U-Pb (SHRIMP-RG) age of zircon from rare-metal (Li, Cs) pegmatites of the Okhmylk deposit of the Kolmozero-Voron’ya greenstone belt (northeast of the Fennoscandian shield)
- 1 — Ph.D. Leading Researcher Institute of Geology Kola Science Center RAS ▪ Orcid
- 2 — Ph.D. Leading Researcher Institute of Geology Komi Science Center of Ural Branch RAS ▪ Orcid
- 3 — Ph.D. Leading Researcher Institute of Geology Kola Science Center RAS ▪ Orcid
- 4 — Ph.D. Leading Researcher Institute of Geology Kola Science Center RAS ▪ Orcid
- 5 — Ph.D. Leading Researcher Institute of Geology Kola Science Center RAS ▪ Orcid
- 6 — Professor of Geological Sciences Stanford University ▪ Orcid
Abstract
The results of isotopic and geochronological study of zircon from rare-metal pegmatites of the Okhmylk deposit are presented. There were no reliable data on the age of lepidolite-spodumene-pollucite pegmatites of this and the other deposits spatially located within the Archean Kolmozero-Voron’ya greenstone belt. The earlier estimates of the pegmatite age indicate a broad time range from 2.7 to 1.8 Ga. Zircon in the studied pegmatites is characterized by inner heterogeneity, where core and rim zones are distinguished. Minor changes are observed in the core zones, they have a spotted structure and contain numerous uranium oxide inclusions. According to X-ray diffraction analysis, zircon crystallinity is preserved completely in these areas. Complete recrystallization with modification of the original U-Pb isotopic system occurred in the zircon rims. New U-Pb (zircon) isotopic and geochronological data of 2607±9 Ma reflect the time of crystallization of pegmatite veins in the Okhmylk deposit. Isotopic data with ages of ~1.7-1.6 Ga indicate later hydrothermal alteration. The obtained results testify to the Neo-Archean age of the formation of the Okhmylk deposit 2.65-2.60 Ga, reflecting the global age of pegmatite formation and associated the world's largest rare-metal pegmatite deposits.
Introduction
Rare-metal pegmatites are an important source of rare metals, including lithium, cesium, tantalum, niobium, beryllium, rubidium, scandium, and REE, and are distributed on all continents in a wide age range, from the Early Precambrian to the Paleozoic [1-5]. A characteristic feature of rare-metal pegmatites is that they are confined to Precambrian greenstone belts, located along ancient deep fault zones. Rare-metal mineralization was found only in those pegmatite veins, which are hosted by mafic rocks. Important tasks in the study of rare-metal pegmatites are to establish their genetic relationship with granites (if any) and to determine the time of rare mineralization formation. Answers to these questions are connected with isotopic and geochronological studies aimed at estimation of the time of formation of both the granites, parental for pegmatites, and the pegmatites themselves. The Kolmozero-Voron’ya Greenstone Belt is confined to the central part of the suture zone separating the Murmansk domain from the Central Kola and Keivy domains. The belt is composed of Late Archean (2.9-2.5 Ga) volcanogenic-sedimentary rocks. It has concentrated deposits of rare-metal pegmatites (Li, Cs with associated Nb, Ta, and Be). In the northwestern part of the belt, there are lithium and cesium Okhmylk, Vasin-Mylk, Oleninskoe, and Polmostundra deposits; in the south-eastern part, the largest spodumene pegmatite deposit Kolmozerskoe is located. The pegmatite fields of several deposits exposed in the northwestern part of the belt are hosted by amphibolite. The Kolmozersky spodumene pegmatites cut an intrusive body of gabbro anorthosite of the Potchemvarek Massif with an age of 2.93 Ga [6]. The age of pegmatites identified within the belt, according to isotopic geochronological determinations with different methods, is estimated in a wide range of 2.7-1.8 Ga [7-10]. Different accessory mineral geochronometers, such as apatite, microlite, cassiterite, minerals of the columbite-tantalite group, garnet, etc., have become increasingly used for isotopic and geochronological studies along with zircon [11-15], and showed multi-stage complex history of the granite pegmatite systems formation. Thus, the pegmatites of the lepidolite-pollucite Vasin-Mylk deposit contain two zircon generations, sharply differing in composition: high-uranium single crystals of the early generation and the bulk of almost uranium-free high-hafnium zircon of the late generation. For late-generation zircon, where uranium content was only 0.2-0.3 ppm, it was not possible to determine U-Pb age [9], so microlite of the early generation was analyzed with U-Pb (ID TIMS) method, and the of age 2.45 Ga was obtained [8]. There is also no generally accepted point of view regarding the genetic affiliation of pegmatites to one or another granitoids, found in the area where the pegmatite veins of the belt are located. The age of granitoids, which are the possible source for rare-metal pegmatite veins in the north-western field, was estimated at ~ 2.5 Ga, and the age of tourmaline-muscovite granites, parental for spodumene pegmatite of the Kolmozersky deposit in the south-eastern part of the belt, was determined as 2.8-2.7 Ga [16, 17].
Geological characteristics of the Okhmylk deposit
Lepidolite-spodumene pegmatites of the Okhmylk deposit occur in amphibolite of the Polmostundra suite of volcanogenic-sedimentary complex of the Kolmozero-Voron’ya greenstone belt, at the contact with staurolite-biotite gneisses and oligoclase gneiss-granites (Fig.1). The pegmatite field occupies about 600 m2, it can be traced for 1.25 km along the strike. The area is characterized by a high content of pegmatite veins of an elongated lenticular shape, approaching elliptical one. The veins cut the host amphibolite, having a northwest strike and a southwest dip at smooth angles, in contrast to the northwest strike and steep northeast dip of the amphibolite. The pegmatite veins contain numerous xenoliths of amphibolite and biotite gneiss. The xenoliths are embodied with a tourmaline crust and a fan of cone-shaped tourmaline crystals perpendicular to their surface. The veins differ in shape, internal structure, and mineral composition, but within the pegmatite field, a general trend can be identified in the change of complex, zoned veins with rich mineralization, developed in the southeast part of the field, with veins of simple structure and composition in the northwest [18]. A well-defined zonal structure is observed both in the cross-sections of the veins and along the strike, zonation is complicated by frequent intermixing of areas of different composition. Spodumene occurs in the central zones, reaching an average of about 10 vol.%. Another feature of the pegmatites is the oriented arrangement of columnar spodumene and tourmaline crystals that grow perpendicular to the casings along with increase of the entire thickness of the veins. Three types of pegmatites with mutual transitions are distinguished at the deposit: muscovite-tourmaline (with black tourmaline); muscovite-spodumene-tourmaline (with black tourmaline); lepidolite-kunzite-tourmaline (with pink tourmaline).
More than 80 minerals have been identified in the pegmatites of the Kolmozero-Voron’ya belt, representing the classes: silicates and their analogs, oxides and hydroxides, salts of oxygen acids (phosphates, carbonates), sulfides and their analogs, halides, and native elements. The mineralogy of the studied granite pegmatites includes:
- Multistage formation of all rock-forming minerals (quartz, microcline, plagioclases, muscovite, and spodumene) and many secondary and accessory minerals (groups of columbite, garnets, tourmaline, triphylite, etc.);
- the presence of a late stage of recrystallization and formation of aggregates of sugary albite in a substantially closed system;
- a poorly studied supergene mineral formation, which is of interest for understanding the migration processes of some elements and their dispersion halos as prospecting criteria [18-20] (the following supergene minerals, previously unknown for these pegmatites, were identified: bermanite, arthurite group, ranciéite, todorokite).
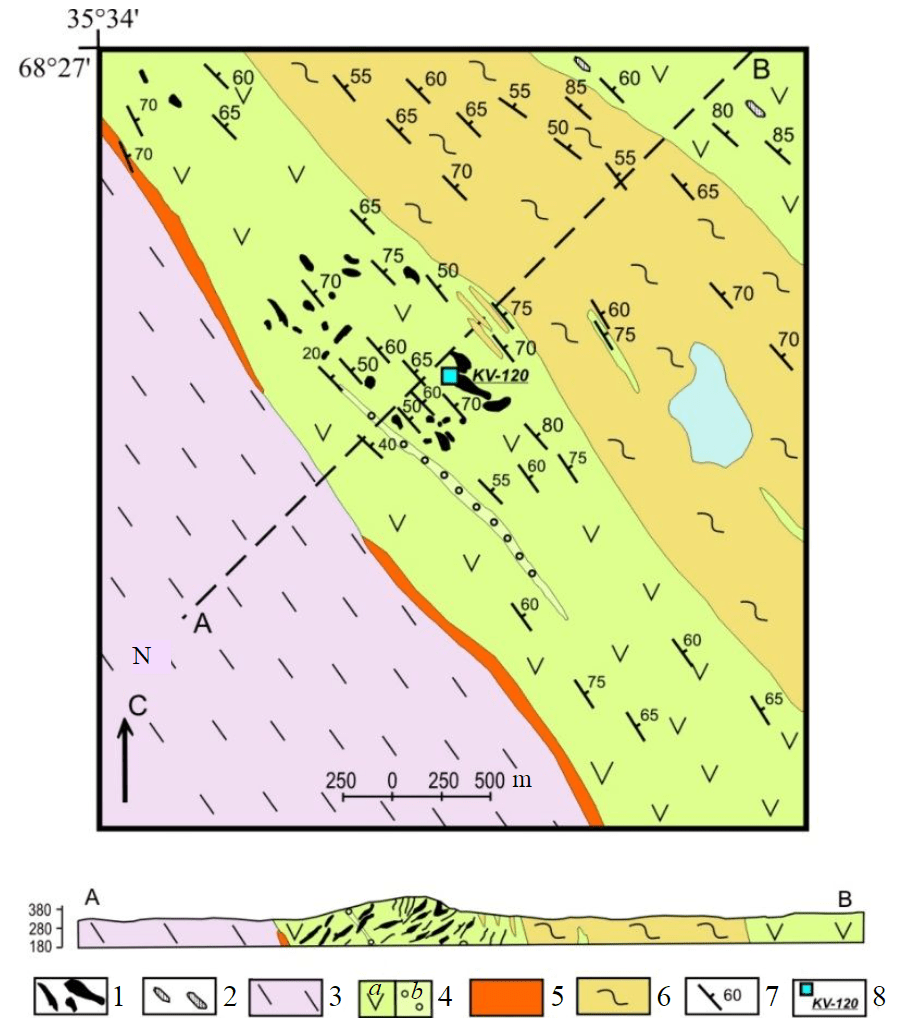
Fig.1. Deposits of Okhmylk rare-metal pegmatites (compiled by I.V.Ginzburg (1950) with the author's amendments) 1 – lepidolite-spodumene-tourmaline pegmatites; 2 – muscovite- tourmaline pegmatites; 3 – oligoclase gneiss-granites; 4 – amphibolites: a – massive, b – ovoid; 5 – biotite gneisses with interlayers of garnet-biotite gneisses; 6 – staurolite-biotite gneisses and muscovite quartzites; 7 – attitude; 8 – sampling site for isotopic geochronological studies
Methodology
To obtain mineralogical information, zircon was studied in rock chip samples, used to prepare polished sections from individual pieces. The zircon in rock chip samples was analyzed with the X-ray diffraction method: powder X-ray diffraction patterns were obtained with the photo method on URS-55, RKD chamber 114.6 mm, 40 kV, 15 mA, Fe radiation. The internal structure of zircon was studied in polished sections using a scanning electron microscope LEO-1450 (Carl Zeiss Microscopy, Germany) equipped with an X-ray energy dispersive detector ULTIM MAX 100 (OXFORD Instruments, Britain). A local U-Pb isotopic geochronological study of zircon was carried out at the SUMaC Center of Stanford University and the US Geological Survey on a SHRIMP-RG ion multi-collector microprobe using the technique described in [21, 22]. The processing of analytical data was carried out using the SQUID-2 program. The determination of Ti, Fe, Y, REE, Hf, U, and Th occurred simultaneously when secondary ions were applied to a selected point on the zircon surface, i.e. at the dating point. The concentration of impurity elements was calculated relative to the composition of Madagascar Green zircon (MAD) [23]. To plot the REE spectra in zircon, the values were normalized to the composition of CI chondrite [24]. The crystallization temperature of zircon was estimated using a Ti-in-Zrn thermometer [25]. ISOPLOT/Ex was used for the construction of U-Pb diagrams with concordia.
Results and discussion
Zircons from sample KV-120 are represented by euhedral, isometric, and close to isometric dipyramidal, dipyramidal-prismatic semitransparent fractured crystals, less often anhedral grains of gray and gray-brown color. The size does not exceed 1 mm and is often 0.3-0.5 mm. Zircon crystals predominantly formed along with albite and quartz. Zircon intergrowths with minerals of the columbite group are not uncommon (Fig.2, a), indicating partly simultaneous crystallization of the tantalite-(Mn) and zircon rims. According to [26], tantalite-(Mn) is the latest generation of the columbite group minerals.
The inner zones of zircons show signs of slight secondary (post-crystallization) alteration in the form of areas with a spotty structure (Fig.2, a), but these zones are not visually as porous as those in strongly altered zircons [27, 28]. The internal parts of zircons contain numerous micrometric-sized inclusions of other mineral phases, among which U(±Pb)-oxides predominate (Fig.2, b). The isometric shape (square and hexagonal sections) of the inclusions, high degree of euhedrality, and composition allow us to consider them as cubic and cuboctahedral crystals of uraninite UO2, syngenetically crystallized with zircon. Thus, the post-crystallization alteration did not deeply affect the original zircon from pegmatites, preserving its crystalline state. The crystalline state of zircon is also indicated by X-ray diffraction studies. In the structure of zircon, there is a phase heterogeneity, expressed in the presence of narrow outer rims, forming euhedral overgrowth on zircon (Fig.2, c). The outer rims do not appear in all individuals, they are light, homogenous, and of different thickness. The rims are broken by cracks radiating from the boundary with the inner zone. The boundary between the inner and outer zones is clear, without gradual transitions (Fig.2, c).
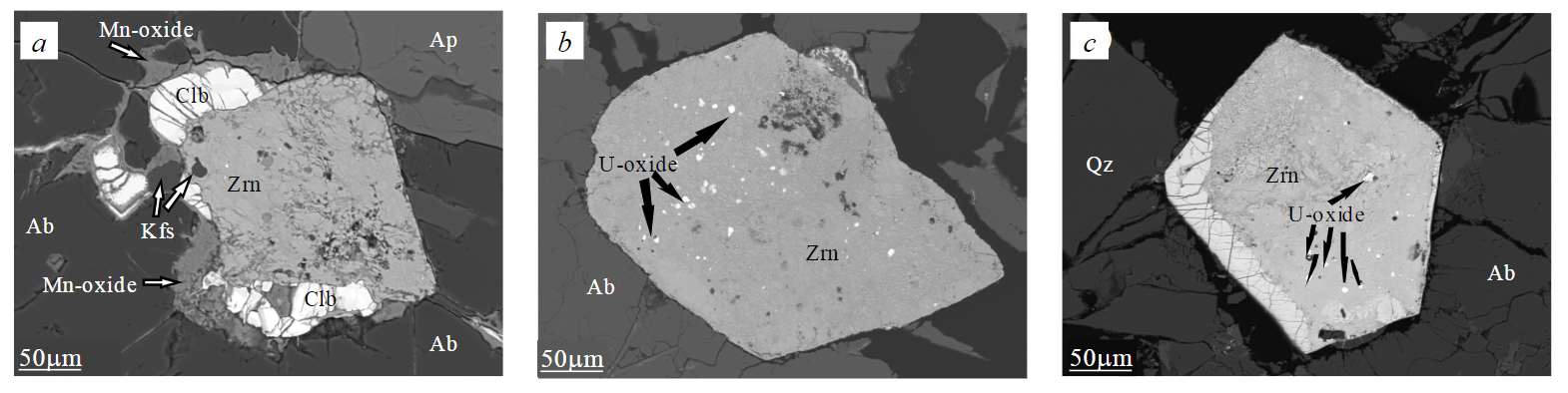
Fig.2. Zircon in back scattered electrons (BSE): a – relations of zircon with accessory and rock-forming minerals; b– uranium oxide inclusions in zircon; c – recrystallized border (light in BSE) around primary zircon containing uranium oxide inclusions Zrn – zircon; Qz – quartz; Ab – albite; Ap – apatite; Kfs – potassium feldspar; Clb – columbite; Mn-oxide – manganese oxide; U-oxide – uranium oxide
The REE spectra in the studied zircons have a differentiated distribution from LREE to HREE. Spectra of 1.1, 1.2, 2.1, and 4.1 points are similar, characterized by high total REE content (300-722 ppm), relatively flat spectrum of LREE (Smn/Lan varies from 0.96 to 34.7), positive Ce-anomaly (Ce/Ce* = 5-46) and enriched in heavy REE (283-719 ppm) (Table 1, Fig.3). The described spectra are characteristic of magmatic zircon [29]. The spectra of points 3.1 and 3.2 follow the general pattern of the above spectra but they are characterized by a very low total REE content (11-12 ppm) and intensive depletion in HREE (7.9-10.1 ppm). There is a small negative Er-anomaly and a well-defined positive Ce one (Ce/Ce* = 10-13). This REE spectrum reflects the recrystallization of the primary zircon. Thus, the considered features of rare and rare earth elements distribution make it possible to distinguish two zones within zircon crystals: one enriched in REE of primary zircon and one significantly depleted in these elements. Table 1 also shows low yttrium content (Y = 8-9 ppm) in the rims compared to the inner zones (Y = 400-700 ppm).
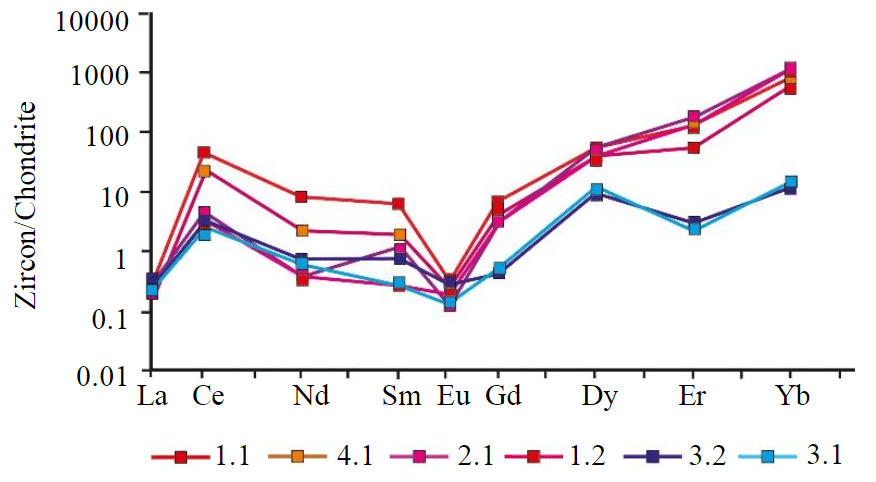
Fig.3. REE distribution in zircon from rare-metal pegmatites (normalized to chondrite according to [24])
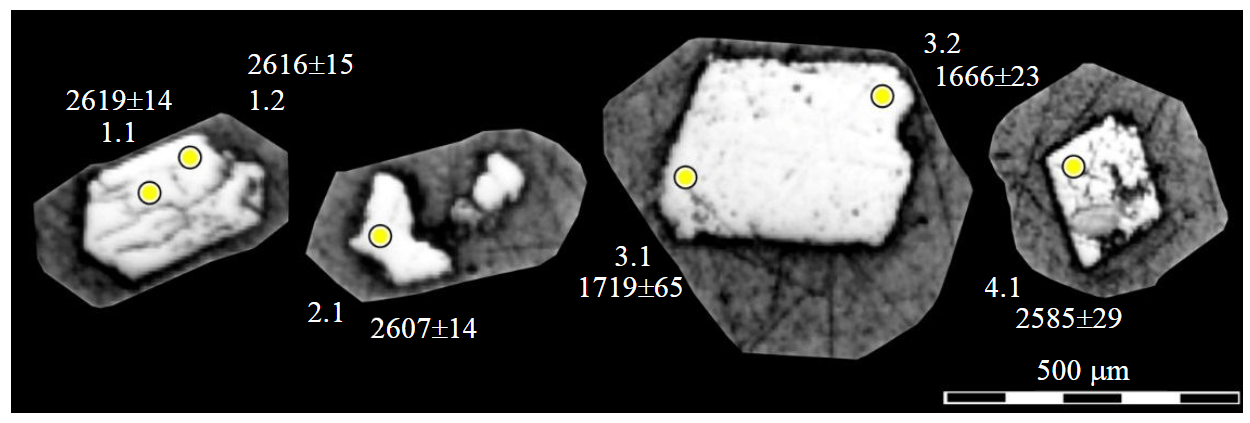
Fig.4. Zircon crystals in reflected light with applied analytical points according to Tables 1 and 2 and dating results (by 207Pb/206Pb, Ma) Light – areas of zircon exposed for the analysis
Determining the crystallization temperature of zircon is an important factor in assessing the crystallization medium. Zircon from rare-metal pegmatites of the studied deposit differs in Ti concentrations in different zones: in the inner, altered zones of zircon, the Ti content averages 10 ppm; in the recrystallized rims, it is about 65 ppm. Zircon crystallization temperatures calculated with the Ti thermometer should be approached with caution as the Ti content in zircon should not exceed 30 ppm for more accurate estimations of crystallization temperatures [25]. For the inner zones of zircon, the temperature can be estimated at 600-800 °C, and for the outer rims (points 3.1 and 3.2) – more than 900 °C (Table 1).
Table 1
The content of rare earth and rare elements (ppm), zircon crystallization temperature
Element |
Point in zircon |
|||||
1.1 |
1.2 |
2.1 |
3.1 |
3.2 |
4.1 |
|
Ti48 |
13.9 |
2.96 |
24.7 |
42.6 |
83.5 |
1.09 |
Fe |
0.54 |
0.07 |
0.50 |
0.19 |
0.71 |
1.80 |
Y |
668 |
736 |
399 |
8.1 |
9.1 |
22.6 |
La |
0.050 |
0.052 |
0.047 |
0.042 |
0.046 |
0.033 |
Ce |
38.7 |
3.21 |
1.85 |
1.88 |
2.38 |
25.9 |
Nd |
4.17 |
0.13 |
0.12 |
0.26 |
0.22 |
0.83 |
Sm |
1.08 |
0.18 |
0.03 |
0.03 |
0.10 |
0.27 |
Eu |
0.013 |
0.005 |
0.012 |
0.005 |
0.013 |
0.009 |
Gd |
1.46 |
0.56 |
0.99 |
0.095 |
0.076 |
0.71 |
Dy |
41.9 |
38.8 |
23.5 |
5.78 |
4.02 |
20.3 |
Er |
59.8 |
74.6 |
41.9 |
0.35 |
0.47 |
15.9 |
Yb |
500 |
605 |
476 |
3.9 |
3.3 |
246 |
Hf |
64757 |
73206 |
72867 |
112747 |
98096 |
167955 |
Eu/Eu* |
0.88 |
0.05 |
0.22 |
0.29 |
0.44 |
0.06 |
Ce/Ce* |
45.7 |
21.4 |
13.0 |
9.65 |
12.6 |
5.29 |
ƩREE |
647 |
722 |
544 |
12.3 |
10.6 |
300 |
ƩLREE |
42.9 |
3.38 |
2.02 |
2.18 |
2.66 |
16.7 |
ƩHREE |
603 |
719 |
543 |
10.1 |
7.87 |
283 |
Ybn/Lan |
14768 |
17243 |
14774 |
136 |
106 |
11178 |
Smn/Lan |
34.7 |
5.5 |
0.96 |
1.15 |
3.63 |
3.36 |
T(Ti), °C |
778 |
643 |
838 |
903 |
994 |
573 |
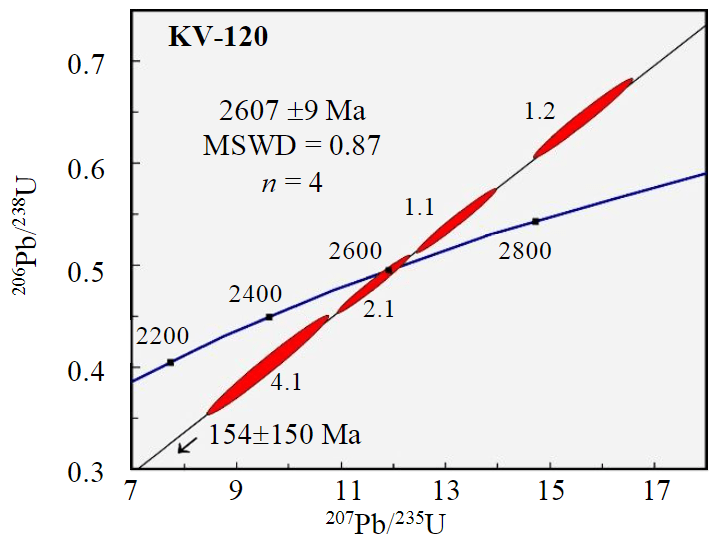
Fig.5. Concordia for zircon from rare-metal pegmatites of the Okhmylk deposit
For the analyzed zircon points (1.1, 1.2, 2.1, and 4.1) a discordance was plotted (Table 1, Fig.4, 5). For them, a discordant age of 2607±9 Ma was obtained, MSWD = 0.87 (Fig.5). Analytical points 3.1 and 3.2 probably reflect the ages of the outer recrystallized zone, located at the zircon crystal face, these points were not included in the discordia calculation. As can be seen in Fig.5, all analytical data are discordant and indicate a disturbance of the U-Pb system due to uneven distribution of uranium and lead at the analytical points. In the recrystallized zircon rims (3.1 and 3.2), uranium concentrations were 0.17-0.19 %, whereas in the points (1.1, 1.2, 2.1 and 4.1) the uranium content was several times higher, ranging from 3.01 to 4.12 mas.%. Thus, the upper intersection of discordia with concordia, which corresponds to an age of 2607±9 Ma, can be taken for the age of zircon crystallization. Analytical data with an age of ~1.7-1.6 Ga indicate a later, presumably, hydrothermal metasomatic process.
Table 2
Results of U-Pb isotopic study of zircon from pegmatites of the Okhmylk deposit
Point number |
206Pbс, % |
Content, % |
232Th/238U |
Isotopic ratios, % |
RhO |
Age, Ma |
D, |
|||||
206Pb* |
U |
207Pb/206Pb |
207Pb/235U |
206Pb/238U |
206Pb/238U |
207Pb/206Pb |
||||||
KV-120-4.1 |
0.02 |
2.19 |
4.12 |
0.056 |
0.139±0.8 |
9.593±10.1 |
0.403±10.1 |
0.985 |
2184±23 |
2582±29 |
+18 |
|
KV-120-1.1 |
0.06 |
1.59 |
3.15 |
0.053 |
0.145±0.5 |
13.201±4.9 |
0.543±5.1 |
0.985 |
2796±22 |
2619±14 |
+7 |
|
KV-120-1.2 |
0.07 |
1.78 |
3.69 |
0.085 |
0.170±1.3 |
15.656±5.0 |
0.645±5.2 |
0.985 |
3209±28 |
2616±15 |
−18 |
|
KV-120-2.1 |
0.06 |
1.54 |
3.01 |
0.021 |
0.179±1.1 |
11.634±5.1 |
0.482±5.1 |
0.985 |
2536±29 |
2607±14 |
+3 |
|
KV-120-3.2 |
0.02 |
0.06 |
0.17 |
0.011 |
0.181±0.5 |
4.570±7.3 |
0.324±7.4 |
0.997 |
1810±33 |
1666±23 |
−8 |
|
KV-120-3.1 |
0.06 |
0.08 |
0.19 |
0.011 |
0.183±0.4 |
6.886±5.6 |
0.474±5.3 |
0.950 |
2503±25 |
1719±65 |
−31 |
Note. The error in the calibration of the standard was 0.29 %. Errors are given at the level of 1σ, 206Pbc, and 206Pb* indicating the contents of ordinary and radiogenic lead, respectively. Measured ratios are corrected for 204Pb, D is discordance: D = 100·[age (207Pb/206U) / age (206Pb/238U) – 1]; RhO is the correlation coefficient between the errors in determining the 206Pb/238U and 207Pb/235U ratios. Values are corrected for mass fractionation, blank contamination, and ordinary lead according to the model [30].
Conclusions
Zircons from the Okhmylk deposit crystallized at the pegmatite stage in a paragenetic association with the pyrochlore group, molybdenite-I, arsenopyrite, and uraninite-I [18, 20]. Zircon seized excess uranium into its crystal lattice as an isomorphic impurity, but later during recrystallization the uranium formed its own mineral phase uraninite (UO2). Under the impact of aqueous fluids and due to radioactive decay, high-uranium zircons were slightly altered, but with preservation of zircon crystallinity. The fluid phase promoted partly removal of the radiogenic lead, as it is evident with the discordant position of the analytical points. Zircon of the metasomatic stage in the outer parts of the primary zircon crystals, formed along with columbite, triphylite, apatite, tourmaline, and beryl groups [20].
The obtained isotopic age of zircon 2607±9 Ma determines the time of its crystallization and serves as a reliable estimation of the time of the Okhmylk deposit formation, reflecting the global era of pegmatite formation, when the world's largest rare metal pegmatite deposits formed [26]. The formation of the zircon outer rims associates with zircon recrystallization in the Paleoproterozoic
(1.7-1.6 billion years ago).
References
- Černý P. Rare-element granite pegmatites. Part I. Anatomy and internal evolution of pegmatite deposits. Geoscince Canada. 1991. Vol. 18. N 2, p. 49-67.
- London D. Pegmatites. The Canadian Mineralogist. Special Publication. Quebec: Society of Economic Geologists. 2008. Vol. 10, p. 368. DOI: 10.2113/gsecongeo.103.8.1730
- London D. Ore-forming processes within granitic pegmatites. Ore Geology Reviews. 2018. Vol. 101, p. 349-383. DOI: 10.1016/j.oregeorev.2018.04.020
- Tkachev A.V. Evolution of metallogeny of granitic pegmatites associated with orogens throughout geological time. Geological Society. 2011. Vol. 350, p. 7-23. DOI: 10.1144/SP350.2
- Mccauley A., Bradley D.C. The global age distribution of granitic pegmatites. The Canadian Mineralogist. 2014. Vol. 52. Iss. 2, p. 183-190. DOI: 10.3749/canmin.52.2.183
- Kudryashov N.M., Mokrushin A.V. Mesoarchean Gabbroanorthosite Magmatism of the Kola Region: Petrochemical, Geochronological, and Isotope-Geochemical Data. Petrology. 2011. Vol. 19. N 2, p. 167-182. DOI: 10.1134/S086959111102007X
- Pushkarev Yu.D., Kravchenko E.V., Shestakov G.I. Geochronometric references of the Precambrian of the Kola Peninsula. Leningrad: Nauka, 1978, p. 136. (in Russian).
- Kudryashov N.M., Lyalina L.M., Apanasevich E.A. Age of Rare-Metal Pegmatites from the Vasin-Myl’k Deposit (Kola Region): Evidence from U-Pb Geochronology of Microlite. Doklady Earth Sciences. 2015. Vol. 461. N 2, p. 321-325. DOI: 10.1134/S1028334X15040042
- Kudryashov N.M., Skublov S.G., Galankina O.L. et al. Abnormally high-hafnium zircon from rare-metal pegmatites of the Vasin-Mylk deposit (northeastern part of the Kola Peninsula). Geochemistry. 2019. Vol. 80. Iss. 3. DOI: 10.1016/j.geoch.2018.12.001
- Morozova L.N. Lithium Kolmozero deposit of rare metal pegmatites: New data on rare element composition (Kola Peninsula). Lithosphere (Russia). 2018. Vol. 18. N 1, p. 82-98 (in Russian). DOI: 10.24930/1681-9004-2018-18-1-082-098
- Chew D.M., Sylvester J.P., Tubrett M.N. U-Pb and Th-Pb dating of apatite by LA-ICPMS. Chemical Geology. 2011. Vol. 280. Iss. 1-2, p. 200-216. DOI: 10.1016/j.chemgeo.2010.11.01
- Rizvanova N.G., Skublov S.G., Cheremazova E.V. Age of hydrothermal processes in the central iberian zone (Spain) according TO U-Pb dating of cassiterite and apatite. Journal of Mining Institute. 2017. Vol. 225, p. 275-283. DOI: 10.18454/PMI.2017.3.275
- Skublov S.G., Krasotkina A.O., Makeev A.B. et al. The First Data on the U-Pb Age (Tims and La-Icp-Ms) Of Rutile from the Ichetju Polymineral Occurrence, the Middle Timan. Journal of Mining Institute. 2018. Vol. 232, p. 357-363. DOI: 10.31897/PMI.2018.4.357
- Salnikova E.B., Chakhmouradian A.R., Stifeeva M.V. et al. Calcic garnets as a geochronological and petrogenetic tool applicable to a wide variety of rocks. Lithos. 2019. Vol. 338-339, p. 141-154. DOI: 10.1016/j.lithos.2019.03.032
- Hao-Cheng Yu, Kun-Feng Qiu, Callum J. et al. HetheringtonApatite as an alternative petrochronometer to trace the evolution of magmatic systems containing metamict zircon. Contributions to Mineralogy and Petrology. 2021. Vol. 176. N 68. DOI: 10.1007/s00410-021-01827-z
- Kudryashov N.M., Udoratina O.V., Kobl M.A., Steshenko E.N. Estimation of the formation time of rare-metal pegmatites of the Kolmozero-Voronya zone based on the isotopic geochronological study of zircon (SHRIMP RG) from tourmaline-muscovite granites. Materialy VII Rossiyskoy konferentsii po izotopnoy geokhronologii, 20-22 noyabrya 2017, Moskva, Rossiya. Institut geologii rudnykh mestorozhdeniy, petrografii, mineralogii i geokhimii Rossiyskoy Akademii nauk (Moskva), 2018, p. 161-163 (in Russian).
- Kudryashov N.M., Udoratina O.V., Coble M., Steshenko E.N. Geochronological and Geochemical Study of Zircon from Tourmaline-Muscovite Granites of the Archaean Kolmozero-Voronya Greenstone Belt: Insights into Sources of the Rare-Metal Pegmatites. Minerals. 2020. Vol. 10. Iss. 9. N 760. DOI: 10.3390/min10090760
- Sosedko A.F. Materials on the geology and geochemistry of granite pegmatites. Moscow: Gosgeoltekhizdat, 1961, p. 152 (in Russian).
- Voloshin A.V., Pakhomovskiy Ya.A. Mineralogy of tantalum and niobium in rare metal pegmatites. Leningrad: Nauka, 1988, p. 242 (in Russian).
- Gordienko V.V. Mineralogy, geochemistry and genesis of spodumene pegmatites. Leningrad: Nauka, 1988, p. 242 (in Russian).
- Ireland T.R. Ion Microprobe Mass-Spectrometry: Techniques and Applications in Cosmochemistry and Geochronology. Advances in Analytical Geochemistry. 1995. Vol. 2, p. 1-118.
- Coble M.A., Vazquez J., Barth A.P. et al. Trace Element Characterization of MAD-559 Zircon Reference Material for Ion Microprobe Analysis. Geostandards and Geoanalytical Research. 2018. Vol. 42. Iss. 4, p. 481-497. DOI: 10.1111/ggr.12238
- Barth A.P., Tani K., Meffre S. Generation of silicic melts in the early Izu-Bonin arc recorded by detrital zircons in proximal arc volcaniclastic rocks from the Philippine Sea. Geochemistry, Geophysics, Geosystems. 2017. Vol. 18. Iss. 10, p. 3576-3591. DOI: 10.1002/2017GC006948
- McDonough W.F., Sun Sh.S. The composition of the Earth. Chemical Geology. 1995. Vol. 120. Iss. 3-4, p. 223-253. DOI: 10.1016/0009-2541(94)00140-4
- Ferry J.M., Watson E.B. New thermodynamic models and revised calibrations for the Ti-in-zircon and Zr-in-rutile thermo-meters. Contributions to Mineralogy and Petrology. 2007. Vol. 154, p. 429-437. DOI: 10.1007/s00410-007-0201-0
- Badanina E.V., Sitnikova M.A., Gordienko V.V. et al. Mineral chemistry of columbite-tantalite from spodumene pegmatites of Kolmozero, Kola Peninsula (Russia). Ore Geology Reviews. 2015. Vol. 64, p. 720-735. DOI: 10.1016/j.oregeorev.2014.05.009
- Nasdala L., Hanchar J.M., Rhede D. et al. Retention of uranium in complexly altered zircon: An example from Bancroft, Ontario. Chemical Geology. 2010. Vol. 269. Iss. 3-4, p. 290-300. DOI: 10.1016/j.chemgeo.2009.10.004
- Zamyatin D.A., Shchapova Yu.V., Votyakov S.L. Alteration and chemical U-Th-total Pb dating of heterogeneous high-uranium zircon from a pegmatite from the Aduiskii massif, middle Urals, Russia. Mineralogy and Petrology. 2017. Vol. 111, p. 475-497. DOI: 10.1007/s00710-017-0513-3
- Hoskin P.W.O. Trace-element composition of hydrothermal zircon and the alteration of Hadean zircon from the Jack Hills, Australia. Geochimica et Cosmochimica Acta. 2005. Vol. 69. Iss. 3, p. 637-648. DOI: 10.1016/j.gca.2004.07.006
- Stacey J.S., Kramers I.D. Approximation of terrestrial lead isotope evolution by a two-stage model. Earth and Planetary Science Letters. 1975. Vol. 26. Iss. 2, p. 207-221. DOI: 10.1016/0012-821X(75)90088-6