Transformation of grains of technological raw materials in the process of obtaining fine powders
Abstract
Crushing and grinding of materials are the most common processes of sample preparation for subsequent analysis and industrial application. Recently, grinding has become one of the most popular methods for producing nano-sized powders. This study investigates certain features of grain transformation in the process of grinding ores with finely dispersed valuable components in order to liberate them, as well as specifics of grinding metallurgical raw materials, metals and their mixtures for using them as initial components in metallurgical and other technological processes. We identified and examined structural and morphological changes of various powders after ultrafine grinding using the methods of scanning electron microscopy and X-ray microanalysis. It was proved that in order to take into account sample preparation artifacts during analytic studies of solid samples and development of technological processes, fine grinding of heterogeneous materials, especially if they contain metals, requires monitoring of the ground product by methods of scanning electron microscopy and X-ray microanalysis.
Introduction
Crushing and grinding are the processes that reduce the size of mineral fragments by breaking them under the influence of external forces, which overcome internal cohesive forces that bind together the particles of the solid matter. In principle, the crushing and grinding processes are similar to each other. The material is fed to grinding after medium or fine crushing. The main principle of the coarse grinding stage is compression, which is performed in such equipment as jaw breakers, conical and roller mills.
Fine grinding (less than 5 μm) mostly uses the mechanisms of collision and attrition and is performed in vibration mills, planetary mills and other specialized equipment. In a planetary mill, the sample and the grinding balls are placed into the grinding bowl; the bowls are fixed on the sun wheel and rotate around the disk center. The sample is successfully ground by means of whipping, crushing and grinding.
When selecting the mill type to obtain required particle size of the materials, it is not enough to monitor only granulometric composition of the sample; evolution of structural and morphological features of the grains needs to be taken into account as well.
It was established that after the coarse grinding stage, no morphological transformations occur in the sample, therefore the grinding product can be monitored by means of granulometry alone.
In case of fine grinding, structural and morphological transformations of the grains can often be observed, as well as changes in their physical and chemical characteristics. When powders with different grain diameters and chemical composition are mixed/ground together, either in a dry or in a wet state, finer powders can agglomerate on the surface of coarser particles and form a more or less stable layer (or patches), which leads to significant changes in the particle size distribution [18, 27, 29, 30].
From the technological perspective, four important processes take place during energy-intensive grinding of powders:
- crushing of dispersed particles to the size, limited by the amount of input energy for particle acceleration and collision;
- mechanical activation of obtained particles;
- particle interaction with the filling and other heterogeneous particles or mutual adhesion of the particles with the formation of an aggregated powder;
- changes of the particle phase state.
The purpose of this study is to examine the effect of different grinding modes and methods on the structural and morphological state of initial powders. Grinding is regarded as a physical and chemical process of exerting an influence on the sample that leads to formation of artifacts in its structural and chemical features, which in some cases reveal valuable characteristics of the sample.
Research methods. As a starting material for grinding, we used different powders of metals (Al, Ni, W, etc.) and their oxides, widely applied in mineral concentration and manufacturing of new materials.
Grinding was performed on a Retsch vibration ball mill MM-301 in the Shared Equipment Center at Saint Petersburg Mining University and on a TTD planetary mill MPP-1-2 in Technics and Technology of Disintegration, Ltd.
In order to identify structural and morphological changes, we examined initial and ground samples by means of scanning electron microscopy (SEM) and X-ray microanalysis. Two methods were used to mount the samples. The first one involved mixing the powders with epoxy and then polishing them sparingly with environmentally friendly diamond suspensions (this method was used to analyze grain sections). The second method implied fixing the powders on a conductive carbon adhesive tape. The vacuum evaporator JEE-400 was used to cover the specimen with a conductive carbon film.
Studies by means of SEM and X-ray microanalysis were performed on two microscopes with different sources of electron emission: tungsten cathode (scanning electron microscope JEOL JSM-6460LV) and Schottky cathode (thermal field emission scanning electron microscope JEOL JSM-7001F) in a wide range of acceleration voltage and current.
All the analytical studies were performed in the Shared Equipment Center at Saint Petersburg Mining University.
Research results
Let us consider the main SEM observations, associated with the interaction of heterogeneous sample particles with each other and with the mill filling.
In the process of grinding hard grains, the material on the internal surface of the filling wears off and contaminates the ground sample. For example, when grinding aluminum oxide in the steel pots of the vibration mill, the filling material in the final product is represented by individual particles no larger than 3 μm, as well as a thin layer of nano-sized particles adsorbed on the ground material (Fig.1). All the identified grains were monitored by means of energy dispersive spectroscopy (EDS), which demonstrated the appearance of iron lines (Kα at 6.3996 keV, Lα at 0.7048 keV) [21, 23, 25] in the X-ray spectrum during the analysis of the above-mentioned particles. EDS was performed on an Oxford Instruments spectroscope INCA X-act.
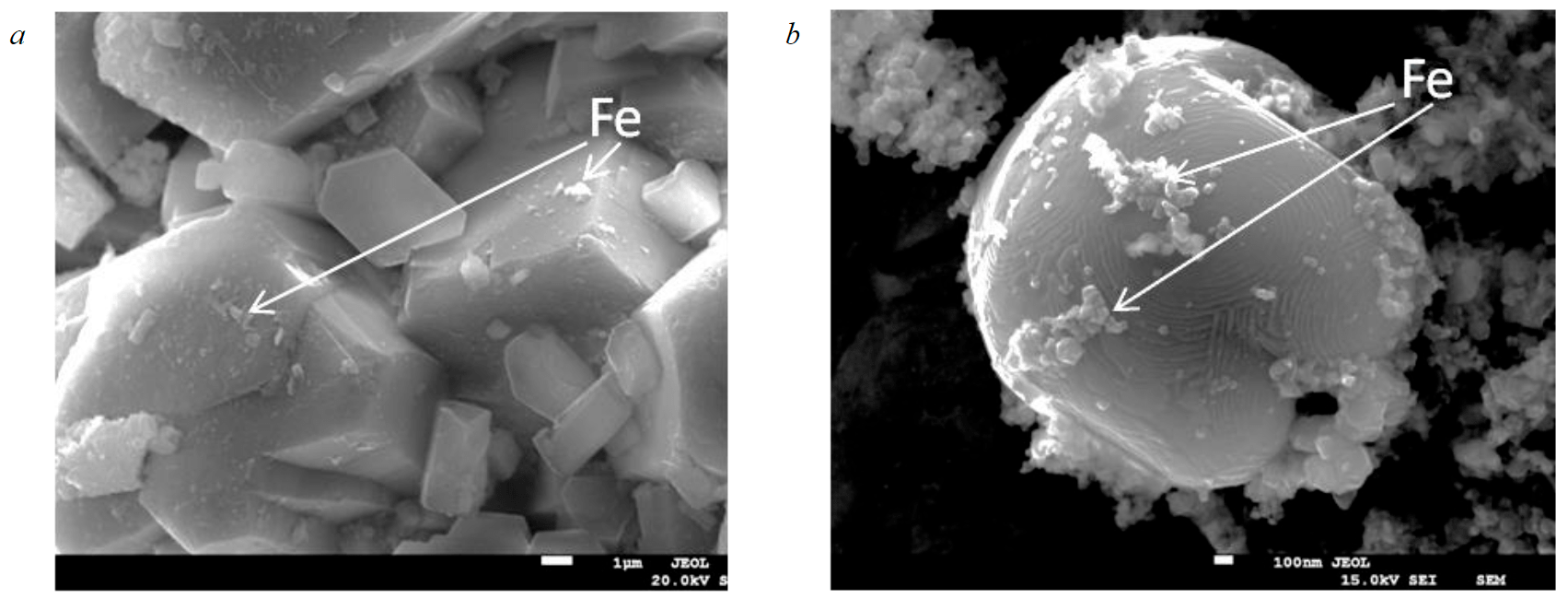
Fig.1. SEM images of aluminum oxide particles, contaminated with iron from the mill filling. Grinding modes for the sample: a – grinding ball diameter of 25 mm, vibration frequency of 20 Hz, milling time of 50 s; b – grinding ball diameter of 25 mm, vibration frequency of 30 Hz, milling time of 120 s
When grinding a heterogeneous sample, its components interact with each other. This process was observed during combined grinding of aluminum sample with nickel in a batch-type planetary mill. In the initial sample, the grains of aluminum were sized up to 80 μm; after grinding, they formed spherical particles up to 4 μm in size, which were sorbed on the surface of nickel (this was confirmed by EDS analysis – in the X-ray spectrum, we observed a line of aluminum Кα at 1.4866 keV, the intensity of which increased depending on the share of this surface layer in the analyzed range) (Fig.2, a, b). When plastic metals, such as lead, aluminum, etc., are ground with other components, the process is characterized by formation of a layer on the particle surface of these components, as well as the surface of the filling (Fig.2, c, f).
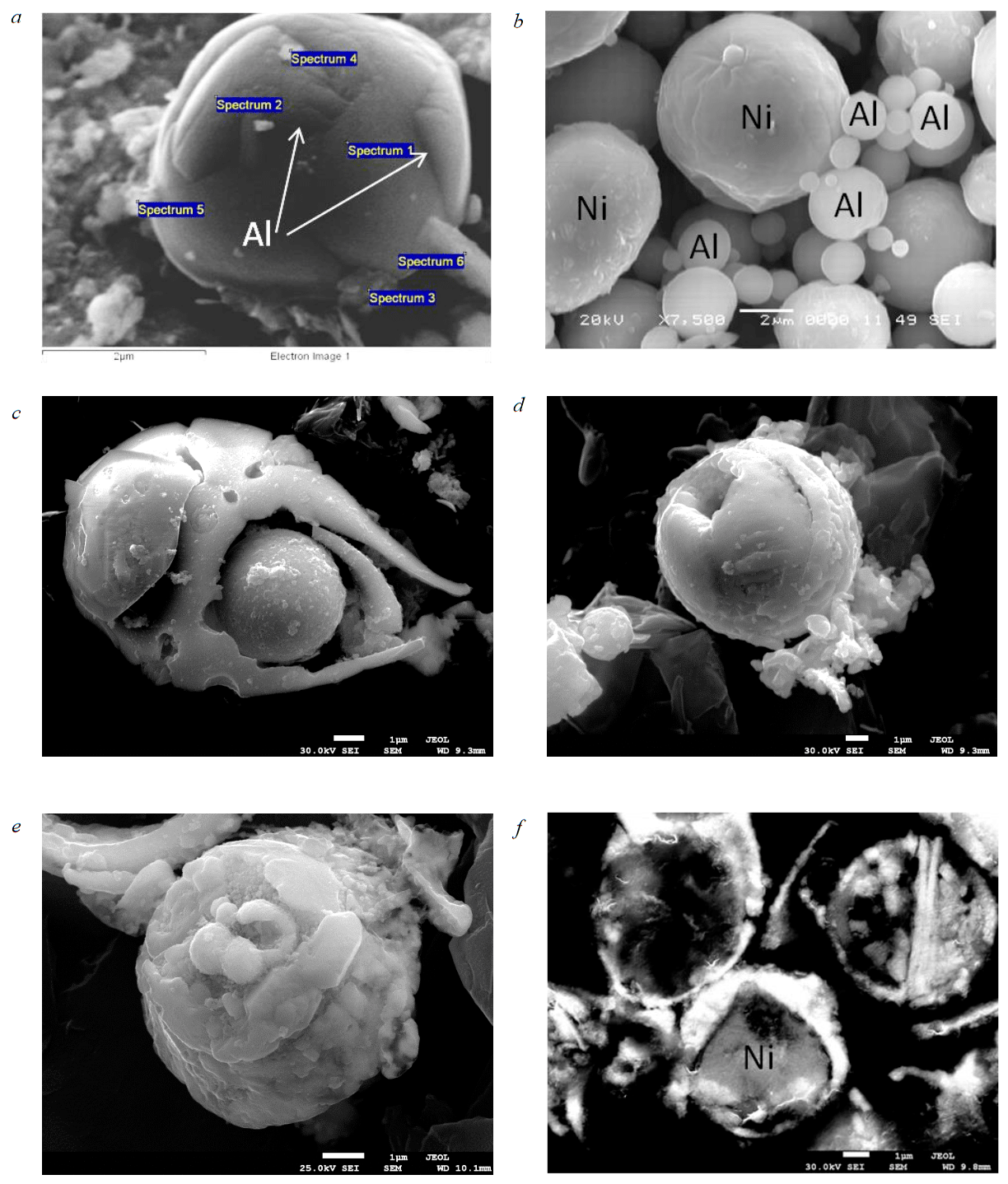
Fig.2. SEM images: a, b – large spherical particles of nickel, covered with aluminum crust, surrounded by separate aluminum particles (grinding mode – centrifugal force of 20 g, ball diameter of 10 mm, rotation speed of 600 rpm, milling time of 2 min); c-e – small spherical particles of nickel with adhered crust; f – section of small spherical particles of nickel with adhered crust (grinding mode – centrifugal force of 20 g, ball diameter of 8 mm, rotation speed of 700 rpm, milling time of 4 min)
Energy-intensive grinding can also be accompanied by changes in the defect structure and external shape of the crystals. For example, changes in the phase state of the particles can be illustrated by the restoration of crystallographic faces of tungsten grains with the formation of new shapes, associated with particle size reduction. As seen from the Fig.3, the crystal shape is close to rhombic dodecahedron, which corresponds to the cubic lattice of tungsten [3, 9, 12, 15]. Performed EDS analysis confirmed single-element composition of the grains. Tungsten was identified by Lα and Mα lines, but as Mα at 1.7750 keV coincides with the silicon line Kα at 1.7398 keV, we used the Lα line at 8.3970 keV [5, 21, 23, 25].
Fine grinding is often accompanied by mechanical activation of the matter, after which its physical properties undergo significant changes. In some cases, this can be used not only to take into account grinding artifacts, but also to develop new methods of enhanced metal recovery [1, 8, 11, 13, 14, 17, 26, 28].
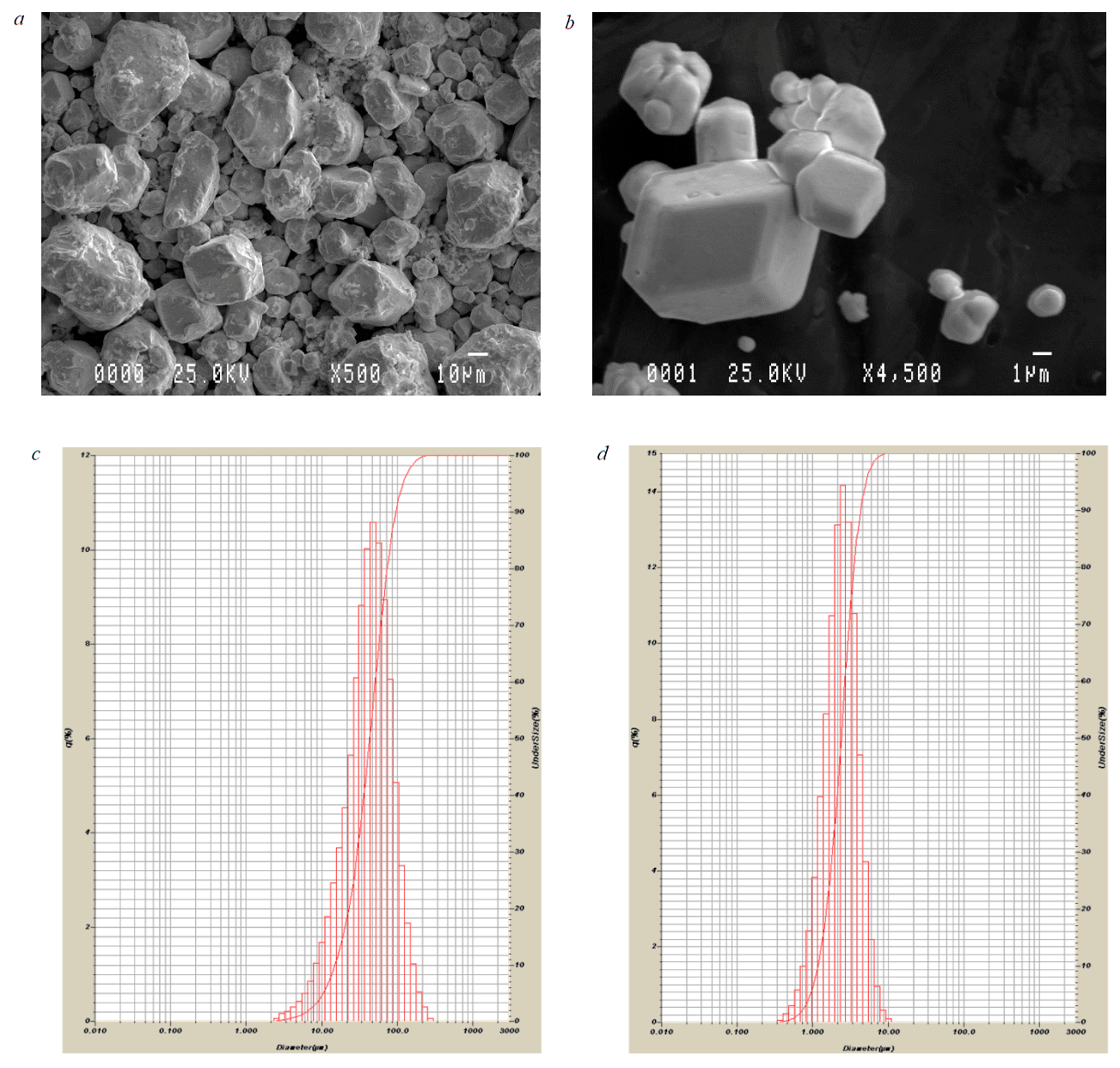
Fig.3. SEM image of initial tungsten powder (a), tungsten crystals after milling (b) (grinding mode – centrifugal force of 28 g, ball diameter of 10 mm, rotation speed of 700 rpm, milling time of 2.1 min); histogram of particle size distribution in the initial tungsten powder (c) and in the sample after milling (d)
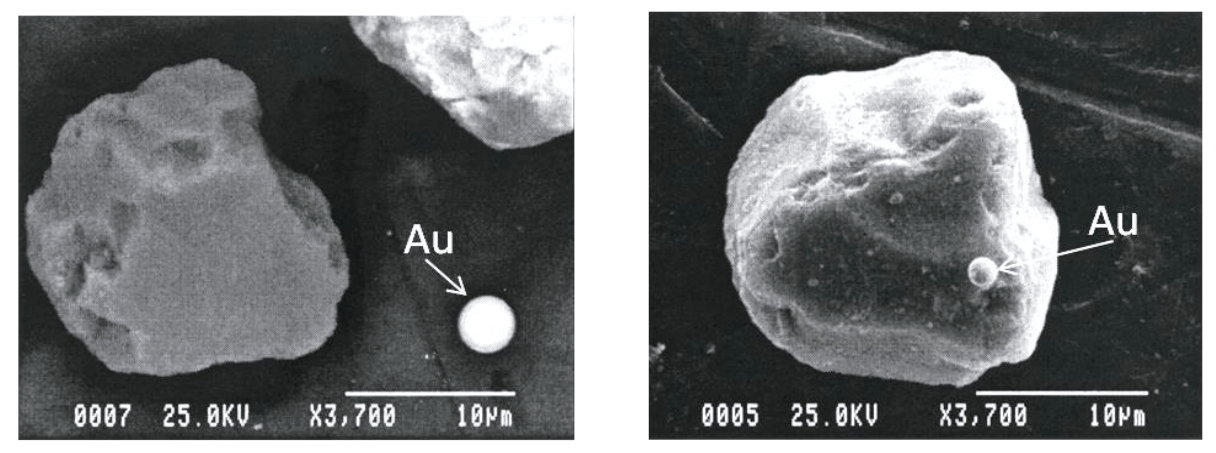
Fig.4. SEM images of spherical gold particles in the sulfide ore after crushing
Analytical studies of solid samples using bulk analysis methods, such as X-ray fluorescence spectroscopy, powder X-ray diffractometry, atomic emission spectroscopy, etc. imply preliminary grinding of samples to a specified particle size. For example, to prepare the samples for powder diffractometry, they need to be ground and reground to 1-3, 5-7, 10 μm (these size ranges are selected at the stage of method development depending on the crystallographic class, morphological features of the grains and the presence of cleavage) [2, 4, 6, 7, 10, 16, 19, 20, 22]. Most commonly, the samples are ground in a vibration mill and then reground with an agate mortar. The particle size is monitored by means of a laser granulometer. However, during long-term regrinding of polymineral mixtures of different hardness, it is important not to grind certain phases to the X-ray amorphous state; for example, this can happen in the samples where quartz and harder particles occur together with clay particles and gypsum. Similar degradation of initial substances can take place in the presence of water-soluble and aqueous crystals, as in the agate mortar a high degree of grinding is achieved by using ethanol as a grinding media. This requires monitoring of the initial and ground sample using SEM in order to adjust sample preparation and, as an option, to perform mineral separation during one of the grinding stages.
In case of finely dispersed nano-sized particles, long-term mixing can cause their agglomeration into spherical components. The same process leads to formation of spherical crystal phases after grinding – for example, in the process of grinding sulfide ore that contains Au grains 1-3 μm in diameter (Fig.4).
Conclusion
In the process of energy-intensive grinding of ores and technological materials, associated with the formation of particles greater than 3 μm, the particles are spherically shaped, sometimes with traces of former crystallographic pattern.
Structural and morphological changes of the grains, obtained in the process of fine energy-intensive grinding of heterogeneous materials, especially if they contain metals, in vibration and planetary mills, demonstrate the necessity to monitor the final product by means of scanning electron microscopy and X-ray microanalysis. This approach will make it possible to take into account artifacts generated during preparation of solid samples for analytical studies and will allow to make necessary adjustments in the course of developing new technological processes.
References
- Alekseeva E.A., Brichkin V.N., Nikolaeva N.V. Technical and mineralogical evaluation of low quality bauxite washability (an example of North Onega deposit). Journal of Mining Institute. 2013. Vol. 202, p. 231-234 (in Russian).
- Afanasova A.V., Rudenko A.P. Research of mechanical activation of technical hydrolysis lignin as a component of fuel briquette. Сhemistry of plant raw material. 2020. N 1, p. 355-363. DOI: 10.14258/jcprm.2020016678 (in Russian).
- Burkhanov G.S. Metal Single Crystals. Moscow: ELIZ, 2002, p. 312 (in Russian).
- Ivanov O.K. The geological method definition of the equilibrium form crystals. Uralian Geological Journal. 2012. N 6 (90), p. 39-48 (in Russian).
- Mustafaev A.S., Pshchelko N.S., Morin A.V., Tsyganov A.B. The measurements of emission parameters of tungsten thermo cathodes in plasma energetic equipments. Journal of Mining Institute. 2010. Vol. 187, p. 91-97 (in Russian)
- Arsentev V.A., Marmandyan V.Z., Samukov A.D., Kabirov A.M. Innovate technologies in waste of extraction and nonmetallic raw materials processing. Journal of Mining Institute. 2012. Vol. 198, p. 219-222 (in Russian).
- Kuskova V.B., Kuskova Y.V. Complex use of iron ores in iron and steel industry. Journal of Mining Institute. 2013. Vol. 202, p. 111-114 (in Russian).
- Lvov V.V., Chitalov L.S. Modern trends in the design of comminution processes and equipment for non-ferrous metals ores Tsvetnye Metally. 2020. N 10, p. 20-26. DOI: 10.17580/tsm.2020.10.03 (in Russian).
- Novokreshchionov V.V., Rodiakina R.V. The process of weld metal structure formation during the electron beam welding of tungsten single crystals. Bulletin of Moscow power engineering institute. 2013. N 4, p. 19-24 (in Russian).
- Sizyakov V.M., Vlasov A.A., Bazhin V.Y., Gembitskii V.V. Factors of alumina dust formation in primary aluminum production. Journal of Mining Institute. 2012. Vol. 198, p. 207-212 (in Russian).
- Sizyakov V.M., Nazarov Yu.P., Brichkin V.N., Sizyakova E.V. Processing of aged dumped tailings of apatite-nepheline ores flotation. Obogashchenie Rud. 2016. N 2, p. 33-39. DOI: 10.17580/or.2016.02.06 (in Russian).
- Streletskii A.N., Portnoi V.K., Leonoviv A.V. Mechanical and Chemical Activation and Caking of Tungsten and its Mixture with Copper and Nickel. Khimiya v interesakh ustoichivogo razvitiya. 2002, p. 245-254 (in Russian).
- Tikhonov N.O., Gospodarikov A.P. The Gaisky concentrating plant semiautogenous grinding complex optimal operating regime justification. Obogashchenie rud. 2015. N 5, p. 3-8. DOI: 10.17580/or.2015.05.01 (in Russian).
- Trushko V.L., Kuskov V.B., Kuskova Y.V. Integrated processing of highgrade iron ores. Obogashchenie rud. 2014. N 1, p. 39-43 (in Russian).
- Balan V.Z., Bochko A.V., Zholud V.V. et al. Ultrasound Analysis of Tungsten Single Crystals of Different Purity Degree. Metally. 2001. N 1, p. 77-81 (in Russian).
- Fadina A.V., Andreev E.E., Lvov V.V. Pre-concentration of raw materials using sensor- based sorting. Journal of Mining Institute. 2013. Vol. 206, p. 139-142 (in Russian).
- Aleksandrova T., Elbendari A., Nikolaeva N. Beneficiation of a Low-grade Phosphate Ore Using a Reverse Flotation Technique. Mineral Processing and Extractive Metallurgy Review. 2020, p. 1-6. DOI: 10.1080/08827508.2020.1806834
- Arole V.M., Munde S.V. Fabrication of nanomaterials by top-down and bottom-up approaches. JAAST: Material Science. Special Issue. 2014. Vol. 1. Iss. 2, p. 89-93.
- Groundwater H., Twardowski M.S., Dierssen H.M. et al. Determining size distributions and composition of particles suspended in water: A new SEM–EDS protocol with validation and comparison to other methods. Journal of atmospheric and oceanic technology. 2012. Vol. 29. Iss. 3, p. 433-449. DOI: 10.1175/jtech-d-11-00026.1
- Gembitskaya I.M.,Kotova E.L., Abdrakhmanov I.A. et al. Insights into the applicability of the X-Ray phase quantification supported by SEM for the rock-forming silicates minerals in the basaltic lava flows. EPITOANYAG-Journal of silicate based and composite materials. 2020. Vol. 72. N 5, p. 174-177. DOI: 10.14382/epitoanyag-jsbcm.2020.29
- 21. Giurlani W., Innocenti M., Lavacchi A. X-ray microanalysis of precious metal thin films: thickness and composition determination. Coatings. 2018. Vol. 8. Iss. 2, p. 84. DOI: 10.3390/coatings8020084
- Ichikawa S., Nakamura T. Approaches to solid sample preparation based on analytical depth for reliable X‐ray fluorescence analysis. X-ray spectrometry. 2016. Vol. 45, Iss. 6, p. 302-307. DOI: 10.1002/xrs.2700
- Holzer G., Fritsch M., Deutsch M., Hartwig J., Forster E. Kα1,2 and Kβ1,3 x-ray emission lines of the 3d transition metals. Physical Review A. 1997. Vol. 56. Iss. 6, p. 4554-4568. DOI: 10.1103/PhysRevA.56.4554
- Duryagina A., Heide G., Talovina I. et al. Mineralogical and morphometric aspects of the rock analysis as the basis for choosing a scheme for ore preparation. VIII International Scientific Conference “Problems of Complex Development of Georesources” (PCDG 2020), 8-10 September, 2020, Khabarovsk, Russian Federation. E3S Web Conferences. 2020. Vol. 192. N 02023 DOI: 10.1051/e3sconf/202019202023
- Newbury D.E., Ritchie N.W.M. Performing elemental microanalysis with high accuracy and high precision by scanning electron microscopy/silicon drift detector energy-dispersive X-ray spectrometry (SEM/SDD-EDS). Journal of Materials Science. 2015. Vol. 50, p. 493-518. DOI: 10.1007/s10853-014-8685-2
- Litvinova N.M., Gurman M.A., Rasskazova A.V., Alexandrova T.N. Research of mineralogical – Technological peculiarities of refractory gold-bearing ores. Eurasian Mining. 2014. N 1, p. 28-33.
- Priyadarshana G., Kottegoda N., Senaratne A. et al. Synthesis of Magnetite Nanoparticles by Top-Down Approach from a High Purity Ore. Journal of Nanomaterials. 2015. Vol. 16. Iss. 1, p. 1-8. DOI: 10.1155/2015/317312
- Vasilev B.U., Malkova Y.M., Mardashov D.V. Control System of a Complete Electric Drive of a Mill for Grinding Gold-containing Ores. IEEE NW Russia Young Researchers in Electrical and Electronic Engineering Conference, 27-30 January, 2020, St. Petersburg and Moscow, Russian Federation. IEEE, 2020, p. 936-941. DOI: 10.1109/EIConRus49466.2020.9039468
- Weßling B. Critical Shear Rate – the Instability Reason for the Creation of Dissipative Structures in Polymers. Zeitschrift Für Physikalische Chemie. 1995. Vol. 191. Part 1, p. 119-135. DOI: 10.1524/zpch.1995.191.part_1.119
- 30. Yadav T.P., Yadav R.M., Singh D.P. Mechanical Milling: a Top Down Approach for the Synthesis of Nanomaterials and Nanocomposites. Nanoscience and Nanotechnology. 2012. Vol. 2. Iss. 3, p. 22-48. DOI: 10.5923/j.nn.20120203.01