Results of complex experimental studies at Vostok station in Antarctica
- 1 — Ph.D. Scientific Director of the Laboratory Empress Catherine II Saint Petersburg Mining University ▪ Orcid
- 2 — Research Engineer Empress Catherine II Saint Petersburg Mining University ▪ Orcid
- 3 — Ph.D. Associate Professor Empress Catherine II Saint Petersburg Mining University ▪ Orcid
- 4 — Ph.D. Head of Department Empress Catherine II Saint Petersburg Mining University ▪ Orcid
- 5 — Ph.D. Leading Engineer Empress Catherine II Saint Petersburg Mining University ▪ Orcid
- 6 — Assistant Empress Catherine II Saint Petersburg Mining University ▪ Orcid
- 7 — Leading Engineer Empress Catherine II Saint Petersburg Mining University ▪ Orcid
- 8 — Research Engineer Empress Catherine II Saint Petersburg Mining University ▪ Orcid
Abstract
Scientific research in the area close to the Russian Antarctic station Vostok has been carried out since its founding on December 16, 1957. The relevance of work to study the region is steadily increasing, which is confirmed by the Strategy for the Development of Activities of the Russian Federation in the Antarctica until 2030. As part of the Strategy implementation, Saint Petersburg Mining University solves the comprehensive study issues of the Vostok station area, including the subglacial Lake Vostok, related to the development of modern technologies and technical means for drilling glaciers and underlying rocks, opening subglacial reservoirs, sampling water and bottom sediments, as well as carrying out comprehensive geological and geophysical research. For the successful implementation of the Strategy, at each stage of the work it is necessary to identify and develop interdisciplinary connections while complying with the requirements for minimizing the impact on the environment. During the season of the 68th Russian Antarctic Expedition, the staff of the Mining University, along with the current research works , began research of the dynamic interactions between the forces of the Earth, from the deepest depths to the surface glacier. Drilling and research programs have been completed. The drilling program was implemented jointly with colleagues from the Arctic and Antarctic Research Institute at the drilling complex of the 5G well. The research program included: shallow seismic studies, core drilling of snow-firn strata, study of the snow-firn strata petrostructural features, studies of cuttings collection filters effectiveness when drilling snow-firn strata and the process of ice destruction in a reciprocating rotational method, bench testing of an acoustic scanner. As a result of drilling in 5G well at the depth range of 3453.37-3534.43 m, an ice core more than 1 million years old was obtained.
Introduction
For more than 65 years, the Antarctic Vostok Station area attracts the attention of researchers in the field of geosciences. Since the station foundation in 1957, Soviet and Russian scientists have carried out continuous work to study the atmosphere, ice dome and underlying rocks of the Antarctic continent. In 1970, scientists from the Leningrad Mining Institute (now the Empress Catherine II Saint Petersburg Mining University), together with the Arctic and Antarctic Research Institute (AARI) staff, began drilling deep holes in the ice, which continues to this day [1]. Nowadays, more than 13000 m of deep wells have been drilled at Vostok station, the core material of which is a source of unique primary information when conducting fundamental research in the field of glaciology, paleoclimatology, geology, and microbiology. Owing to the ice core from the Vostok station that a continuous sequence of data on changes in the gas composition of the atmosphere and climate over the past 420 thousand years was established [2]. The study of mineral inclusions found in ice cores, the size of which reaches 8 mm, allowed us to draw conclusions about the geological composition of subglacial rocks of Central Antarctica [3].
The rapid progress of drilling operations in the mid-90s and the proximity of one of the most important scientific achievements of the 21st century – the opening of the subglacial Lake Vostok – dictated the need for comprehensive geophysical research in the area of the Vostok station. As a result of borehole research, Russian geophysicists established the propagation speed of seismic and electromagnetic waves in ice, and also measured the thickness of the glacier at the area of the supposed lake opening [4]. In the course of work to solve the problems of mapping and studying the deep structure in the Lake Vostok area, carried out jointly with scientists from Italy and the USA, data on the area, volume, depth, and altitude of the lake were established [5].
Despite long-term research, Antarctica remains the least studied continent, the need and relevance of scientific research of which is enshrined in the Government of the Russian Federation order dated June 30, 2021 N 1767-r “On approval of the action plan for the implementation of the Strategy for the Development of Activities of the Russian Federation in Antarctica until 2030”. Saint Petersburg Mining University takes part in the implementation of the 21st Event “Comprehensive studies of the subglacial Lake Vostok and the Earth’s paleoclimate in the area of the Russian Antarctic station Vostok”, within the framework of which it is necessary to solve a range of research, development and organizational tasks.
As part of the 68th Russian Antarctic Expedition season, the Mining University staff, along with current research works, began research into the dynamic interactions between the forces of the Earth, from the deepest depths to the glacier surface. These studies are aimed at confirming new knowledge obtained by scientists [6], and concern the study of the deepest layers of our planet behavior using seismic waves in Antarctica. A joint drilling program with AARI and our own research programs were completed, the main sites of which are shown in Fig.1.
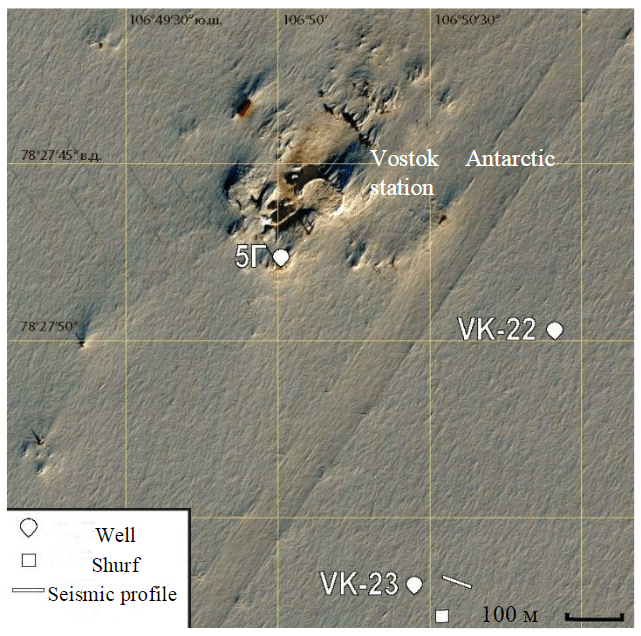
Fig.1. Mining University research sites during the RAE 68th season in the area of Vostok station (www.google.com/maps)
Drilling of a 5G-5 deep well in order to obtain an ancient ice core was carried out at the drilling complex named after Professor B.B. Kudryashov.
The research program aimed at studying the snow-firn strata and creating new technical means for drilling glaciers included the following work: shallow seismic research; core drilling of snow-firn strata; study of the snow-firn strata petrostructural features, studies of cuttings collection filters effectiveness when drilling snow-firn strata; and the process of ice destruction in a reciprocating rotational method; bench testing of an acoustic scanner.
Experiments related to the creation of new technical means for drilling glaciers were carried out in the laboratories of the 5G well drilling complex, taking into account the thermobaric conditions of the Vostok station. Particular attention was paid to establishing interdisciplinary connections between well drilling, geophysical and petrophysical studies of the glacier.
Mining University staff, together with colleagues from the AARI, performed 90 trips when drilling ice in 5G-5 well, which were distributed according to the intended purpose as follows: 57 – drilling; 10 – expansion; 9 – kerosene extraction; 7 – freon delivery; 7 – geophysical studies of the well.
As a result of drilling operations, 81.06 m of core material was obtained from the depth range of 3453.37-3534.43 m with an average trip of 1.42 m and a 100% core recovery. As a result of drilling 5G-5 well, a core more than 1 million years old was extracted [2]. A set of borehole geophysical studies was carried out in 5G well (BGS), which was carried out before and after the completion of drilling operations and was including thermometry, barometry, cavernometry, and inclinometry. BGS data made it possible to assess changes in the technical condition of the well that occurred after the season of the 67th Russian Antarctic expedition (RAE), to plan and carry out a set of measures (expansion, freon delivery, kerosene extraction) necessary to ensure trouble-free operation in the well.
To study the characteristics of the snow-firn strata of the glacier, a shallow well VK-23 was drilled with the core and cuttings material selection and a pit 3 m deep was dug. The pit was located 100 m northeast of the VK-23 well and was oriented to the east. After cleaning and sketching the pit, a measuring tape was installed on its working wall, and employees of the Laboratory of Climate and Environmental Change of the AARI (LCEA) and the Mining University carried out a stratigraphic description of the working wall, took samples to analyze the chemical composition, the content of cosmogenic and stable isotopes, measured the density of snow -firn strata.
Shallow seismic surveys
In Antarctica, one of the most popular methods of geophysical research is seismic exploration, which makes it possible to determine the thickness of the glacier, subglacial relief, water layer thickness, the depth of seismic geological boundaries, their dip and extension, and using geological data [7, 8], establish the geological nature of the identified boundaries [5]. The major difficulties in performing seismic sounding arise due to the presence of a thick near-surface snow-firn layer in which the acoustic wave is attenuated [9, 10]. At the same time, the waves that arise after the explosion in the snow-firn layer and propagate along its surface are characterized by high intensity and weakly attenuate with distance from the source of excitation, which masks the target reflections [4, 11]. Despite the large volume of research carried out, the elastic waves propagation in the snow-firn horizon study is an urgent task, the solution of which was aimed at experimental and methodological work during the season of the 68th RAE at Vostok station. Initially, the performance of seismic equipment was tested in the area of the Progress station, and then experimental and methodological work was carried out in the area of the Vostok station.
Methods
The purpose of the experimental and methodological work was to study the possibility of conducting seismic exploration of the snow-firn strata in the climatic and mining-geological conditions of the Vostok station area using the cableless telemetric seismic recording system (CTSRS) “SCOUT” (developed by “SKB SP” AO, Saratov). The work included: determining the average operating time of autonomous battery modules in conditions of low negative temperatures without recharging; testing methods for working with “SCOUT” CTSRS in Antarctica; assessment of the convenience of field work with a tablet computer for controlling the “SCOUT” CTSRS in conditions of low negative temperatures.
At the Vostok station, the performance of cableless autonomous recorders (CAR) was assessed in conditions of negative temperatures, which was carried out at a temperature of –50 °C for 55 hours. It has been established that the maximum intensity of discharge of CAR batteries occurs in the first 6 hours and amounts to 10-11 % (Fig.2). The discharge time of CAR batteries at a temperature of –50 °C is in the range of 80-120 hours and is 30-50 % of their maximum recording duration in off-line mode in normal conditions.
The source of excitation of elastic waves was the blow of a sledgehammer on an anvil (metal die). During experimental and methodological work at the Progress station, a steel rectangular block such a die are its large mass and deep penetration into loose rocks, including snow, during impact, which makes seismic work difficult. During experimental and methodological work at the Vostok station, steel round pancakes were first used as dies, but they split from the blows of a sledgehammer and were also buried (Fig.3, b, c).
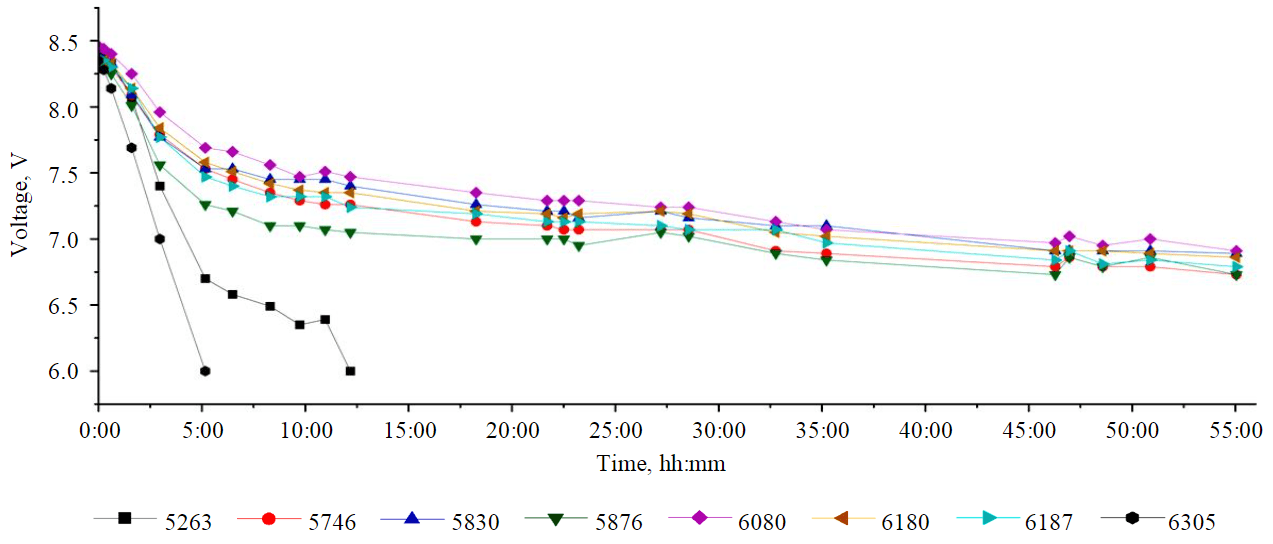
Fig.2. Graph of changes in the charge of CAR batteries at an ambient temperature of –50 °C, line number – CAR serial number
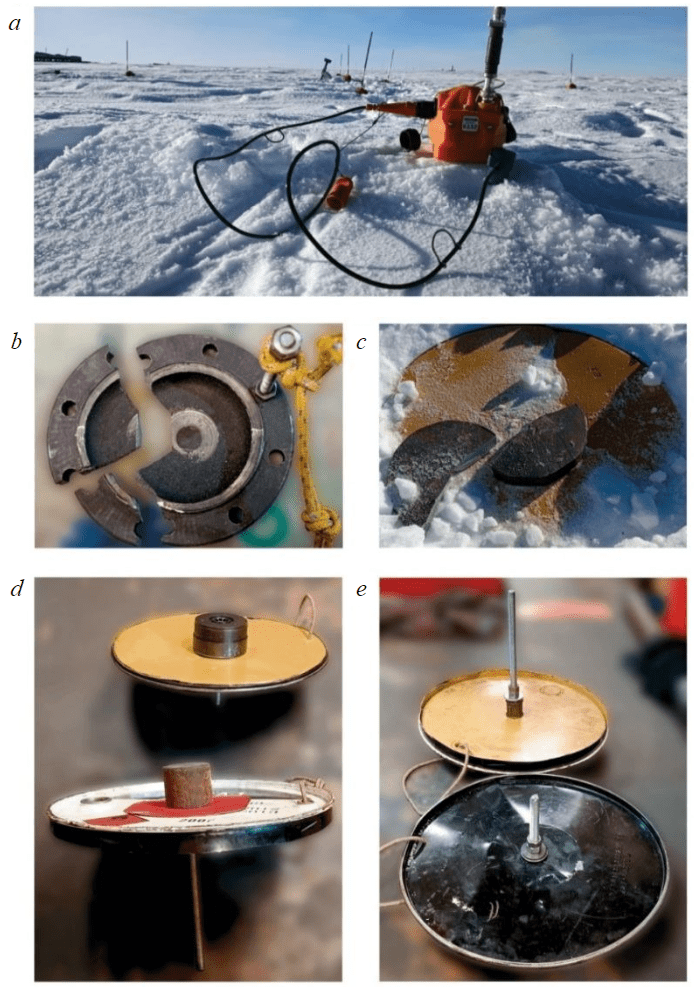
Fig.3. Carrying out experimental and methodological work near Vostok station (a). Die designs: b – split die 12 mm thick; c – 30 mm thick; d – dies 110 mm thick (upper) and 90 mm (lower) with welded steel fingers and fixed steel circles with a diameter of 600 mm; e – dies with a thickness of 110 mm (upper) and 90 mm (lower) (bottom view)
Based on the results of the work carried out, a technical solution was implemented aimed at eliminating shortcomings in the design of the elastic waves excitation sources tested (Fig.3, d, e). Two steel blanks with a thickness of 90 and 110 mm and a diameter of 146 and 110 mm, respectively, were chosen as dies. To avoid deepening of the dies, a threaded steel pin was welded to the lower end of each blank, onto which a steel circle 2 mm thick and 600 mm in diameter was placed and pressed with nuts. The new design of elastic waves excitation sources made it possible to ensure a tight connection of its elements, eliminating the appearance of additional vibrations, and to increase the contact area of the source with the snow surface, which prevents the rams from deepening during impacts during geophysical research (Fig.3, a).
Results discussion
During the experimental and methodological work, seven profiles were carried out, of which: three – cold snow-firn sediments (Vostok station); two – warm snow-firn sediments (Progress station); two – loose terrigenous sediments (Progress station) (Table 1).
Table 1
Shallow seismic research in Antarctica during the of RAE 68th season
Parameter |
Values |
||||||
Work area |
Progress 09.01.2023 |
Vostok 06.02.2023 |
|||||
Air temperature, °С |
–6 |
–45 |
|||||
Atmospheric pressure, mm Hg |
745 |
470 |
|||||
Wind, m/s |
0 (alm) |
6-8 |
|||||
Profile number |
1 |
2 |
3 |
4 |
5 |
6 |
7 |
Arrangement(P – profile, C – cross) |
P |
P |
P |
P |
P |
P |
C |
Soil type |
Wet (warm) snow |
Terrigenous sediments |
Cold snow-firn sediments |
||||
Receiving points spacing, m |
2 |
10 |
2 |
10 |
5 |
10 |
5 |
Excitation points pitch, m |
2 |
10 |
2 |
10 |
5 |
10 |
5 |
Sampling step, ms |
2 |
2 |
2 |
2 |
2 |
2 |
2 |
Signal recording length, s |
3 |
3 |
3 |
3 |
3 |
3 |
3 |
Number of PP, pcs |
7 |
7 |
7 |
7 |
7 |
7 |
7 |
Obtained seismic data processing was carried out at the Department of Geophysics of the Mining University by Associate Professor G.D.Gorelik for profile N 6 using the refracted waves method (RWM). Observation geometry has been introduced into the trace headings: coordinates of receiving points and generation points, spacings were calculated. For each seismic trace, the amplitudes were adjusted using the SeisPro seismic data processing software package, then the first arrival time was picked (Fig.4, a). The resulting hodographs were exported to the “Easy Refraction” module, which involves manually identifying the fractures of the first arrival hodographs and estimating wave propagation velocities directly by the processing geophysicist. As a result of refractory processing, a refractive boundary was established at a depth of about 5 m (Fig.4, b), which is consistent with well drilling data and corresponds to the depth of snow-firn layer compaction. The wave speed was determined in the near-surface part of the section (depths 0-5 m), which is in the range of 450-600 m/s, and in the compacted part (depths greater than ~ 5 m) – about 1350 m/s.
Summary
Analysis and interpretation of geophysical research data in Antarctica, obtained during the RAE 68th season, allow us to draw the following conclusions:
- In the conditions of East Antarctica at an ambient temperature of –50 °C, the “SCOUT” CTSRS is operational for 4-5 days and allows you to record seismic vibrations using electrodynamic seismic receivers.
- The depth of seismic research was 10 m, which is 4-5 times less than the length of the profile placement.
- In the field conditions of Antarctic (Progress and Vostok stations), the built-in modules of the GPS navigation system turned out to be effective – the CAR was connected to the GPS system automatically in 2-5 seconds.
- When testing the geophysical complex, significant deviations (more than 20 %) were recorded in the readings of temperature sensors at a temperature of –50 °C.
- The information obtained during experimental and methodological work made it possible not only to assess the physical properties of the snow-firn layer, but also to establish the necessary set of measures to continue seismic research in the area of Vostok station.
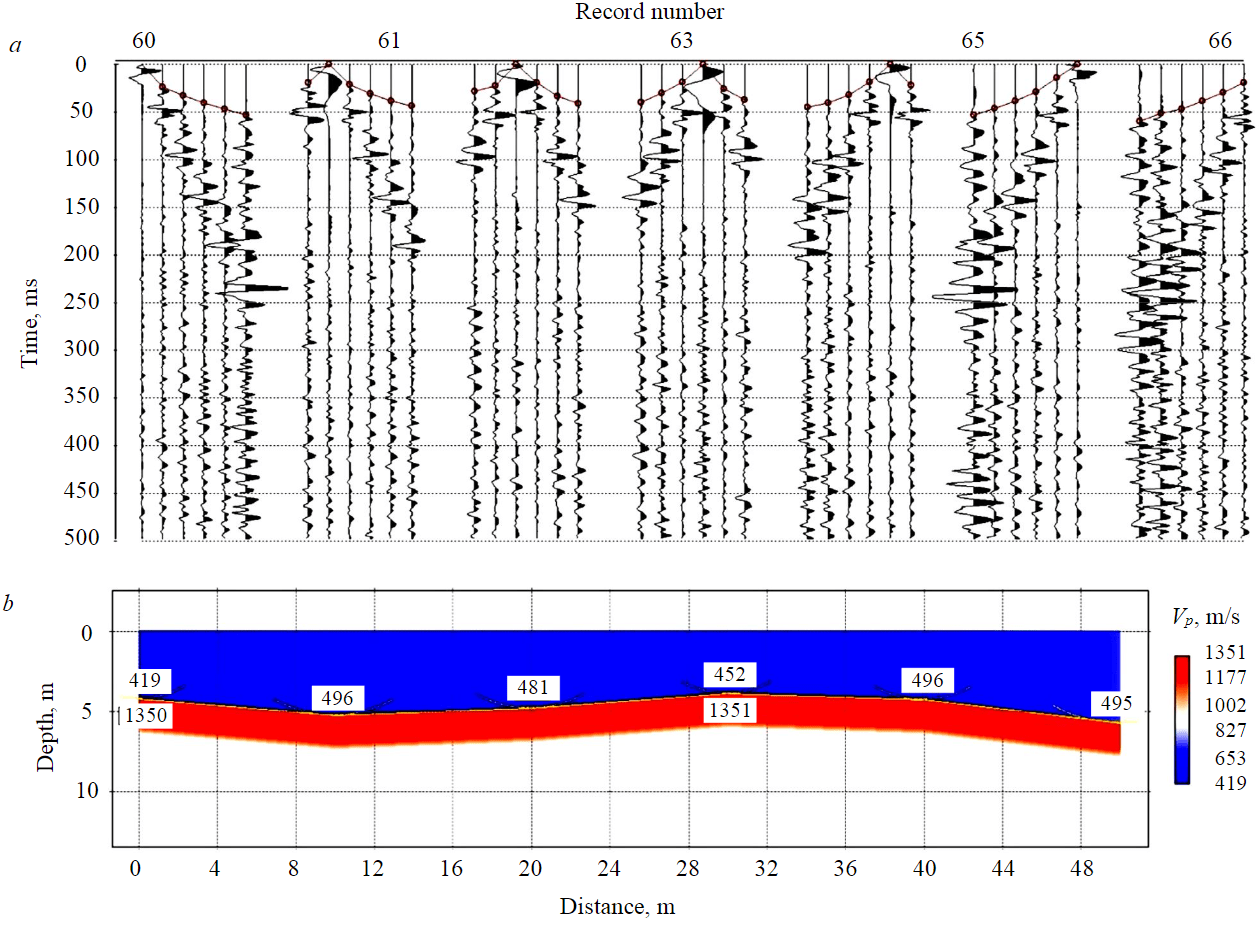
Fig.4. Seismograms (a) recorded on profile N 6 and depth-velocity section (red line – hodograph of the first arrivals) (b) obtained as a result of processing the RWM data; Vp – velocity of longitudinal waves
Snow-firn strata Core drilling
A complex study of the Antarctic ice sheet, as well as the subglacial continental platform, is impossible without drilling the snow-firn layer. Drilling of the Antarctic glacier upper layers is carried out for the purposes of geophysical, seismic, glaciological, microbiological and other types of research [12].
Currently, Mining University staff are substantiating and developing a technology for drilling snow-firn layers using air as a cleaning agent. When creating a new technology, the physical and mechanical properties of the snow-firn layer are taken into account, as well as previous experience in using air as a cleaning agent when drilling ice. The main properties of the snow-firn layer include low density, high porosity and permeability. The density of the snow-firn strata gradually increases with increasing depth, which was repeatedly confirmed when constructing density profiles based on the results of core drilling [13]. The porous, permeable structure of the snow-firn strata, which contributes to the loss of the cleaning agent in the massif and, as a consequence, the destruction of the well walls, has the greatest impact on the drilling process. The experience of using compressed air when drilling snow-firn formations has shown the low efficiency of drilling rigs with direct air supply to the bottom due to catastrophic losses of the cleaning agent and the formation of ice seals on drilling equipment [14-16]. The use of drilling rigs on a load-carrying cable with reverse bottom-hole air circulation can significantly increase productivity, energy efficiency and accident-free drilling compared to currently used auger and thermal core drilling methods.
In order to further clarify the data on the density characteristics of the snow-firn strata in the area of Vostok station, as well as to take samples of ice cuttings for further experimental studies, VK-23 well was drilled during the RAE 68th season.
Methods
The drilling site was chosen at a point with coordinates 78°28’18” S. 106°50’48” E, located 800 m from Vostok station. The distance from the drilling site to the station facilities, as well as to the construction site of the Vostok oil and gas complex, was chosen in such a way as to exclude the influence of artificial snow accumulation on the interpretation of the results of drilling operations. Drilling was carried out using a mobile drilling unit (MDU) LGGE (Laboratoire de Glaciologie Géophysique del’ Environnement) [17].
The drilling crew consisted of three people: a control panel operator, a driller servicing the tool, and a driller extracting ice cores and collecting ice cuttings samples. From each drilling run (the run was set to 0.5 m), cuttings and core material samples were collected, then they were packed into separate bags, which were marked in accordance with the run number and drilling interval. Cuttings and cores were delivered to the utility rooms of the 5G drilling complex, where they were stored at a temperature of –53 °C.
Studies of cuttings and core samples were carried out in the glaciological laboratory of the 5G complex (average temperature is –20 °C). Sieving of samples by sieve analysis was carried out dry using a set of manual laboratory sieves (GOST R 51568-99) with mesh sizes: 1.6; 1.25; 1; 0.8; 0.63; 0.4; 0.25 mm. The sieves were installed in a column from top to bottom from the largest cell to the smallest. Measurements of sample mass (as well as subsequent measurements of core mass) were carried out using electronic balances with an error of ±0.1 g. The core sample was prepared by quartering. The weight of one cuttings sample was 0.2 kg, sieving time was 1 min. Sieve analysis of cuttings was carried out at intervals of 2 m along the depth of the well. The yield of size classes in mass ratio was calculated using the formula
where mi – size class weight; ∑mi – size classes weight total sum.
The fractional composition was determined from three independent samples, which were alternately sieved on sieves; based on the sieving results, the average value for the interval was derived.
The density profile of the snow-firn strata was constructed by measuring the individual cores density. Measurements were carried out at intervals of 0.5 m in depth, starting from a depth of 5.5 m (the horizon from which 100 % core recovery began). Core density was determined by the formula
where mк – core weight, kg; Dк – average core diameter, m; lк – core length, m.
The average core diameter was calculated as the average of three measurements along the core length.
Results discussion
A total of 16 hours and 50 minutes were spent on drilling the well to a depth of 36 m (core depth 35.94 m), excluding the time for preparing the drilling site and conservation the well. 72 runs were carried out; when drilling the first 5.5 m from the surface, it was not possible to obtain an undisturbed core due to the low strength of the firn upper layers; from a depth of 5.5 m, the core yield was 100 %. Ice cuttings samples were taken from each run for further research; the cuttings yield was about 1 kg per 0.5 m of penetration.
Sieve analysis showed high convergence of sieving results for wells VK-23 and VK22 (drilled in the RAE 67th season) [12]), which is clearly visible in Fig. 5, a. As the depth of the well increases, the cuttings become finer-grained and is dominated by small size classes, which is explained by the structure of the snow-firn strata and the mechanism of its destruction.
Measurements of ice mass density from the extracted ice cores made it possible to construct a density profile for the VK-23 well (Fig.5, b). The process of changing the density of snow-firn strata with depth in the area of drilling well VK-23 corresponds to the general trend for wells drilled in the area of Vostok station [13], in particular for VK-22 well [12], located 600 m from the VK-23 drilling site. From the graph it is clear that at an interval of 5-10 m, which corresponds to fine-grained firn, a sharp increase in density from 325 to 425 kg/m3 occurs. Further, the density profile has a less differentiated character, while the density gradient decreases up to the top of the medium-grained firn (17 m) and averages 15 kg/m3 per 1 m. Around the 17 m mark, the firn density reaches 500 kg/m3, then at a depth 35 m density value exceeds 600 kg/m3, gradient values are 6.2 kg/m3.
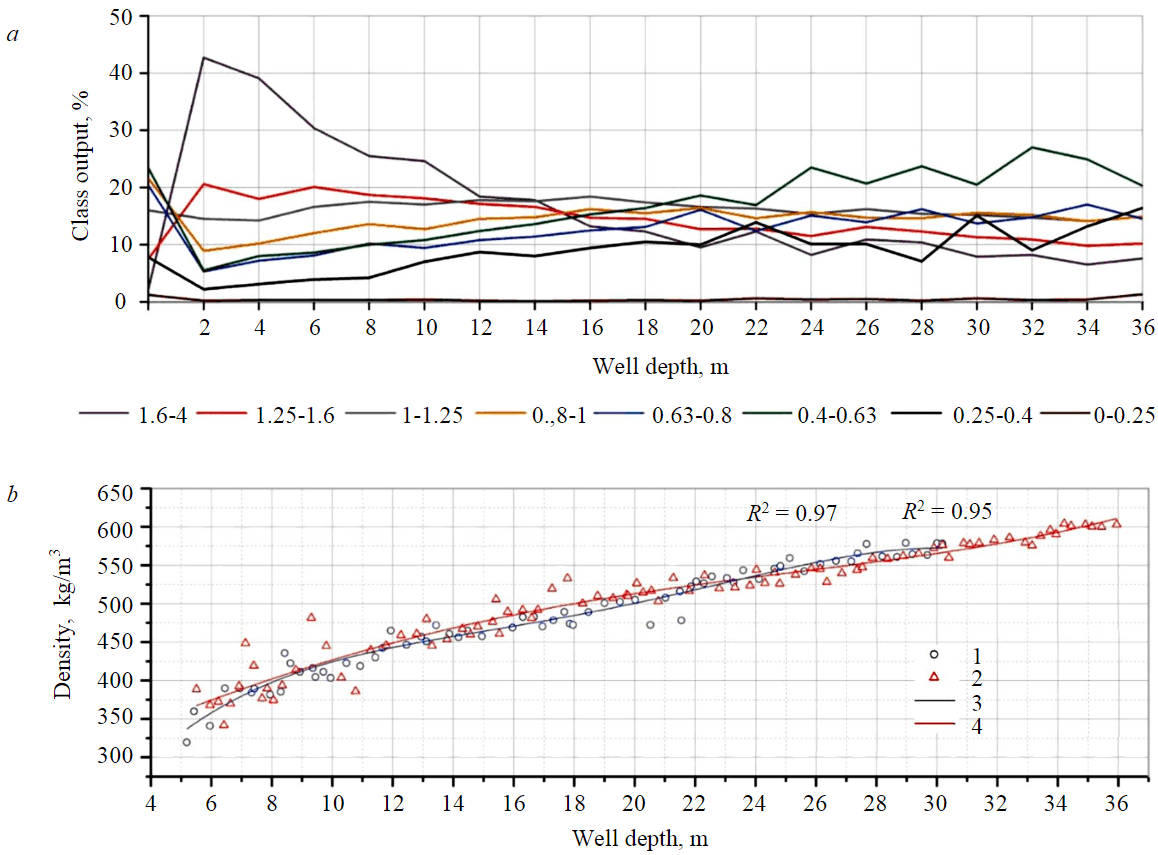
Fig.5. Dependence of changes in the granulometric composition of ice cuttings from VK-23 well with increasing depth (a) and the density profile of the snow-firn strata, constructed from cores VK-22 and VK-23 wells (b) 1 – VK-22; 2 – VK-23; 3 – polynomial trend line (VK-22); 4 – polynomial trend line (VK-23)
Summary
Data obtained during drilling of VK-23 well allow us to expand our understanding of the snow-firn strata properties near Vostok station. The resulting dependence of changes in the density of the snow-firn layer with depth complements the consolidated density profile compiled earlier in LCEA [17], thereby reducing the scatter of values and increasing its reliability. The selection of ice cuttings samples during drilling allowed us to continue the work on studying the process of drilling snow-firn strata with air blowing, which began in the 67th RAE [12]. Subsequent granulometric analysis made it possible to obtain unique data on the dependence of changes in cuttings size with increasing drilling depth, which will be used in the development of new drilling equipment by both domestic and foreign specialists.
Snow-firn strata petrostructural features study
In recent years, near-surface part of the Antarctic dome glaciological studies have focused on studying temperature, gas inclusions, chemical composition, and physical characteristics of snow and firn [18-22]. Research is underway to study the nature of the processes that occur during snow compaction and its transition to firn [23]. The analysis of the crystalline structure of firn sediments is carried out on the Spitsbergen islands and in Greenland [24], although they are mostly devoted to the study of subglacial rocks [25]. In the area of Vostok station, LCEA staff carried out similar work, but the object of study was glacier ice recovered from the 5G deep well [26].
To conduct detailed glaciological studies, it is necessary to study the territory geological structure features, dismember the complete stratigraphic section, and identify units and horizons with a similar structure. It is important to take this information into account in order to avoid obtaining an “apparent” age and geologically absurd results during geochronological studies of the Antarctic ice dome [27]. Petrostructural features, variations in structural and rheological characteristics provide information necessary for studying the stressed state of the glacier and constructing paleodynamic and paleoclimatic reconstructions. To study the physical and mechanical properties of the rock, the evolution of ice crystals size and shape data is needed, intercrystalline spatial relationships within the entire ice mass. It should be noted that insufficient knowledge of the snow-firn horizons stratigraphic section stratification in the Vostok station area, as well as the boundaries and factors of the snow-firn and firn-ice transition, does not allow us to reliably substantiate the parameters of the developed drilling technologies and equipment.
Methods
During the 68th RAE seasonal work, VK-23 well was drilled to a depth of 36 m. Using the core recovered from the well, in addition to joining, accurate measurement of length and density, a description of plates made from individual cores was carried out to study the petrostructural features of firn in the area Vostok station. Plates for further study were made in the glaciological laboratory. The minimum thickness of the polished plates, at which there was no destruction of firn and loss of individual grains from it, was selected experimentally and amounted to 5 mm.
After fabrication, the plates were placed on a light table for macroscopic characterization under polarizing glass. Then the most representative samples from the core were studied under a microscope. Typically, during microstructural analysis of ice and firn cores, samples for study are selected at intervals of tens of meters [24]. In this study, after it was established that the microstructure of adjacent intervals differed slightly, sampling was carried out in 5 m increments.
Results discussion
Petrostructural studies of snow-firn sediments selected from the Antarctic ice dome upper part section, detailed macro- and microscopic descriptions of more than seven polished firn plates produced in the glaciological laboratory were carried out. An example of a microscopic description is given below.
The run N 50 (core interval 25-25.5 m). Firn is medium-grained, composed of a heterogeneous mass, which can be conditionally divided into grains of two groups. More than 50 % of the rock is made of well-crystallized euhedral and hypohedral grains of tabular shape, the size of which reaches 2.5-3.0 mm; there are large isometric grains with six and four faces. The edges are clear and even. The transparency of the crystals increases, gas inclusions and single cracks parallel to the faces are noted in large grains. The second group is represented by grains up to 1 mm. They are much less crystallized; their edges are uneven and broken. There are elongated and elongated crystals, filling 10-20 % of the rock volume, up to 1 mm in size. The plate is cut along the entire core, which indicates an increase in the rock strength.
Petrostructural studies of the upper part of snow-firn sediments made it possible to perform stratigraphic stratification of the ice dome upper part section, based on the peculiarities of the granulometric composition and structure of the rocks. A schematic section is given in Table 2. Four horizons of firn sediments were identified, in the intervals 0-5; 5-10; 10-18; 18-35 m and more.
It has been established that with depth there is an increase in the grain size of firn sediments and an increase in the proportion of larger fraction crystals, which is due to the processes of recrystallization under the influence of directed stress and time. An increase in pressure with depth ensures an improvement in the strength properties of the studied rocks and an increase in their density. This correlates with a common feature of most of the ice physical and structural characteristics that makes up the Antarctic Ice Sheet, which is the evolutionary change of ice rock layers through the process of compaction and metamorphism [13]. In this case, at a depth of 10 m, a conditional boundary can be drawn, which corresponds to the end of lithogenesis and the beginning of metamorphism. This is due to the fact that the core, recovered from the underlying intervals, has a granoblastic structure, characteristic of metamorphic rocks. In the interval of 10-17 m, relict granular structures, typical of terrigenous sediments, are still observed, but with depth their contrast decreases significantly.
Таблица 2
Schematic stratigraphic section of the near-surface part of the Antarctic ice dome in the area of Vostok station
Summary
In the Antarctic ice dome section near-surface part, four horizons of snow-firn sediments are identified, characterized by different petrostructural features and density properties (Fig.5, b). The position of geological boundaries at depths of ≈ 5 m and 10-12 m is confirmed by the results of surface seismic work; at these intervals there is a significant increase in the elastic waves propagation speed (see Fig.4, b).
In the future, it is planned to conduct similar studies at a distance from the VK-23 well to correlate the obtained data, construct structural maps of the selected horizons, and validate the results obtained by area. An important role here can be played by the physical and mechanical properties of the selected horizons, which should also be taken into account in the stratigraphy of snow-firn sediments. It is necessary to reduce the thickness of the sections, which will increase their transparency, and study in more detail the intergranular space, grain orientation, gas inclusions, and voids.
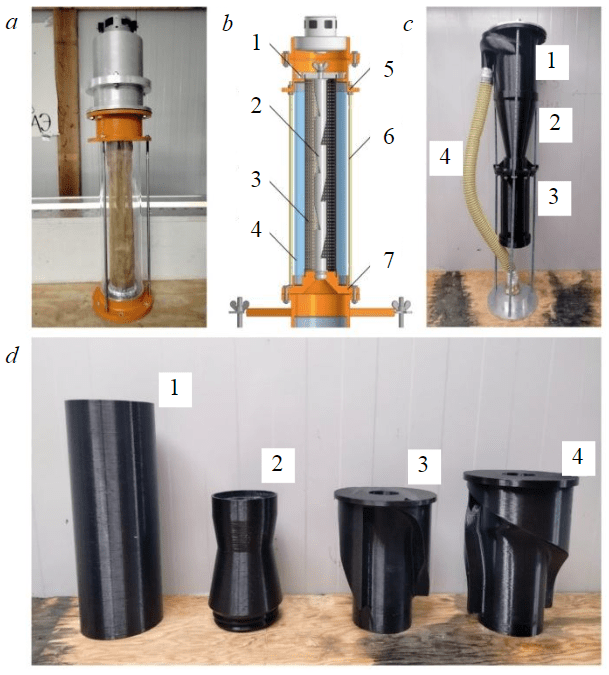
Fig.6. Designs of cuttings collection filters: a – general view of the experimental stand upper part before testing the mesh cuttings collection filter; b – mesh cuttings collection filter (1 – mesh filter flange; 2 – sludge lifting tube; 3 – mesh filter; 4 – transparent plexiglass pipe; 5 – top flange; 6 – studs; 7 – lower flange); c – cyclone filter with one inlet pipe (1 – cylindrical part of the cyclone with tangential supply; 2 – conical part of the cyclone; 3 – sludge collection tank; 4 – sludge lifting hose); d – cyclone filter with two and three inlets (1 – sludge collection tank; 2 – conical part of the cyclone; 3 – upper part of the cyclone with two inlets; 4 – top part of a cyclone with three inlets)
Cuttings collection filters when drilling snow-firn strata effectiveness study
The drilling mode parameters selection should be carried out in such a way that complete and timely cleaning of the bottom of the well from rock destruction products is achieved. Specifics of ice drilling with projectiles on a load-carrying cable [28], when the cleaning agent circulates in the borehole zone, additionally requires ensuring high efficiency of the cuttings collection compartment. Failure to comply with this requirement leads to the fact that the cuttings, without being caught from the cleaning agent (in this case, compressed air), will re-enter the annulus of the well and settle down to the bottom, which reduces the mechanical drilling speed and can lead to sticking of the projectile.
During the implementation of domestic and international deep ice drilling projects, many cuttings collection systems were tested, which were distinguished by unique design solutions that had not been used before. Thus, in the drilling unit of the CRREL laboratory (Cold Regions Research and Engineering Laboratory), the cleaning a well from cuttings principle was implemented by dissolving it in an aqueous solution of ethylene glycol, which was located in a special tank inside the body of the unit [29]. In the LGGE laboratory unit, the cuttimgs was separated from the filling liquid using a centrifuge, which was driven by the drilling unitelectric drive [30]. In JARE (Japanese Antarctic Research Expedition) [31] and HT (Hans Tausen) [32] drill units a similar design mechanism was used to compact ice cuttings using a booster JARE or a piston pump HT. The greatest efficiency in terms of the quality of cuttings collection and energy consumption was shown by systems operating on the principle of mechanical filtration, which involves filtering a mixture of filling fluid and cuttimgs through a filter element (mesh, grating, membrane, etc.). Cuttings collection compartments of KEMS (core electromechanical drill in Russian) and DISC (Deep Ice Sheet Coring Drill) projectiles [33] work on this principle. Despite the variety of designs of ice cuttings systems and the physical processes underlying their operation, there is no clear understanding of the effectiveness of these systems in air drilling.
In order to study the process of cuttings collection when drilling snow-firn strata with reverse bottom-hole air circulation, experimental work was carried out during the season of the 68th RAE, the planning of which was reflected in previously published works [12].
Methods
The idea of the experimental research was to find the optimal design of cuttings-collecting filters for a drilling unit with reverse bottom-hole circulation of compressed air. The studies were carried out on an experimental bench simulating the process of transporting ice cuttings by an ascending air flow inside a drill bit. To increase the power capacity of the stand, an additional turbine with a power of 2400 W was added to its design; to simplify the loading of ice cuttings samples, a loading tray was added to the stand design.
Four models of cuttings collection filters have been prepared (Fig.6). The first model was a cuttings collection mesh filter, the design of which is similar to the design of the KEMS-132 cuttings collection filter (Fig.6, a, b).
Ice cuttings, carried away by the air flow, passing through the lower flange, rises through the cuttings lifting tube, in the walls of which there are holes. Through these holes, the cuttings enters the cavity of the mesh filter – a brass mesh, soldered on two shells and representing a cylindrical surface. The mesh size is selected so that the mesh is impermeable to cuttings, but permeable to air. Ice cuttings, filtered by the mesh, remains in the filter, and the purified air passes through the holes in the mesh filter flange through the motor turbine and is released into the atmosphere.
The remaining filter models were variations of cyclone filters, the operation of which is based on the inertial separation principle (Fig.6, c, d). Air and particles suspended in it (cuttings) enter the working cavity of the cyclone through the inlet pipe located tangentially to the cylindrical surface of the cylinder body. The air flow swirls inside the cyclone, the centrifugal force acting on the particles throws them towards the inner walls of the cyclone. Colliding with each other and the walls of the cyclone, the particles settle in the lower part of the cyclone and accumulate in the bunker. Cyclone filters are made using 3D printing and differ from each other in the number of inlet pipes and the geometry of the working space.
Experimental work to determine the efficiency of cuttings collection was carried out in the following sequence. A model of a cuttings collection filter was installed on an experimental stand. Next, using a loading tray, a sample of cuttings (800 g) obtained during drilling operations was sequentially fed into the base of the stand. Using a laboratory autotransformer and adjusting the rotation speed of the motor turbine, the air flow speed was selected at which the sample was transferred into the filter without any residue. At the end of the experiment, the rotation of the turbine stopped, and the cuttings collection filter assembly was removed from the stand. The efficiency of the cuttings collection filter was assessed by the ratio of the mass of collected cuttings to the mass of the sample:
where mc – mass of captured cuttings, kg; ms – sample mass, kg.
Results discussion
Experiments with a mesh filter showed its low efficiency in trapping ice cuttimgs from an upward flow of compressed air. Particles of ice cuttings entering the filter continued to be suspended in turbulent air flows, which led to their re-crushing on the brass mesh of the filter. The crushed particles could freely pass through a previously impenetrable mesh, thus getting into the rotating parts of the turbine and settling on the walls of the experimental unit. As a result of a series of experiments, the average efficiency of strainers was 57.8 %, which, together with the large time costs for maintenance and repair, served as the basis for refusing their further use as cuttings collection filters when drilling with purging. Cyclone filters in all versions have shown high cuttings collection efficiency: cyclone filter with one inlet pipe – 96.3 %; with two inlets – 92 %; with three sub-waters – 96.8 %; mesh filter – 57.8 %. The high efficiency of cyclone filters is explained by the fact that the size of the cuttings belongs to class –I (very coarse dust), which corresponds to the area of rational use of cyclone-type dust collectors (Recommendations for the design and purification of air from dust in exhaust ventilation systems. Moscow: Stroyizdat, 1985).
Summary
The high efficiency of cyclone filters proves the prospects of their use when drilling snow-firn strata with reverse bottom-hole air circulation. A logical continuation of work in this direction is a more detailed study, including tests with a wider range of cyclone designs, speed ranges of air flow and ice cuttings samples from various drilling horizons.
Study of the destruction process using a reciprocating rotation method
The first work on the creation of a fundamentally new technical means for drilling subglacial rocks and taking samples of bottom sediments – a dynamically balanced drilling unit (DBDU) – was carried out at the Mining University more than 15 years ago [34]. A distinctive feature of the DBDU is the absence of a spacer system that perceives the reactive moment during the reciprocal rotational movement of the rock-cutting tool with a high frequency and amplitude of vibrations. Issues related to the study of dynamic processes in an electromechanical system were discussed in detail [34, 35], while the process of destruction of rocks by a reciprocating rotational method has not been studied.
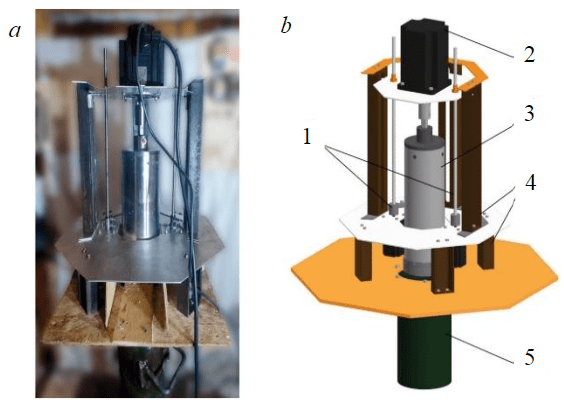
Fig.7. Physical (a) and digital (b) models experimental stand for studying the process of ice destruction by reciprocating rotation 1 – core pack feeding mechanism; 2 – electric drive for rotation of the core set; 3 – core set; 4 – supporting frame; 5 – artificial face
To study the process of destruction of ice, subglacial rocks and bottom sediments using a reciprocating-rotational method, an experimental stand was created, the first testing of which was carried out when drilling ice in the drilling complex of Professor Kudryashov at Vostok station during the season of the 68th RAE.
Methods
Testing of the experimental stand under the Vostok station conditions was carried out to assess the performance of its design and prepare technical specifications for the development of control and telemetry systems (Fig.7).
The testing of the experimental stand took place in the following sequence: installation of the experimental stand; carrying out commissioning work and assessing the performance of the structure and control system; elimination of detected deficiencies using the technical capabilities of Professor Kudryashov drilling complex; testing the performance of a rock-cutting tool with double-sided cutters when drilling holes in ice using a reciprocating rotary method; preparation of technical specifications for the modernization of the stand and the development of control and telemetry systems, taking into account the results obtained.
Results discussion
The experimental studies of the ice destruction process using the reciprocating rotation method main result was the establishment of the fact of its applicability when drilling glaciers. Three test experiments were carried out aimed at checking the performance of the experimental stand and identifying design flaws, as well as four experiments with a 100 % yield of an ice core sample.
Drilling using the reciprocating rotary method was carried out with the following established drilling parameters: drill bit rotation speed – 150 rpm; drill bit oscillation frequency – 1 Hz; vibration amplitude of the crown – 210 degrees; the feed speed of the core set to the bottom is 4.8 m/h. In 5 minutes of drilling using the reciprocating method, a core with a diameter of 110 mm and a length of 220 mm was obtained, which corresponds to a drilling speed of 2.64 m/h.
During the experimental stand performance testing, the following shortcomings were identified: high metal consumption; insufficient supporting frame rigidity; poor visualization and control of the ice destruction process; ineffective cuttings removal system; unprotected power and control circuits from cuttings; incorrect software operation in low temperature conditions. Despite the identified shortcomings, the stand showed its applicability in studying the process of ice destruction using the reciprocating rotation method, which is confirmed by the obtained core material.
Summary
The identified deficiencies are planned to be eliminated during the modernization of the experimental unit. Further work includes: upgrading the supporting frame to increase rigidity and reduce metal consumption; development of a system for telemetry and monitoring of drilling operating parameters (amplitude and frequency of oscillations of the rock-cutting tool, flow rate of the drilling fluid); improvement of the system for removing cuttings from the borehole; development of crowns with different geometries, taking into account the boundary conditions of the stress-strain state of the ice mass.
In the future, it is planned to conduct research on the process of subglacial rocks destruction using a reciprocating rotation method at a modernized experimental stand. The research results will be used in the development of drilling rigs and drilling tools for drilling subglacial rocks, taking into account their physical and mechanical properties.
Acoustic scanner bench testing
The essence of acoustic logging (AL) is reduced to the excitation of elastic waves in the ultrasonic and sound ranges in a well, which propagate through rocks and drilling fluid (filling fluid) and are received by one or more receivers located in the same well. A wave, reaching the interface between two media with different elastic properties, is divided into two components – reflected and transmitted waves. Structurally, the emitter and receivers are combined in one downhole module, which can be installed in the assembly of a drilling rig or geophysical complex or be a separate rig. Measurements are carried out both during the drilling process and after its completion [36].
Adaptation of acoustic research systems for Antarctic conditions is an urgent scientific and practical task, the solution of which, including at Vostok station, will make it possible to implement [37-39]: continuous profilometry and caliper survey along the entire wellbore; well construction geometric model; identification of fractured areas in the borehole space; search for rock inclusions in ice; drilling unit joints quality in the ice mass study; studying the features of the propagation of elastic wave fields in various types of ice in natural occurrence; study of the physical and rocks mechanical properties in natural occurrence and process fluids in a well [40-42].
The proposed technology for studying subglacial Lake Vostok involves the thermal formation of a local cavity (cavern) in the lower section of the well for the controlled rise of lake water into it when the lake is opened. At the same time, it is planned to use acoustic logging to obtain data necessary for operational forecasting and modeling of the processes of cooling and freezing of lake water in the extended section of the well.
The first stage of testing (implementation) of acoustic methods for studying wells at the Vostok station is ultrasonic scanner “Scanner-2000” bench testing produced by “NPO Poisk” AO, which operates on the principle of reflected waves. During the seasonal work of the 64th RAE, bench and downhole tests of an ultrasonic scanner were carried out at the drilling complex of Professor Kudryashov at Vostok station, which revealed a number of significant shortcomings, which the manufacturer tried to eliminate by upgrading the software.
Methods
Further bench tests of the ultrasonic scanner were carried out during the 68th RAE season on a specially assembled bench (Fig.8) and were carried out in two stages:
- Measurements of the calibration diameters of containers along the circumference were carried out in standard metal barrels and half-barrels with a diameter of 0.56 m and in a metal cup with a diameter of 0.30 m and were aimed at checking the performance of the equipment and determining the speed of sound at positive and negative temperatures of the filling liquid and the environment.
- Determination of the size and shape of a cavern in an ice block formed during experimental research during the season of the 64th RAE using a thermohydraulic drilling unit – reamer 90/180 (THDU).
The operating parameters of the acoustic scanner were selected manually sequentially in the “Radius” measurement mode. In each test measurement, for a given pulse (micro, 1, 5, 20), its power was changed by discretely moving the slider along the pulse power scale with a step of 2 in the range from 0 to 200 per units. Thus, for one rotation of the device around its axis by 360°, 100 measurements were carried out with different pulse powers.
The time for one measurement along a circle, taking into account the return of the module to its original position (reverse), was 130 s, after which the device by default switches to the “Bottom” measurement mode. The fill fluid temperature was measured using two independent methods: a low-temperature float-type mercury thermometer and a Fluke thermocouple meter.
Results discussion
The first stage of testing an ultrasonic scanner in calibration tanks gave positive results for metal barrels and half-barrels, but negative results for a metal cup, which is presumably due to its small geometric dimensions. Obtaining the calibration tanks known diameter value along the circumference under the given conditions for testing the device made it possible to determine the dependence of the sound speed on the temperature of the drilling fluid, which was JET-A-1 aviation kerosene, used when drilling the 5G well.
The second stage of testing the ultrasonic scanner was carried out in a cavern formed in an ice block by a thermohydraulic expansion drill during the season of the 64th RAE. The cavern profile had straight and slightly inclined (conical, with a slope of no more than 10 degrees) sections, as well as sections with a sharp change in diameter (twice), which corresponds to the proposed technology for opening the subglacial Lake Vostok using a thermal method. Measurements of the cavity diameter were carried out at various horizons with a discrete descent of the device.
The first positive results were obtained at horizon 9 at an ambient and liquid temperature of –24 °С, impulse – 1 and power – 123 (Fig.9, а), which was then increased to 133 (Fig.9, b) and to 142 (Fig.9, c). Three measurements differ from each other, and the second one is closest to the true diameter value determined by a mechanical hand-held caliper. At the same time, in none of the three measurements was a clear ice-kerosene interface observed in the cavernogram. Repeating the tests every other day at the same operating parameters, but with a decrease in the temperature of the environment and liquid to –30°С also did not give a positive result. Subsequently, positive results were obtained at horizons 7 and 8. The measurement results at other horizons were negative, which made it impossible to build a 3D model of the cavern.
In the “Bottom” measurement mode, a positive result was obtained only once and allowed us to confidently determine the distance from the bottom end of the scanner to the bottom of the well, equal to 0.41 m.
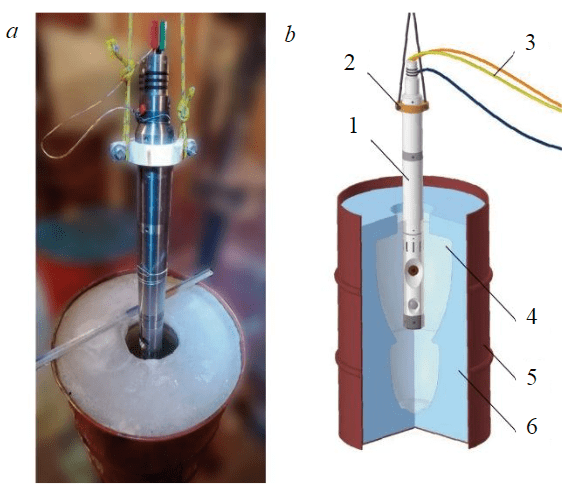
Fig.8. Test bench of the geophysical complex: a – general view; b – 3D model 1 – scanner; 2 – suspension; 3 – connecting wires; 4 – cavity with working fluid; 5 – barrel; 6 – ice
Conclusions
During testing ultrasonic scanner “Scanner-2000”, more than 5,000 measurements were made to check the performance and select the operating parameters of the ultrasonic scanner in an ice cavern, but only four measurements gave a confident positive result, which is an unsatisfactory result of testing the device, despite the manufacturer upgrade. However, despite the identified shortcomings in the operation of the device, the ultrasonic method of measuring cavities in the ice mass to construct their geometric model is fundamentally applicable.
It should be noted that for the effective use of the “Scanner-2000” ultrasonic scanner in the conditions of the Vostok station, its deep modification is required, which should include: installation of additional sources and receivers of acoustic waves into the device; making changes to the mathematical and statistical processing of data in the Scan Polar software; identifying the influence of the temperature factor on the correct operation of the device; providing the ability to measure complex geometric surfaces.
Conclusion
During the season of the 68th RAE, Mining University staff, together with colleagues from the AARI, completed a drilling program in well 5G-5, which resulted in an ice core parallel to the core previously extracted from well 5G-2 [43]. Studying new core material with a modern instrumentation system allows us to assess the reliability of previously obtained results and acquire new scientific knowledge.
In addition to the drilling work, shallow seismic surveys and core drilling of the snow-firn strata were carried out. The petrostructural features of the snow-firn strata were studied and the effectiveness of cuttings collection filters when drilling the snow-firn strata was determined. The process of ice destruction by a reciprocating rotation method was studied and bench tests of an acoustic scanner were carried out.
It is necessary to note the interdisciplinary connections establishment between drilling, geophysical and petrophysical studies of the glacier, as well as the petrostructural features of its constituent rocks. The results obtained during various studies correlate with each other. The results of microscopic analysis of the petrographic structure and texture of firn made it possible to identify four horizons that differ in strength and density properties. This trend can be seen in the firn density profile from the core extracted from the shallow well VK-23 at selected intervals 0-5; 5-10; 10-17; > 17 m. The position of the identified horizons is confirmed by the results of surface seismic work, which showed the presence of refractive boundaries at depths of ≈ 5 and 10-12 m.
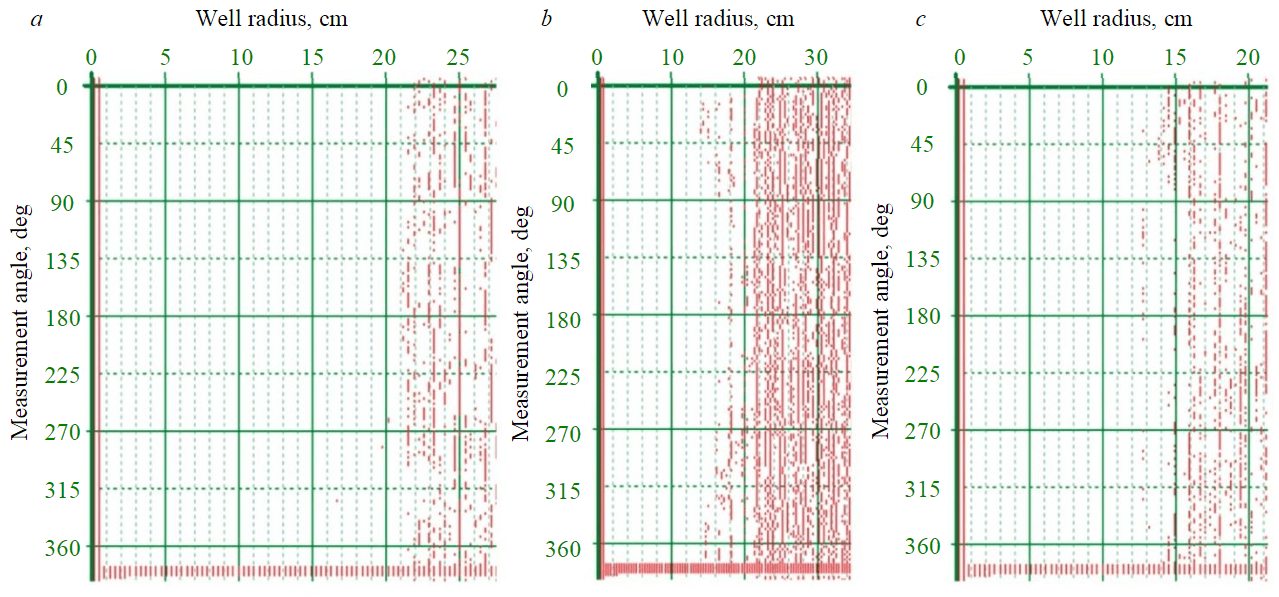
Fig.9. Measuring the diameter of an ice hole in the “Radius” mode with the engine turned on at an impulse power of 123 (a); 133(b); 142 (c)
The cuttings obtained during drilling of the snow-firn layer was used to conduct effectiveness of cuttings collection filters studies, which took into account its physical and mechanical properties and particle size distribution. The results showed the high efficiency of cyclone-type filters.
The study of the process of ice destruction using the reciprocating rotation method showed its effectiveness in drilling ice masses and made it possible to obtain data for the development of technical specifications for the design of a stand for a multifactorial experiment to study the process of destruction not only of ice, but also of subglacial rocks.
“Scanner-2000” acoustic scanner bench tests revealed shortcomings in the operation of the geophysical complex at low temperatures in an ice hole filled with kerosene. The test results showed that the echolocation geophysical method is applicable to the borehole conditions of Antarctica, but requires other technological and technical approaches to determining the geometric characteristics of the object under study.
An analysis of the research work carried out showed the effectiveness of an interdisciplinary approach to the interpretation of data obtained by different methods, and increasing the reliability of the results of experimental studies. An interdisciplinary approach is especially relevant when carrying out geophysical work, due to the ambiguity of solving inverse problems of geophysics and the variety of petrophysical parameters of geological structures [44-46].
References
- Litvinenko V.Foreword: Sixty-year Russian history of Antarctic sub-glacial lake exploration and Arctic natural resource development. Geochemistry. 2020. Vol. 80. Iss. 3. N 125652. DOI: 10.1016/j.chemer.2020.125652
- Lipenkov V.Ya., Ekaykin A.A. Hunting for Antarctica's oldest ice. Led i Sneg. 2018. Vol. 58. N 2, p. 255-260 (in Russian). DOI: 10.15356/2076-6734-2018-2-255-260
- 3. Leitchenkov G., Antonov A., Luneov P., Lipenkov V.Geology and environments of subglacial Lake Vostok. Philosophical Transactions of the Royal Society A. Vol. 374. Iss. 2059. N 20140302. DOI: 10.1098/rsta.2014.0302
- Popov S.V. Six decades of radar and seismic research in Antarctica. Led i Sneg. 2021. Vol. 61. N 4, p. 587-619 (in Russian). DOI: 10.31857/S2076673421040110
- Popov S.V., Chernoglazov Yu.B. Subglacial Lake Vostok, East Antarctica: coastline and surrounding bodies of water. Led i Sneg. 2011. Vol. 1 (113), p. 13-24 (in Russian).
- Yang Y., Song X. Multidecadal variation of the Earth’s inner-core rotation. Nature Geoscience. 2023. Vol. 16, p. 182-187. DOI: 10.1038/s41561-022-01112-z
- Mikhalsky E.V., Tkacheva D.A., Skublov S.G. et.al. Low-grade Sandow Group metasediments of the Denman Glacier area (East Antarctica): Chemical composition, age and provenance from U–Pb detrital zircon data, with some palaeotectonic implications. Polar Science. 2020. Vol. 26. N 100587, p. 1-18. DOI: 10.1016/j.polar.2020.100587
- Jacobs J., Mikhalsky E., Henjes-Kunst F. et.al. Neoproterozoic geodynamic evolution of easternmost Kalahari: Constraints from U-Pb-Hf-O zircon, Sm-Nd isotope and geochemical data from the Schirmacher Oasis, East Antarctica. Precambrian Research. 2020. Vol. 342. N 105553, p.1-20. DOI: 10.1016/j.precamres.2019.105553
- Popov S.V., Masolov V.N., Lukin V.V., Popkov A.M. Domestic seismic, radar and seismological studies of subglacial Lake Vostok. Led i Sneg. 2012. Vol. 52. N 4, p. 31-38 (in Russian). DOI: 10.15356/2076-6734-2012-4-31-38
- Sysoev A.P., Gorelik G.D. Parametric method of compensation for near-surface heterogeneity in processing CDP data. Russian Geology and Geophysics. 2017. Vol. 58. N 6, p. 948-954 (in Russian). DOI: 10.15372/GiG20170610
- Gospodarikov A.P., Revin I.E., Morozov K.V. Composite model of seismic monitoring data analysis during mining operations on the example of the Kukisvumchorrskoye deposit of AO Apatit. Journal of Mining Institute. 2023. Vol. 262, p. 571-580. DOI: 10.31897/PMI.2023.9
- Ignatiev S.A., Vasilev D.A., Bolshunov A.V. et al. Experimental research of ice cuttings transport by air while drilling of the snow-firn layer. Led i Sneg. 2023. Vol. 63. N 1, p. 141-152 (in Russian). DOI: 10.31857/S2076673423010076
- Ekaykin A.A., Tchikhatchev K.B., Veres A.N. et al. Vertical profile of snow-firn density in the vicinity of Vostok station, Central Antarctica. Led i Sneg. 2022. Vol. 62. N 4, p. 504-511 (in Russian). DOI: 10.31857/S2076673422040147
- 14. Lange G.R. Deep rotary core drilling in ice: Technical Report 94. Hanover, New Hampshire: USA CRREL, 1973, p. 47.
- Kapitsa A.P. Experience of drilling ice in Antarctica with cleaning the bottom with air. Drilling exploration wells using the core method with bottom hole cleaning with air. Moscow: Gosgeoltekhizdat, 1958, p. 78-81 (in Russian).
- Patenaude R.W., Marshall E.W., Gow A.J. Deep core drilling in ice, Byrd Station, Antarctica. Technical Report 60. Wilmette, Illinois: USA SIPRE, 1959, p. 12.
- Veres A.N., Ekaykin A.A., Lipenkov V.Ya. et al. First data on the climate variability in the vicinity of Vostok Station (central Аntarctica) over the past 2,000 years based on the study of a snow-firn core. Arctic and Antarctic Research. 2020. Vol. 66, N 4, p. 482-500 (in Russian). DOI: 10.30758/0555-2648-2020-66-4-482-500
- ShibayevYu.A., Tchikhatchev K.B., Lipenkov V.Ya et al.Seasonal variations of snowpack temperature and thermal conductivity of snow in the vicinity of Vostok station, Antarctica. Arctic and Antarctic Research. 2019. Vol. 65. N 2, p. 169-185 (in Russian). DOI: 10.30758/0555-2648-2019-65-2-169-185
- Ekaykin A.A., Teben’kova N.A., Lipenkov V.Ya. et al.Underestimation of Snow Accumulation Rate in Central Antarctica (Vostok Station) Derived from Stake Measurements. Meteorologiya i gidrologiya. N 2, p. 114-125 (in Russian).
- Lipenkov V.Ya. How air bubbles form in polar ice. Earth’s Cryosphere. 2018. Vol. 22. № 2, p. 16-28 (in Russian). DOI: 10.21782/KZ1560-7496-2018-2(16-28)
- Lipenkov V.Ya., Salamatin A.N. Steady-state size distribution of air bubbles in polar ice. Led i Sneg. 2014. Vol. 54. N 4, p. 20-31 (in Russian). DOI: 10.15356/2076-6734-2014-4-20-31
- Ekaikin A.A., Vladimirova D.O., Teben'kova N.A. et al. Spatial variability of the isotopic composition and rate of snow accumulation at the snow-measuring site of Vostok station (Central Antarctica). Problemy Arktiki i Antarktiki. 2019. Vol. 65. N 1, p. 46-62 (in Russian). DOI: 10.30758/0555-2648-2019-65-1-46-62
- Tchikhatchev K.B., Lipenkov V.Ya. On modeling the non-stationary process of snow-firndensification in the polar ice sheet. Arctic and Antarctic Research. N 4 (106), p. 76-87 (in Russian).
- Machguth H., MacFerrin M., van As D. et al. Greenland meltwater storage in firn limited by near surface ice formation. Nature Climate Change. 2016. Vol. 6. N 4, p. 390-395. DOI: 10.1038/nclimate2899
- Sirotkin A.N., Talovina I.V., Duriagina A.M.Mineralogy and geochemistry of alkaline lamprophyres of north-western Spitsbergen (Svalbard). Geochemistry. 2020. Vol. 80. Iss. 3. N 125508. DOI: 10.1016/j.chemer.2019.04.004
- Krikun N.S., Grokhotov E.I., Volkova V.I. Primary results of studies of petrostructural features of the near-surface part of the ice dome of Antarctica and plans for the future Breakthrough technologies in the exploration, development and production of hydrocarbon resources: Tezisy dokladov II Mezhdunarodnoi nauchno-prakticheskoi konfe-rentsii, 7-9 iyunya 2023, Sankt-Peterburg, Rossiya. St. Petersburg: Sankt-Peterburgskii gornyi universitet, 2023, p. 35 (in Russian).
- Lipenkov V.Ya., Polyakova E.V., Duval P., Preobrazhenskaya A.V. Internal structure of the Antarctic ice sheet in the vicinity of Vostok station from the deep-ice core thin-section studies. Arctic and Antarctic Research. 2007. N 76, p. 68-77 (in Russian).
- Kulchitskiy A.A., Mansurova O.K., Nikolaev M.Yu. Recognition of defects in hoisting ropes of metallurgical equipment by an optical method using neural networks. Ore and Metals. 2023. N 3, p. 81-88 (in Russian). DOI: 10.17580/chm.2023.03.13
- Ueda H.T., Garfield D.E. Drilling through the Greenland Ice Sheet. Special Report 126. Hanover, New Hampshire: USA CRREL, 1968, p. 7.
- Donnou D., Gillet F., Manouvrier A. et al. Deep Core Drilling: Electro-Mechanical or Thermal Drill? Proceedings of the Second International Workshop/Symposium on Ice Drilling Technology, 30-31 Aug 1982, Calgary, Alberta, Canada. USA CRREL, 1984. Spec. Rep. 84-34, p. 81-84.
- Tanaka Y., Takahashi A., Fujii Y. et al. Development of a JARE deep ice core drill system. Memoirs of National Institute of Polar Research. 1994. Spec. Iss. 49, p. 113-123.
- Johnsen S.J., Hansen S.B., Sheldon S.G. et al. The Hans Tausen drill: design, performance, further developments and some lessons learned. Annals of Glaciology. 2007. Vol. 47, p. 89-98. DOI: 10.3189/172756407786857686
- Mason W.P., Shturmakov A.J., Johnson J.A. et al. A new 122 mm electromechanical drill for deep ice-sheet coring (DISC): 2. Mechanical design. Annals of Glaciology. 2007. Vol. 47, p. 35-40. DOI: 10.3189/172756407786857640
- Zagrivny E.A., Poddubny D.A.Dynamically balanced drilling machine for a load-carrying cable for taking bottom sediments of subglacial lakes in Antarctica. Issues in Modern Machines Mechanics: Proceedings of the VII International scientific Conference, June 25-30 2018, Russia. Ulan-Ude: Publishing Department of East Siberia State University of Technology and Management, 2018. Vol. 1, p. 197 (in Russian).
- Zagrivniy E.A., Poddubniy D.A. Drill at a carrying cable with senserless control of electrc drive with autoresonant swinging movement. Izvestiya Tula State University. 2016. Iss. 3, p.178-187 (in Russian).
- Siegert M.J., Makinson K., Blake D. et al.An assessment of deep hot-water drilling as a means to undertake direct measurement and sampling of Antarctic subglacial lakes: experience and lessons learned from the Lake Ellsworth field season 2012/13. Annals of Glaciology. 2014. Vol. 55. Iss. 65, p. 59-73. DOI: 10.3189/2014AoG65A008
- Morin R.H., Williams T., Henrys S. et al. Downhole Measurements in the AND-1B Borehole, ANDRILL McMurdo Ice Shelf Project, Antarctica. Terra Antarctica. 2007. Vol. 14. Iss. 3, p. 167-174.
- Rudakov M.L., Duka N.E. Analysis of properties of deafeners to design personal ear protectors. Mining Informational and Analytical Bulletin. 2022. N 3, p. 165-180 (in Russian). DOI: 10.25018/0236_1493_2022_3_0_165
- McAfee C.W.I., Rix J., Quirk S.J. et al. Non-contact measurement system for hot water drilled ice boreholes. Annals of Glaciology. 2021. Vol. 62, p. 223-232. DOI: 10.1017/aog.2020.85
- Van T.N., Thang V.P. et al. A comprehensive method for determining the dewaxing interval period in gas lift wells. Journal of Petroleum Exploration and Production Technology. 2023. N 13, p. 1163-1179. DOI: 10.1007/s13202-022-01598-8
- Islamov S.R., Bondarenko A.V., Gabibov A.F., Mardashov D.V. Polymer compositions for well killing operation in fractured reservoirs. Advances in Raw Material Industries for Sustainable Development Goals. London, UK. Taylor&Francis Group, 2021. p. 343-351. DOI: 10.1201/9781003164395-43
- Islamov S.R., Bondarenko A.V., Mardashov D.V.A selection of emulsifiers for preparation of invert emulsion drilling fluids. Topical Issues of Rational Use of Natural Resources: Proceedings of the XV International Forum-Contest of Students and Young Researchers under the auspices of UNESCO, May 17-19, 2019, St. Petersburg. Vol. 2. London: Taylor & Francis Group, 2020, p. 487-494. DOI: 10.1201/9781003014638-2
- Ekaykin A.A., Lipenkov V.Ya., Veres A.N. et al. On the possibility to restore the climatic signal in the disturbed record of stable water isotope content in the old (0.4–1.2 Ma) Vostok ice (Central Antarctica). Led i Sneg. 2019. Vol. 59. N 4, p. 437-451 (in Russian). DOI: 10.15356/2076-6734-2019-4-463
- Egorov A.S., Vinokurov I.Yu., Telegin A.N. Scientific and Methodical Approaches to Increase Prospecting Efficiency of the Russian Arctic Shelf State Geological Mapping. Journal of Mining Institute. 2018. Vol. 233, p. 447-458. DOI: 10.31897/PMI.2018.5.447
- Litvinenko V.S., Kozlov A.V., Stepanov V.A. Hydrocarbon potential of the Ural–African transcontinental oil and gas belt. Journal of Petroleum Exploration and Production Technology. 2017. Vol. 7. N 1, p. 1-9. DOI: 10.1007/s13202-016-0248-4
- Litvinenko V.S., Leitchenkov G.L., Vasiliev N.I. Anticipated sub-bottom geology of Lake Vostok and technological approaches considered for sampling. Geochemistry. 2020. Vol. 80. Iss. 3. N 125556. DOI: 10.1016/j.chemer.2019.125556