Hydrogeoecological conditions of technogenic groundwater in waste disposal sites
- 1 — Ph.D., Dr.Sci. Head of Center Institute of Economics, Ural Branch of the RAS ▪ Orcid
- 2 — Ph.D., Dr.Sci. Head of Laboratory Institute of Economics, Ural Branch of the RAS ▪ Orcid
- 3 — Ph.D. Senior Researcher Institute of Economics, Ural Branch of the RAS ▪ Orcid
Abstract
The specific hydrogeoecological conditions of aquifers of some technogenic formations, mainly iron ore skarn-magnetite and titanium-magnetite formations, are considered. The resulting wastes, which are stored in waste disposal sites during development of deposits, due to the impact of a number of factors (natural and technogenic) form technogenic waters. Waste disposal facilities are complex engineering structures (dumps and sludge storages), which in turn create their own hydrogeoecological conditions, which must be investigated in order to prevent and minimize environmental and economic damage caused by these objects to the aquatic environment. The paper presents long-term field and laboratory studies of the aquatic environment under the influence of a waste disposal facility in the Middle Urals – one of the largest tailings, representing a potential environmental and man-made hazard. This tailing dump contains tens of tons of waste – enrichment tailings and creates specific hydrogeoecological conditions on the territory. Based on many years of monitoring studies, an analysis of these conditions was carried out – the quality of groundwater affected by the tailings was assessed. It is shown that groundwater is of technogenic nature, i.e. are man-made waters that have a significant impact on the surface and underground hydrospheres of the territory.
Introduction
During the development of deposits, waste, that must be stored in waste disposal sites (WDS) and which represent technically complex structures, is generated. They can be divided into “dry” storage facilities (various dumps) and “wet” storage facilities (tailing dumps). As a result of natural and technogenic processes impact (precipitation, condensation, discharge of technical water), groundwater is formed in WDS in the form of technogenic aquifers.
The purpose of the study is to research the conditions for the formation and discharge of groundwater in WDS. At the same time, it is necessary to assess the factors of groundwater volume and quality formation in WDS, as well as their impact on the surface and underground hydrosphere.
The studies were carried out in the Middle Urals, where more than 10 billion tons of waste are located. WDS are placed in specific hydrological and hydrogeological conditions in the open structure of the mountain-folded Urals and, in turn, create specific hydrogeoecological conditions. All this determines the need to study the hydrogeoecological conditions of waste disposal sites, the impact of which on the aquatic environment is often assessed as catastrophic [1-3].
Methods
The work used hydrogeoecological analysis, geochemical and retrospective methods. The work is based on a large number of field observations that the authors have been conducting for more than 20 years at waste disposal sites from the enterprises of mining and industrial complex of the Middle Urals. The paper analyzes the results of the own monitoring studies of environmental components – surface and ground waters, as well as geological materials.
As an example, the sludge storage of the Kachkanarsky Mining and Processing Plant (MPP) was studied, the chemical composition of its waste, mg/dm2: Sс – 0.013; ТiО2 – 0.7; Y – 0.01; СаО – 19.2; ZnО2 – 0.1; SiO2 – 47.5; Fe – 6.3; MgO – 13.4. To control the groundwater quality in the area of tailing dump impact, a regime network of observation wells was created, including 12 wells of the same type, which are located on the first aquifer of groundwater from the surface. The depth of the wells was 21-47 m, the diameter was 112-132 mm, with 127 mm casing of the upper zone of clay-grus formations and isolation of the annular space from the ingress of surface melt and rain water into the aquifer of fractured-pore groundwater to be opened for prevention of distortion of the results of hydrochemical testing of groundwater. The wells are located at the foot of the sludge storage dams outside the outlets of the sludge water filtrate.
The main principles of well placement relative to the tailings boundaries are accessibility of the approach to the places of laying, uniformity of distribution along the tailings perimeter and compliance with the direction of groundwater flows movement.
The impact of discharge from the tailing dump on the underground hydrosphere is reflected by three wells. Ground and surface water samples were taken quarterly according to established methods and analyzed in accredited chemical laboratories. In total, more than 200 samples were analyzed.
Discussion of the results
The mountainous and folded Urals (Sverdlovsk, Chelyabinsk, Orenburg regions and Bashkiria) are assigned to the groundwater basin of fracture zones in the rocks of the Middle and Lower Paleozoic [4-6]. The geological structure of the basin is extremely complex. Long-term continental development led to formation of a relatively low-mountain relief with a preserved weathering crust that plays a dual role. First, the residual crust slows down the infiltration of precipitation; secondly, it helps to equalize infiltration. In areas of clayey crusts development, colmatation of cracks in the weathering zone is noted. Therefore, great importance is attached to linear zones of increased fracturing, which formed in the post-Hercynian and especially in recent times.
With the end of the Hercynian era of folding, geological development of the region did not stop, it continued in the form of physical, chemical weathering and arch-block uplifts with erosion processes. As a result, a vertically regular system of cracks of varying intensity forming fracture zones was formed. The zones were formed at different times, but the basis for the emergence of each subsequent one, according to the principle of inherited development, was the previous one. Although the filtration and storage properties, chemical composition of waters, speed of movement and unloading of fractured zones differ, they form one aquifer.
Thus, two taxonomic units or two aquifer complexes can be distinguished. One of the complexes is composed of loose Mesozoic and Cenozoic deposits, and the second is a single aquifer complex of rock fracture zones from Cambrian to Devonian (Fig.1).
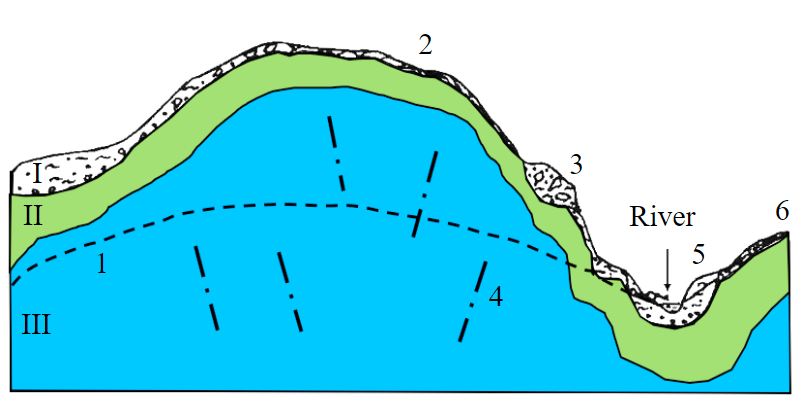
Fig.1. Groundwater layout in the hydrogeological massifs of the Urals Aquifer systems: I – loose Mesozoic and Cenozoic deposits; II – zones of regional fracturing; III – zones of tectonic faults 1 – groundwater level; 2 – zone of aeration and seasonal fluctuations
in the level of groundwater; 3 – groundwater of WDS; 4 – tectonic disturbances; 5 – groundwater of alluvial deposits
Mesozoic and Cenozoic formations of alluvial, eluvial-deluvial and technogenic genesis contain pore water. All of them form separate aquifers that differ in filtration and storage properties, thickness, sediment composition and occurrence conditions.
The WDS aquifer was formed in connection with the development of numerous deposits of solid minerals in the Urals, inevitably accompanied by excavation of ores and waste rocks from the bowels, which are stored in dumps. They are supplemented by sludge from processing plants and ash from thermal power plants, tailings from dredges in river valleys. Dumps and sludge storages have a local distribution, but play a significant role in changing the natural situation in the area of mines, forming a technogenic landscape. Large volumes and areas of dumps contribute to the accumulation of atmospheric precipitation, melted snow water, and condensation of vapors from the air [7-9].
Estimation of the factors of groundwater volume and quality formation in WDS
There are dry (for example, dumps of off-balance ores) and wet (tailing and sludge dumps) types of waste storage [10-12]. During dry storage, technogenic waters are formed mainly under the influence of atmospheric precipitation. In wet storage, the main role during the operation of the facility is played by production water, and after the termination of its operation, atmospheric precipitation [13-15]. There are three most characteristic options for the WDS location on the relief [16-18]:
- on the watershed – during dry storage, technogenic waters are formed only under the influence of atmospheric precipitation, and during wet storage – industrial waters (Saldinskoye sludge storage);
- on the slope – formation of the chemical composition of technogenic waters involves flat runoff flowing along the relief into the dump (Tonsky dump of the Vysokogorsky Mining and Processing Plant);
- in the channel part of temporary or permanent watercourses, surface waters are involved in the formation of technogenic waters (Cheremshanskoye sludge storage, open-hearth slag dump of OAO NTMK).
The most important hydrometeorological factors are the amount of precipitation, their average intensity, duration, chemical composition and pH. The morphometric parameters of the WDS can have a great influence on groundwater discharge. Therefore, in dry storage, the determining factor is the capacity of the WDS – the larger it is, the longer the contact of atmospheric water with the dump and the higher the volume of groundwater. In this case, the length and width of the WDS can be significant characteristics [19].
At the Goroblagodatsky deposit, groundwater of technogenic deposits has a wide area development around existing mines and the open pit mine. A certain role in changing the natural environment of the deposit area is played by waste rock dumps and sludge storages, which occupy huge areas (dump 6 – 1120000 m2, dumps 13, 14, 15 – 2250000 m2, Polovinkinsky sludge storage – 2.3 million m2). Large areas of dumps and their height contribute to the accumulation of liquid atmospheric precipitation, melted snow water, condensation of air moisture and their gradual consumption, which manifests itself in the form of hollows, permanent and temporary sources, in feeding small reservoirs.
Infiltration of atmospheric precipitation, snowmelt, replenishment due to surface runoff, and condensation of air moisture leads to accumulation of water under dump N 6 and their sudden breakthrough to the surface. Directly in the area of the breakthrough, the catchment area of dump N 6 is 524 thousand m2, the volume is 17.5 million m3. Depending on the amount of precipitation and condensation of air moisture, breakthroughs can occur both annually and with a break of up to two years. Breakthroughs in the summer (starting from the second half of summer) are characterized by suddenness, and in the case of their annual manifestations, the flow rate reaches 60-70 m3/h, the duration of the outflow 15-20 days. The total reserves of waters pouring out during this time are 21-25 thousand m3. In cases of water accumulation for two years, breakthroughs also occur suddenly, but the flow rate increases significantly, amounting to approximately 300 m3/h with a total outflow duration of up to 95 days.
In winter, the flow of water occurs at the initial moment slowly with the formation of ice, which, as it grows, moves towards the village of Blagodat. The gradual movement of water leads to flooding of the basements of houses, road to the crushing and processing plant N 3 is blocked by icing. The height of the icing reaches 0.5-3.0 m, the area is approximately 70 thousand m2. Only with an increase in the flow rate of the breakthrough, the water makes its way, and the comparatively fast unloading of the water accumulated under the dump takes place along the ditch. The total volume of water, subject to accumulation for several years, is significant – up to 500-600 thousand m3 (Fig.2).
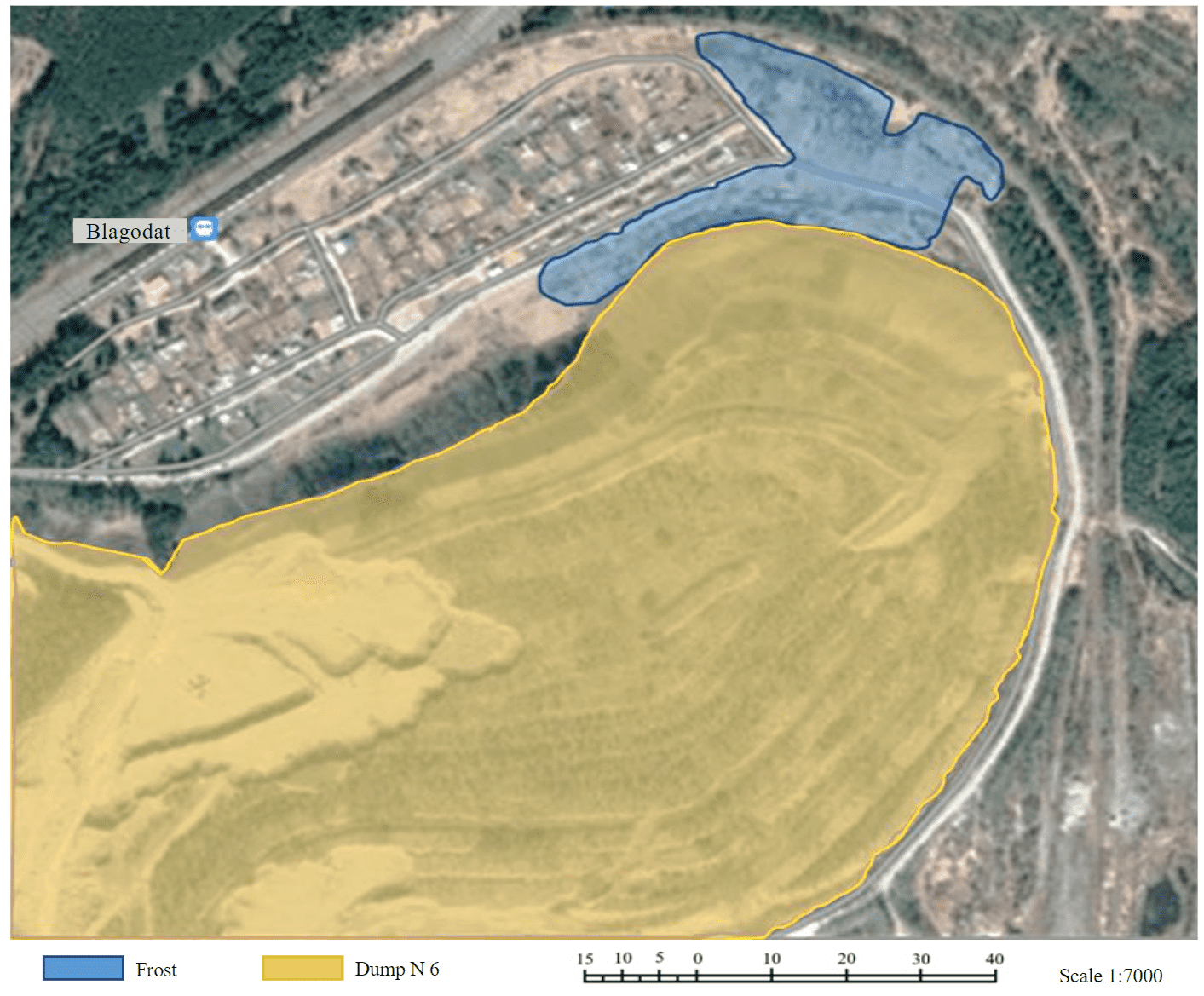
Fig.2. Scheme of the location of ice from groundwater from the WDS at the Goroblagodatsky field
The accumulation of a large mass of water and a sudden breakthrough are explained by the fact that the dump is composed of particles of inhomogeneous size - clay, wood, rubble and blocks. Cold air in winter, warm air in summer ventilates the dump, bringing heat or cold according to the season. In winter, the dump is frozen in the edge parts, the inner parts remain unfrozen due to the high power. Condensation of air moisture occurs both in warm and cold periods with the formation of a rock-ice lens at the border of the thawed and frozen parts of the dump. The accumulation of water occurs both in the form of ice and in liquid form. It is retained as a dam, by a rock-ice lens, and in its marginal parts by layers of clays that create conditions for accumulation. With the accumulation of water to a critical level, the lens thaws and a sudden breakthrough occurs, usually in November-December. Breakthroughs have been occurring continuously for 50 years.
At the Estyuninskoye deposit, a permanent spring was recorded at the foot of the northern dump. At the same time, there was a hidden discharge of water from technogenic deposits into underground workings, which became apparent after the occurrence of a landslide cirque in the area of rock fall. Dumps are located on the surface of relatively flat areas of the terrain, adjacent to the slopes of the mountains and are always above the groundwater level. The thickness and area of the underlying clay deposits are such that they allow water to be retained all year round, so such aquifers can be conside-red permanent.
Sludge storages occupy low relief areas, for example, in river valleys, in depleted quarries, where, in addition to water involved in the transport of sludge, groundwater is recharged [20-23]. Similar aquifers were formed at the Gusevogorskoye deposit of titanomagnetites (tailing dump), at the Cheremshanskoye sludge dump of the Vysokogorskoye deposit, at the Polovinkinsky and Saldinsky sludge storages of the Goroblagodatsky ore field. Under the sludge reservoirs, groundwater level rose by 15-20 m with formation of spreading domes, springs formed at the base of the dams.
Geological and technical section along the observation network well: technogenic deposits tQ (depth of occurrence 0-3 m, layer thickness 3 m); deluvial loam Q (depth of occurrence 3-3.9 m, layer thickness 0.9 m); eluvial light yellow dense loam Q (depth of occurrence 3.9-8 m, layer thickness 4.1 m); gabbro S1I1-2 (depth of occurrence 8-27 m, layer thickness 19 m); drilling diameter 127-132 mm, casing depth 110 mm; drilling depth 6.7 m; water level – appearance 12 m, steady 12 m.
Assessment of groundwater quality formation in WDS
Formation of groundwater composition during their interaction with the solid phases of WDS occurs as a result of leaching [24], i.e., selective dissolution of one or several components of solid phase by liquid. Dissolution occurs until the chemi-cal potentials of dissolving component in the liquid and solid phases are equal. The limiting concentration of the component Сn achieved in this case corresponds to the state of a saturated solution and is called solubility, the value of which depends on many factors. Therefore, when studying the patterns of interaction in the water-rock system, natural modeling methods are of great importance.
To predict the removal of components from the solid phase of WDS into the liquid one, it is necessary to determine the following parameters: leaching rate constant, day–1; initial specific volume of the substance, units; filtration or infiltration rate, dm/day; substance density, g/dm3; concentration of saturated solution, g/dm3.
To determine the leaching rate constant, a filtration experiment is carried out on a special stand. A 500 ml test sample is placed in a filtration column, and distilled water is filtered through it with a pH corresponding to the pH of the sediments or filtration waters of the area. Filtration rate should be close to the real situation. From the moment the filtrate arrives, the countdown begins and sampling is carried out.
The study of the removal of pollutants from slimes and slags showed that slags with a higher metal content, a more coarsely dispersed composition and, as a result, a smaller area of contact between the solid and liquid phases, have a lower leaching capacity [25-27]. The authors calculated the time of complete leaching of metals in the considered waste. This period takes into account the contact time of water and solid phases. For dry-storage waste with an average rainfall of about eight hours and an average rainless period of 40 days, the total duration of contact between sediments and solid phase will be about one day per year. With this in mind, the time for complete leaching of metals from sludge by atmospheric precipitation will be hundreds, and from slag – thousands of years [28-30].
When examining the territory of the tailing dump in Kachkanar, carried out in different seasons, seven sources of formation of groundwater discharge were identified (Table 1). Thus, the total permitted wastewater discharge as a result of groundwater discharge from the tailings is 30,796.0 thousand m3/year. With sewage, suspended solids, sulfates, nitrogen compounds, iron, vanadium, copper, and oil products enter water bodies. The main environmentally hazardous component in the sludge water cycle is the nitrate ion supplied by quarry waters [31].
Assessment of groundwater impact in WDS on the surface and underground hydrosphere
Let us analyze the impact on surface and ground waters of one of the largest tailings in the Middle Urals, which is the object of waste disposal – tailings for the enrichment of titanomagnetite ores of the Gusevogorskoye deposit. The area of the tailing dump is 19.75 km2. The structure of the tailing dump includes dams: N 1 (filled with a maximum height of 82.5 m), N 2 (filled with a maximum dam height of 61.5 m) and eastern (filled with a height of 79 m). The filtration waters of the dams form three wastewater outfalls that enter the river Vyya. Table 2 compares the volumes of actual wastewater discharge from tailing dump into the river with permitted values in the dynamics of recent years.
As can be seen from Table 2, for all issues over the considered period of time, there are sharp fluctuations in volumes with periodic excesses of the allowable discharge. The increase in the volume of wastewater is due to the growth of the dam body due to its alluvium, the decrease in volume is due to the increase in the dam body due to the loading of downstream slopes with rock.
Table 1
Groundwater discharge indicators of the tailings in Kachkanar
Source of emission of pollutants |
Pollutant |
Wastewater discharge volume |
|||
thousand m3/year |
mg/dm3 |
t/year |
|||
Rogalevsky compartment |
Filtration through dam N 3
|
Suspended solids |
1361.0 |
4.0 |
5.444 |
Dry residue |
387.0 |
526.707 |
|||
BOD20 |
2.5 |
3.403 |
|||
BOD5 |
1.9 |
2.586 |
|||
Sulfates |
54.0 |
73.494 |
|||
Nitrate ion |
45.0 |
61.245 |
|||
Nitrite ion |
0.18 |
0.245 |
|||
Ammonia nitrogen |
0.1 |
0.136 |
|||
Iron |
0.34 |
0.463 |
|||
Vanadium |
0.01 |
0.014 |
|||
Copper |
0.0126 |
0.017 |
|||
Oil products |
0.11 |
0.150 |
|||
Filtration through dam N 4 |
Suspended solids |
1499.0 |
3.75 |
5.621 |
|
Dry residue |
385.0 |
577.115 |
|||
BOD20 |
5.3 |
7.945 |
|||
BOD5 |
3.5 |
5.247 |
|||
Sulfates |
53.0 |
79.447 |
|||
Nitrate ion |
45.0 |
67.455 |
|||
Nitrate ion |
0.19 |
0.285 |
|||
Ammonia nitrogen |
0.1 |
0.150 |
|||
Iron |
0.3 |
0.450 |
|||
Vanadium |
0.003 |
0.004 |
|||
Copper |
0.0089 |
0.013 |
|||
Oil products |
0.11 |
0.165 |
|||
Total for the Rogalevsky compartment 2860.0 thousand m3/year |
|||||
Intermediate compartment |
Filtration through dam N 1 |
Suspended solids |
521.0 |
4.0 |
2.084 |
Dry residue |
393.0 |
204.753 |
|||
BOD20 |
3.0 |
1.563 |
|||
BOD5 |
2.0 |
1.042 |
|||
Sulfates |
56.0 |
29.176 |
|||
Nitrate ion |
40.0 |
20.840 |
|||
Nitrite ion |
0.08 |
0.042 |
|||
Ammonia nitrogen |
0.39 |
0.203 |
|||
Iron |
0.21 |
0.109 |
|||
Vanadium |
0.002 |
0.001 |
|||
Copper |
0.001 |
0.001 |
|||
Oil products |
0.05 |
0.026 |
|||
Filtration through dam N 2 |
Suspended solids |
3632.0 |
2.75 |
9.988 |
|
Dry residue |
384.0 |
1394.688 |
|||
BOD20 |
2.9 |
10.533 |
|||
BOD5 |
2.0 |
7.264 |
|||
Sulfates |
51.0 |
185.232 |
|||
Nitrate ion |
45.0 |
163.440 |
|||
Nitrite ion |
0.13 |
0.472 |
|||
Ammonia nitrogen |
0.1 |
0.363 |
|||
Iron |
0.34 |
1.235 |
|||
Vanadium |
0.01 |
0.036 |
|||
Copper |
0.0126 |
0.046 |
|||
Oil products |
0.19 |
0.690 |
|||
Filtration through the eastern dam |
Suspended solids |
3095.0 |
2.78 |
8.604 |
|
Dry residue |
388.0 |
1200.860 |
|||
BOD20 |
2.7 |
8.357 |
|||
BOD5 |
1.8 |
5.571 |
|||
Sulfates |
53.0 |
164.035 |
|||
Nitrate ion |
45.0 |
139.275 |
|||
Nitrite ion |
0.2 |
0.619 |
|||
Ammonia nitrogen |
0.1 |
0.310 |
|||
Iron |
0.34 |
1.052 |
|||
Vanadium |
0.003 |
0.009 |
|||
Copper |
0.0094 |
0.029 |
|||
Oil products |
0.11 |
0.340 |
|||
Total for the Intermediate compartment 7248.0 thousand m3/year |
|||||
Vyisky compartment |
Siphon outlet from the tailing dump in the river Vyya |
Suspended solids |
20688.0 |
9.0 |
186.192 |
Dry residue |
304.0 |
6289.152 |
|||
BOD20 |
3.0 |
62.064 |
|||
BOD5 |
2.0 |
41.376 |
|||
Sulfates |
56.0 |
1158.528 |
|||
Nitrate ion |
40.0 |
827.520 |
|||
Nitrite ion |
0.08 |
1.655 |
|||
Ammonia nitrogen |
0.39 |
8.068 |
|||
Iron |
0.21 |
4.344 |
|||
Vanadium |
0.002 |
0.041 |
|||
Copper |
0.001 |
0.021 |
|||
Oil products |
0.05 |
1.034 |
|||
Total for the Vyisky compartment 20,688.0 thousand m3/year |
Table 2
Dynamics of volumes of actual wastewater discharge from tailings into the river Vyya Findings and their comparison with allowed values
Name |
Wastewater discharge, thousand m3 |
Multiplicity of exceeding the actual discharge of normatively permissible discharge value |
|
Actual |
Permissible discharge standard |
||
2018 |
|||
Dam N 1 |
60 |
132 |
– |
Dam N 2 |
3940 |
3750 |
1.1 |
Eastern dam |
1130 |
1910 |
– |
2019 |
|||
Dam N 1 |
521.0 |
120.0 |
4.3 |
Dam N 2 |
3632.0 |
5100.0 |
– |
Eastern dam |
2090.0 |
2890.0 |
– |
2020 |
|||
Dam N 1 |
429.7 |
521.0 |
– |
Dam N 2 |
4049.6 |
3632.0 |
1.1 |
Eastern dam |
3201.5 |
2090.0 |
1.5 |
The concentrations of chemical elements and substances in the outlets show that there are excesses over the permitted discharge for a number of indicators:
- In dam release N 1: dry residue – up to 1.1 times (1 year out of 5); BOD20 – up to 1.2 times (1 out of 5); sulfates – up to 1.1 times (1 out of 5); nitrate ion – up to 2.1 times (5 out of 5); nitrite ion – up to 2.5 times (1 out of 5); iron – up to 2.1 times (2 out of 5); vanadium – up to 1.7 times (1 out of 5); copper – up to 3.0 times (2 out of 5).
- In dam release N 2: dry residue – up to 1.1 times (1 year out of 5); sulfates – up to 1.3 times (1 out of 5); nitrate ion – up to 1.8 times (4 out of 5); nitrite ion – up to 2.6 times (2 out of 5); iron – up to 1.3 times (1 out of 5); vanadium – up to 1.4 times (1 out of 5); copper – up to 2.0 times (1 out of 5); oil products – up to 2.2 times (3 out of 5).
- Filtration through the eastern dam: dry residue – up to 1.1 times (1 year out of 5); sulfates – up to 1.4 times (1 out of 5); nitrate ion – up to 1.8 times (5 out of 5); nitrite ion – up to 4.0 times (3 out of 5); iron – up to 1.4 times (1 out of 5); copper – up to 3.0 times (1 out of 5); oil products – up to 2.2 times (2 out of 5).
When conducting mining operations, explosives, containing high concentrations of nitrates, are used, which are also a characteristic pollutant and have significant excesses. For explosions, ammonium nitrate with a nitrogen content of up to 30 % is used. Every year, the enterprise uses thousands of tons of explosives, and, accordingly, thousands of tons of nitrogen enter the environment.
Table 3
The results of natural waters control at the river Vyya
Composition indicators |
MPCf* for water bodies, |
Background concentrations, |
The content of pollutants in the control section |
Suspended solids |
+0.75 to the background |
3.0 |
3.0 |
Dry residue |
– |
67.7 |
315.0 |
BOD20 |
3.0 |
5.0 |
4.98 |
Sulfates |
100 |
4.90 |
45.0 |
Nitrate nitrogen (nitrate ion) |
9.1 |
0.99 |
94.0 |
Nitrite ion |
0.02 |
0.03 |
0.055 |
Vanadium |
0.001 |
0.0015 |
0.0037 |
Copper |
0.001 |
0.002 |
0.0029 |
* As a criterion for the groundwater pollution degree, the value of the maximum permissible concentration for fishery water bodies (MPCf) is taken, due to the fact that groundwater in the tailings area is involved in feeding surface water bodies and watercourses of fishery value.
Table 4
Results of groundwater sampling in the area of the tailings
Name of indicator |
MPCf, mg/dm3 |
2018 |
2019 |
2020 |
|||
Content |
MPC ratio |
Content |
MPC ratio |
Content |
MPC ratio |
||
Well 9 |
|||||||
Sulfates |
100 |
49.3 |
– |
33.4 |
– |
37.9 |
– |
Chlorides |
300 |
26.0 |
– |
4.86 |
– |
10.2 |
– |
Nitrates |
40 |
3.03 |
– |
2.77 |
– |
0.43 |
– |
Manganese |
0.01 |
1.27 |
127 |
0.096 |
9.6 |
0.154 |
15.4 |
Copper |
0.01 |
0.009 |
– |
0.014 |
1.4 |
0.003 |
– |
Iron |
0.1 |
0.466 |
4.66 |
0.3 |
3 |
3.18 |
31.8 |
Zinc |
0.01 |
0.012 |
1.2 |
0.014 |
1.4 |
0.031 |
3.1 |
Vanadium |
0.001 |
0.0664 |
66.4 |
0.027 |
27 |
0.009 |
9 |
Dry residue |
1000 |
232.0 |
– |
53.0 |
– |
172.3 |
– |
Suspended solids |
3.25 |
0.3 |
– |
581.6 |
179 |
812.3 |
250 |
Well 10 |
|||||||
Sulfates |
100 |
98.1 |
– |
– |
– |
– |
– |
Chlorides |
300 |
5.2 |
– |
– |
– |
– |
– |
Nitrates |
40 |
3.4 |
– |
– |
– |
– |
– |
Manganese |
0.01 |
4.0 |
400 |
– |
– |
– |
– |
Copper |
0.01 |
0.031 |
3.1 |
– |
– |
– |
– |
Iron |
0.1 |
0.326 |
3.26 |
– |
– |
– |
– |
Zinc |
0.01 |
0.034 |
3.4 |
– |
– |
– |
– |
Vanadium |
0.001 |
0.0328 |
32.8 |
– |
– |
– |
– |
Dry residue |
1000 |
348.0 |
– |
– |
– |
– |
– |
Suspended solids |
3.25 |
2.58 |
– |
– |
– |
– |
– |
Well 11 |
|||||||
Sulfates |
100 |
71.3 |
– |
79.3 |
– |
96.6 |
– |
Chlorides |
300 |
3.47 |
– |
8.1 |
– |
12.2 |
– |
Nitrates |
40 |
1.6 |
– |
2.34 |
– |
1.17 |
– |
Manganese |
0.01 |
0.126 |
12.6 |
0.058 |
5.8 |
0.116 |
11.6 |
Copper |
0.01 |
0.022 |
2.2 |
0.007 |
– |
0.003 |
– |
Iron |
0.1 |
0.217 |
2.17 |
2.83 |
28.3 |
1.13 |
11.3 |
Zinc |
0.01 |
0.006 |
– |
0.034 |
3.4 |
0.009 |
– |
Vanadium |
0.001 |
0.0177 |
17.7 |
0.015 |
15 |
0.036 |
36 |
Dry residue |
1000 |
176.0 |
– |
101.0 |
– |
302.0 |
– |
Suspended solids |
3.25 |
18.98 |
5.8 |
545.6 |
168 |
812.3 |
250 |
Note. As a criterion for the degree of groundwater pollution, it is proposed to take the background well 4-n, due to the fact that some pollutants (for example, copper, manganese, vanadium, iron) are typical for the area, which is associated with numerous ore deposits in the area under consideration and a large number of operating and decommissioned mine workings of various depths and areas, as well as waste storage facilities, wastewater outlets of industrial enterprises.
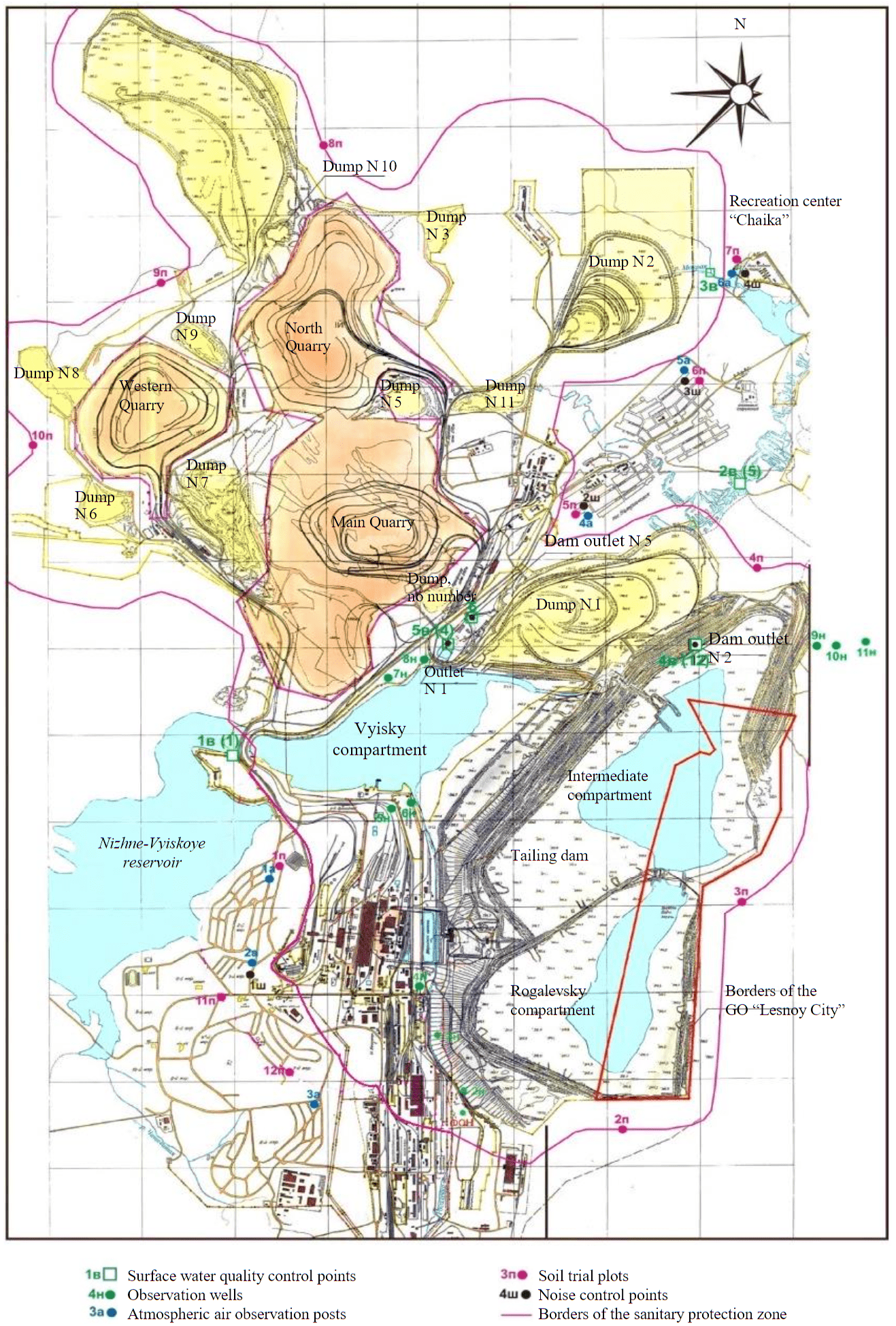
Fig.3. Location of observation points in the monitoring network
Objective indicators of the level of negative impact of a technogenic object on a watercourse are the background values of pollutants controlled in effluents and the results of analyzes in the control section after all outlets [32-34]. To determine the background concentrations of substances in the rivers, a target located above the outlets of wastewater was taken. Control over the state of the river Vyya after discharges of wastewater from all outlets is made by the enterprise that carries out a survey at a control site located below the outlets. The average annual results of monitoring the natural waters of the river are given in Table 3.
Analysis of Table 3 shows that, compared with the background values of chemicals concentrations in the river Vyya, concentration of the following pollutants in the control section increases: dry residue – by 246.4 mg/l (4.7 times, without exceeding the MPCf value); sulfates – by 40.13 mg/l (by 9.2 times, without exceeding MPCf); nitrate nitrogen – by 92.5 mg/l (by 93.5 times); nitrite ion – by 0.05 mg/l (2.5 times); vanadium – by 0.0022 mg/l (2.57 times); copper – by 0.0008 mg/l (1.4 times).
The mechanism of tailings impact on the state of groundwater is the leakage of sludge water through the dam N 1 (outlet of sewage N 2), dam N 2 and the eastern dam (outlets N 3 and 9) in the valley of the river Vyya, where zone of technogenic impact on the state of underground hydrosphere is planned.
The study of groundwater in the area of the tailings is carried out on a network of observation wells specially created for this purpose (Fig.3) [35]. The impact of the tailing dump on groundwater is characterized by the content of pollutants in wells 9, 10 and 11 of the observation network [35]. Testing of these wells was carried out quarterly. The results of testing are presented in Table 4.
The results of studies of groundwater of the regional fracture aquifer, taken in the area of the tailing dump, indicate significant groundwater pollution in terms of the following indicators: manganese (up to 400 MPC), iron (up to 67 MPC), zinc (up to 4 MPC), vanadium (up to 67 MPC), suspended solids (up to 250 MPC).
Conclusion
In hydrogeology, as one of the branches of the Earth sciences, a lot of material has been accumulated for the formation of a new scientific direction on groundwater in waste disposal sites. It has been established that the hydrogeological conditions of groundwater in waste disposal sites are often difficult in terms of the conditions for the costs formation and groundwater quality. The factors of groundwater formation in the WDS, which are associated with the conditions of placement and morphometric parameters, granulometric and chemical composition of the waste, are determined.
Using the example of one of the WDS in the Middle Urals, it is shown that groundwater from waste disposal facilities has a significant negative impact on the surface and underground hydrosphere. Therefore, it is necessary to systematically study hydrogeological conditions of the underground waters of the WDS both at the stage of operation and during the period of waste conservation.
References
- Konovalov V.E. Rehabilitation of mining landscapes. Ekologicheskaya i tekhnosfernaya bezopasnost gornopromyshlennykh regionov: Trudy VI Mezhdunarodnoi nauchno-prakticheskoi konferentsii 10 aprelya 2018 / Ed by A.I.Semyachkov. Ekaterinburg: Institut ekonomiki UrO RAN, Uralskii gosudarstvennyi gornyi universitet, 2018, p. 89-95 (in Russian).
- Semyachkov A.I., Pochechun V.A., Konovalov V.E., Ganin E.V. Ecological concept of mining landscapes rehabilitation.Ekaterinburg: Institut ekonomiki UrO RAN, 2020, p. 190 (in Russian).
- Maksimovich N.G., Pyankov S.V. Kizelovsky coal basin: environmental problems and solutions.Perm: Permskii gosudarstvennyi natsionalnyi issledovatelskii universitet, 2018, p. 288 (in Russian).
- Danilov A., Smirnov Y., Korelskiy D. Effective methods for reclamation of area sources of dust emission. Journal of Ecolo-gical Engineering. 2017. Vol. 18. Iss. 5, p. 1-7. DOI: 10.12911/22998993/74947
- Strizhenok A.V., Korelskiy D.S. Improvement of the system of industrial environmental monitoring of atmospheric air in the area of anthropogenic arrays impact. Journal of Physics: Conference Series. 2019. Vol. 1384 (1). N 012052.
DOI: 10.1088/1742-6596/1384/1/012052 - Semyachkov A., Slawikowskaja Yu., Pochechun V. Methodological Features of the Assessment of Economic Damage from Adverse Environmental Consequences in Conditions of Territories with a Developed Mining Complex. Ecology and Industry of Russia. 2018. Vol. 22. N 4, p. 46-51 (in Russian). DOI: 18412/1816-0395-2018-4-46-51
- Strategic directions and priorities of regional development in the face of global challenges. Under total ed. of Doctor of Economics Yu.G.Lavrikova, Doctor of Economics E.L. Andreeva. Ekaterinburg: UrO RAN, 2019, p. 504 (in Russian).
DOI: 10.17059/1_2019 - Antoninova N.Yu., Rybnikova L.S., Slavikovskaya Yu.O., Shubina L.A. Environmental and economic aspects of selecting reclamation directions for industrial mining and metallurgical waste disposal sites. Russian Mining Industry. 2022. N S1, p. 71-77 (in Russian). DOI: 30686/1609-9192-2022-1S-71-77
- Lyashenko V.I., Vorobiev A.E., Khomenko O.E., Dudar T.V. Development of Technologies and Technical Facilities to Reduce the Environmental Impact in the Area of Influence of the Tailings Dam. Vestnik Magnitogorskogo gosudarstvennogo tekhni-cheskogo universiteta im. G.I.Nosova. 2022. Vol. 20. N 1, p. 25-41 (in Russian). DOI: 10.18503/1995-2732-2022-20-1-25-41
- Plokhov A.S., Kharko P.A., Pashkevich M.A. Effect of tailings storage facility on surface water at copper-pyrite deposit. Mining Informational and Analytical Bulletin. 2021. N 4, p. 57-68 (in Russian). DOI: 10.25018/0236_1493_2021_4_0_57
- Khansivarova N.M. Assessment of the mechanical stability and protection of groundwater in the territories of the distribution of dispersed subsidence soils. Natural and Technical Sciences. 2021. N 10 (161), p. 120-125. DOI: 10.25633/ETN.2021.10.06
- Kovrigin A.A., Slesarev M.Yu. A change model of the hydraulic characteristics of the territory occupied by the landfill of solid industrial and domestic waste. Vestnik MGSU. 2021. Vol. 16. N 6, p. 770-780 (in Russian). DOI: 10.22227/1997-0935.2021.6.770-780
- Lyamin I.A., Ruzmanov R.Yu. Estimation of ground water protection on the waste disposal site of a mining enterprise. Bulletin of Perm University. Geology. 2020. Vol. 19. N 3, p. 241-246 (in Russian). DOI: 10.17072/psu.geol.19.3.241
- Baev O.A., Silchenko V.F. Impact analysis of waste ponds on surface and underground water (Review). Ecology and water management. 2020. N 4 (7), p. 100-113 (in Russian). DOI: 10.31774/2658-7890-2020-4-100-113
- Rybnikova L.S., Rybnikov P.A. Physico-chemical model of groundwater formation in the areas of flooded copper-pyrite mines of the Urals. Mining Informational and Analytical Bulletin. 2019. N S37, p. 584-595 (in Russian). DOI: 10.25018/0236-1493-2019-11- 37-584-595
- Saburov H.M., Muradov B.Z., Mukhamedgaliev B.A. Environmental pollution industrial waste. Bulatovskiye chteniya. 2019. Vol. 4, p. 110-111 (in Russian).
- Konovalov V.E., Germanovich Iu.G. Substance migration at minerals production and primary processing. Izvestiya vysshikh uchebnykh zavedeniy. Gornyy zhurnal. 2018. N 2, p. 30-39 (in Russian). DOI: 10.21440/0536-1028-2018-2-30-39
- Khayrulina E.А. Influence of slurry storage facility with salt-bearing wastes onthesurface and groundwaters. Geographical bulletin. 2018. N 2 (45), p. 145-155 (in Russian). DOI: 10.17072/2079-7877-2018-2-145-155
- Gyulmammadov Ch.D. Impact of human technogenic activity on hydrogeological conditions. Science and world. 2020. N 2-1 (78), p. 15-19 (in Russian).
- Kuznetsov Yu.S., Chaplia B.Yu., Khafizov A.R., Shulgina A.P. Environmental aspects of large-tonnage production liquid waste storage into the underground storage. Petroleum Engineering. 2019. Vol. 17. N 3, p. 56-61 (in Russian). DOI: 10.17122/ngdelo-2019-3-56-61
- Kletskina O.V., Oschepkova A.Z. About methodical approach to substantiation of criteria of admissible impact of the subject of landfill on underground waters with use of hydrogeological modeling. Geologiya i poleznyye iskopayemyye Zapadnogo Urala. 2019. N 2 (39), p. 337-342 (in Russian).
- Pichura V.I., Skok S.V. Influence of urban systems on hydrogeological and hydrochemical conditions of aquifers. Visnik Natsіonalnogo unіversitetu bіoresursіv і prirodokoristuvannya Ukraini. 2019. N 6 (82), p. 1-16. DOI: 10.31548/dopovidi2019.06.001
- Simion A.F., Drebenstedt C., Lazar M. The Influence of Tailings Storage Facilities in the Eastern Part of Jiu Valley on the Water Quality. Gornaya nauka i tekhnika. 2019. Vol. 4. N 4, p. 292-301. DOI: 10.17073/2500-0632-2019-4-292-301
- Kharlyamov D.A., Smirnova N.N., Sharafutdinov R.N., Mavrin G.V. An assessment of groundwater quality in territories with va-rious anthropogenic influence. International Research Journal. N 2-2 (116), p. 28-33 (in Russian). DOI: 10.23670/IRJ.2022.116.2.036
- Trofimova Yu.V., Rastorguev A.V. Assessment of the solid industrial and municipal waste landfill as a source of groundwater pollution. Inzhenernyye izyskaniya. 2019. Vol. 13. N 3, p. 28-39 (in Russian). DOI: 10.25296/1997-8650-2019-13-3-28-38
- Degtyar M., Galkina O. Ecological monitoring of the environmental state of waste concrete objects. Naukovi notatky. 2019. N 65, p. 55-60 (in Russian).
- Fisun N.V. Ecologic potential of the environmental and hydrogeological systems in the area of kuchinsky municipal solid waste landfill (Moscow region). Proceedings of higher educational establishments. Geology and Exploration. 2018. N 3, p. 58-64.
- Ponamarev Yu., Khazіpova V. Monitoring underground and surface waters in the area of polygon effect of industrial waste. Pozharnaya i tekhnosfernaya bezopasnost: problemy i puti sovershenstvovaniya. 2018. N 1 (1), p. 143-146. (in Russian)
- Kolesnikova L.A., Kovalchuk T.V. Problems and prospects of environmental safety of mining regions. Mining Informational and Analytical Bulletin. 2021. N 2-1, p. 275-286 (in Russian). DOI: 10.25018/0236-1493-2021-21-0-275-286
- Shepelev I.I., Eskova E.N., Pilyaeva O.V. Maintaining ecological stability of the operated natural – technical system under the impact of anthropogenic factors. Problemy regionalnoy ekologii. 2021. N 1, p. 76-82. DOI: 10.24412/1728-323X-2021-1-76-82
- Musikhina T.A., Iurlov A.A., Zemtsova E.A., Filatov V.Yu. Comprehensive assessment of the toxicity of river and waste waters, formed on the territory contaminated by industrial waste. Theoretical and Applied Ecology. 2021. N 4, p. 133-139. DOI: 10.25750/1995-4301-2021-4-133-139
- Lyashenko V.I., Golik V.I., Dyatchin V.Z. Increasing environmental safety by reducing technogenic loadin mining regions. Izvestiya vysshikh uchebnykh zavedeniy. Chernaya metallurgiya. 2020. Vol. 63. N 7, p. 529-538. DOI: 10.17073/0368-0797-2020-7-529-538
- Akhmadiyev A. K., Ekzaryan V. N. Rehabilitation of the natural environment as the structural element of ecological Mining Informational and Analytical Bulletin. 2020. N 2, p. 112-120 (in Russian). DOI: 10.25018/0236-1493-2020-2-0-112-120
- Glukhov V.V., Movchan K.S. Economic assessment of technology for waste processing taking into account impact on environment. St. Petersburg State Polytechnical University Journal. Economics. 2019. Vol. 12. N 1, p. 159-167. DOI: 10.18721/JE.12113
- Tagilcev S.N., Cherednichenko A.V., Melnik V.V. Aggregation methods hydrogeomechanical, electrical and dowsing to select areas of hydrogeological wells. Mining Informational and Analytical Bulletin. 2020. N 3-1, p. 236-246 (in Russian). DOI: 10.25018/0236-1493-2020-31-0-236-246