburg Mining University ▪ Orcid ▪ Elibrary ▪ Scopus
- 2 — Ph.D. Adjunct Professor United Nations University, Institute for Integrated Management of Material Fluxes and of Resources ▪ Orcid ▪ Scopus
- 3 — Postgraduate Student Saint Petersburg Mining University ▪ Orcid
Abstract
The mining industry is one of the most challenging in ensuring environmental safety. During the last century, the Karabash Copper Smelting Plant was processing sulfide ores and depositing the tailings into storage facilities that now occupy an area of more than 50 hectares. To date, abandoned tailings are a significant source of natural water, air, and soil pollution in the Karabash city district. The article comprehensively examines the environmental impact of the Karabashmed copper smelter, one of the oldest metallurgical enterprises in Russia. The effects of seepage from the two Karabashmed tailings facilities on water resources were assessed. We revealed that even outside the area of the direct impact of processing waste, the pH of natural water decreases to values 4-5. Further downstream, the infiltration water from the tailings pond No. 4 reduces the pH of river water to 3.0-3.5. The presented results of environmental engineering surveys are derived from sampling water and bottom sediments of the Ryzhiy Stream and the Sak-Elga River, sample preparation, and quantitative chemical analysis. The study revealed significant exceedances of the maximum permissible concentrations for a number of chemical elements in the impact zone of the copper ore processing tailings.
Introduction
Waste from mining and processing of minerals poses a severe threat to the environment and human health, especially in spent mines where conservation and reclamation works have not been carried out [1-4], which is comparable to the impact of the most dangerous industries [5, 6]. The natural oxidation of sulfide minerals under the combined action of water and oxygen forms acidic effluents with a high content of sulfates and metal ions in the solution, which can pollute both underground and surface waters [7, 8]. Most often, such effluents are formed during the oxidation of iron sulfides under supergene conditions: pyrite and, less frequently, pyrrhotite [9]. Acid runoff is a significant source of pollution in many mining provinces, and the methods used to reduce environmental impacts are usually expensive and time-consuming. In some cases, work to minimize ecological damage may lead to secondary pollution [10].
Man-made environmental disasters during the extraction and processing of sulfide ores, such as on the tributary of the Guadalquivir River in Spain [11] with a decrease in pH from 8.5 to 4.5 and water pollution with sludge waste containing 0.6 % As, 1.2 % Pb and 0.8 % Zn, or an outflow of 280000 m3 of acid mine waters in the Sichuan Basin in China [12] have been registered and studied in detail in many regions. The long-term anthropogenic load on the natural media in the vicinity of Karabash has led to pollution and degradation of adjacent landscapes. As a result of the activity of the Karabashmed enterprise, an adverse environmental situation has developed in the area of its impact. Since the enterprise was commissioned in 1910, it has been significantly affecting all natural resources due to the insufficient purification of industrial waste gases and the lack of processing of copper ore tailings.
By 1989, the state of the environment in the impact zone of the Karabash copper smelter reached the level of an environmental catastrophe. A significant release of sulfur dioxide into the atmospheric air (about 90 % of the total mass of pollutants) provoked acid rain, damaging the soil cover. Because of the oppression of biocenosis and pollution of soils and water bodies with metals, the Regional Committee for Nature Protection and the Minister of Metallurgy of the USSR decided to stop the copper smelting in Karabash in 1989.
This measure stopped emissions of pollutants into the atmospheric air from the territory of the enterprise and, as a result, improved the environmental situation [13]. For the first time in a long while, the restoration of a phytocenosis began on the western slope of Zolotaya Mountain. In 1998, it was decided to resume the reorganized Karabashmed copper smelting plant operation. The restarted production raised the anthropogenic load and negatively affected the environment. Without modern gas cleaning equipment, emissions of pollutants into the atmospheric air led to a significant excess of the Maximum Permissible Concentrations (MPC), and the lack of a waste recycling system provoked secondary pollution of the atmosphere and hydrosphere. The composition of emissions into the atmospheric air is shown in Fig.1.
Since 2004, the reorganized enterprise has been intensively attempting to reduce the negative impact on the environment, primarily on atmospheric air. Among other environmental measures, copper smelting furnaces were modernized. In 2006, Ausmelt melting units (Australia) were introduced into the production cycle. The commissioning of new equipment took three years, but the first results in reducing the negative environmental impact became noticeable from the moment the new furnaces were installed. The dynamics of the atmospheric emission volumes by various pollutants during the period of copper smelting in 1907-2013 are shown in Fig.2.
Analyzing Fig.2, it can be noted that because of the long-term activity of the enterprise, a signifi-cant mass of pollutants was released into the atmospheric air: more than 14.5 million tons, of which the bulk is sulfur dioxide, inorganic dust, and zinc oxide. In the period from 2007 to 2013, which coincides with the introduction of environmental protection measures at the industrial site, there has been a significant reduction in emissions of sulfur dioxide (a six-fold decrease), dust (300 times), and zinc oxide (100 times).
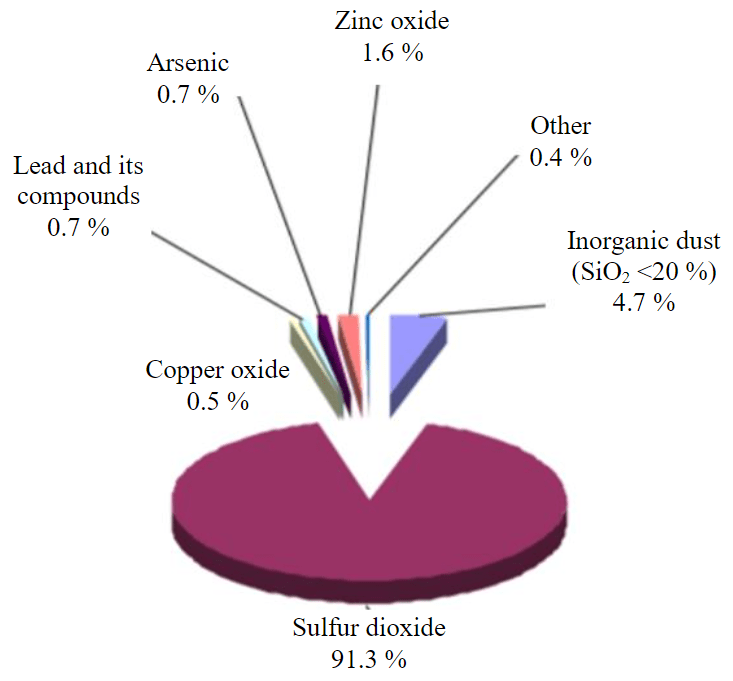
Fig.1. The composition of the main pollutants emitted into the atmospheric air from 1907 to 2006
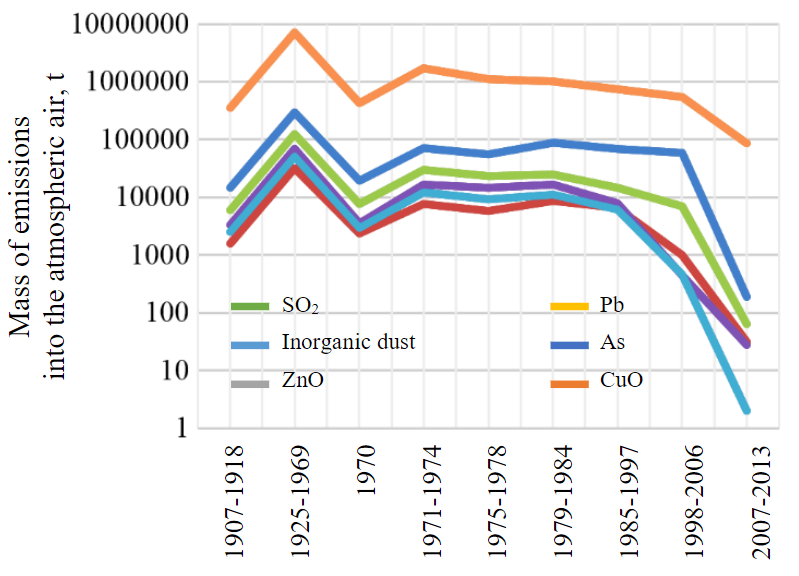
Fig.2. Emissions of pollutants into the atmosphere for the entire period of operation of the copper smelter in the Karabash urban district of Chelyabinsk Oblast (as of 01.01.2014)
The negative impact of copper smelting on the environment is complex [14]. Industrial pollutants released into the atmospheric air accumulate in surface watercourses, bottom sediments, and soils.
The soil cover in the impact zone has been significantly transformed, and because of acid precipitation, the phytocenosis has been suppressed, up to the formation of man-made wastelands. Metals in mobile and immobile modes of occurrence accumulate in the upper layers of the soil cover. The mobile metals are available for absorption by plants, so their contents are significantly heightened in root systems, particularly in copper, zinc, and lead cases. Except for the element accumulation by vegetation, there is a low infiltration rate into the soil with atmospheric precipitation [15, 16].
Depending on climatic conditions, potentially dangerous immobile metals can turn into mobile ones and vice versa. Mobile modes of occurrence of metals pose the greatest danger since they are most accessible to living organisms. As a result, the accumulated environmental damage can affect the vegetation cover in the impact zone of the enterprise for a long time.
Numerous investigators have studied and analyzed the impact of Karabashmed on the quality of the soil cover in the affected area. The results of these analyses are presented in Table 1.
Table 1
Studies of the negative impact of the copper smelter on the soil cover
Source |
Bulk content of elements, mg/kg |
|||||||
Cu |
Zn |
As |
Cd |
Pb |
Cr |
Ni |
Fe |
|
[17] |
12200 |
– |
– |
– |
– |
– |
– |
196700 |
[18] |
4383.2 |
– |
– |
27.3 |
2260.3 |
– |
– |
– |
[19] |
1066 |
10953.2 |
235.8 |
– |
1233.6 |
700.9 |
991.5 |
46.5 |
[20] |
4031.9 |
1882.4 |
724.7 |
19.1 |
1168.7 |
– |
– |
– |
[21] |
2569 |
2655.4 |
– |
11.4 |
586.6 |
– |
– |
– |
[22] |
– |
– |
293.7 |
7.6 |
– |
398.1 |
246.9 |
– |
[23] |
10720 |
3388 |
– |
25 |
– |
– |
– |
48750 |
[24] |
4340 |
1877 |
493 |
12.7 |
900 |
313 |
280 |
4750 |
[25] |
30 |
50 |
– |
6 |
5 |
– |
10 |
220 |
[26] |
3100 |
– |
– |
– |
1300 |
900 |
1200 |
– |
[27] |
637.5 |
3380 |
– |
25.6 |
117.5 |
– |
618 |
– |
[28] |
16416.4 |
6851.8 |
825.5 |
15.2 |
2146.3 |
1191.1 |
944.4 |
257288 |
Background concentration |
20.56 |
17.2 |
– |
1.1 |
6.3 |
– |
7.8 |
– |
MPC* |
3 |
23 |
2 |
2 |
6 |
6 |
4 |
– |
* Maximum Permissible Concentrations are taken from the Russian Sanitary Rules and Norms, 1.2.3685-21 “Hygienic standards and requirements for ensuring the safety and (or) harmlessness of environmental factors for humans”.
Based on the presented results, we conclude that in the area affected by copper smelting, there are many-fold exceedances of the maximum permissible concentrations in the soil cover for several elements: copper, zinc, arsenic, lead, iron, etc. The specific association of the elements indicates that Karabashmed is the source of contamination.
In addition to the negative impact on atmospheric air and soil, pollution of the water basin is observed in the impact zone of Karabashmed and around the tailings storage facilities. The main pollutants of surface watercourses – primarily of the Sak-Elga River and the Argazinskiy Reservoir – are the drainage waters from tailings, stormwater, and industrial effluents of the enterprise, the collapse zone of the spent Stalinskiy (Tisovskiy) mine, the Ryzhiy Stream, sulfide-silicate deposits in the river floodplain (Fig.3).
When infiltrating through the tailings, rain and meltwater are enriched with potentially toxic elements which and later discharge into water bodies. The Ryzhiy Stream is one of the most well-known pollution sources in the study area (Fig.4, a). Its waters are characterized by extremely high metal content.
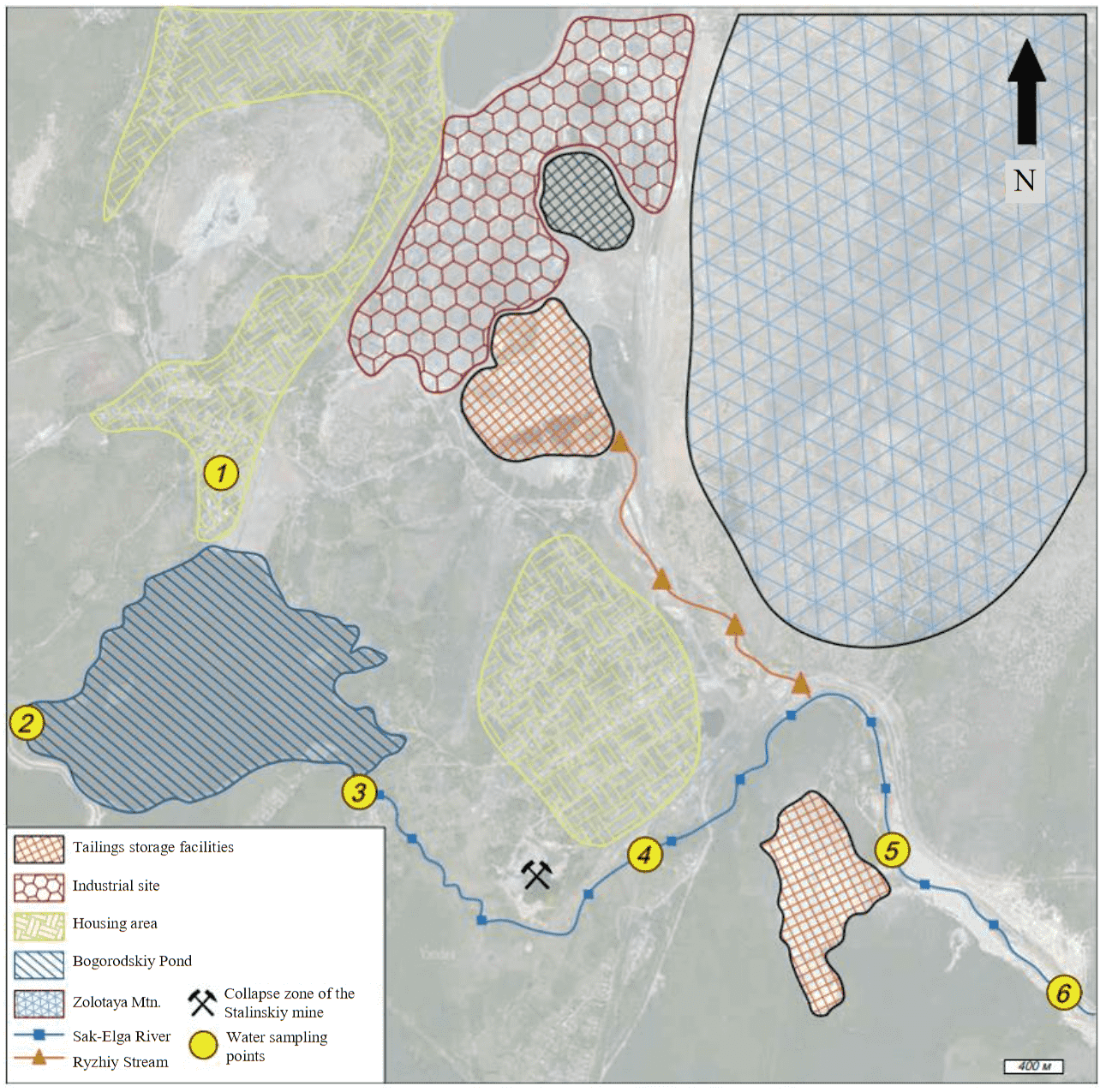
Fig.3. Sketch map of the study area
The stream flows into the Sak-Elga River. Due to the difference in debits (the ratio is from 1:12 to 1:30 depending on the season), the metal content in the water decreases by two orders of magnitude.
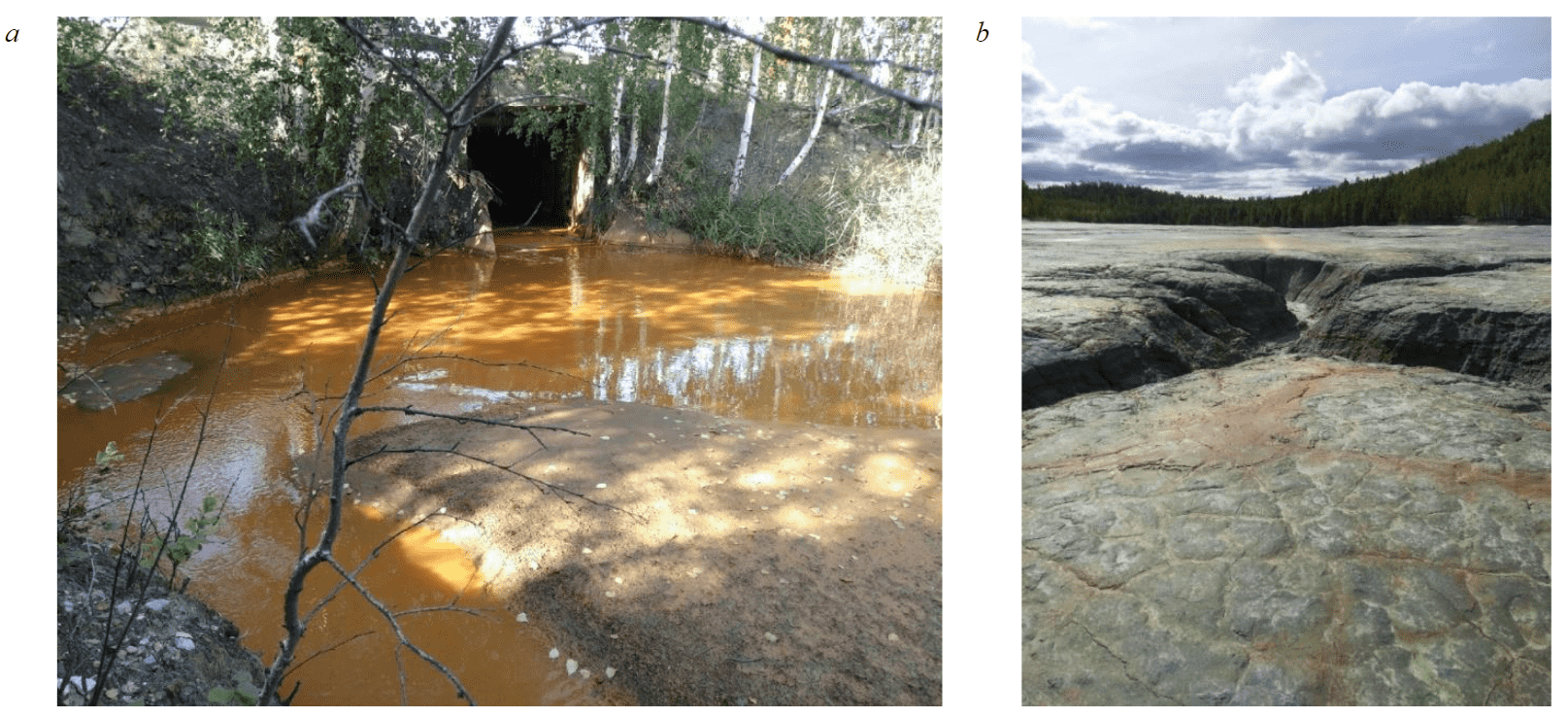
Fig.4. Sources of pollution: the Ryzhiy Stream (a), the tailings storage facility N 4 (“Novoye”) (b)
Many authors have analyzed the negative impact of tailings and industrial wastewater in various studies. During the field campaign, we investigated the Ryzhiy Stream, its confluence with the Sak-Elga River, mine waters, and certain lakes and reservoirs of Chelyabinsk Oblast. As a result of the dilution of the acidic polluted waters of the Ryzhiy Stream with the water of the Sak-Elga River, which has an average pH of 7.5, an alkaline hydrolytic geochemical barrier is formed. Metals precipitate there as hydroxides accumulated in the bottom sediments. Metal concentrations in bottom sediments are many-fold higher than the MPC, and these deposits can be secondary pollutants of water resources. The results of the analysis of water pollution by various authors are presented in Table 2.
Table 2
Studies on the negative impact of the copper smelter on water resources
Source |
pH |
Bulk content of elements, mg/l |
|||||||
Cu |
Zn |
Cd |
Pb |
Fe |
Ni |
As |
SO4 |
||
[29] (the Ryzhiy Stream) |
1.58 |
132.5 |
420.8 |
2.49 |
3.72 |
382.4 |
4.63 |
– |
– |
[29] (mine drainage, 1994) |
2.1 |
24 |
350 |
– |
– |
70 |
– |
0 |
3900 |
[29] (mine drainage, 1997) |
2.5 |
35 |
100 |
– |
– |
170 |
– |
0 |
6500 |
[29] (mine drainage, 1998) |
2.7 |
13 |
0 |
– |
– |
0 |
– |
– |
2000 |
[29] (mine drainage, 2000) |
6.6 |
0 |
0 |
– |
– |
0 |
– |
– |
500 |
[29] (mine drainage, 2014, 70 m) |
– |
0.02 |
0.2 |
– |
– |
0.05 |
– |
– |
230 |
[30] (the Ryzhiy Stream, 2008) |
2 |
55 |
96 |
0.43 |
0.012 |
390 |
– |
0.015 |
– |
[31] (lakes, 2001) |
7.1 |
9.3 |
107 |
0.2 |
1.5 |
334 |
3.6 |
– |
1557 |
[32] (Sak-Elga River, 2014) |
3.8 |
0.85 |
– |
– |
– |
72.1 |
– |
– |
– |
[33] (the Ryzhiy Stream, 2014) |
5.25 |
30 |
65 |
– |
– |
180 |
– |
– |
– |
[34] (lake Uvildy, 2005) |
– |
12.4 |
12 |
– |
– |
– |
– |
– |
– |
[35] (the Ryzhiy Stream, 2016) |
2.7 |
51.34 |
167.39 |
1.1 |
1.38 |
629.15 |
3.42 |
0.62 |
– |
[36] (the Ryzhiy Stream, 2011) |
– |
75 |
100 |
0.8 |
0.1 |
700 |
- |
0.01 |
– |
[37] (the Ryzhiy Stream, 2017) |
– |
45 |
40 |
1.3 |
1.2 |
42 |
0.9 |
- |
– |
[38] (drainage water, 2017) |
– |
87 |
47 |
– |
– |
1200 |
– |
1.8 |
– |
[24] (Sak-Elga River, 2011) |
2.4-2.7 |
58 |
87 |
– |
1.5 |
375 |
– |
– |
– |
[39] (drainage water, 2018) |
2.03 |
458 |
543 |
– |
– |
5674 |
– |
– |
– |
[40] (the Ryzhiy Stream, 2014) |
3 |
50 |
2 |
– |
0.5 |
200 |
– |
– |
90 |
Background concentration |
6.82 |
3.2 |
11.2 |
0.02 |
0.27 |
7.68 |
4.25 |
– |
– |
MPC* |
– |
0.001 |
0.01 |
0.005 |
0.006 |
0.1 |
0.01 |
0.05 |
100 |
* Maximum Permissible Concentrations are taken from the Order of the Ministry of Agriculture of the Russian Federation of December 13, 2016, N 552.
In addition to the direct atmospheric air pollution, Karabashmed negatively impacts the hydro- and lithosphere through the accumulated waste of copper ore processing. The industrial cycle includes flotation, which produces vast volumes of tailings, i.e., a finely dispersed mass of aluminosilicate and sulfide composition [41]. Residuals of this type should be stored in specially equipped facilities. Tailings are a constant source of secondary environmental pollution: dusting surfaces increase the load on atmospheric air and promote the migration of pollutants, and the absence or violation of the integrity of hydrofiltration screens contributes to the infiltration of pollutants with storm drains into soils and water bodies.
In the vicinity of Karabash, two tailings storage facilities (TSF) affect the environment most significantly: the TSF N 3 with 4.5 million tons of accumulated waste and the TSF N 4 (“Novoye”) with 5.9 million tons (Fig.4, b).
The range of studies on tailings’ chemical and phase composition is minimal. In this regard, the question of studying the composition of tailings at different depths and the dynamics and nature of the wastewater infiltration process remains open. Table 3 presents the results of various authors’ chemical analyses of the tailings.
Table 3
Studies of the chemical composition of accumulated processing tailings
Source |
Bulk content of elements, mg/kg |
|||||
Cu |
Zn |
Fe |
As |
Pb |
Mn |
|
[30] |
370 |
330 |
77000 |
170 |
230 |
– |
[36] |
500 |
500 |
100000 |
100 |
250 |
– |
[40] |
2000 |
800 |
124000 |
– |
– |
– |
[29] |
1540 |
1572 |
337421 |
– |
4116 |
435 |
[42] |
1066.9 |
10953 |
– |
235.8 |
1233 |
3374.8 |
[43] |
370 |
390 |
– |
– |
– |
– |
[33] |
6 |
10.4 |
220 |
– |
1 |
– |
MPC* |
3 |
23 |
– |
2 |
6 |
60 |
* Maximum Permissible Concentrations are taken from the Russian Sanitary Rules and Norms, 1.2.3685-21 “Hygienic standards and requirements for ensuring the safety and (or) harmlessness of environmental factors for humans”.
To reduce the negative impact of accumulated copper processing waste, it is necessary to develop comprehensive environmental protection solutions, including a project for the reclamation of tailings bodies and wastewater treatment [44].
The main objectives of the work are (i) to monitor the zone of the negative environmental impact of tailings; (ii) to identify distribution patterns of pollutants from the TSF; (iii) to assess the possibility of recycling accumulated copper processing waste; (iv) to examine the correlation between concentrations of valuable elements and the depth of the tailings; and (v) to forecast the feasibility of develo-ping a comprehensive environmental protection system in contaminated areas.
Methods
The technogenic sediments of the Karabash copper smelting plant are a typical example of accumulated environmental harm. The TSF N 3 and N 4 are of particular interest. The conside-red tailings are the residuals of the processing of sulfide copper-pyrite ores. Their composition is determined by the mineral composition of the ore entering the processing plant. Pyritic deposits, as a rule, are characterized by a variety of forms and types of occurrence of minerals in host rocks. Copper-pyrite deposits of the Southern Urals are represented by iron, copper, and zinc sulfides: 60-90 % pyrite, 0.2-25 % sphalerite, and 0.1-10 % chalcopyrite. Tennantite, galena, magnetite, hematite, bornite, etc., are minor minerals. Quartz, sericite, chlorite, barite, and calcite are the most common non-metallic minerals.
The studied TSF Nos. 3 and 4 were formed from 1958 to 1990 with multi-tiered protecting dams of ca. 10 m in height. To assess the potential danger of sulfide-containing waste, monitoring studies were conducted in the area adjacent to the tailings of the Karabash copper smelter. The TSF N 3 covers an area of 39.5 hectares and accommodates, according to various estimates, from 3.0 to 4.5 million tons of tailings. The TSF N 4 occupies an area of 27.7 hectares and holds from 4.0 to 5.9 million tons of tailings. In the vertical section of the TSF N 4, three contrasting zones are distinguished: an oxidation zone up to 1.7 m deep from the daylight surface; a cementation zone with a thickness of up to 1.5 m; and a cementation zone with signs of secondary sulfide accumulation. Below a depth of 3 m, conspicuous layers of black color appear with a characteristic smell of organic matter, which indicates the reducing environment with little or no free oxygen.
The conducted studies allowed us to assess the level of transformation of artificial deposits, the possibility of their recycling to extract potential mineral raw materials, and the level of their impact on natural resources.
The monitoring included visual assessment of the tailings; sampling of the section from the surface and up to a depth of 4 m at the TSF Nos. 3 and 4; and sampling of water and bottom sediments of the Sak-Elga River.
To assess the vertical migration of pollutants and the degree of preservation of valuable components in the TSF Nos. 3 and 4, we drilled 12 wells to different depths. Sampling was carried out in the summer (August 2020) following GOST 12071-2014 “Soils. Sampling, packing, transportation and keeping of samples”. We took 46 samples using a manual drill sampler with a rotary handle. The description of the sampling conditions is shown in Fig.5.
The samples from the tailings were placed in sealed polyethylene bags for further transportation in a cooled state. Sample preparation was carried out in the laboratory of the Research and Educational Center for the Collective Use of High-Tech Equipment at Saint Petersburg Mining University.
The qualitative and quantitative composition of the tailings was determined using the Shimadzu XRF-1800 X-ray fluorescence spectrometer. The analysis was carried out following GOST R 56157-2014 “Soil. Procedures (methods) of analysis of composition and properties of soil samples. General requirements for development”. The samples were dehumidified in a drying cabinet at a temperature of 40 °C for several days until the mass stabilized. A ball mill was used to grind the samples. The samples were crushed until a dispersion of 0.35 mm was reached. The powdered samples were mixed with a binder (cellulose) and pressed into tablets using the Spex manual hydraulic press.
The gross content of copper, gold, and silver in the studied tailings was determined using an ICPE-9000 optical emission spectrometer.
The decomposition of samples to determine the gross content of elements was carried out according to the MVI-80-2008 method, “Methodology for measuring the mass fraction of elements in soil and bottom sediment samples by atomic emission and atomic absorption spectrometry”.
To assess the migration ability of potentially toxic impurities washed out by precipitation, we examined the Sak-Elga River upstream and downstream from the influx into the Bogorodskiy Pond; upstream and downstream from the confluence with the Ryzhiy Stream; and in the area of unloading acidic effluents from the TSF N 4.
The determination of potentially toxic elements in the waters was carried out using the method of atomic absorption spectrometry and induction-coupled plasma spectrometry. Analytical work was carried out on the Shimadzu ICPE-9000 device and the Shimadzu atomic absorption spectrometer AA6300 (Japan).
Determination of the content of elements in sediment samples was carried out according to RD 52.18.289-2022 “Mass fraction of mobile forms of metals in soil. Measurement technique by atomic absorption spectrometry”.
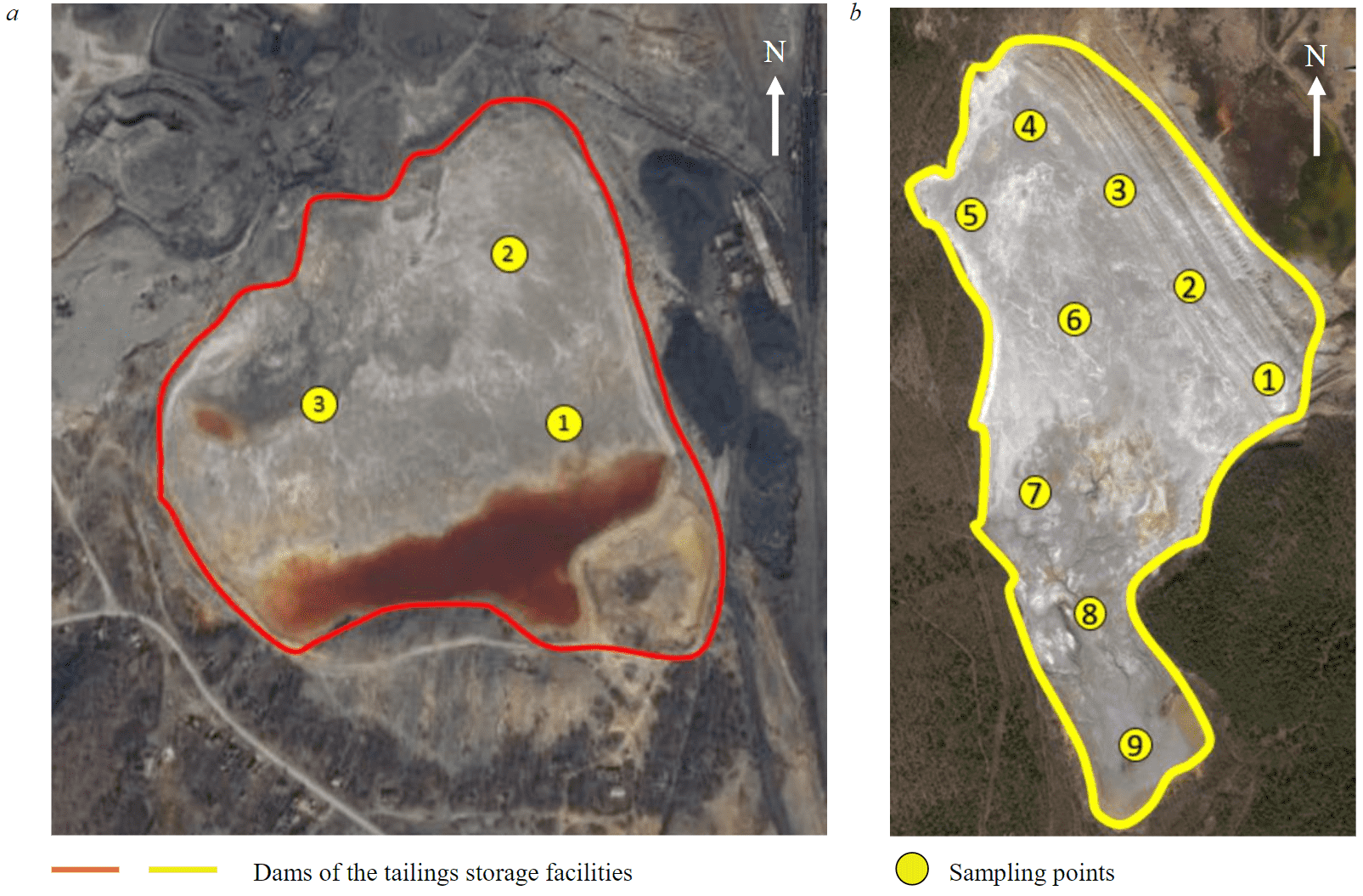
Fig.5. Characteristics of sampling conditions in the territories of the storage facilities N 3 (a) and N 4 (b) N 1: 3 samples from the depth of 0; 0.5; and 1 m; N 2: 2 samples from 0 and 0.5 m; N 3: 4 samples from 0; 0.5; 1; and 2 m (а); N 1: 4 samples from the depth of 0; 0.5; 1; and 2 m; N 2: 6 samples from 0; 0.5; 1; 2; 3; and 4 m; N 3: 5 samples from 0; 0.5; 1; 2; and 3 m; N 4: 4 samples from 0; 0.5; 1; and 2 m; N 5: 5 samples from 0; 0.5; 1; 2; and 2.5 m; N 6: 4 samples from 0; 0.5; 1; and 2 m; N 7: 4 samples from 0; 0.5; 1; and 1.5 m; N 8: 1 superficial sample; N 9: 4 samples from 0; 0.5; 1; and 2 m (b)
Results and discussion
The conducted studies allowed us to establish that acid drainage runoff is formed during the infiltration of atmospheric precipitation through the body of the TSF. The physico-chemical composition of the solution directly depends on the minerals composing the body of the tailings through which it passes.
The analysis of tailings sampled from different horizons makes it possible to identify a direct dependence of the concentration of pollutants on the depth of a layer. Thus, the water accumulates the maximum mass of pollutants in an acidic environment while passing from the surface of the TSF to its base. The sulfide minerals that make up the bulk of the TSF react with the sediments of a neutral medium and produce a highly acidic solution. The resulting acidic runoff mobilizes non-ferrous metals and provokes their migration into the deeper horizons with the subsequent increase in their concentration therein.
Drainage sub-basement waters reach aquifers and the nearest surface watercourses. In this case, the main receiver of such waters is the Ryzhiy Stream, which flows into the Sak-Elga River, a tributary of the Miass River.
The Ryzhiy Stream has exceptionally high concentrations of metals in its waters. At its confluence with the Sak-Elga River, a complete transformation of river waters occurs, characterized by a drop in pH to 3.0, and water quality deteriorates significantly at the point of mixing of watercourses. During the summer low streamflow period, the qualitative elemental composition of river waters becomes close to the composition of sub-basement waters. The excess concentrations of copper, zinc, iron, and manganese over the natural background values reach a 1000-fold level. After the confluence of the Ryzhiy Stream into the Sak-Elga River, the concentrations of pollutants gradually decrease downstream due to the difference in the flow rates; however, the waters are still characterized by a high pollution level.
The analysis of the content of mobile forms of metals in absolutely dry samples of bottom sediments confirms the negative anthropogenic impact of infiltration wastewater from the accumulated processing waste on the qualitative composition of the Sak-Elga River. The maximum concentration of mobile forms of metals is observed at point 5 in cases of copper, 1990.0 mg/kg (Enrichment Factor, EF = 663.3); zinc, 5750.0 mg/kg (EF = 250.0); arsenic – 172.8 mg/kg (EF = 86.4); nickel, 128.0 mg/kg (EF = 32.0); iron, 21,266.0 mg/kg. At point 6, the content of mobile forms of metals decreases, but their concentrations also significantly exceed the MPC: copper, 613.0 mg/kg (EF = 204.3); zinc, 4900.0 (EF = 213.0); arsenic, 68.0 (EF = 34.0); nickel, 116.0 (EF = 29.0); iron, 19,080.0.
In addition, dry tailings are exposed to wind erosion that creates man-made dust deposits on the soil and vegetation cover over an area of more than 40 km2, high-contrast in metal content with a total EF of more than 400, which corresponds to an environmental disaster zone. The geochemical association of metals in technogenic sediments is as follows: Hg148-Cu87-Sb43-Ag40-Pb37-As20-Bi19-Zn13-Ni1.7.
The combination of these factors indicates the need to significantly reduce the environmental load by eliminating the impact of the accumulated waste. There is a need to assess the possible recycling of accumulated waste. For this purpose we analyzed the tailings from the storage facilities Nos. 3 and 4 (Table 4).
Table 4
Composition of the samples from the processing tailings, g/t
Compound |
Tailings Storage Facility N 3 |
Tailings Storage Facility N 4 |
||||
Depth, m |
0.5 |
1 |
2 |
1 |
2 |
4 |
SO3 |
441.9 |
407.2 |
440.3 |
252.1 |
331.3 |
366.9 |
Fe2O3 |
263.3 |
265.1 |
271.2 |
166.6 |
207.9 |
195.9 |
SiO2 |
194.2 |
201.5 |
167.5 |
388.7 |
300.1 |
265.9 |
Al2O3 |
52.6 |
56.8 |
57.8 |
12.4 |
83.9 |
108.8 |
CaO |
21.4 |
30.9 |
25.8 |
36.6 |
26.3 |
19.1 |
K2O |
10.1 |
10.0 |
8.2 |
17.3 |
12.6 |
15.1 |
BaO |
5.9 |
5.1 |
5.1 |
5.3 |
8.3 |
4.5 |
TiO2 |
1.5 |
2.2 |
1.3 |
3.3 |
2.6 |
1.8 |
ZnO |
1.4 |
1.8 |
1.5 |
0.9 |
0.9 |
0.5 |
As2O3 |
0.3 |
0.5 |
0.6 |
0.6 |
035 |
0.2 |
PbO |
0.3 |
0.3 |
0.3 |
0.1 |
031 |
0.2 |
ZrO2 |
0.1 |
0.1 |
0.06 |
0.1 |
0.1 |
0.08 |
SrO |
0.07 |
007 |
0.06 |
0.08 |
0.1 |
0.06 |
MgO |
2.8 |
9.9 |
9.2 |
16.2 |
16.9 |
12.6 |
Cu |
884.8 |
3732.4 |
5526.6 |
1916.5 |
2459.2 |
4464 |
Au |
0.77 |
0.82 |
0.87 |
0.19 |
1.17 |
0.49 |
Ag |
9.76 |
11.33 |
14.59 |
1.83 |
2.01 |
7.42 |
The results of the analysis of TSF Nos. 3 and 4 show (Fig.6) that iron, sulfur, aluminum, and silicon are the major elements, while gold and silver are significant impurities.
Pyrite (FeS2) is the tailings’ main mineral, forming iron sulfate and sulfuric acid when interacting with atmospheric precipitation and oxygen. With the decomposition of residual pyrite, drainage waters containing sulfuric acid and trivalent iron sulfate are formed, which leads to significant pollution of aquifers and surface watercourses.
A layer-by-layer sampling of tailings from different depths revealed the regularity of the accumulation of non-ferrous metals and the growth of their content with increasing depth. The gold content in the tailings (0.83-3.48 g/t) is comparable to its levels in pyrite ores, so the waste can be regarded as a potential technogenic mineral raw material suitable for recycling to extract valuable components. We carried out an experiment simulating the process of recycling the accumulated tailings. The Albion acid leaching method was taken as a basis. An installation for process simulation in laboratory conditions has been designed. The lab-scale plant consists of a 2000 ml glass cup, a turbine agitator, a Memmert water bath, an oxygen tank, and a 150MI pH meter with a Hanna electrode.
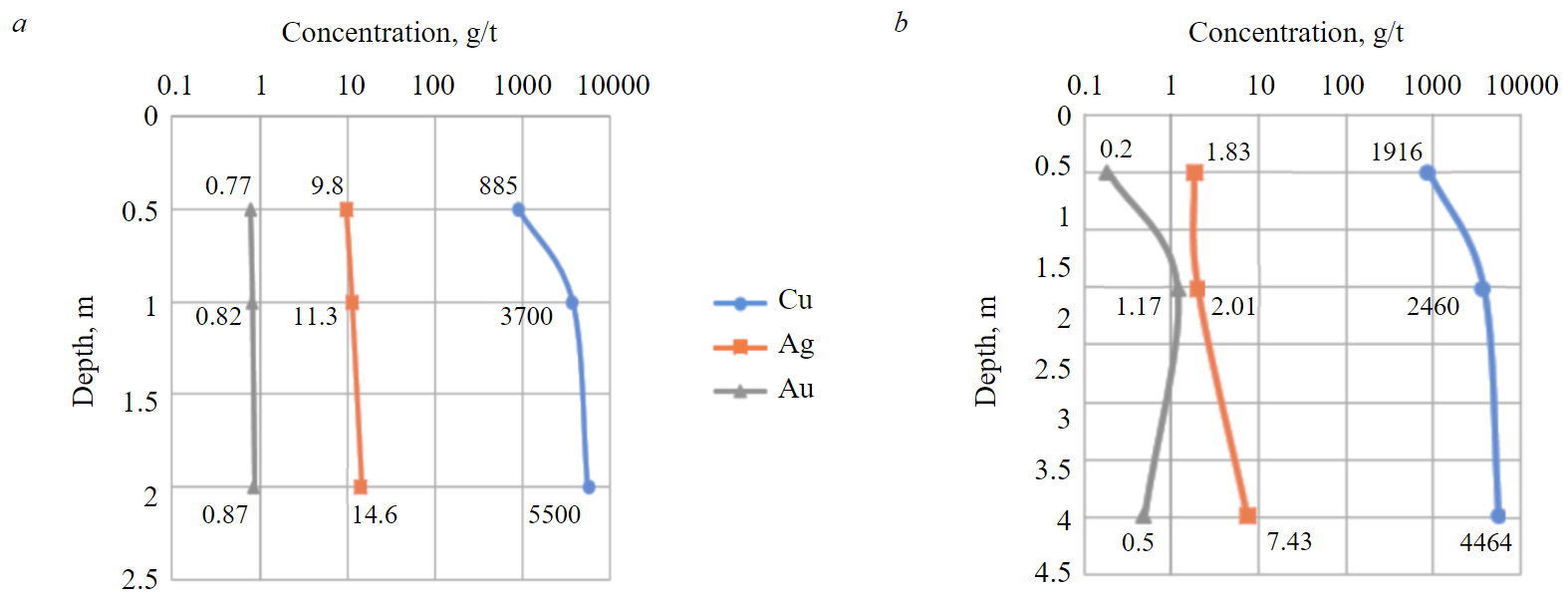
Fig.6. Distribution of elements along the vertical sections of tailings N 3 (a) and N 4 (b)
During the experiment, the leaching of copper and zinc from low-grade finely ground rocks was stimulated for 48 hours at an elevated temperature of ca. 95 °C with further filtration through a Bunsen flask. Due to the limited mass of material, the experiment was carried out on the tailings from the facility N 3 (point 3, 2 m depth) with average contents of 0.72 % Cu and 0.92 % Zn.
The resulting green color of the solution indicates an intensive transition of zinc into the solution, which was confirmed by analyses. The results of the experiment are shown in Table 5.
Secondary waste processing using the Albion method aims to extract at least 98 % of copper and zinc. The production of copper, as the main valuable component, at the level of 43-44 % does not correspond to the degree of extraction at the current smelting plant, 98.5 %. It can be concluded that recycling the tailings by acid leaching is economically impractical.
Table 5
Changes in the content of Cu and Zn during the simulation of the Albion process
Element |
Initial content in tailings, % |
Initial content in 1 liter |
End content in 1 liter |
Experimental extraction, % |
Extraction at the processing plant, % |
Cu |
0.72 |
3456 |
1510.4 |
43.7 |
98.5 |
Zn |
0.92 |
4416 |
3888.1 |
88.0 |
99.0 |
It is necessary to develop environmental protection measures to reduce the negative impact of accumulated waste by combining methods of step-by-step land rehabilitation and the treatment of sub-basement wastewater. The approach includes the phased implementation of the engineering reclamation and biological restoration on the entire surface of TSF Nos. 3 and 4 with a solution for the neutralization of infiltrating acid effluents. One such solution may be the creation of a geochemical barrier with an alkaline and/or sorption filter loading for neutralization and sorption of acidic wastewater in the area of their discharge along the perimeter of tailings.
It is also possible to preserve man-made deposits by shielding the surface with compacted natural clays or insulating the walls and surface of tailings with polymer sheets (geomembranes) with further revegetation. These conservation options will prevent the discharge of acid effluents from tailings dumps into surface watercourses, as well as preserve a potential technogenic deposit for further extraction of useful components.
Conclusion
A review of the published data allows us to conclude that the enterprise and the accumulated waste from the processing of copper ores have a negative environmental impact. In the period from 1907 to 2006, the atmospheric air was affected eminently, as over 14 million tons of pollutants were emitted. To date, the volume of pollutant emissions into the air has been reduced down to the maximum permissible concentrations thanks to the new gas cleaning equipment. Accumulated waste from the processing of copper ores continues to pollute the environment. The drainage runoff of tailings dumps is discharged into groundwater and surface watercourses, polluting them with dissolved metals; due to prolonged pollution, bottom sediments with high concentrations of metals are formed in the Sak-Elga River.
The analysis of the water samples and tailings revealed that: 1) the water of the Ryzhiy Stream contains high concentrations of metals – Cu (more than 5000-fold exceedance over the MPC), Zn (10,000-fold), Fe (more than 3900-fold), etc., and pollutes the Sak-Elga River; 2) the levels of non-ferrous metals in the tailings increases with the depth. At a depth of more than 2 m from the surface of the tailings, the concentrations of non-ferrous metals are Cu 5500 g/t; Au 0.87 g/t; and
Ag 14.6 g/t. This fact allows us to consider tailings storage facilities Nos. 3 and 4 as secondary sources of mineral resources.
The experiment on the secondary processing of accumulated waste showed that acid leaching is impractical. The extraction ratio was 43.7 % for copper and 88 % for zinc. At the same time, the extraction of copper and zinc from the prepared ore with a similar metal content at the processing plant reaches 99 %. It is necessary to develop a comprehensive solution to reduce the negative impact of accumulated waste by reclamation of the tailings storage facilities.
References
- Sudarikov S.M., Yungmeister D.A., Korolev R.I., Petrov V.A. On the possibility of reducing man-made burden on benthic biotic communities when mining solid minerals using technical means of various designs. Journal of Mining Institute. Vol. 253, p. 82-96. DOI: 10.31897/PMI.2022.14
- Cánovas C.R., Macías F., Basallote M.D. et al. Metal (loid) release from sulfide-rich wastes to the environment: The case of the Iberian Pyrite Belt (SW Spain). Current Opinion in Environmental Science and Health. 2021. Vol. 20. N 100240.
DOI: 10.1016/j.coesh.2021.100240 - Danilov A.S., Smirnov Y.D., Korelskiy D.S. Effective methods for reclamation of area sources of dust emission. Journal of Ecological Engineering. 2017. Vol. 18. Iss. 5, p. 1-7. DOI: 10.12911/22998993/74947
- Plokhov A.S., Kharko P.A., Pashkevich M.A. Effect of tailings storage facility on surface water at copper-pyrite deposit. Mining Informational and Analytical Bulletin. N 4, p. 57-68 (in Russian). DOI: 10.25018/0236_1493_2021_4_0_57
- Erzova V.A., Rumynin V.G., Sudarikov S.M. et al. Influence of North-West Nuclear Complexfacilities on Groundwater Contamination (Leningrad Region). Bulletin of the Tomsk Polytechnic University. Geo Assets Engineering. Vol. 332. N 9, p. 30-42 (in Russian). DOI: 10.18799/24131830/2021/9/3351
- Rumynin V.G., Nikulenkov A.M., Rozov K.B. et al. The status and trends in radioactive contamination of groundwater at a LLW-ILW storage facility site near Sosnovy Bor (Leningrad region, Russia). Journal of Environmental Radioactivity. 2021. Vol. 237. N 106707. DOI: 10.1016/j.jenvrad.2021.106707
- Pozo-Antonio S., Puente-Luna I., Lagüela-López S., Veiga-Ríos M. Techniques to correct and prevent acid mine drainage: A review. DYNA. 2014. Vol. 81, p. 73-80. DOI: 10.15446/dyna.v81n186.38436
- Kharko P.A., Matveeva V.A. Bottom Sediments in a River under Acid and Alkaline Wastewater Discharge. Ecological Engineering & Environmental Technology. 2021. Vol. 22. N 3, p. 35-41. DOI: 10.12912/27197050/134870
- Kasimov N.S. Landscape Ecogeochemistry. Мoscow: Publisher Filimonov М.V., 2013, p. 208 (in Russian).
- Zhihong Tu, Qi Wu, Hongping He et al. Reduction of acid mine drainage by passivation of pyrite surfaces: A review. Science of the Total Environment. 2022. Vol. 832. N 155116. DOI: 10.1016/j.scitotenv.2022.155116
- Pain D.J., Sánchez A., Meharg A.A. The Donana ecological disaster: Contamination of a world heritage estuarine marsh ecosystem with acidified pyrite mine waste. Science of the Total Environment. 1998. Vol. 222, p. 45-54. DOI: 10.1016/S0048-9697(98)00290-3
- Bo Li, Guo Liu, Yuhan Nie, Zhong Ye. Causes and Effects of a Water Spill from the Underground Pit of the Dashu Pyrite Mine, Southern Sichuan Basin, Southwest China. Mine Water and the Environment. 2021. Vol. 40. Iss. 4, p. 864-876. DOI: 10.1007/s10230-021-00825-8
- Bachurina A.V., Zalesov S.V., Tolkach O.V. The Еffectiveness of Disturbed Lands Forest Reclamation in the Zone of Copper-melting Works. Ecology and Industry of Russia. 24. N 6, p. 67-71 (in Russian). DOI: 10.18412/1816-0395-2020-6-67-71
- Argimbaev K.R., Kornev A.V., Kholodnyakov G.A. Substantiation of the Possibility of Involvement in Processing of the Iron-Containing Tails with the Subsequent Clotting of the Obtained Concentrates. Journal of Mining Institute. 2013. Vol. 206, p. 120-124 (in Russian).
- Matveeva V.A., Lytaeva T., Danilov A.S. Application of Steel-Smelting Slags as Material for Reclamation of Disturbed Lands. Journal of Ecological Engineering. Vol. 19. Iss. 6, p. 97-103. DOI: 10.12911/22998993/93511
- Petrov G.V., Kalashnikova M.I., Fokina S.B. Regularities of selenium and chromium behavior in redox processes during hydrometallurgic treatment of solid phase products of rhenium extraction. Journal of Mining Institute. Vol. 220, p. 601. DOI: 10.18454/pmi.2016.4.601
- Vodyanitskii Y.N., Minkina T.M., Kubrin S.V. et al. Iron sulphides and their effect on the XRF measurement of the bulk chemical composition of badland soils near the Karabash copper smelter, Southern Urals, Russia. Geochemistry: Exploration, Environment, Analysis. 2018. Vol. 19. Iss. 2, p. 176-183. DOI: 10.1144/geochem2017-081
- Smorkalov I.A., Vorobeichik E.L. Soil respiration of forest ecosystems in gradients of environmental pollution by emissions from copper smelters. Russian Journal of Ecology. Vol. 42. N 6, p. 464-470 (in Russian). DOI: 10.1134/S1067413611060166
- Minkina T.M., Linnik V.G., Nevidomskaya D.G. et al. Forms of Cu (II), Zn (II), and Pb (II) compounds in technogenically transformed soils adjacent to the Karabashmed copper smelter. Journal of Soils and Sediments. 2018. Vol. 18. Iss. 6, p. 2229-2230. DOI: 10.1007/s11368-017-1777-2
- Kumar A.T., Maleva M., Kiseleva I. et al. Toxic metal(loid)s contamination and potential human health risk assessment in the vicinity of century-old copper smelter, Karabash, Russia. Environmental Geochemistry and Health. 2020. Vol. 42. Iss. 12, p. 4113-4124. DOI: 10.1007/s10653-019-00414-3
- Shimalina N.S., Pozolotina V.N., Orekhova N.A., Antonova E.V. Assessment of biological effects in Plantago major L. Seed progeny in the zone of impact from a Copper Smelter. Russian Journal of Ecology. Vol. 48. N 6, p. 513-523 (in Russian). DOI: 10.1134/S1067413617060108
- Lezhnin V.L., Kazantsev V.S., Polzik E.V. A Multifactor Assessment of Effects of Technogenic Pollution on the Occurrence of Lung Cancer in the Population of an Industrial Town. Hygiene and Sanitation. Vol. 93, p. 26-30 (in Russian).
- Elizareva E.N., Yanbaev Y.A., Redkina N.N. et al. Assessment of Soil Pollution in the Zone of Influence of Metallurgical Enterprises. Vestnik of Orenburg State Pedagogical University. N 9, p. 8-13 (in Russian).
- Tatsy Y.G. Assessment of the Distribution of Heavy Metals around a Cu Smelter Town, Karabash, South Urals, Russia. E3S Web of Conferences. Vol. 1. N 19010. DOI: 10.1051/e3sconf/20130119010
- Ulrikh D.V., Timofeeva S.S. Phytoremediation of Polluted Soil and Industrially Affected Subsoil of Waste Storage in the Copper Mining Areas in the South Ural. Mining Informational and Analytical Bulletin. N. 3, p. 341-349 (in Russian).
- Tatsii Y.G., Udachin V.N., Aminov P.G. Environmental geochemistry of mercury in the area of emissions of the Karabashmed copper smelter. Geochemistry International. Vol. 55. N 10, p. 935-945. DOI: 10.1134/S0016702917100093
- Shabanov M.V., Strekulev G.B. Geochemical Processes of Accumulation of Heavy Metals in the Landscapes of the Southern Urals. Bulletin of the Tomsk Polytechnic University. Geo Assets Engineering. Vol. 332. N 1, p. 184-192 (in Russian). DOI: 10.18799/24131830/2021/1/3011
- Mikhailov G.G., Lonzinger T.M., Morozova A.G. et al. Evaluation of Effectiveness of Composite Sorbent For Rehabilitation of Contaminated Sites of Karabash Industrial Area. Bulletin of the South Ural State University. Series “Metallurgy”. Vol. 14. N 3, p. 12-18 (in Russian).
- Ulrikh D.V. Scientific substantiation and development of technologies for the integrated restoration of technogenically disturbed territories in the areas of mining and processing of copper ores: dis. … d-ra tekhn. nauk. Chelyabinsk: Yuzhno-Uralskii gosudarstvennyi universitet, 2020, p. 44 (in Russian).
- Yurkevich N.V., Saeva O.P., Pal’chik N.A. Arsenic mobility in two mine tailings drainage systems and its removal from solution by natural geochemical barriers. Applied Geochemistry. 2012. Vol. 27. Iss. 11, p. 2260-2270. DOI: 10.1016/j.apgeochem.2012.05.012
- Brooks S.J., Udachin V., Williamson B.J. Impact of copper smelting on lakes in the southern Ural Mountains, Russia, inferred from chironomids. Journal of Paleolimnology. Vol. 33. Iss. 2, p. 229-241. DOI: 10.1007/s10933-004-3936-x
- Denisov S.E., Ulrikh D.V., Zhbankov G.O. Formation of Hydrochemical River Regime under Extreme Contamination by Waste Water (the Sak-Elga River in the Chelyabinsk Region). IOP Conference Series: Materials Science and Engineering. 2017. Vol. 262. N 012186. DOI: 10.1088/1757-899X/262/1/012186
- Korneeva T.V., Kucher D.O. Complex of Geochemical and Geophysical Approaches Using for Investigation of the Vertical and Lateral Distribution of Highly Toxic Solutions (Karabash District). Interexpo GEO-Siberia. Vol. 2, p. 116-121 (in Russian).
- Rogozin A.G., Gavrilkina S.V. Causes for high concentration of copper and zinc in the water of some lakes in the Southern Urals. Water Resources. 2008. Vol. 35. Iss. 6, p. 701-707. DOI: 10.1134/S0097807808060092
- Mikhailov G.G., Morozova A.G., Lonzinger T.M. Research of Surface Phase Formation in Interaction of Granular Composite Sorbent with Technological Multicomponent Saline Solutions. Solid State Phenomena. 2017. Vol. 265, p. 845-850. DOI: 10.4028/www.scientific.net/SSP.265.845
- Yurkevich N.V., Saeva O.P., Karin Y.G. Geochemical anomalies in two sulfide-bearing waste disposal areas: Fe, Cu, Zn, Cd, Pb, and As in contaminated waters and snow, Kemerovo and Chelyabinsk regions, Russia. Toxicological & Environmental Chemistry. 2015. Vol. 97. Iss. 1, p. 76-89. DOI: 10.1080/02772248.2015.1041955
- Maleva M.G., Borisova G.G., Shiryaev G.I. et al. Adaptive potential of Typha latifolia under extreme technogenic pollution. AIP Conference Proceedings. 2019. Vol. 2063. N 030013. DOI: 10.1063/1.5087321
- Abrosimova N., Bortnikova S. The Role of Anionogenic Elements (As, Sb, Mo, Se, S, P, N, Cl, F, C) in the Formation of Technogenic Geochemical Anomalies. IOP Conference Series: Earth and Environmental Science. 2017. Vol. 95. Iss. 4. N 042024. DOI: 10.1088/1755-1315/95/4/042024
- Zhbankov G.O., Denisov S.E., Ulrikh D.V. Efficiency analysis and justification of waste water purification methods for the water protection system against pollution. IOP Conference Series: Materials Science and Engineering. 2018. Vol. 451. N 012236. DOI: 10.1088/1757-899X/451/1/012236
- Ulrikh D.V., Bryukhov M.N., Timofeeva S.S. Rationale for technique of operational sampling apatite-staffelite ores of kovdorsky deposit. Mining Informational and Analytical Bulletin. Vol. 4, p. 139-143 (in Russian).
- Pashkevich M.A., Kharko P.A. The use of a composite mix to remove metals from acidic drainage waters at tailings facilities. Obogashchenie rud. 2022. N 4, p. 40-47 (in Russian). DOI: 10.17580/or.2022.04.07
- Minkina T.M., Linnik V.G., Nevidomskaya D.G. et al. Heavy Metals Compounds in Soils in the Zone of Karabash Copper Smelter. Current Problems of Status and Evolution of Taxons of the Biosphere. Moscow: Vernadsky Institute of Geochemistry and Analytical Chemistry of the Russian Academу of Sciences (GEOKHI RAS), 2017. Vol. 26, p. 294-300 (in Russian).
- Ryzhova L.P., Nosova E.V. To the Question of the Effectiveness of Technogenous ore Mineral Deposits Mining. Mining Informational and Analytical Bulletin. N 8, p. 49-55.
- Altushkin I.A., Levin V.V., Sizikov A.V., Korol Y.A. Experience of development of porphyry copper type deposits in the Urals. Journal of Mining Institute. Vol. 228, p. 641-648. DOI: 10.25515/PMI.2017.6.641