Thermal history of diamond from Arkhangelskaya and Karpinsky-I kimberlite pipes
Abstract
This work studies and compares the main morphological, structural, and mineralogical features of 350 diamond crystals from the Karpinsky-I and 300 crystals of the Arkhangelskaya kimberlite pipes. The share of crystals of octahedral habit together with individual crystals of transitional forms with sheaf-like and splintery striation is higher in the Arkhangelskaya pipe and makes 15 %. The share of cuboids and tetrahexahedroids is higher in the Karpinsky-I pipe and stands at 14 %. The share of dodecahedroids in the Arkhangelskaya and Karpinsky-I pipes are 60 % and 50 %, respectively. The indicator role of the nitrogen-vacancy N3 center active in absorption and luminescence is shown. Crystals with the N3 absorption system have predominantly octahedral habit or dissolution forms derived from the octahedra. Their thermal history is the most complex. Absorption bands of the lowest-temperature hydrogen-containing defects (3050, 3144, 3154, 3188, 3310 cm −1 , 1388, 1407, 1432, 1456, 1465, 1503, 1551, 1563 cm −1 ), are typical for crystals without N3 system, where in the absorption spectra nitrogen is in the form of low-temperature A and C defects. The above mentioned bands are registered in the spectra of 16 % and 42 % of crystals from the Arkhangelskaya and Karpinsky-I pipes, respectively. The diamond of the studied deposits is unique in the minimum temperature (duration) of natural annealing. Based on a set of features, three populations of crystals were distinguished, differing in growth conditions, post-growth, and thermal histories. The established regularities prove the multi-stage formation of diamond deposits in the north of the East European Platform and significant differences from the diamonds of the Western Cisurals. The results suggest the possibility of the existence of primary deposits dominated by diamonds from one of the identified populations.
Introduction
Two primary diamond deposits were discovered on the East European Platform: the Lomonosov deposit of the Zolotitskoye kimberlite field and the Grib deposit of the Kepinskoye field (Arkhangelsk region) [1, 2]. The development of these deposits began only in the XXI century, and their diamonds have not yet been sufficiently investigated by modern analytical methods [3-5]. The kimberlite pipes of these deposits are overlain by Carboniferous and cut through the Upper Vendian deposits. The kimberlite bodies are cratered and their denudation rate is insignificant [1, 6]. The areas promising for the discovery of primary deposits are covered by late deposits. Consequently, placer deposits are not predicted in the territory, and the typomorphic features of the diamond are not significant for the direct purposes of prospecting geology and are not studied enough [2, 3]. Some researchers suggest the possibility of finding primary sources of Ural diamonds in the north of the East European Platform. In this regard, the study of diamond typomorphic features is essential for solving applied prospecting problems and in terms of fundamental studies of diamond formation, issues of regional geology, and mine-
rageny [4]. The study of diamonds is one of the few ways to identify the patterns of deep processes that led to the formation of kimberlite pipes [5, 7, 8].
Diamond has a very stable structure, an elementary chemical composition, and no post-growth homogenization [9-11]. Indicators of changes in growth conditions and post-growth processes remain in the zoning of diamond crystals, including after the imposition of secondary inhomogeneities [12, 13]. Most other deep-seated minerals, except for zircon [14-16], are homogenized during long-term natural annealing and show no growth zoning [13, 17, 18]. Changing environmental conditions are often studied by the zoning of the chemical composition or micro impurities [19-21]. In diamonds, the volume distribution of crystal structure defects is also studied [11, 22-24]. The thermal history of diamonds is determined by the ratio of nitrogen concentrations in the form of the main nitrogen defects С, А, В
[12, 25]. These defects include one C(N), two A(N2), and four B(N4V) substituting nitrogen atoms [26]. During natural annealing of diamond, the main nitrogen defects of the crystal structure and complexes of interstitial atoms B’ are transformed [25] in the series С(N) → А(N2) → B(N4V) +B’(In), where V is a vacancy, I is an interstitial carbon atom, N is a substituting nitrogen atom.
Nitrogen-vacancy defects NV, H3(N2V), N3(N3V), and their ionized analogs are formed during the transformation. At this stage, the formation of the nitrogen–hydrogen defect N3VH also occurs [27, 28]. Nitrogen-vacancy defects appear as intermediate defects during the transformation of the main ones. Two approaches can be used to determine the thermal history of diamond crystals and the post-growth evolution of the environment. The first one is to study the zoning of individual crystals [11, 29] since the zoning of each crystal reflects some stages of its ontogeny. This approach involves making diamond plates and therefore is not always possible.
The second approach is to study large samples of crystals with the identification of individual diamond populations or trends in their characteristic features [30]. Populations may differ in thermal history, impurity concentration, crystal morphology, and composition of inclusions. The methodological complexity of the second approach lies in the presence of zones and sectors in most crystals. Sequential growth zones differ in the set of impurities and thermal activity, and the superposition of their characteristics significantly complicates the interpretation of the results. The implementation of the second approach implies the need to identify and analyze statistical patterns between various morphological and physical (spectroscopic) characteristics. Thus, three groups of crystals with different morphological and spectroscopic characteristics were previously identified in diamonds from the commercial Lomonosov deposit [4]. Further study of the morphology and physical characteristics of diamonds from these deposits is important both for understanding the patterns of diamond formation in different regions and for solving the problem of searching for buried sources, as well as for comparison with the Timan and the Urals diamonds.
The Arkhangelskaya and Karpinsky-I kimberlite pipes contain many yellow crystals [3, 30]. The yellow color is due to C or N3 defects, of which N3 are active in luminescence [31]. The C and N3 defects correspond to different conditions of crystal annealing and, accordingly, are indicators of their thermal history. Identification of these defects makes it possible to distinguish crystals from different stages of diamond formation. The main objective of the study is a comprehensive non-destructive study of diamond crystals from the Arkhangelskaya and Karpinsky-I pipes and the determination of the thermal history of individual diamond populations.
Methodology
In 2020 the mineralogical features of 350 crystals of the Arkhangelskaya pipe and 300 crystals of the Karpinsky-I pipe from industrial mining were studied. A comprehensive spectroscopic study of 164 crystals from the Arkhangelskaya pipe and 152 crystals from the Karpinsky-I pipe was carried out. The size of the crystals varies in the range of 3-5 mm. IR absorption spectra were recorded on a VERTEX-70 (Bruker, Germany) Fourier spectrometer with a Hyperion1000 microscope at a resolution of 2 cm−1. The total nitrogen concentration (Ntot) and the proportion of nitrogen in the form of B defects (NBS) were calculated using the known proportionality coefficients [25]. The absorption coefficients of the bands В’ (αB’), 3107 cm−1 (α3107) were determined. The PL spectra at room temperature were recorded on a Horiba FL-3 spectrometer with Xe excitation by a 450 W lamp, excitation (λEX) at 350 and 450 nm, with spectral slit widths of the excitation monochromator and detecting monochromator of 10 and 1 nm, respectively. The spectra at the temperature of 77 K, excited by 488 and 787 nm lasers λEX, were recorded on an InVia (Renishaw) spectrometer. Absorption spectra in the range of 200-800 nm with a spectral slit width of 1 nm were recorded on a UV-2550 spectrometer (Shimadzu, Japan) at room temperature. The absorption coefficient at a peak of 415 nm was determined relative to the background line (aN3) 450-420 nm with linear approximation. The minimum determined value of the absorption coefficient in the zero-phonon N3 line was 0.01 cm–1; the color is affected by the N3 system at an absorption coefficient of more than 0.2 cm−1.
The main goal of studying the morphology of crystals was to identify the growth form for further analysis of their thermal history: octahedra, cuboids, and individual crystals of mixed habit were identified. To determine the growth form of crystals with signs of strong dissolution, it is necessary to make diamond plates or study surface cathode luminescence. Crystals whose growth form is not unambiguously detected by the methods used were analyzed as a separate group.
Discussion
A distinctive feature of diamonds from the Lomonosov deposit, in comparison with the well-studied diamond from the primary sources of the Siberian Platform [30], is the high content of curved dodecahedroids and tetrahexahedroids [3]. These types can be very close in appearance since they represent the limiting dissolution form of both cuboids and octahedral crystals. Many of these crystals are distinguished by bulk yellow and yellow-green luminescence; according to the classification of Yu.L.Orlov [32], they can be attributed to the second variety. There are 13 % of such crystals in the Arkhangelskaya pipe, and 25 % in the Karpinsky-I pipe. These values refer to the diamond mined in 2020 and are fully consistent with previously published data [2, 3]. For many of the studied crystals assignment to I or II types of J.L.Orlov's classification is debatable (Table 1).
In the entire sample, only one crystal of IV type of Orlov's classification was found, and it was a crystal from the Arkhangelskaya pipe. This is a fragment of an indefinite habit with a well-defined gray, fibrous, thin, but opaque rim. Eight dark gray and black individual crystals from the Arkhangelskaya pipe sample are conditionally assigned to V type, based on the presence of a more translucent central zone, which can be seen in the chip, and a higher concentration of black fine inclusions in the peripheral zone. The luminescence of such crystals is visually very weak. In the IR and visible regions, the light absorption by such crystals exceeds the range of optical density detected by the spectrometers used. Typical crystals in terms of morphology with different dissolution degrees are shown in Fig.1. The top two rows represent the octahedral-dodecahedroid morphological series with increasing degree of dissolution, and the bottom one – cuboid – tetrahexahedroid – dodecahedroid.
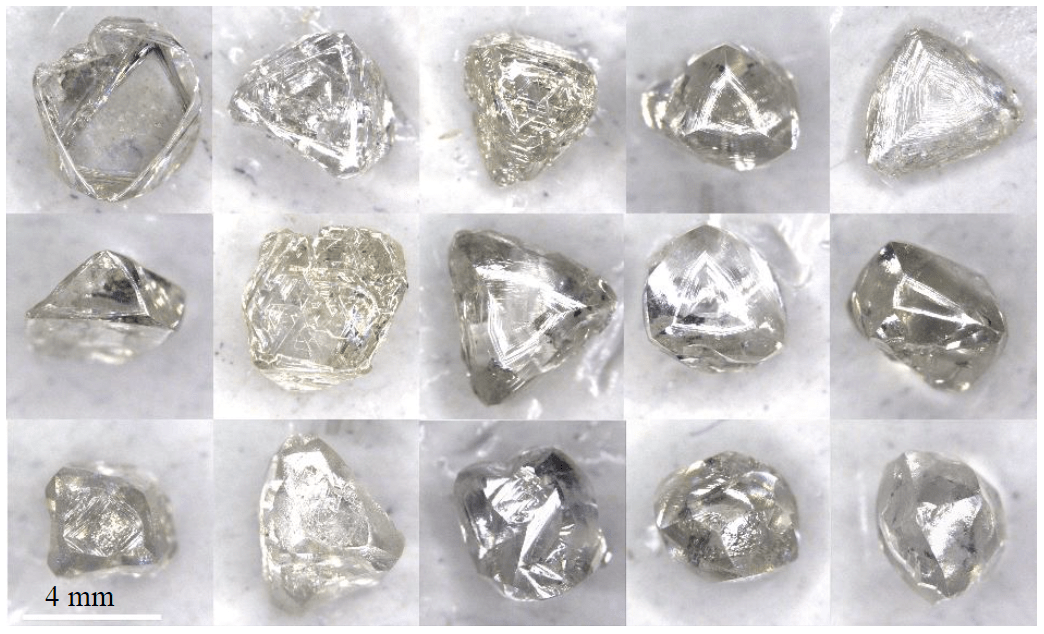
Fig.1. Typical in terms of morphology crystals of Arkhangelskaya and Karpinsky-I kimberlite pipes with different degrees of dissolution
In the studied collection, dodecahedroids (50-60 %) are sharply dominated by crypto-laminar and fine-laminar, smooth-faced Uralic type and with sheaf-like and sheaf-splintery striation. Characteristic of the deposit are crystals with contrasting concentric striation. Interpretation of such striation requires additional studies; it may correspond to contrasting growth zoning or post-growth plastic deformation. There are single flat-faced sharp-edge octahedra without traces of dissolution in Karpinsky-I and Arkhangelskaya pipes. The share of crystals of octahedral habit is less than 10 %. Together with crystals with sheaf-like and splintery striation near the edges of the octahedra, their share reaches 15 %. Surfaces of a different area with sheaf-like and splintery striation form a typical habit of crystals of transitional forms. Crystals with parallel striations are not found.
Table 1
Mineralogical classification of diamonds by Yu.L. Orlov, frequency of occurrence of crystals by type and habit, %
A.P.Karpinsky-I pipe (300 samples) |
||||||||||
Color |
Type I |
Types II+III |
Type V |
Type VII |
Total by color |
|||||
Octahedra |
Mixed-habit |
Dodecahedroid |
Shapeless |
Tetrahexahedroid |
Tetrahexahedroid+cube |
Cube |
||||
Colorless |
0.0 |
3.2 |
24.8 |
2.7 |
1.0 |
1.4 |
1.4 |
0.0 |
0.0 |
34.4 |
Yellow |
2.7 |
3.7 |
11.4 |
0.9 |
5.1 |
5.5 |
0.7 |
0.0 |
0.0 |
30.2 |
Gray-yellow |
1.8 |
1.4 |
2.7 |
1.8 |
5.0 |
2.7 |
0.0 |
0.0 |
0.0 |
15.5 |
Brown |
0.7 |
0.7 |
7.1 |
2.3 |
0.3 |
1.8 |
0.3 |
0.0 |
0.0 |
13.2 |
Yellow-brown |
0.0 |
0.0 |
0.0 |
0.0 |
0.0 |
0.0 |
0.0 |
0.0 |
0.0 |
0.0 |
Pink |
0.0 |
0.0 |
0.7 |
0.0 |
0.0 |
0.0 |
0.0 |
0.0 |
0.0 |
0.7 |
Gray |
0.3 |
0.7 |
2.2 |
0.0 |
0.0 |
0.0 |
0.0 |
0.3 |
0.0 |
3.5 |
Dark gray |
0.0 |
0.3 |
0.7 |
0.0 |
0.0 |
0.0 |
0.0 |
0.7 |
0.0 |
1.7 |
Pigmentation patches |
0.0 |
0.0 |
0.7 |
0.0 |
0.0 |
0.0 |
0.0 |
0.0 |
0.0 |
0.7 |
Total |
5.6 |
9.9 |
50.3 |
7.8 |
5.6 |
11.4 |
6.1 |
1.0 |
0.0 |
100.0 |
Arkhangelskaya pipe (350 samples) |
||||||||||
Colorless |
2.4 |
2.8 |
22.0 |
1.2 |
0.0 |
0.4 |
0.0 |
0.0 |
1.2 |
29.9 |
Yellow |
3.9 |
1.6 |
8.7 |
0.8 |
5.9 |
3.5 |
0.4 |
0.0 |
0.4 |
25.2 |
Gray-yellow |
0.0 |
0.0 |
2.0 |
0.0 |
1.2 |
0.0 |
0.0 |
0.0 |
0.4 |
3.9 |
Brown |
2.0 |
0.8 |
4.7 |
0.8 |
0.4 |
0.0 |
0.0 |
0.0 |
0.0 |
8.7 |
Yellow-brown |
0.0 |
0.4 |
2.0 |
0.0 |
0.0 |
0.4 |
0.0 |
0.0 |
0.0 |
2.8 |
Pink |
0.0 |
0.0 |
0.8 |
0.0 |
0.0 |
0.0 |
0.0 |
0.0 |
0.0 |
0.8 |
Gray |
0.0 |
1.2 |
15.7 |
0.8 |
0.0 |
0.0 |
0.0 |
2.0 |
3.9 |
23.6 |
Dark gray |
0.0 |
0.0 |
2.0 |
0.0 |
0.0 |
0.0 |
0.0 |
1.2 |
0.0 |
3.1 |
Pigmentation patches |
0.0 |
0.0 |
1.2 |
0.0 |
0.0 |
0.0 |
0.4 |
0.0 |
0.4 |
2.0 |
Total |
8.3 |
6.7 |
59.1 |
3.5 |
7.5 |
4.3 |
0.8 |
3.1 |
6.3 |
100.0 |
The color is dominated by gray crystals with numerous graphite inclusions that affect the perception of color. Among pure colors, crystals with a yellow hue of varying intensity dominate. Among the fantasy colors, yellow prevails; purple and pink crystals, not typical for the primary deposits of the Siberian Platform, are less common. In the pipes of the Lomonosov deposit, the share of brown crystals is less than 10 %.
Crystals of an octahedral habit in equal proportions are colorless, yellow and yellow with a gray-brown hue. Among the mixed-form crystals, colorless and yellow ones are equally common; brown and grayish-yellow ones are found in single cases. Dodecahedroids in the Arkhangelskaya and Karpinsky-I pipes make up the majority (15 %) of colorless crystals with a high degree of transpa-rency, about 5-7 % are yellow and straw-yellow diamonds with different hue intensity, while 5 % are yellow with a gray (smoky) component in coloration. Tetrahexahedroids have a specific color: yellow with a low degree of transparency, and yellow with a smoky or gray-green component. Their transparency is lowered due to scattered finely dispersed inclusions, which cannot be detected by nondestructive methods [33], however, tetrahexahedroids and crystals close to them demonstrate different degrees of saturation with inclusions. Tetrahexahedroids are characterized by the presence of intense yellow-green PL, usually uniformly distributed in the volume, but there are samples in which the luminescence is visually determined as surface one.
When carrying out a morphological description of crystals for the subsequent analysis of spectroscopy data, the most important parameter is the identification of the dominant growth mechanism. There are two main growth mechanisms: tangential of {111} faces and normal growth of subparallel {100} surfaces. In the first case, flat-faced octahedra are formed, and in the second – cuboids. Sometimes there are crystals of mixed habit, with joint growth of {111} faces and subparallel {100} surfaces. Crystals the central part of which grew by the normal mechanism and outer zones – by the tangential one are often observed. The difference in the growth mechanism is the basis for the diversity of crystal morphology and its spectroscopic characteristics. In growth pyramids <100> formed during the growth of subparallel {100} surfaces, a3017 is systematically higher and Ntot is lower than in synchronous zones of <111> pyramids [22, 23]. Pyramids <100> in crystals of mixed habit often contain submicron inclusions, in which methane and graphite are detected [34].
The basic parameter that determines the spectroscopic characteristics of diamond crystals is the concentration of nitrogen impurity. NBS, αB’, and α3107 parameters depend on this parameter, as well as the thermal history, and the crystal growth mechanism. A feature of the studied samples is the frequent occurrence in the spectra of two absorption systems, the nature of which has not yet been defined [4]. These systems are revealed in the spectra if there is no B’ band in the absorption and there is no N3 absorption. The first system consists of narrow 3050, 3144, 3154, 3188, and 3310.5 cm−1 lines, along with them, in most cases, the 1363 cm−1 band is recorded. The second includes lines 1388, 1407, 1432, 1456, 1465, 1503, 1551, 1563 cm–1 bands. The systems are registered in the spectra of 14 and 2.5 % crystals of the Arkhangelskaya pipe and 14 and 28 % of diamonds of the Karpinsky-I pipe. Previously, these systems were described in single crystals as a rare feature – the lowest temperature form of hydrogen-containing defects [27]. At the same time, the studied set of samples does not contain crystals of Ib type (without A defects) or Iab, in which C defects are the dominant form of nitrogen. In the studied yellow crystals, the concentration of C defects is below the detection limit of IR spectroscopy. The absorption of the N3 defect (Fig.2, a) is detected in the spectra of 35 % of the crystals of the Karpinsky-I pipe and 47 % of the Arkhangelskaya pipe. The distribution of crystals by aN3 is lognormal (Fig.2, b). The B’ band is present in 78 % and 62 % of the spectra of crystals from the Arkhangelskaya and Karpinsky-I pipes, respectively. It is detected in the spectra of 44 % of crystals without N3 in the absorption from the Arkhangelskaya pipe and 43 % from the Karpinsky-I pipe.
Among the studied crystals from the Karpinsky-I pipe, there are no nitrogen-free ones; the ma-ximum value of NBS is 65 % (Fig.3, a). Most of the crystals are located in the range of 600-1400 ppm Ntot. The crystal arrangement on the Taylor diagram (NBS-Ntot parameters) is very compact: more than 90 % have an NBS value of less than 30 %. The distribution of Ntot is unimodal, with a maximum in the range of 1050-1200 ppm.
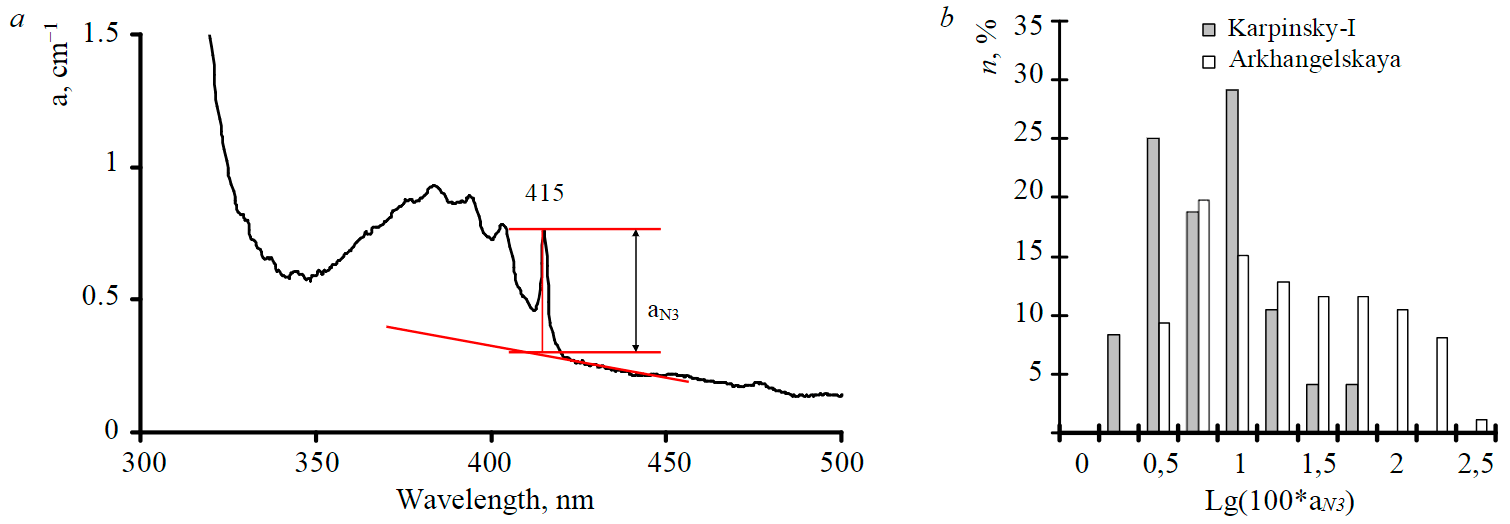
Fig.2. The absorption spectrum of a crystal with the N3 system (a) and distribution of diamond crystals from the Karpinsky-I and Arkhangelskaya pipes (b) according to the absorption coefficient of the N3 system in the absorption spectrum
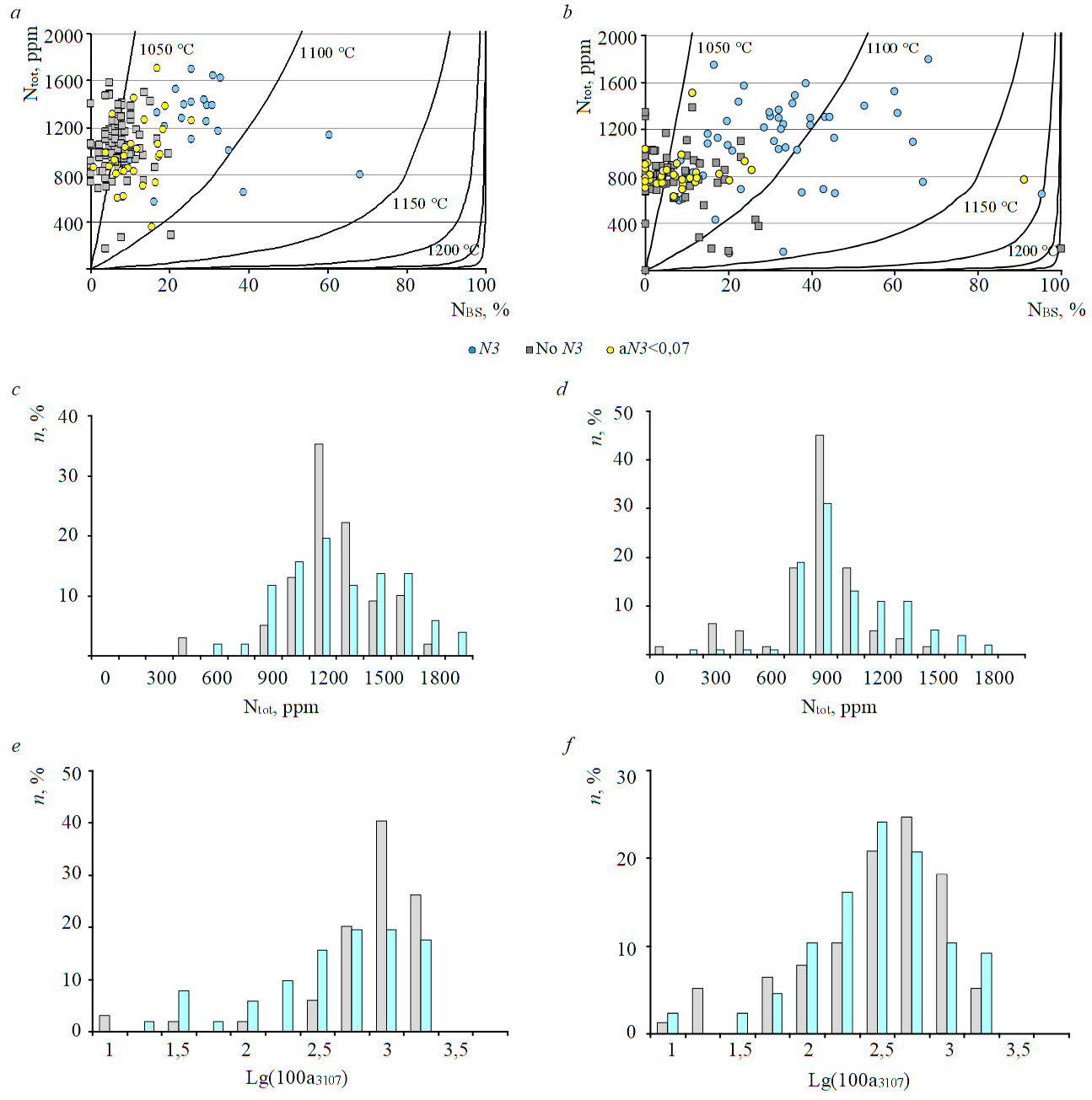
Fig.3. Distribution of crystals of A.P. Karpinsky-I (a, c, e) and Arkhangelskaya (b, d, f) diamond pipes: on Taylor diagram (a, b), model time 3Ga, on total nitrogen concentration (c, d), on the absorption coefficient of 3107 cm−1 band N3VH defect (e, f) with the division of crystals by the presence of N3 in the absorption spectrum
Among the crystals of the Arkhangelskaya pipe, one is nitrogen-free and one with an NBS value of 100 % (Fig.3, b). The PL spectrum of this crystal exhibits lines at 536 and 575 nm, which are characteristic of IaB type crystals with signs of plastic deformation. The crystal distribution of the Arkhangelskaya pipe in Ntot is more compact (Fig.3, c, d), and the crystal distribution field in the Taylor diagram is wider in both NBS and Ntot than for crystals from the Karpinsky-I pipe. The distribution of Ntot is unimodal, with a maximum in the range of 900-1050 ppm. The crystals of both pipes with the N3 system in the absorption spectra have a higher NBS value and a broader Ntot distribution. The distribution fields on the Taylor diagram of crystals with and without the N3 system overlap, but only single crystals without the N3 system fall above 20 % NBS. On the frequency distribution over the absorption coefficient of the 3107 cm−1 band of the N3VH defect, the maximum in crystals with the N3 absorption system is characterized by a smaller value than in crystals without this defect (Fig.3, e, f). This regularity is valid both for the crystals of the Arkhangelskaya and Karpinsky-I pipes. The obtained results show a significant difference between the diamond of these pipes and the diamond-bearing objects of the Urals [35]. Diamond from the alluvial placers of the Western Cisurals is characterized by a distribution over the entire Taylor diagram, there are also low-nitrogen crystals, many crystals with high NBS. The diamond of the Rassolninskoye deposit has a unimodal distribution in Ntot of about 20-40 % NBS, while in the diamond of the studied collections, the main part of the distribution falls in the region of 0-20 %.
All crystals of the octahedral habit from the Karpinsky-I pipe have the N3 system in the absorption spectra (Fig.4, a). In the Arkhangelskaya pipe, most of the crystals of the octahedral habit have the N3 system in the absorption spectra, the other three have Ntot less than 300 ppm (Fig.4, b). It appears that the concentration of N3 defects in these three crystals is below the detection limit according to the method used because of the low value of Ntot.
The PL spectra of most crystals at room temperature and UV excitation contain the N3 system or a broad amorphic band with a maximum at about 550 nm (S3) (Fig.5).
Crystals have blue or green PL. In addition to these bands, the S2 system with a zero-phonon line at 489 nm (poorly detected against the background of the S3 system), a group of lines at 583 + 605 + 627 nm, and a line at 787 nm are recorded. In crystals with the N3 system, it appears in the absorption spectra of 98 % of crystals and dominates in the spectra of 70-80 % of them. In crystals without the N3 system in the absorption, it appears in the 40-55 % of PL spectra (Table 2). The PL spectra of the studied crystals are much more diverse at a temperature of 77 K and an excitation of 488 nm. The main systems observed under these conditions were described earlier [3, 4].
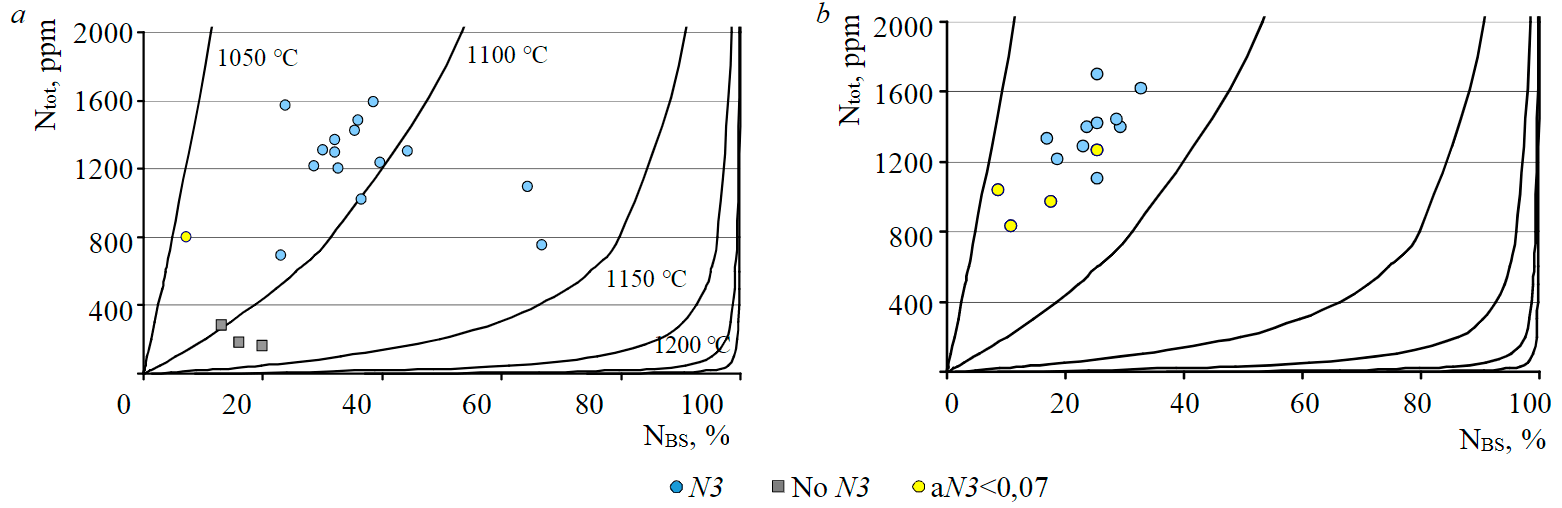
Fig.4. Distribution of diamond crystals of the octahedral habit of the Karpinsky-I (a) and Arkhangelskaya (b) pipes on the Taylor diagram with the separation of crystals by the presence of the N3 system in the absorption spectrum
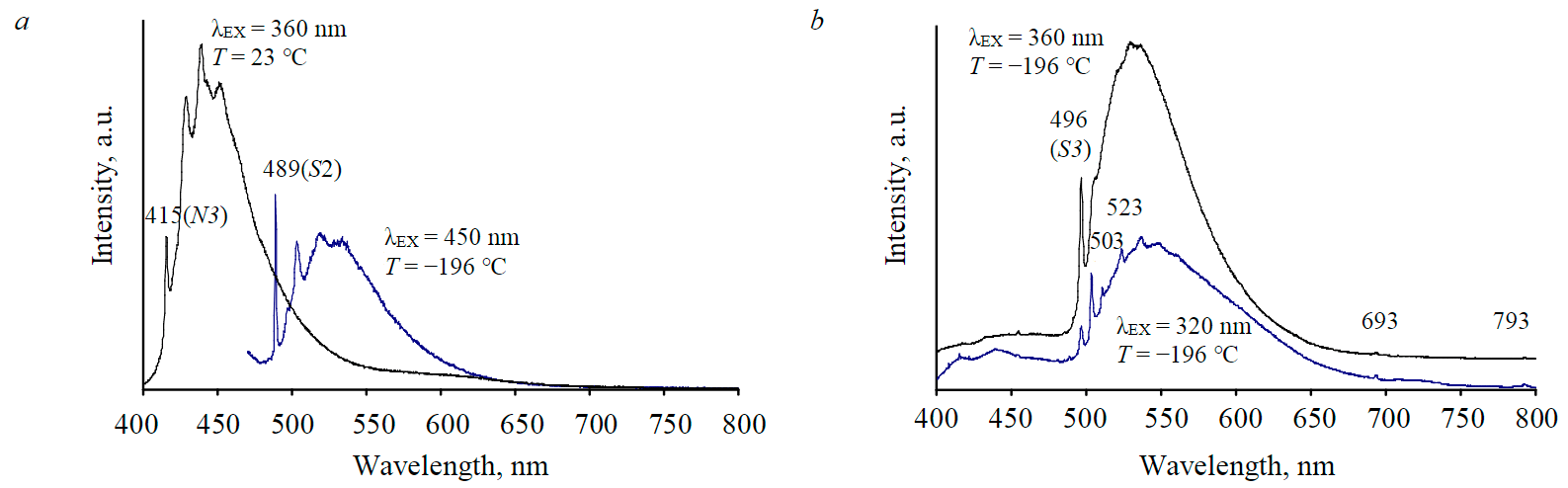
Fig.5. Luminescence spectra of crystals with the system N3 and S2(489 nm) (a), S1 and S3 (b) under 77 K and excitation wavelength λEX of 320, 360, 450 nm
Table 2
Occurrence frequency of luminescence systems in the spectra of diamond crystals of the Karpinsky-I and Arkhangelsk pipes
Pipe |
Luminescence system |
||||||||
N3 |
S3 |
S2 |
*H3 |
*693nm |
*700/787 nm |
*926nm |
*933nm |
*Н2 |
|
Recorded/Dominates**, % |
|||||||||
Karpinsky-I N3 in absorption |
98/67 |
74/31 |
64 |
12 |
23 |
58 |
41 |
14 |
2 |
No N3 in absorption |
39/5 |
94/92 |
45 |
30 |
80 |
93 |
85 |
1 |
3 |
Arkhangelskaya N3 in absorption |
98/83 |
51/17 |
89 |
15 |
27 |
62 |
35 |
25 |
2 |
No N3 in absorption |
54/27 |
76/73 |
68 |
34 |
90 |
95 |
62 |
6 |
5 |
|
|
|
|
|
|
|
|
|
|
*At 77 K. ** Under excitation at 360 nm.
Thus, the presence of the N3 system in the absorption spectrum is statistically related to other parameters: crystal morphology, thermal history, and nitrogen–hydrogen defect concentration. In addition, N3 defects are active in luminescence and can be detected in crystals without a 415 nm peak in the absorption PL spectra. In the IR absorption spectrum of such crystals, there may be no B and B’ absorption bands, but the N3 system is registered in the PL. This sign is an indicator of the beginning of transformation of A-B stage defects. In a diamond with a high hydrogen concentration, most of the N3 defects are transformed into N3V defects at the beginning of nitrogen transformation at the A-B stage. As a result, in cuboids and crystals of mixed habit containing a lot of hydrogen in micro inclusions,
N3 appears at a high degree of nitrogen aggregation. This effect explains the dominance of the blue luminescence of octahedra and the dissolution forms derived from them. It follows that dodecahedroids with N3 blue luminescence are dissolution-exposed individual crystals of octahedral habit.
The study confirms the connection between the detected characteristics of the diamond crystal and the dominant mechanism of its growth. All crystals grown predominantly by the tangential mechanism (octahedra and octahedroids) have an appreciable fraction of nitrogen in the form of B defects and are distinguished by the presence of the N3 system in the absorption and luminescence spectra. Crystals grown by the normal mechanism have a low degree of nitrogen aggregation and do not contain N3 defects. Rounded dodecahedroids as the limiting form of dissolution [36] can be derivatives of both octahedral crystals and cuboids or crystals of mixed habit. However, individual crystals of a mixed habit are most often transformed upon dissolution into tetrahexahedroids with relics of cuboid surfaces. The work [1] presents images of crystals weighing more than 50 carats from the Grib deposit. As can be seen in the illustration [1, Fig.3.15], only two flat-faced octahedra are yellow, the rest are colorless or slightly colored, being the crystals of transitional forms and with signs of strong dissolution. This observation also confirms for large crystals, the revealed pattern of the complex thermal history of octahedral crystals and the high concentration of N3 defects in them. Thus, even without a detailed study of crystal anatomy, it is possible to identify groups (populations) of crystals isolated by a set of features, which were formed under different conditions and differ in their post-growth history with respect to both natural annealing and the degree of dissolution.
Conclusions
Diamond crystals from the Arkhangelskaya and Karpinsky-I pipes have a unimodal distribution of nitrogen concentration of about 800-1100 and 900-1400 ppm, respectively, with a nitrogen aggregation degree of less than 20 %. The N3 system of nitrogen-vacancy defects is detected in the absorption spectra of 30 % of crystals from the Karpinsky-I pipe and 52 % of the Arkhangelskaya pipe crystals. The B’ band is in the spectra of 62 % and 78 % of samples from Karpinsky-I and Arkhangelskaya pipes, respectively. All octahedral habit crystals in these pipes have the N3 system in their absorption spectra.
Crystals of octahedral habit formed earlier than dodecahedroids and cuboids, but post-growth processes changed their morphology less. The Karpinsky-I pipe is unique in its high proportion of crystals with an extremely low natural annealing temperature. Determining the range of thermal stability of defects in such diamonds is a task for further research. Differences in the thermal history and degree of dissolution of crystals different in morphology indicate a multistage formation of diamond from deposits of the north of the East European Platform.
References
- Ustinov V.N., Neruchev S.S., Zagaynyy A.K. et al. Diamond potential in the north of the East European Platform. St. Petersburg: Nauka, 2021, p. 410 (in Russian).
- Palazhchenko O.V.Comprehensive studies of diamonds from deposits of the Arkhangelsk kimberlite province: generalization, genetic and practical consequences. Vestnik Moskovskogo universiteta. Seriya 4. Geologiya. 2008. N 2, p. 68-75.
- Garanin V.K., Kriulina G.Yu., Garanin K.V., Samosorov G.G.Arkhangelsk diamonds. New data.Moscow:
IP Skorokhodov V.A, 2018,p. 232 (in Russian). - Kriulina G.Yu., Vasilev E.A., Garanin V.K. Structural and mineralogical features of diamond from M.V. Lomonosov Deposit (Arkhangelsk Province): new data and their interpretation. Doklady Akademii nauk. 2019. Vol. 486. N 6, p. 695-698 (in Russian). DOI: 10.31857/S0869-56524866695-698
- Khachatryan G.K., Palazhchenko O.V., Garanin V.K. et al. Genesis of “nonequilibrium” diamond crystals from the Karpinsky-1 kimberlite pipe by CL and IR spectroscopy. Vestnik Moskovskogo universiteta. Seriya 4. Geologiya. 2008. N 2, p. 38-45 (in Russian).
- Pashkevich M.A., Alekseenko A.V. Reutilization Prospects of Diamond Clay Tailings at the Lomonosov Mine, Northwestern Russia. Minerals. 2020. Vol. 10. p. 17. DOI: 10.3390/min10060517
- 7.Alekseev I., Alekseev I.V. Zircon as a Mineral Indicating the Stage of Granitoid Magmatism at Northern Chukotka, Russia. Geosciences. 2020. Vol. 10. Iss, 5, p. 1-3. DOI: 10.3390/geosciences10050194
- Serebryakov E.V., Gladkov A.S. Geological and structural characteristics of deep-level rock mass of the Udachnaya pipe deposit. Journal of Mining Institute. 2021. Vol 250, p. 512-525. DOI: 10.31897/PMI.2021.4.4
- Hainschwang T., Fritsch E., Notari F., Rondeau B. A new defect centre in type Ib diamond inducting one phonon infrared absorption: the Y centre. Diamond and Related Materials. 2012. Vol. 21, p. 120-126. DOI: 10.1016/j.diamond.2011.11.002
- Howell D., Griffin W.L., Piazolo S. et.al.A spectroscopic and carbon-isotope study of mixed-habit diamonds: Impurity characteristics and growth environment. American Mineralogist.2013. Vol. 98. N 1, p. 66-77. DOI: 10.2138/am.2013.4179
- 11.Hainschwang T., Notari F., Fritsch E., Massi L. Natural, untreated diamonds showing the A, B and C infrared absorptions (“ABC diamonds”), and the H2 absorption. Diamond and Related Materials. 2006. Vol. 15. Iss. 10, p. 1555-1564. DOI: 10.1016/j.diamond.2005.12.029
- Gaillou E., Post J.E., Bassim N.D. et al. Spectroscopic and microscopic characterizations of color lamellae in natural pink diamonds. Diamond and Related Materials. 2010. Vol.19. Iss. 10, p. 1207-1220. DOI: 10.1016/j.diamond.2010.06.015
- 13.Skublov S.G., Rumyantseva N.A., Li Qiuli et al. Zircon Xenocrysts from the Shaka Ridge Record Ancient Continental Crust: New U-Pb Geochronological and Oxygen Isotopic Data. Journal of Earth Science. 2022.Vol. N 1, p. 5-16.
DOI: 10.1007/s12583-021-1422-2 - Melnik A.E., Skublov S.G., Rubatto D. et al. Garnet and zircon geochronology of the Paleoproterozoic Kuru-Vaara eclogites, northern Belomorian Province, Fennoscandian Shield. Precambrian Research. 2021. Vol. 353. N 106014, p. 1-16. DOI:10.1016/j.precamres.2020.106014
- Machevariani M.M., Alekseenko A.V., Bech J.Complex Characteristic of Zircon from Granitoids of the Verkhneurmiysky Massif (Amur Region). Minerals. 2021. Vol.11, p. 29. DOI: 10.3390/min11010086
- Shchukina E.V., Agashev A.M., Soloshenko N.G. et al.Origin of V. Grib pipe eclogites (Arkhangelsk region, NW Russia): geochemistry, Sm-Nd and Rb-Sr isotopes and relation to regional Precambrian tectonics. Mineralogy and Petrology. 2019. Vol.113. Iss. 5, p. 593-612. DOI: 10.1007/s00710-019-00679-7
- Levashova E.V., Skublov S.G., Popov V.A. Distribution of Trace Elements Controlled by Sector and Growth Zonings in Zircon from Feldspathic Pegmatites (Ilmen Mountains, the Southern Urals. Geosciences. 2021. Vol.11. N 7, p. 1-21.
DOI: 10.3390/geosciences11010007 - Shchukina E.V., Agashev A.M., Pokhilenko N.P. Metasomatic origin of garnet xenocrysts from the V.Grib kimberlite pipe, Arkhangelsk region, NW Russia. Geoscience Frontiers. 2016. Vol. 8. Iss.4, p. 641-651. DOI: 10.1016/J.GSF.2016.08.005
- Pharoe B.K., Evdokimov A.N., Gembitskaya I.M., Bushuyev Ya. Yu.Mineralogy, geochemistry and genesis of the post-Gondwana supergene manganese deposit of the Carletonville-Ventersdorp area, North West Province, South Africa. Ore Geology Reviews.2020. Vol. 120. N 103372, p. 1-16.DOI: 10.1016/j.oregeorev.2020.103372
- 20.Gulbin Y.L., Mikhalsky E.V. Modeling of mineral parageneses and thermobarometry of metavolcanic rocks of the Ruker Group in the Southern Prince Charles Mountains, East Antarctica. Geology of Ore Deposits. 2020. Vol. 62. N 7, p. 584-598.
- Abdel Gawad A.E., Ene A., Skublov S.G. et al. Trace Element Geochemistry and Genesis of Beryl from Wadi Nugrus, South Eastern Desert, Egypt. Minerals. 2022. Vol. 12, p. 22. DOI: 10.3390/min12020206
- Zedgenizov D.A., Kalinin A.A., Kalinina V.V. et al. The transformation features of impurity defects in natural diamonds of various habits under high P–T conditions. Doklady Earth Sciences. 2016. Vol. 466. Iss. l, p. 32-37 (in Russian). DOI: 10.1134/S1028334X16010086
- Zedgenizov D.A., Kalinin A.A., Kalinina V.V. et al. Nitrogen and hydrogen aggregation in natural octahedral and cuboid diamonds. Geochemical journal. 2017. Vol. 51. Iss. 2, p. 181-192. DOI: 10.2343/geochemj.2.0452
- Taylor W.R., Jaques A.L., Ridd M. Nitrogen-defect aggregation characteristics of some Australasian diamonds: Time-temperature constraints on the source regions of pipe and alluvial diamonds. American Mineralogist. 1990. Vol.75, p. 1290-1310.
- Zaitsev A.M. Optical Properties of Diamond: A Data Handbook. Berlin: Springer Verlag, 2001, p. 502.
DOI: 10.1007/978-3-662-04548-0 - Dishler B. Handbook of Spectral Lines in Diamond Volume 1: Tables and Interpretations. Springer Heidelberg Dordrecht London–New York, 2012, p. 470. DOI: 10.1007/978-3-642-22215-3
- Fritsch E., Hainschwang T., Massi L., Rondeau B.Hydrogen-Related Optical Centers in Natural Diamond. New Diamond and Frontier Carbon Technology. 2007. Vol. 17. N 2, p. 63-89.
- Goss J.P., Briddon P.R., Hill V. et al. Identification of the structure of the 3107 cm−1 H-related defect in diamond. Journal of Physics: Condensed Matter. 2014. Vol. 26. N 145801, p. 1-6. DOI: 10.1088/0953-8984/26/14/145801
- Kriulina G.Y., Garanin V.K., Vasilyev E.A. et al. New data on the structure of diamond crystals of cubic habitus from the Lomo-nosov Deposit. Moscow University Geology Bulletin. 2012. Vol. 67. N 5, p. 282-288 (in Russian). DOI: 10.3103/S0145875212050055
- Zinchuk N.N., Koptil' V.I. Typomorphism of diamonds of the Siberian platform. Moscow: Nedru, 2003, p. 603 (in Russian).
- Hainschwang T., Fritsch E., Notari F. et al.The origin of color in natural C center bearing diamonds. Diamond and Related Materials. 2013. Vol. 39, p. 27-40. DOI: 10.1016/j.diamond.2013.07.007
- Orlov Yu.L. Mineralogy of diamond. Moscow: Nauka, 1984, p. 170 (in Russian).
- Popova A.N., Sukhomlinov V.S., Mustafaev A.S. Accounting for Interelement Interferences in Atomic Emission Spectroscopy: A Nonlinear Theory. Applied Sciences. 2021. N 11, p. 1-17. DOI: 10.3390/app112311237
- Smit K.V., Shirey S.B., Stern R.A. et al.Diamond growth from C-H-N-O recycled fluids in the Zimbabwe lithosphere: evidence from CH4 micro-inclusions and δ13C-δ15N-N content in Marange mixed-habit diamonds. Lithos. 2016. Vol. 265, p. 68-81.
- Vasilev E.A., Klepikov I.V., Lukianova L.I. Сomparison of diamonds from the rassolninskaya depression and modern alluvial placers of the Krasnovishersky District (Ural region). Geology of Ore Deposits. 2019. Vol. 61. N 7, p. 598-605 (in Russian). DOI: 10.1134/S1075701519070134
- Khokhryakov A.F., Pal’yanov Yu.N. The evolution of diamond morphology in the process of dissolution: Experimental data. American Mineralogist. 2007. Vol. 92. N 5-6, p. 909-917. DOI: 10.2138/am.2007.2342