Features of olivine crystallization in ordinary chondrites (Saratov meteorite): geochemistry of trace and rare earth elements
Abstract
The paper discusses the geochemistry of major (EPMA) and trace (SIMS) elements in olivine of porphyritic, nonporphyritic chondrules, and the matrix of equilibrated ordinary chondrite Saratov (L4). Olivine corresponds to forsterite and is rather heterogeneous (Fo 73-77). No differences in the content of the major elements in the olivine of the chondrule and the matrix of the meteorite were found. However, the content of major and trace elements in olivine within chondrules varies considerably; high values found in olivine from barred chondrules. Olivine from porphyritic chondrules and the matrix of the Saratov meteorite have similar concentrations of trace elements. High concentrations of refractory (Zr, Y, Al) and moderately volatile (Sr and Ba) trace elements in barred olivine chondrule indicate the chondrule melt formation due to the melting of precursor minerals and its rapid cooling in the protoplanetary disk, which is consistent with the experimental data. The olivine of the chondrules center of the Saratov meteorite differs from the olivine of the chondrules rims and meteorite matrix by the increased values of the Yb/La ratio. No relict grains and magnesian cores of olivine were found in meteorite chondrules. Individual grains in the chondrules are distinguished by their enrichment in trace elements relative to the rest of the olivine grains in the chondrule.
Introduction
Ordinary chondrites are the most common type of meteorite and are an agglomerate of chondrules and single grains of silicate minerals. Chondrules – submillimeter silicate spherules, composed of olivine, pyroxene, and mesostasis (glass), are among the most ancient formations of the solar system [1]. The material of ordinary chondrites did not experience core-mantle differentiation and segregation, which allowed to preserve the primary properties reflecting the physical and chemical conditions of the protoplanetary disk at the early stage of the solar system development. However, most common chondrites show signs of thermal metamorphism at varying degrees of intensity. The accretion of planetesimals resulted in the formation of chondrite parent bodies and the accumulation of short-lived 26Al and 50Fe isotopes on them, which led to the formation of an internal heating source and the action of secondary processes (thermal metamorphism, fluid activity) [2].
Thermal metamorphism led to the homogenization of the compositions of the main silicate minerals (olivine, pyroxene), recrystallization of chondrule glass into plagioclase, coarsening of grains of kamacite-taenite, troilite, and chromite, and the appearance of new mineral phases (apatite, merrillite, Ca-pyroxene) [3]. Depending on the distance from the heating source, chondrites are subdivided into unequilibrated (petrologic type 3) and equilibrated (4, 5, and 6), where the degree of mineral equilibrium increases with increasing petrologic type number (p.t.) and signs of metamorphism are more pronounced.
The study of the chemical and isotopic composition of chondrules makes it possible to reconstruct the conditions of formation and peculiarities of the evolution of primary substance in the protoplanetary disk. Meteorites are the main source of information about the primary extraterrestrial material. One of the large meteorites, weighing about 200 kg, Saratov, fell in a stone rain on the villages of Mikhailovskoye, Shakhovskoye, Donguz, and Belaya Gora in the Saratov region on6 October 1918 [4]. The meteorite belongs to the low-iron group L of equilibrated ordinary chondrites (EOC) 4 p.t., it is characterized by the high porosity of the material [5] and the presence of large quantities of the Q phase, which concentrate the noble gases [6, 7]. It is characterized by a low stage of impact metamorphism S2/S3 [8] and a low degree of earth weathering W0.
To date, studies of EOC silicate minerals have been limited to the composition of major or minor elements, which has led to difficulties in assessing the petrological type of grains delivered from the Itokawa asteroid [9]. Trace and rare earth elements are widely used in assessing geochemical conditions, which makes it possible to use them to study the conditions for the formation of minerals of various genesis, such as zircon [10], tourmaline [11], beryl [12], pyroxene [13], and also glasses [14]. Trace elements in olivine and pyroxene are slightly affected by diffusion under the conditions of thermal metamorphism [15, 16] observed in the parent bodies of chondrites, which allows them to be used in the study of EOC minerals. A study of the composition of refractory elements in olivine, pyroxene, and glass chondrules of unequilibrated ordinary chondrites (UOC) has revealed different types of crystallization of porphyritic chondrules and allowed us to determine their relative cooling rates [17-19]. The composition of moderately volatile elements generally reflects the interaction of the chondrule with the surrounding gas in the protoplanetary cloud [20, 21]. The first results of a study of the compositions of EOC silicate minerals have established heterogeneity in the content of trace elements [22, 23] and the ratio of oxygen isotopes [24] in EOC olivine and pyroxene.
Isolated grains of refractory olivine significantly enriched with MgO and differing in oxygen isotope composition from chondrule olivine are sometimes observed in porphyritic chondrules [25, 26] of UOC, as well as more magnesian cores of olivine grains framed with rims of less magnesian forsterite [27]. The presence of relict olivine grains in porphyritic chondrules may indicate their formation as a result of the melting of precursor minerals. The precursor material considered is refractory inclusions (CAI and AOA) [28], fine-grained chondrite matrix grains, chondrules, and fragments of chondrules from previous generations. It also includes planetesimals [29], H2O ice [30], relict olivine, and dust clusters [31].
Recent studies of olivine porphyritic chondrules from the Orlovka meteorite have shown that they have not experienced equilibration concerning trace elements during thermal metamorphism, and the detected geochemical difference between chondrules confirmed the hypothesis of melting of mineral precursors of chondrules in the protoplanetary disk [32].
The study of the trace element composition of olivine chondrules from the EOC of the Saratov meteorite allowed us to establish the influence of thermal metamorphism on the trace element content and to reveal differences in the conditions of chondrules formation of different structures.
Analytical methods
The Saratov chondrite sample (L4) was provided by the head of the IPGG RAS laboratory, Prof. L.K.Levskii.
The chemical composition of the minerals at the major element level was determined by EPMA at IPGG RAS on a Jeol JXA-8230 microprobe analyzer with four WDS. The meteorite substance was placed in a standard epoxy resin washer, which was coated with carbon after polishing. Spot measurements of mineral composition were carried out with an accelerating voltage of 20 kV and a current of 20 nA for olivine. The focused beam diameter was 3 μm. Natural minerals, pure oxides, and metals have been used as standards. The ZAF algorithm was used to correct the matrix effect. The Kα1 lines were measured for all elements.
The content of trace and rare earth elements (REE) in the minerals was determined by secondary ion mass spectrometry (SIMS) using the Cameca IMS-4f ion microprobe at the Valiev IPT RAS, Yaroslavl Branch using the methodology described in [33]. The preparation was coated with gold before measurement. Cameca IMS-4f ion microprobe imaging conditions: the primary beam of ions 16O2¯, beam diameter ~ 20 µm; ion current 5-7 nA; primary beam accelerating voltage 15 keV. The measurement error did not exceed 10 % for impurities with concentrations >1 ppm and 20 % for concentrations < 1 ppm. The trace element composition of the rock-forming minerals was determined as close as possible to the major element analysis points of the EPMA measurements. The REE spectra in the minerals were CI-normalized [34].
Results and discussion
The petrographic study of the Saratov meteorite made it possible to establish troilite, chromite, and silicate chondrules of porphyritic, barred, radial, and cryptocrystalline structures. The main body of the meteorite is composed of silicate chondrules of various sizes and their fragments. Silicate chondrules can be visually divided into three size fractions: the largest – 1-2 mm in diameter (are represented by porphyritic, rarely by barred structures); medium-sized – 0.5-0.3 mm; and small-sized – less than 0.2 mm. Chondrules of medium and small sizes are represented by all of the above structures. The silicate chondrules of the Saratov meteorite have virtually no metal and silicate rims.
The variety of chondrules structures, subdivided into porphyritic and nonporphyritic (barred, radial, cryptocrystalline, etc.), reflects the differences in melt cooling conditions. It has been experimentally established that the formation of porphyritic chondrules occurs from a slowly cooling melt (1-500 °C/h) heated below the liquidus temperature (1400-1700 °C) and retaining a large number of relic grains. Crystallization of barred chondrules requires heating the melt slightly above the liquidus temperature and rapid cooling (500-3000 °C/h). Radial chondrules are characterized by high melt temperatures and almost instant cooling (1000-3000 °C/h) [35].
The meteorite matrix is mainly composed of small chondrules, chondrules fragments, or individual large grains of olivine. Occasionally small metallic formations are also found. The matrix is highly fragmented and characterized by high porosity. The presence of the matrix, significant differ-rences in its isotopic and chemical composition [36], as well as the presence of presolar grains and primary condensates (corundum, hibonite, spinel, and perovskite) indicate the existence of regions of the protoplanetary disk that did not participate in the formation of chondrules.
Olivine in the Saratov meteorite is most commonly observed in euhedral or subhedral grains that compose the porphyritic chondrules of the meteorite. Depending on the chondrule, grain sizes can vary from 500 to 100 µm. In barred chondrules, olivine is observed as elongated skeletal crystals, sometimes intergrown and forming beams oriented most often in parallel, but sometimes intersecting each other. Individual subhedral olivine grains with an average size of 200-300 µm in elongation are observed in the meteorite matrix. The composition of the olivine corresponds to forsterite and is quite heterogeneous (Fo 73-77), but no differences between the chondrite and matrix olivine in the composition of the major elements have been established. In porphyritic chondrules, at the boundary with anorthoclase, olivine grains are overgrown with small (20-30 µm) pigeonite and augite grains.
The distribution of trace elements in the silicate minerals of the Saratov meteorite was studied in two olivine-pyroxene porphyritic (3POP-2, 3POP-4) and one olivine-pyroxene barred chondrules (3BOP-1) (Fig.1).
The 3POP-2 chondrule is oval, does not exceed 2 mm in elongation, and is composed of large (500-300 µm) euhedral grains of olivine and small subhedral grains of enstatite, the interstices between which are filled with poorly crystallized mesostasis of oligoclase-labrador composition.
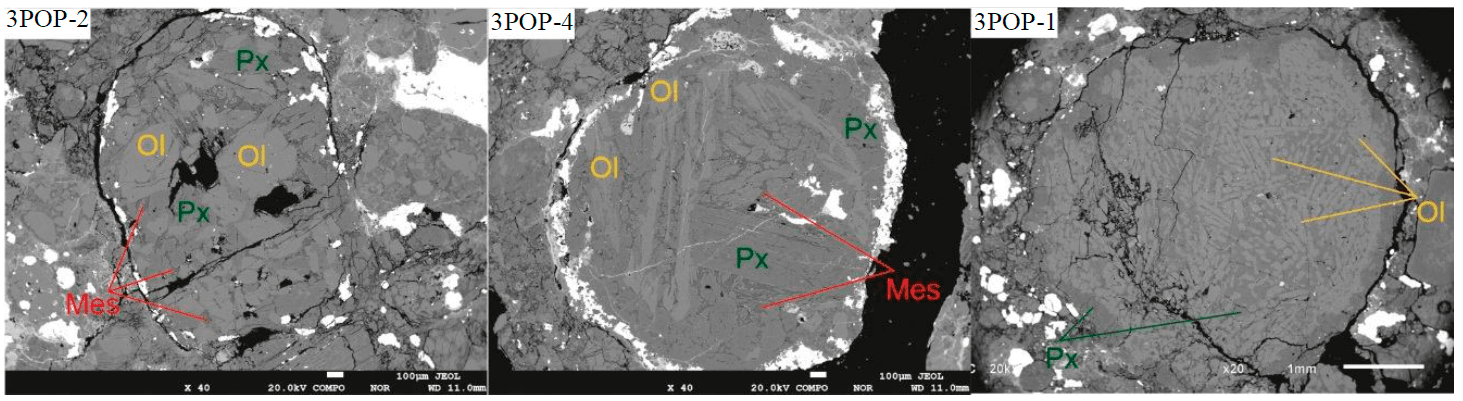
Fig.1. BSE images of the olivine-pyroxene porphyritic chondrules 3POP-2 and 3POP-4 and olivine-pyroxene barred chondrule 3BOP-1 of the Saratov meteorite Ol – olivine, Px – pyroxene, Mes – mesostasis
Chondrule olivine is rather heterogeneous in terms of major and trace element content, but no geochemical patterns are observed between the content of elements in the olivine of the chondrule center and rim. However, anhedral olivine grains of small size (100 µm) located in the rim of the chondrule is significantly richer in trace elements than large, well-cut ones (Table 1). The distribution spectra of trace elements in chondrule olivine are practically not differentiated (Fig.2, a). The content of trace elements is an order of magnitude lower than the chondrite values. In general, there is an enrichment of LREE relative to HREE in 3POP-2 chondrule olivine.
Table 1
Composition of olivine from 3POP-2 chondrule of the Saratov meteorite
Component |
3POP-2 |
|||||
Center |
Center |
Rim |
Rim |
Matrix |
Matrix |
|
SiO2 |
38.77 |
38.84 |
38.77 |
37.92 |
38.78 |
38.74 |
Al2O3 |
0.01 |
0.01 |
bdl |
bdl |
0.01 |
|
MgO |
39.11 |
38.91 |
39.05 |
37.54 |
39.11 |
39.11 |
TiO2 |
bdl |
0.01 |
bdl |
bdl |
bdl |
bdl |
CaO |
0.01 |
0.02 |
0.01 |
|
0.02 |
0.05 |
FeO |
21.71 |
22.02 |
21.85 |
23.95 |
21.66 |
21.97 |
MnO |
0.50 |
0.47 |
0.48 |
0.59 |
0.45 |
0.48 |
Cr2O3 |
bdl |
0.01 |
0.02 |
bdl |
0.01 |
bdl |
NiO |
0.01 |
bdl |
bdl |
bdl |
0.02 |
0.01 |
Total amount |
100.12 |
100.30 |
100.18 |
100.00 |
100.05 |
100.36 |
Zr |
0.08 |
0.07 |
0.07 |
0.73 |
0.06 |
0.13 |
Hf |
0.05 |
0.07 |
0.03 |
|||
Y |
4409 |
4352 |
4128 |
4153 |
4142 |
4755 |
Al |
0.01 |
0.01 |
0.01 |
0.05 |
0.01 |
0.01 |
Ti |
90.0 |
75.5 |
72.2 |
1146 |
55.8 |
850 |
Nb |
98.5 |
106 |
77.2 |
108 |
106 |
121 |
Ca |
0.02 |
0.01 |
0.01 |
0.08 |
0.01 |
0.02 |
La |
0.01 |
0.01 |
0.01 |
0.02 |
0.01 |
0.01 |
Ce |
0.02 |
0.01 |
0.03 |
0.04 |
0.02 |
0.02 |
Pr |
0.01 |
0.01 |
0.01 |
bdl |
||
Nd |
0.04 |
0.06 |
0.06 |
0.03 |
||
Sm |
|
0.03 |
||||
Eu |
0.01 |
0.01 |
0.01 |
0.02 |
0.01 |
0.01 |
Gd |
0.02 |
0.04 |
0.01 |
0.03 |
||
Dy |
|
0.02 |
|
|||
Er |
|
0.02 |
0.02 |
|
||
Yb |
0.04 |
0.01 |
0.02 |
0.02 |
||
Lu |
0.01 |
0.01 |
0.01 |
bdl |
||
Sr |
0.14 |
0.11 |
0.14 |
3.02 |
0.12 |
0.73 |
Ba |
0.23 |
0.08 |
0.16 |
1.30 |
0.07 |
1.17 |
V |
14.4 |
13.1 |
22.0 |
27.8 |
12.4 |
14.7 |
Ni |
24.8 |
22.8 |
34.4 |
33.2 |
26.4 |
354 |
Cr |
358 |
371 |
847 |
1464 |
362 |
404 |
Rb |
1.16 |
1.16 |
1.24 |
1.77 |
0.95 |
2.15 |
Note. Empty cell – no element detected; bdl – element content is below the detection limit.
Chondrule 3POP-4 is characterized by its rounded shape, the total absence of pores and cracks, and size not exceeding 2 mm (Fig. 1). Chondrule consists of large (1-1.5 mm in elongation) hypidiomorphic elongated phenocrysts of olivine and smaller (200-300 µm) grains of low-Ca pyroxene, interstices between which are filled with mesostasis with a composition close to anorthoclase. A thin, intermittent metallic rim runs along the border of the chondrule.
Olivine in the 3POP-4 chondrule is represented by large, strongly elongated grains, and its composition relates to forsterite (Fo 75). There is no difference between chondrule and matrix olivine in the composition of major and minor elements (Table 2) except for slight enrichment of olivine from chondrule center in Ce and Cr, and matrix olivine in Ti and Ni (Fig.2, b).
The trace elements spectra are subhorizontal and practically undifferentiated. The chondrule and matrix olivine is depleted in rare elements compared to the chondritic values. Still, it has a high content of trace earth elements compared to the olivine of porphyritic chondrules of UOC. In the olivine of the chondrule rim and the matrix of the Saratov meteorite, there is a predominance of HREE over LREE. However, the olivine of the chondrule center is enriched in LREE compared to other olivines of the chondrule, which is reflected in the reverse distribution of REE and the predominance of LREE over HREE.
For olivine from the chondrule rim and the meteorite matrix, the Ca/Al ratio is close to the chondrite value (with the direct correlation of La and Yb preserved), but for olivine from the chondrule center it differs greatly.
Barred chondrule 3BOP-1 is large (4 mm in diameter), rounded, highly porous, and fissured (see Fig.1). A chondrule is characterized by a silicate pyroxene rim composed of several lamellae groups. The gaps between the lamellae are filled with anhedral segregations of low-Ca pyroxene and mesostasis. High-Ca pyroxene phases are often present at the boundary of pyroxene with mesostasis.
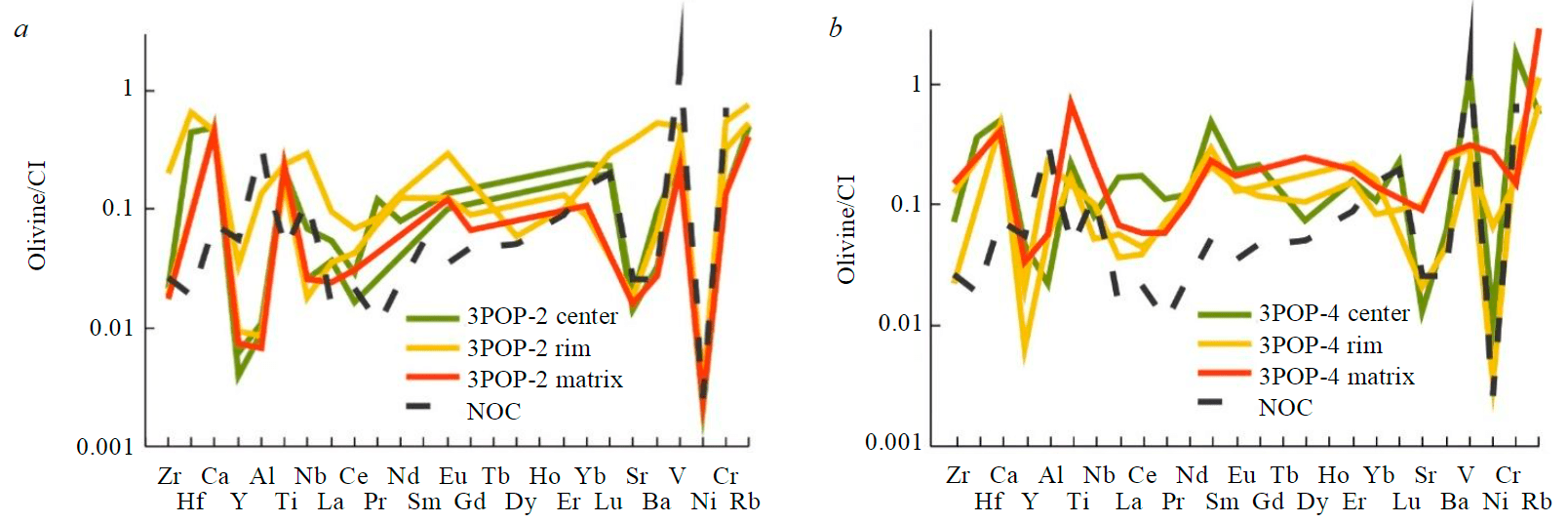
Fig.2. CI-normalized spider diagrams for trace elements in olivine from 3POP-2 (a) and 3POP-4 (b) chondrules
Table 2
Composition of olivine from 3POP-4 and 3BOP-1 chondrules of the Saratov meteorite
Component |
3POP-4 |
3BOP-1 |
|||||||
center |
Rim |
Rim |
Matrix |
center |
center |
Rim |
Matrix |
||
SiO2 |
38.55 |
38.57 |
38.75 |
38.69 |
38.92 |
38.76 |
38.80 |
38.96 |
|
Al2O3 |
bdl |
0.03 |
0.01 |
0.03 |
bdl |
0.03 |
bdl |
bdl |
|
MgO |
38.89 |
38.87 |
39.06 |
38.69 |
39.45 |
39.05 |
39.29 |
39.19 |
|
TiO2 |
0.01 |
bdl |
bdl |
0.11 |
0.04 |
0.03 |
bdl |
0.03 |
|
CaO |
0.01 |
0.02 |
0.01 |
0.02 |
0.01 |
0.04 |
0.03 |
0.02 |
|
FeO |
21.10 |
21.41 |
21.57 |
21.87 |
21.85 |
21.96 |
22.03 |
21.76 |
|
MnO |
0.51 |
0.42 |
0.47 |
0.45 |
0.48 |
0.49 |
0.47 |
0.46 |
|
Cr2O3 |
0.01 |
0.03 |
0.02 |
0.05 |
0.02 |
0.02 |
0.04 |
0.01 |
|
NiO |
0.01 |
bdl |
0.01 |
0.03 |
bdl |
bdl |
bdl |
bdl |
|
Total amount |
99.09 |
99.35 |
99.91 |
99.94 |
100.77 |
100.38 |
100.64 |
100.43 |
|
Zr |
0.26 |
0.47 |
0.08 |
0.56 |
0.39 |
1.24 |
0.93 |
0.15 |
|
Hf |
0.04 |
0.04 |
0.04 |
0.03 |
0.03 |
||||
Y |
4695 |
4242 |
4178 |
3815 |
4285 |
4142 |
4641 |
4088 |
|
Al |
0.07 |
0.03 |
0.01 |
0.05 |
0.11 |
0.14 |
0.31 |
0.01 |
|
Ti |
187 |
1960 |
453 |
478 |
595 |
375 |
179 |
74.0 |
|
Nb |
101 |
66.1 |
78.2 |
322 |
281 |
273 |
200 |
166 |
|
Ca |
0.02 |
0.03 |
0.01 |
0.06 |
0.02 |
0.07 |
0.05 |
bdl |
|
La |
0.04 |
0.01 |
0.01 |
0.02 |
0.03 |
0.02 |
0.02 |
0.01 |
|
Ce |
0.11 |
0.02 |
0.03 |
0.04 |
0.14 |
0.02 |
0.09 |
0.03 |
|
Pr |
0.01 |
0.01 |
0.01 |
0.01 |
0.01 |
0.01 |
0.01 |
|
|
Nd |
0.06 |
0.05 |
0.05 |
0.03 |
0.11 |
0.03 |
|||
Sm |
0.08 |
0.03 |
0.05 |
0.04 |
0.04 |
0.03 |
0.04 |
|
|
Eu |
0.01 |
0.01 |
0.01 |
0.01 |
0.01 |
bdl |
0.01 |
|
|
Gd |
0.05 |
0.03 |
0.03 |
bdl |
0.03 |
0.06 |
0.02 |
||
Dy |
0.02 |
0.03 |
0.07 |
0.04 |
0.02 |
0.07 |
0.01 |
||
Er |
0.03 |
0.03 |
0.04 |
0.03 |
0.02 |
0.05 |
0.03 |
|
|
Yb |
0.02 |
0.01 |
0.03 |
0.02 |
0.08 |
0.06 |
0.05 |
0.01 |
|
Lu |
0.01 |
0.01 |
0.01 |
0.01 |
bdl |
||||
Sr |
0.10 |
0.78 |
0.16 |
0.73 |
0.24 |
0.75 |
0.15 |
0.10 |
|
Ba |
0.15 |
0.60 |
0.11 |
0.66 |
0.89 |
0.18 |
0.78 |
0.13 |
|
V |
71.2 |
16.8 |
13.3 |
17.9 |
27.3 |
21.3 |
44.0 |
14.1 |
|
Ni |
119 |
36.1 |
739 |
3063 |
29.8 |
26.4 |
29.3 |
29.4 |
|
Cr |
4872 |
871 |
432 |
398 |
554 |
786 |
1838 |
383 |
|
Rb |
1.36 |
2.74 |
1.65 |
7.26 |
1.46 |
0.94 |
1.04 |
1.82 |
Note. See notes in Table 1.
Olivine in the 3BOP-1 chondrule center is represented by elongated thin grains that become smaller and more isometric near the chondrule rim. Olivine lamellae can reach 1 mm in elongation, while anhedral grains are usually characterized by small size (200 µm). According to the composition of the main and trace elements, chondrule olivine is homogeneous and corresponds to forsterite (Fo 75) (Table 2). At the same time, the matrix olivine is significantly depleted in refractory incompatible elements (Zr, Y, and LREE) compared to chondrule olivine. Olivine of the chondrule center is enriched in Al, Ce, and Sr.
The trace elements spectra in olivine are slightly differentiated (Fig.3, a). The content of trace elements is somewhat depleted relative to chondrite values and generally corresponds to the level of rare elements in olivine from UOC. There is also a slight predominance of HREE over LREE, while the chondrite Ca/Al ratio in the olivine of the 3BOP-1 chondrule is disturbed. Olivine from the matrix of the meteorite is generally depleted in refractory elements (Zr, Y, and Al) relative to olivine from the chondrule.
Conclusions
Chondrules 3POP-2, 3POP-4, and 3BOP-1 of the Saratov meteorite differ in the composition of trace elements in olivine. Despite the strong heterogeneity of trace element contents in chondrule olivine, there are no significant differences between olivine from porphyritic chondrule 3POP-2 and 3POP-4. Olivine from the barred chondrule 3BOP-1 is characterized by a high Yb/Ti ratio and enrichment in HREE and Y compared to olivine from porphyritic chondrules (Fig.3, b).
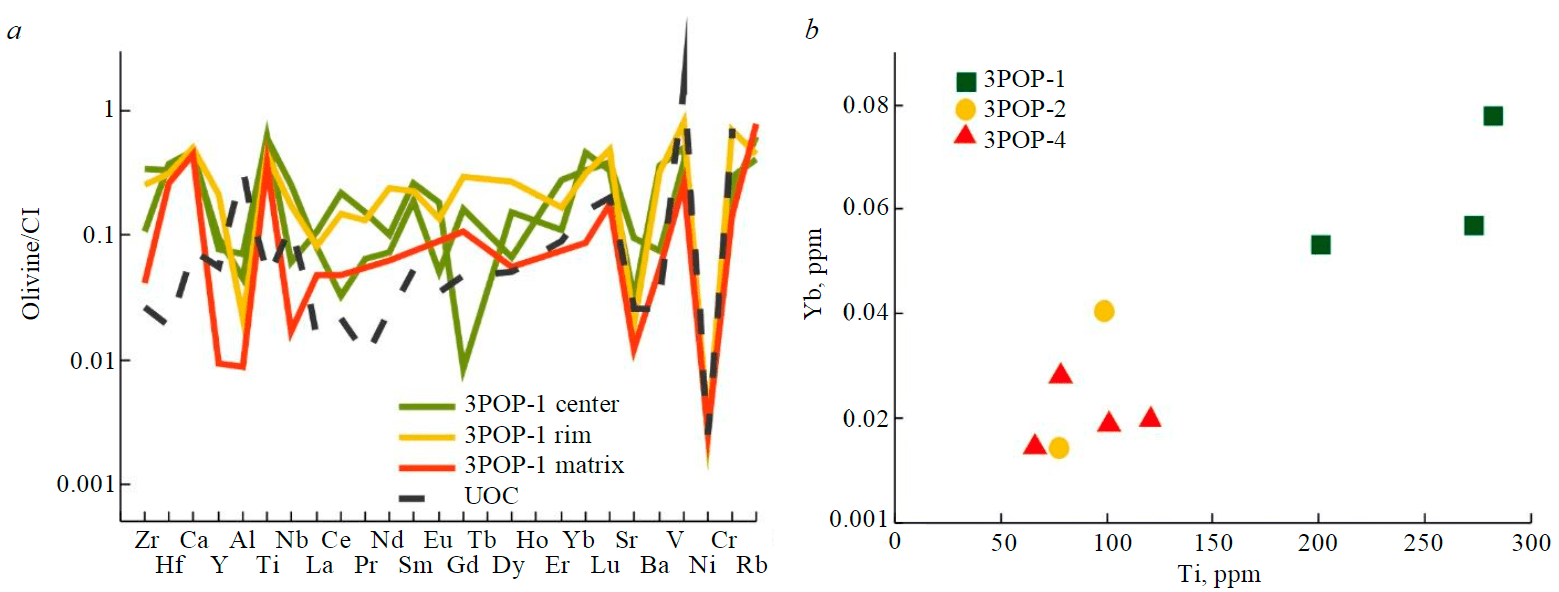
Fig.3. Spider diagrams for CI chondrite- normalized trace elements (a) and the Ti and Yb ratio in olivine of the 3BOP-1 chondrule (b)
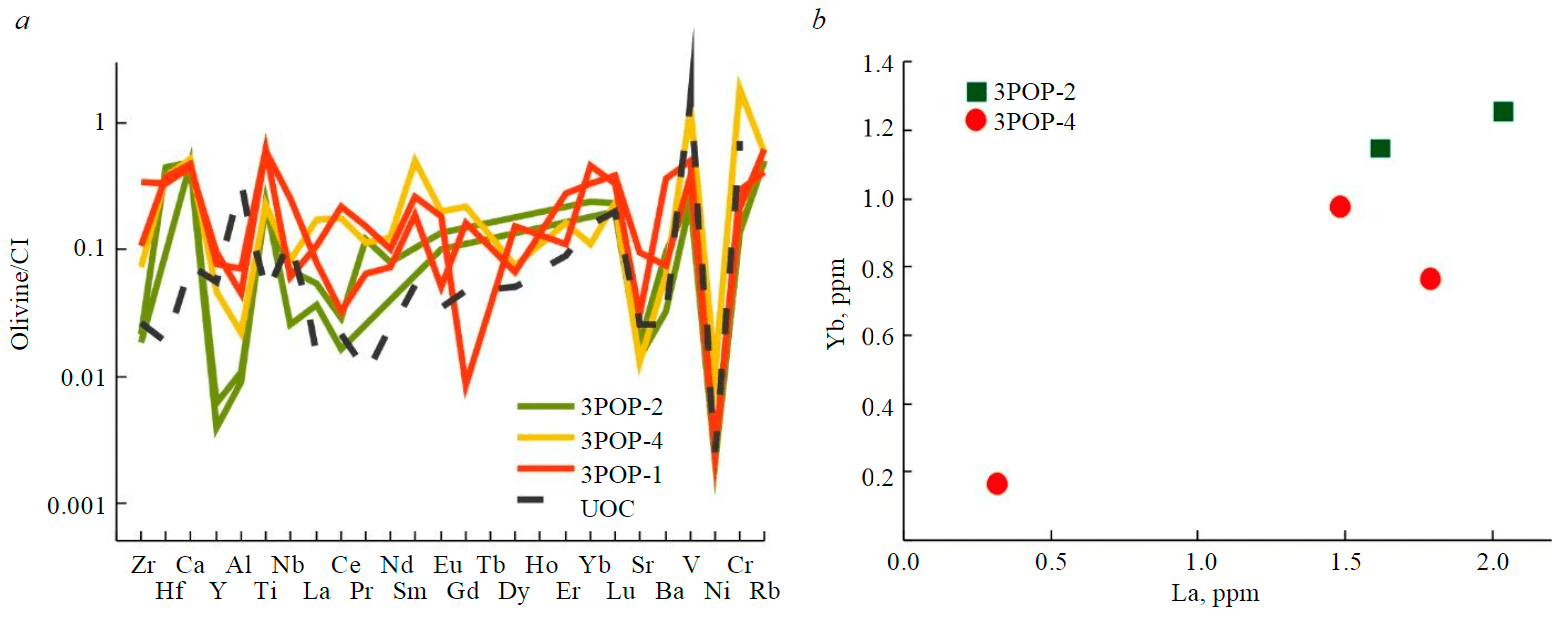
Fig.4. Spider diagrams for trace elements normalized to CI chondrite (a) and the La and Yb ratio (b) in olivine chondrules of the Saratov meteorite
The trace elements spectra in olivine from the barred chondrule are characterized by a strong enrichment of refractory elements (Zr, Y, and Al), as well as increased concentrations of moderately volatile incompatible elements (Sr and Ba) (Fig.4, a). In general, the olivine of all three chondrules is depleted in trace elements relative to chondrite values, while the concentrations of trace elements vary significantly within the chondrule.
High contents of trace elements in olivine are characteristic of chondrules formed at very fast cooling rates (more than 1000 °C/h) [37]. When a chondrule is formed by condensation, an unfractionated Ca/Al ratio, a positive correlation of La and Yb, and an unfractionated content of refractory rare lithophile elements should be observed in it [38].
The high concentration of simultaneously incompatible refractory and moderately volatile elements in olivine from the barred chondrule cannot be a sign of direct condensation in the protoplanetary disk and rather agrees with the hypothesis of the melting of chondrule precursor minerals. At the same time, a direct correlation between Yb and La is characteristic of olivine only from porphyritic chondrules (Fig.4, b).
The olivine of the chondrules center of the Saratov meteorite differs from the olivine of the chondrules rim of the meteorite matrix by increased values of the Yb/La ratio (Fig.5, a). However, there are no differences between the olivine of the center, the rim of the chondrules, and the meteorite matrix on the spectrum of the rare elements (Fig.5, b).
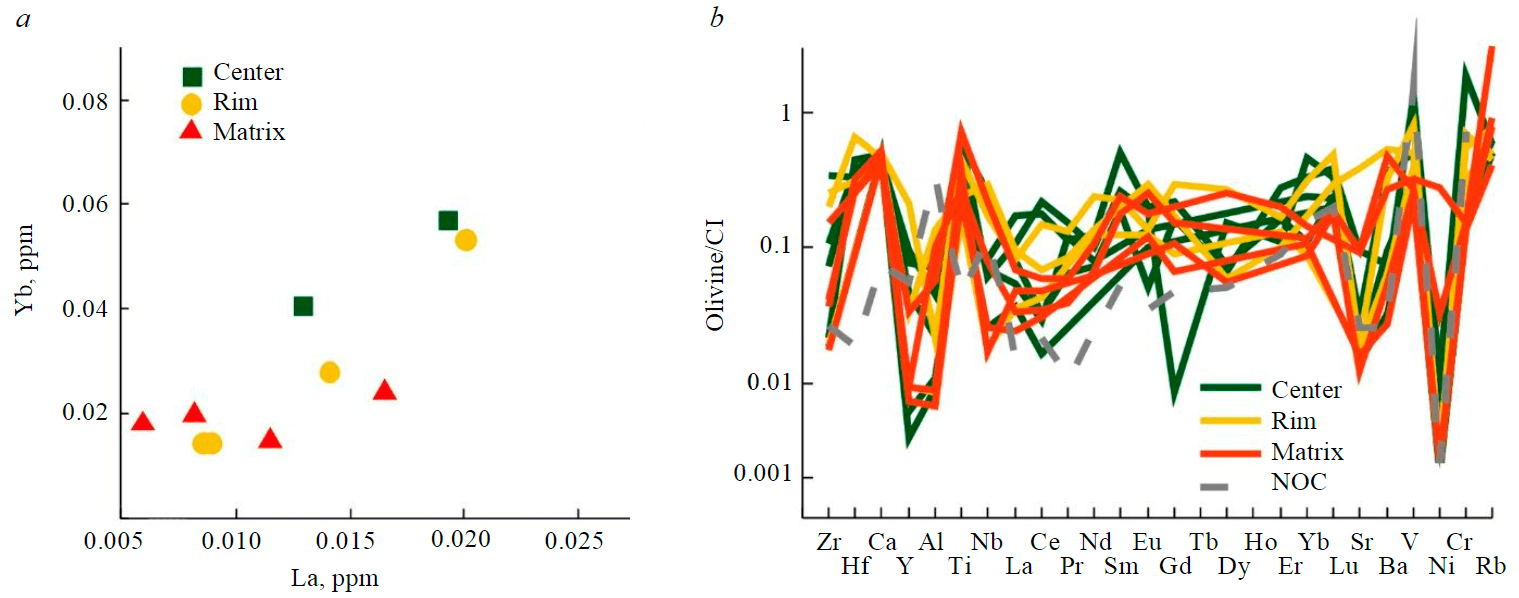
Fig. 5. La and Yb ratio (a) and spider diagrams for CI chondrite-normalized trace elements (b) in olivine of the Saratov meteorite
Thus, in the olivine of the chondrules and matrix of the Saratov meteorite, homogenization of trace elements under the influence of thermal metamorphism is not observed. Differences in the content of trace elements in olivine from porphyritic and barred chondrules reflect the high rate of cooling of the barred chondrule melt and confirm the hypothesis that the chondrule melt appeared as a result of the melting of precursor minerals.
References
- Pape J., Mezger K., Bouvier A.S., Baumgartner L.P. Time and duration of chondrule formation: Constraints from 26Al-26Mg ages of individual chondrules. Geochimica et Cosmochimica Acta. 2019. Vol. 244, p. 416-436. DOI: 10.1016/j.gca.2018.10.017
- Tomkins A.G., Weinberg R.F., Schaefer B.F., Langendam A. Disequilibrium melting and melt migration driven by impacts: Implications for rapid planetesimal core formation. Geochimica et Cosmochimica Acta. 2013. Vol. 100, p. 41-59. DOI: 10.1016/j.gca.2012.09.044
- Jones R.H., McCubbin F.M., Dreeland L. et al. Phosphate minerals in LL chondrites: A record of the action of fluids during metamorphism on ordinary chondrite parent bodies. Geochimica et Cosmochimica Acta. 2014. Vol. 132, p. 120-140. DOI: 10.1016/j.gca.2014.01.027
- Obolonskaya E.V., Popova E.E. The collection of meteorites from the Mining Museum of the Saint Petersburg Mining University. Meteorit Chelyabinsk – god na Zemle: Materialy Vserossiyskoy nauchnoy konferentsii, 14-15 fevralya 2014, Chelyabinsk. Chelya-binsk: Chelyabinskiy gosudarstvennyy kraevedcheskiy muzey, 2014, p. 355-363 (in Russian).
- Lewis J.A., Jones R.H., Garcea S.C. Chondrule porosity in the L4 chondrite Saratov: Dissolution, chemical transport, and fluid flow. Geochimica et Cosmochimica Acta. 2018. Vol. 240, p. 293-313. DOI: 10.1016/j.gca.2018.08.002
- Fisenko A.V., Verchovsky A.B., Shiryaev A.A. et al. On the carrier phase of the “planetary” noble gases: TEM, Raman, and stepped combustion data for acid-resistant residues from the Saratov (L4) meteorite. Meteoritics & Planetary Science. 2018. Vol. 53. N 11, p. 2343-2356. DOI: 10.1111/maps.13130
- Matsuda J.-i., Morishita K., Nara M., Amari S. Noble gases in oxidized residue prepared from the Saratov L4 chondrite and Raman spectroscopic study of residues to characterize phase Q. Meteoritics & Planetary Science. 2016. Vol. 51, p. 70-79. DOI: 10.1111/maps.12587
- Pesonen L.J., Terho M., Kukkonen I.T. Physical properties of 368 meteorites: Implications for meteorite magnetism and planetary geophysics. Proceedings of the NIPR Symposium on Antarctic Meteorites. 1993. N 6, p. 401-416.
- Nakamura T., Noguchi T., Tanaka M. et al. Itokawa Dust Particles: A Direct Link Between S-Type Asteroids and Ordinary Chondrites. Science. 2011. Vol. 333, p. 1113-1116. DOI: 10.1126/science.1207758
- Skublov S.G., Rumyantseva N.A., Li Q., Vanshtein B.G. et al. Zircon xenocrysts from the Shaka Ridge record ancient continental crust: New U-Pb geochronological and oxygen isotopic data. Journal of Earth Science. 2022. Vol. 33(1), p. 5-16. DOI: 10.1007/s12583-021-1422-2
- Alekseev V.I., Marin Yu.B. Tourmaline as an Indicator of Tin Occurrencesof Cassiterite-quartz and Cassiterite-silicate Formations (a Case Study of the Verkhneurmiysky Ore luster, Far East). Journal of Mining Institute. 2019. Vol. 235, p. 3-9. DOI: 10.31897/PMI.2019.1.3
- Gavrilchik A.K., Skublov S.G., Kotova E.L. Trace Element Composition of Beryl From the Sherlovaya Gora Deposit, South-Eastern Transbaikalia, Russia. Proceedings of the Russian Mineralogical Society. 2021. Vol. 150. N 2, p. 69-82. DOI: 10.31857/S0869605521020052
- Berezin A.V., Salimgaraeva L.I., Skublov S.G. Evolution of the minerals composition during eclogitic metamorphism in the Belomorian mobile belt (on the example of Vichennaya Luda Island). Petrologiya. 2020. Vol. 28, p. 85-107 (in Russian). DOI: 10.31857/S086959032001001X
- Ashikhmin D.S., Skublov S.G. Composition Heterogeneity of Xenoliths of Mantle Peridotites from Alkaline Basalts of the Sverre Volcano, the Svalbard Archipelago. Journal of Mining Institute. 2019. Vol. 239, p. 483-491. DOI: 10.31897/PMI.2019.5.483
- Chakraborty S. Diffusion Coefficients in Olivine, Wadsleyite and Ringwoodite. Reviews in Mineralogy and Geochemistry. 2010. Vol. 72. N 1, p. 603-639. DOI: 10.2138/rmg.2010.72.13
- Cherniak D.J. REE diffusion in olivine. American Mineralogist. 2010. Vol. 95, p. 362-368. DOI: 10.2138/am.2010.3345
- Jacquet E., Alard O., Gounelle M. Trace element geochemistry of ordinary chondrite chondrules: The type I/type II chondrule dichotomy. Geochimica et Cosmochimica Acta. 2015. Vol. 155, p. 47-67. DOI: 10.1016/j.gca.2015.02.005
- Jacquet E., Alard O., Gounelle M. Chondrule trace element geochemistry at the mineral scale. Meteoritics & Planetary Science. 2012. Vol. 47. N 11, p. 1695-1714. DOI: 10.1111/maps.12005
- Jacquet E., Alard O., Gounelle M. The formation conditions of enstatite chondrites: Insights from trace element geochemistry of olivine-bearing chondrules in Sahara 97096 (EH3). Meteoritics & Planetary Science. 2015. Vol. 50. N 9, p. 1624-1642. DOI: 10.1111/maps.12481
- Varela M.E., Sylvester P., Engler A., Kurat G. Nonporphyritic chondrules from equilibrated Rumuruti and ordinary chondrites: Chemical evidence of secondary processing. Meteoritics & Planetary Science. 2012. Vol. 47. N 10, p. 1537-1557. DOI: 10.1111/j.1945-5100.2012.01417.x
- Varela M.E., Sylvester P., Brandstätter F., Engler A. Nonporphyritic chondrules and chondrule fragments in enstatite chondrites: Insights into their origin and secondary processing. Meteoritics & Planetary Science. 2015. Vol. 50. N 8, p. 1338-1361. DOI: 10.1111/maps.12468
- Sukhanova K.G., Skublov S.G., Galankina O.L. et al. Trace Element Composition of Silicate Minerals in the Chondrules and Matrix of the Buschhof Meteorite. Geochemistry International. 2020. Vol. 58, p. 1321-1330. DOI: 10.1134/S001670292012006X
- Dutta A., Bhattacharya A., Mishra M. et al. Trace elements and REE geochemistry of olivine and enstatite chondrules in ordinary chondrites: Insights into their cosmochemical genesis. 80th Annual Meeting of the Meteoritical Society, May 2017, Santa Fe, New Mexico, USA. 2017. N 6088.
- McDougal D., Nakashima D., Tenner T.J. et al. Intermineral oxygen three-isotope systematics of silicate minerals in equilibrated ordinary chondrites. Meteoritics & Planetary Science. 2017. Vol. 52, p. 2322-2342. DOI: 10.1111/maps.12932
- Jacquet E., Piralla M., Kersaho P., Marrocchi Y. Origin of isolated olivine grains in carbonaceous chondrites. Meteoritics & Planetary Science. 2020. Vol. 56, p. 13-33. DOI: 10.1111/maps.13583
- Marrocchi Y., Euverte R., Villeneuve J. et al. Formation of CV chondrules by recycling of amoeboid olivine aggregate-like precursors. Geochimica et Cosmochimica Acta. 2019. Vol. 247 p. 121-141. DOI: 10.1016/j.gca.2018.12.038
- Ruzicka A., Floss C., Hutson M. Relict olivine grains, chondrule recycling, and implications for the chemical, thermal, and mechanical processing of nebular materials. Geochimica et Cosmochimica Acta. 2008. Vol. 72, p. 5530-5557. DOI: 10.1016/j.gca.2008.08.017
- Jacquet E., Marrocchi Y. Chondrule heritage and thermal histories from trace element and oxygen isotope analyses of chondrules and amoeboid olivine aggregates. Meteoritics & Planetary Science. 2017. Vol. 52. N 12, p. 2672-2694. DOI: 10.1111/maps.129852672
- Libourel G., Krot A.N. Evidence for the presence of planetesimal material among the precursors of magnesianchondrules of nebular origin. Earth and Planetary Science Letters. 2007. Vol. 254, p. 1-8. DOI: 10.1016/j.epsl.2006.11.013
- Tenner T.J., Nakashima D., Ushikubo T. et al. Oxygen isotope ratios of FeO-poor chondrules in CR3 chondrites: Influence of dust enrichment and H2O during chondrule formation. Geochimica et Cosmochimica Acta. 2015. Vol. 148, p. 228-250. DOI: 10.1016/j.gca.2014.09.025
- Bischoff A., Schleiting M., Wieler R., Patzek M. Brecciation among 2280 ordinary chondrites – Constraints on the evolution of their parent bodies. Geochimica et Cosmochimica Acta. 2018. Vol. 238, p. 516-541. DOI: 10.1016/j.gca.2018.07.020
- Sukhanova K.G., Kuznetsov A.B., Skublov S.G. Geochemical features of chondrules of the Orlovka (H5) meteorite as evidence of the melting of precursor minerals. Doklady Akademii nauk. Nauki o Zemle. 2022. Vol. 504. N 1, p. 28-33 (in Russian).
- Portnyagin M., Almeev R., Matveev S., Holtz F. Experimental evidence for rapid water exchange between melt inclusions in olivine and host magma. Earth and Planetary Science Letters. 2008. Vol. 272, p. 541-552. DOI: 10.1016/j.epsl.2008.05.020
- Palme H., Lodders K., Jones A. Solar system abundances of the elements. Treatise on Geochemistry (Second Edition). 2014. Vol. 2, p. 15-36. DOI: 10.1016/B978-0-08-095975-7.00118-2
- Chondrules: Records of Protoplanetary Disk Processes. Eds. S.S. Russell, H.C. Connolly Jr, A.N. Krot. Cambridge University Press, 2018, p. 450.
- Hezel D.C., Palme H. The chemical relationship between chondrules and matrix and the chondrule matrix complementarity. Earth and Planetary Science Letters. 2010. Vol. 294, p. 85-93. DOI: 10.1016/j.epsl.2010.03.008
- Kennedy A.K., Lofgren G.E., Wasserburg G.J. An experimental study of trace element partitioning between olivine, orthopyroxene and melt in chondrules: equilibrium values and kinetic effects. Earth and Planetary Science Letters. 1993. Vol. 115 (1-4), p. 177-195. DOI: 10.1016/0012-821X(93)90221-T
- Engler A., Varela M.E., Kurat G. et al. The origin of non-porphyritic pyroxene chondrules in UOCs: Liquid solar nebula condensates? Icarus. 2007. Vol. 192, p. 248-286. DOI: 10.1016/j.icarus.2007.06.016