Comprehensive assessment of hydraulic fracturing technology efficiency for well construction during hydrocarbon production
Abstract
The oil and gas industry has been an integral and fundamental sector of the Russian economy for the past few years. The main problems of this industry have traditionally been the deteriorating structure of oil reserves; depreciation of main assets; slowdown and decline in oil production. Recently these have been complicated by a number of new negative trends related to underinvestment, limited financial resources, deteriorating access to new equipment and technologies. The task of the research is to make a comprehensive assessment of hydraulic fracturing technology during well construction and to increase the recovery and intensification of hydrocarbons production. In this research, modeling techniques were used to assess the productivity of each fracture. Geophysical methods (seismic survey) were used to determine the geomechanical properties of the formation. Comprehensive assessment of hydraulic fracturing technology during well construction was carried out, which allowed to increase vertical permeability and unite disparate parts of the reservoir in practice, and to determine the development efficiency of the hydrocarbon field.
Introduction
Modern Russian and international experience in improving hydrocarbon recovery and intensification of production is based on high technological and economic efficiency of a sufficiently large number of technologies developed and commercially exploited [1]. The primary ones for offshore fields development are drilling and exploitation of horizontal wells and their various modifications, as well as hydraulic fracturing. For the development of low-producing, low-yield and offshore oil and gas fields at the present stage, it is necessary to develop new technologies and machinery for drilling horizontal wells [2, 3].
Statement of the problem
At the field named after V.Filanovskiy, the Western Neocomian deposit has been in production since 2016, and pilot production at the Aptian deposit started in 2021 and is still in progress. At the beginning of 2022, the Western Neocomian deposit was being exploited by 16 horizontal production wells with an average oil flow rate of 1282 tons/per day, which enables production of 7.3 % of the current recoverable reserves per year.
The experience gained from the use of horizontal wells (HW), as well as the geological and geophysical features of the fields have led to the application of hydraulic fracturing (HF) technology at all the investigated sites. An effective technique for additional stimulation of horizontal wells is the application of HF technology, which can significantly increase the productivity of the well, increase the area of drainage in formations with low permeability [4, 5].
Over the last decade there have been major developments in HF technology, both worldwide and in Russia. The use of modern equipment, new technologies and HF design modeling makes it possible to conduct effective operations in vertical, deviated and horizontal wells [6, 7].
There is a contradiction between the needs of practice and the possibilities of theory, which currently does not consider the application of hydraulic fracturing technology for the efficient operation of oil and gas wells in hydrocarbon production [8, 9].
A comprehensive evaluation and selection of technology for efficient hydrocarbon (HC) production is a highly relevant scientific problem. Its solution will increase the efficiency of horizontal wells construction at hydrocarbon production. It is necessary to conduct a comprehensive assessment of hydraulic fracturing technology for the efficient construction of oil and gas wells in hydrocarbon production [10, 11].
Methodology
Methods were used to assess the productivity of each fracture without separate testing. Geophysical methods (seismic surveys), such as the direction of minimum and maximum horizontal stresses, which provided data on natural fracturing of the formation, tectonic disturbances, and rock sample investigations and minifrac tests on wells located in the study area were used to determine the geomechanical properties of the formation [10, 11].
The most reliable results on the optimum number of fractures and their effect on well operation parameters during exploitation are obtained by modeling [12, 13].
Discussion
Most of the hydraulic fracturing processing in vertical wells has been done similarly to the hydraulic fracturing already conducted [14, 15]. In order to successfully perform multiple hydraulic fracturing in a horizontal well before drilling, the authors investigated the following problems: determining the direction of minimum and maximum horizontal stress, spatial orientation of the horizontal wellbore in the planned well; selection of wellbore design; necessity to cement the horizontal section; determination of stimulation intervals; choice between creation of transverse or longitudinal hydraulic fractures relative to the horizontal wellbore; horizontal wellbore perforation and related issues – orientation, phasing, type and kind of perforators used; choice of HF type with fixation of the hydraulic fracture created, acid fracturing or a combination of these types; method of isolating each fracture; optimum distance between fractures, their height and width and characteristics of the technology used (such as TSO end screening); optimal use of calibration tests to optimize fracturing fluid volumes and sand-carrying fluid; methods of controlling the removal of the fixing material (proppant) [16, 17].
According to HF theory, the fracture propagates along the maximum stress or perpendicular to the minimum stress. As shown in [18, 19], it is possible to control the direction of fracture pro-pagation along or across the axis of the horizontal wellbore. To orient the fractures, it is necessary to align the borehole along the direction of the maximum or minimum stress, depending on the problems to be solved (Fig.1) [20, 21].
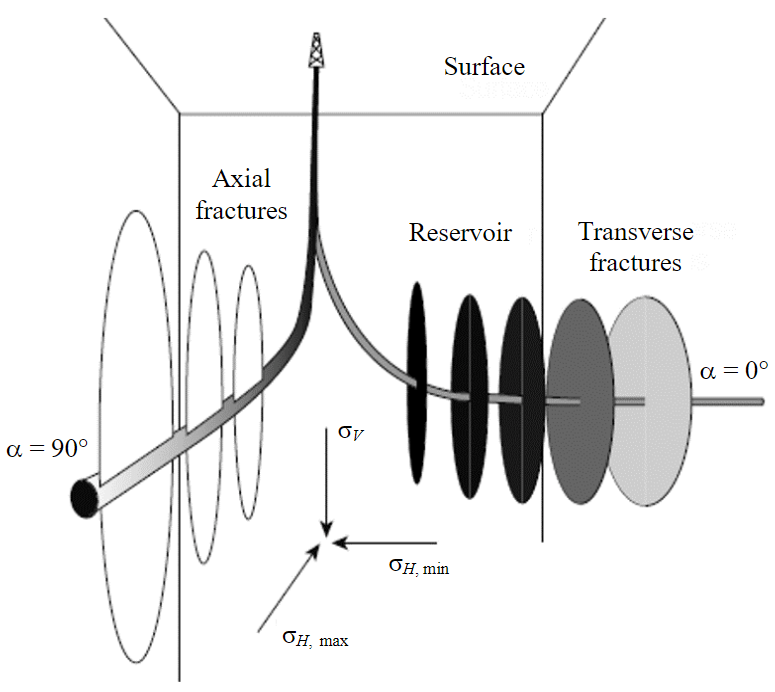
Fig.1. Schematic representation of fracture orientation
The authors found that one of the main reasons for stimulation of horizontal wells is to increase vertical permeability and connectivity of reservoirs, and to create fractures that are perpendicular to the horizontal wellbore in the vertical plane. If the horizontal well is drilled in the direction that coincides with the direction of the minimum main stress, the fractures created will be perpendicular to the horizontal well. However, if the horizontal well is drilled in the direction of the maximum main stress, the fractures created will be parallel to the horizontal well [22, 23].
The results of the authors' research have shown that an important aspect is the selection of the optimum number of fractures to be placed in the well. On the one hand, they should provide the expected productivity of the well, on the other hand, their number should be minimized, based on material and technical support. This problem is solved with the help of multifunctional software “Monitoring GDIS”, which allows to calculate the flow rate of the well and its productivity in dynamics, to determine the necessary stimulation intervals along the wellbore [24, 25].
It has been established that planned fractures in a horizontal wellbore must be mechanically isolated with packers and sliding sleeve ports. Efficient isolation technologies are based on multi-packer assemblies using catalyst-swellable (so-called “rubber”) or pressure-blown hydraulic packer systems. The main difference between these systems lies in their functionality. The former are used in wellbores with non-cased endings, the latter in cased and cemented endings [26, 27].
Creating multiple hydraulic fractures in a horizontal well is a technologically complex and costly operation. HF operations in horizontal wells are often unsuccessful due to low working fluid efficiency and the use of equipment that is not capable of performing the required technology. Therefore, an important factor is the choice and application of HF technology, which must be controlled at each stage of the operation [28, 29].
At the field named after V.Filanovskiy, HF was used for the first time on exploration well 15a drilled in the western section of the field.
HF has been carried out offshore in Russia for the first time. The equipment was specially designed for this purpose and the transport vessel, on which the equipment was placed, was upgraded [30, 31].
The HF technology activities were carried out according to the following plan: conducting a full mini-test, including injection test (substitution stage), reopening test, friction calibration test, proppant pack calibration test, flow rate increase and decrease test; conducting main HF.
During operations, the following was injected: total mass of proppant – 50000 kg; mass of proppant in formation – 50000 kg, of which 500 kg were left in the tubing according to calculations and injection technology; volume of cross-linked gel for main HF and mini-test – 210.2 m3; volume of linear gel – 18.4 m3.
The resulting fracture geometry was: total fracture half-length about 90 m; effective fracture half-length 60 m; fracture height in the near-bottomhole zone 69 m; average fracture height 45 m; fracture width in the near-bottomhole zone in oil-saturated sandstones 4 mm; average fracture width 2.5 mm; dimensionless fracture conductivity 2.7 [32].
Analysis of multi-zone HF in wells G-3a, H-9а, 15а, 16а
With assistance from Baker Hughes, 32 multistage HF operations were carried out on four wells in the field named after V.Filanovskiy. The project was designed to stimulate injectivity. The project was designed to perform injectivity stimulation on injection wells G-3a, N-9a and production stimulation on production wells 15a and 16a.
Planned depths of HF closure ports along the length of the lower completion string:
- well G-3а – gas injection, top of HF ports – 3040.63; 3240.11; 3448.41; 3740.28; 3989.88; 4199.80 m;
- well N-9a – water injection, top of HF ports – 2567.0; 2880.2; 3050.6; 3235.0; 3418.9; 3660.2 m;
- well 15а – production, top of HF ports – 3249.9; 3403.8; 3558.2; 3749.2; 3974.8; 4118.9; 4268.2; 4399.7; 4509.1; 4605.5 m.
- well 16a – production, top of HF ports – 2195.6; 2347.2; 2441.5; 2549.7; 2655.6; 2750.1; 2850.2; 2950.6; 3049.9; 3149.1 m.
Injection wells G-3a, H-9a were fractured six times at six HF ports in each well, production wells 15a and 16a were fractured 10 times at 10 HF ports in each well.
Coiled tubing of 66.7 mm diameter and 5000 m length on the barrel was used to open (close) HF ports and bottomhole filters using hydraulically activated NV-2 bottomhole tool. The use of a coiled tubing with this diameter was determined by the wellbore trajectories and the depths of HF ports and bottomhole sand filters.
For each well, an assessment and selection of materials used in the MHF works at wells G-3a, N-9a, 15a, 16a was carried out. The following types of works were carried out: installation of wellhead equipment and fitting the wellhead with high and low pressure lines; calibration running of coiled tubing to the bottomhole with well flushing; running of coiled tubing with bottomhole tool to close the sand filters and open the lower HF port; conducting HF operation; running of coiled tubing with bottomhole tool to close the previous HF port and open the next HF port (working operations are repeated at each HF port); after closing the upper HF port, running of coiled tubing to the bottomhole with flushing; running of coiled tubing with bottomhole tool to open sand filters; gaslift development with nitrogen injection until the HSP flow is stopped (or until one tanker vo-lume of formation fluids is extracted); dismantling of wellhead equipment and moving to the next well [33].
The authors have established: MHF of production wells contributed to 2.6-fold increase of oil flow rate at well 15a and 4-fold increase of oil flow rate at well 16a. The oil flow rates before and after MHF were compared: well 15a – 70.1 tons/day before HF and 184.2 tons/day after HF; well 16a – 49.1 and 198.7 tons/day, respectively.
Indicators for the HF design model for well 15a were taken from the geomechanical properties data on Aptian deposit, such as the direction of minimum and maximum horizontal stresses (Fig.2). The HF fluid used was similar to well 16a: guar-based fluid (carrier fluid) YF130STLUKOIL; displacement fluid WF115.
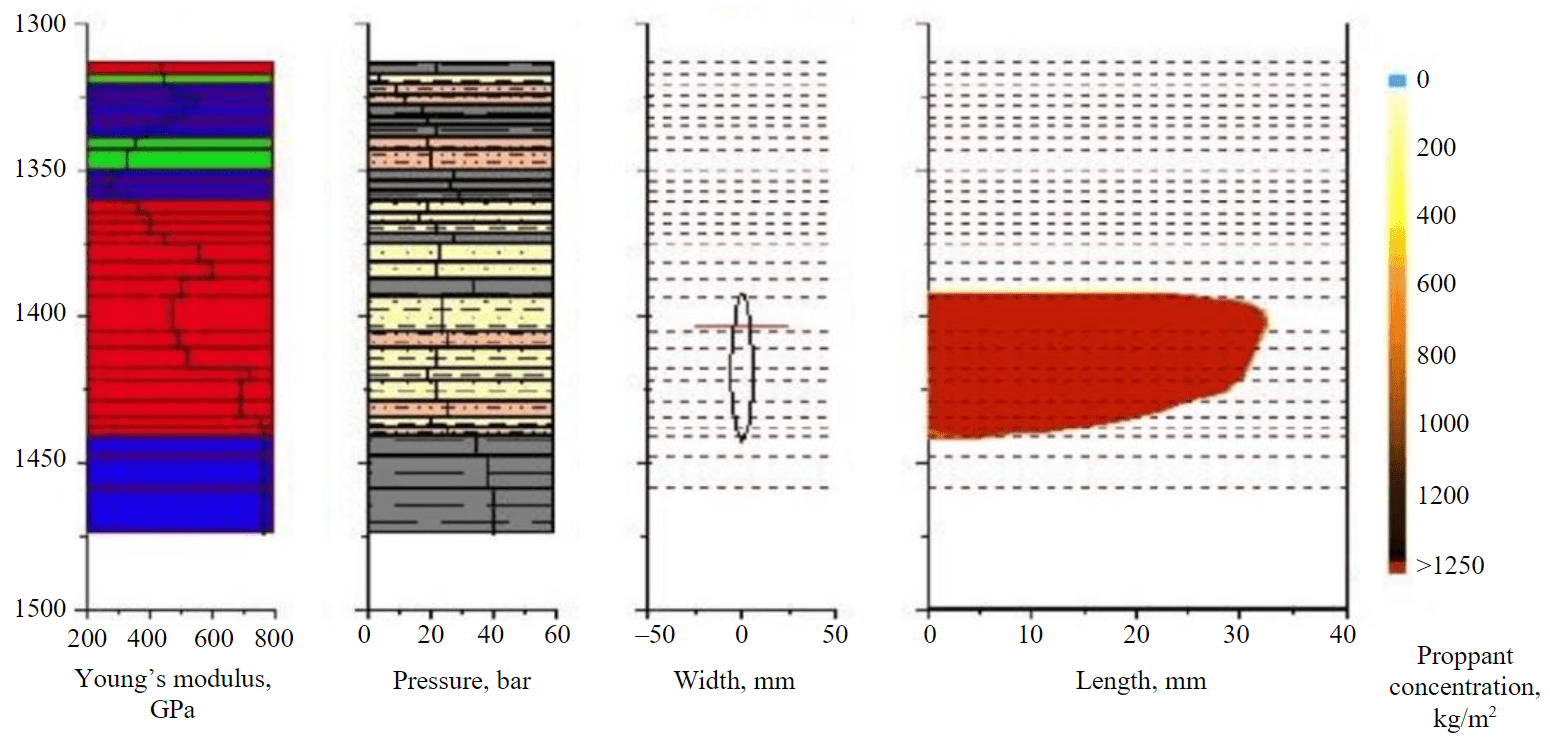
Fig.2. Model of fracture
The proppant permeability was calculated based on the following parameters: static temperature 64 °C; pressure on proppant 115 bar; proppant concentration is 5.08 kg/m2; average Young’s modulus value is 3.258 GPa.
Based on the results of HF design modeling by the authors (Fig.2), the well productivity coefficient was calculated and a comparison was made for longitudinal and transverse fractures. It was found that longitudinal fractures have a big advantage (Fig.3).
The graph (Fig.4) shows flow rate dynamics and cumulative production for a 1218×5000×37 m cube with an average permeability of 608 mD of one or two fractures, pressure drop is 27 atm.
According to the authors' investigations on cumulative production for longitudinal fractures, HF technology will have an advantage in a horizontal well over a highly permeable reservoir and will increase vertical permeability (Fig.5) and unite disparate parts of the reservoir.
During HF conducting at well 15a, the surface pressure at injection is within the expected range and fully confirms the preliminary calculations of the required pump power, made in preparation for the project. Two pumps have enough power for HF of formation К1а.
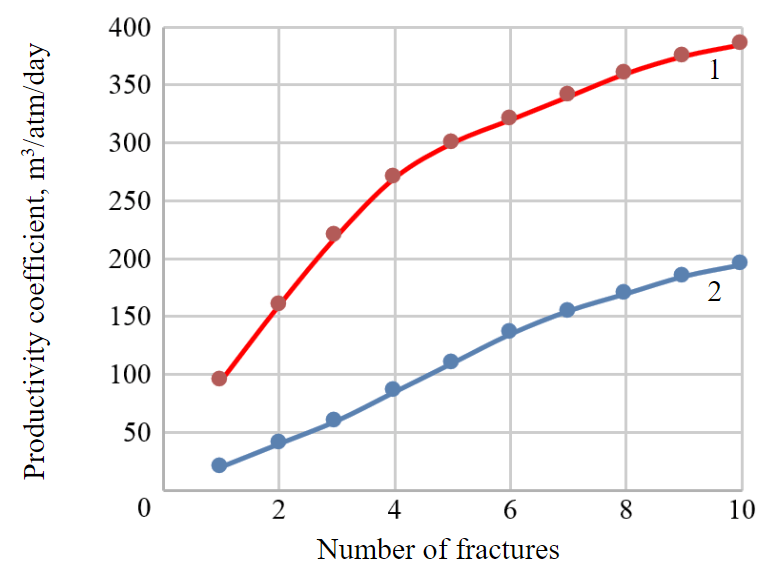
Fig.3. Productivity coefficient for longitudinal (1) and transverse (2) fractures
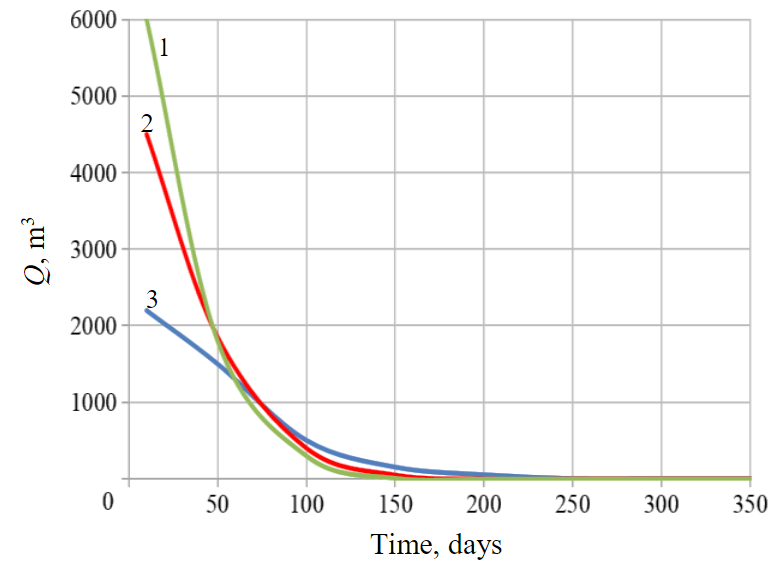
Fig.4. Well drilling scenario for different longitudinal fractures 1 – Nf = 1; 2 – Nf = 2; 3 – Nf = 3
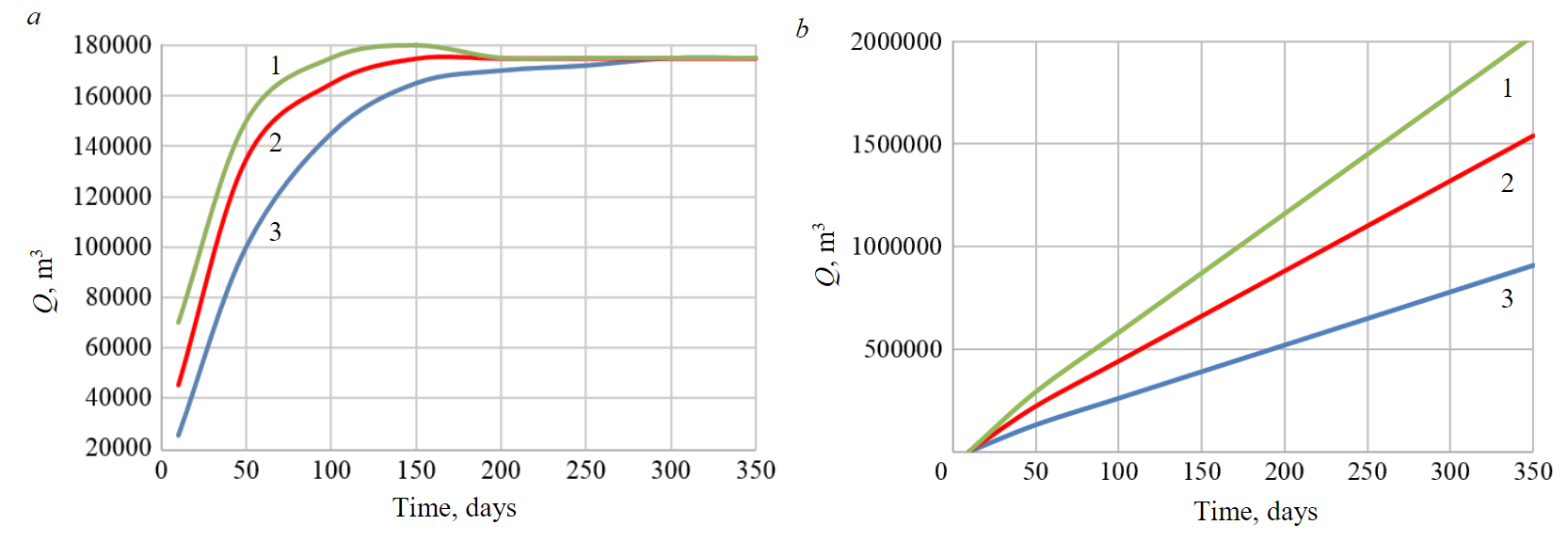
Fig.5. Cumulative production scenario for longitudinal fractures: а – I scenario; b – II scenario 1 – Nf = 1; 2 – Nf = 2; 3 – Nf = 3
The minimum estimated pressure gradient of fracture closure in formation К1а is 14.37 kPa/m.
An evaluation of friction pressure loss showed that friction loss prevail as the fluid moves through the tubing. The estimated total friction loss in the near-bottomhole zone is 3.4 atm at a flow rate of 3 m3/min.
Thermometry after the mini-test concluded that the fracture was growing between 1335 and 1376 m (along the wellbore).
During the main HF, 180 m3 cross-linked gel and 49000 kg of proppant were injected from the surface. A planned operating flow rate of 3.0 m3/min was achieved.
Interpretation report from the XMAC acoustic logging tool: the upper boundary of the HF fracture is in the lower part of the Alba formation (К1аl) at a depth of 1320 m (along the wellbore) or 1295 m (by absolute marks); the lower boundary of the HF fracture is in the lower part of the Apt formation (К1а) at a depth of 1371 m (along the wellbore) or 1345 m (by absolute marks); the XMAC instrument reached only 1373.4 m depth (along the wellbore) and the lower boundary of the interpretation is only 1371.5 m (along the wellbore); the assessment of the bottomhole pressure behaviour, lack of effective pressure variations shows a possible HF fracture development also in the lower interval of the К1а formation, located at the depth of 1361-1370 m (by absolute marks) [34, 35].
Conclusions
The most reliable results for the optimum number of fractures and their effect on well operation parameters are obtained from modeling the HF fracture design.
The direction of the minimum and maximum horizontal stress for selecting the orientation of the horizontal wellbore and the type of hydraulic fractures created when it is necessary to control their propagation into the water-saturated zone are determined.
The concept of HF design modeling has been found to include three different approaches:
- Determining the optimum direction of the horizontal wellbore and its spatial location in relation to minimum and maximum formation stresses plays a significant role in the design of a multiple hydraulic fracture operation.
Economic benefit from the use of HF technology at the field named after V.Filanovskiy
Indicator |
February 2021 |
July 2021 |
November 2021 |
Oil production, thousand tons |
35.4572 |
149.4096 |
13.8384 |
Commercial oil, thousand tons |
35.45649 |
149.4066 |
13.83812 |
Revenue, million rubles |
684.6117 |
3574.956 |
387.9644 |
Operating expenses, million rubles |
505.1065 |
193.0372 |
19.37293 |
Operating costs (with inflation), million rubles |
505.1065 |
196.8979 |
20.1556 |
Benefit from the ES, million rubles |
151.4169 |
846.8665 |
95.74611 |
MET, million rubles |
213.2751 |
1238.755 |
142.923 |
Income tax, million rubles |
–6.75399 |
427.8607 |
44.97716 |
Taxable income, million rubles |
–33.77 |
2139.303 |
224.8858 |
Cash flow from operating activities, million rubles |
–27.016 |
1711.443 |
179.9086 |
Cash flow from investing activities, million rubles |
0 |
0 |
0 |
Net cash flow, million rubles |
–27.016 |
1711.443 |
179.9086 |
Net cash flow, million $ |
–0.37008 |
24.27578 |
2.649612 |
Discounted net cash flow, million rubles |
–0.37008 |
21.10938 |
2.003487 |
Indicators for calculations |
2017 |
2018 |
2019 |
Property tax, % |
0.02 |
0.02 |
0.02 |
for capital expenses, units |
1.000 |
1.020 |
1.040 |
industry prices, units |
1.000 |
1.020 |
1.040 |
on electricity, units |
1.000 |
1.054 |
1.109 |
on salaries, units |
1.000 |
1.064 |
1.120 |
Nominal exchange rate of the national currency, rub/$ |
73 |
70.5 |
67.9 |
Discount coefficient, units |
1 |
0.869565 |
0.756144 |
Net oil price on an FCA basis, $/ton |
206 |
259 |
311 |
Increase in net price from duty exemptions, $/ton |
58.5 |
80.4 |
101.9 |
Oil price, rub/ton |
15038 |
18259.5 |
21116.9 |
MET rate |
6015 |
8291 |
10328 |
Commercial oil calculation coefficient, units |
0.99998 |
||
Self-cost of oil, rub/ton |
1611 |
1292 |
1399.94 |
NPV, million $ |
22.74278 |
- Two cases are theoretically possible – the horizontal wellbore is positioned strictly in the direction of the maximum or minimum horizontal stress.
Several criteria must guide the choice of an efficient technology – achieving maximum hydraulic fracture efficiency through maximum interference time, minimum damage to the created fractures and the reservoir, and maximum conductivity of the consolidated hydraulic fractures.
- The optimum geometry of the multiple hydraulic fractures created in a horizontal wellbore depends entirely on the choice of working fluid used, the fixing material and the timeliness and quality of the removal of the working fluid decomposition products.
Five hydraulic fractures were modeled on each of the 1000 m production and water injection wells and seven fractures were modeled on the 1500 m gas injection wells. The fractures are transverse to the horizontal wellbore of predominantly submeridian direction corresponding to the direction of the selected faults, with a port spacing of about 200 m. Parameters of the fractures are set based on the results of HF performed in well 15a. At wells 77-R and 78-Raptskaya deposit of field named after V.Filanovskiy, put into pilot development in November-December 2020, HF is schedu-led for early 2024.
Data from wells 15a and 16a were used (considering the ST). The time interval is 10 months – the time period of the HF effect. Operating costs have been taken into account in the calculation due to increased oil production.
At the field named after V.Filanovskiy, “LUKOIL-Inzhiniring” has obtained an economic benefit of $22.74278 million from the use of HF technology (see table).
The authors have found the following: the conducted optimization of HF design is aimed at crea-ting fractures of maximum conductivity and minimum length; the application of hydraulic fracturing technology during well construction allowed to increase vertical permeability and unite disparate parts of the reservoir in practice, which determined the efficiency of hydrocarbon field development in the field under study.
References
- Agzamov A.K., Efendiyev G.М., Moldabayeva G.Z. et al. Results of numerical experiments to establish the influence of drawdown degree on gas and condensate recovery factors. Oil and Gas Studies. 2022. N 4, p. 57-76 (in Russian). DOI: 10.31660/0445-0108-2022-4-57-76
- Bembel R.M., Sukhov V.A., Schetinin I.A. Ways of Increasing Geological Efficiency of Hydrocarbon Fields Development in Western Siberia. Oil and Gas Studies. 2017. N 6, p. 6-10 (in Russian). DOI: 10.31660/0445-0108-2017-6-6-10
- Bosikov I.I., Klyuev R.V., Egorova E.V. Assessment of Oil and Gas Potential Prospects of the North Eastern Unit of the South Khulym Deposit. Sustainable Development of Mountain Territories. 2019. Vol. 11. N 1 (39), p. 7-14 (in Russian). DOI: 10.21177/1998-4502-2019-11-1-7-14
- Gayduk V.V. The Nature of the Oil and Gas Potential of the Tersko-Sunzhensky Oil and Gas-Bearing Region. Geology, Geophysics and Development of Oil and Gas Fields. 2019. N 2, p. 40-46 (in Russian). DOI: 10.30713/2413-5011-2019-2-40-46
- Danilov V.N. Formation of Thrusts and Hydrocarbon Potential of Urals Foredeep. Russian Oil and Gas Geology. N 1, p. 57-72 (in Russian). DOI: 10.31087/0016-7894-2021-1-57-72
- Borodkin V.N., Smirnov O.A., Lukashov A.V. et al. Seismogeological Model and Petroleum Prospects of the Paleozoic-Mesozoic Strata Belonging to the North of Western Siberia on the Basis of 2D, 3D Seismic Exploration. Petroleum Geology. Theore-tical and applied studies. 2022. Vol. 17. N 2 (in Russian). DOI: 10.17353/2070-5379/18_2022
- Ivlev D.A. Method for Regional Forecast of Oil and Gas Potential Territories by Machine Learning Algorithms on the Example of the Tyumen Formation of Western Siberia. Bulletin of the Tomsk Polytechnic University. Geo Assets Engineering. 2021. Vol. 332. N 1, p. 41-53 (in Russian). DOI: 10.18799/24131830/2021/1/2998
- Kaukenova A.S. Oil and gas potential of the south Turgay basin. Proceedings of higher educational establishments. Geology and Exploration. 2020. N 3, p. 38-45 (in Russian). DOI: 10.32454/0016-7762-2020-63-3-38-45
- Kobylinsky D.A. Criteria for determining the oil and gas potential of the territory based on the data of ground geochemical survey performed on the ground and artificial sorbent. The Eurasian Scientific Journal. 2020. Vol. 12. N 6. N 51NZVN6.20.
- Bosikov I.I., Mazko A.I., Mayer A.V., Gagarina O.V. A Comprehensive Analysis of Conditions and Features of Oil-And-Gas Content within the Akhlovskaya Structural Zone (The North Caucasus). Oil and Gas Studies. N 2, p. 25-38 (in Russian). DOI: 10.31660/0445-0108-2021-2-25-38
- Panikarovskii E.V., Panikarovskii V.V., Anashkina A.E. Vankor oil field development experience. Oil and Gas Studies. 2019. N 1, p. 47-51 (in Russian). DOI: 10.31660/0445-0108-2019-1-47-51
- Nasibova G.J., Mukhtarova Kh.Z. Oil and gas prospects of the Shamakhy-Gobustan depression in relation to its structural and tectonic properties. Bulletin of the Tomsk Polytechnic University. Geo Assets Engineering. 2021. Vol. 332. N 4, p. 7-16 (in Russian). DOI: 10.18799/24131830/2021/04/3143
- Sevostyanova R.F., Sitnikov V.S. The Development of Ideas about the Structure and Oil and Gas Potential of Nepa-Botuoba Anteclise and Adjacent Part of Predpatomskii Trough. Journal of Mining Institute. 2018. Vol. 234, p. 599-603. DOI: 10.31897/PMI.2018.6.599
- Cherkashin V.I., Sabanaev K.A., Gadzhieva T.R. et al. Tectonic structure and prospects on oil and gas content in the sedimentary cover of the Caspian Sea bed. Trudy Instituta geologii Dagestanskogo nauchnogo tsentra RAN. 2018. N 4 (75), p.24-29 (in Russian). DOI: 10.31161/2541-9684-2018-62-4-25-30
- Ulmasvay F.S., Dobrynina S.A., Sidorchuk E.A. New Regularities in the Distribution of Oil and Gas in Sedimentary Stratum (by the Case of Ciscaucasia). Actual Problems of Oil and Gas. 2018. N 1 (20) (in Russian). DOI: 10.29222/ipng.2078-5712.2018-20.art8
- De Oliveira D.M., Sobral L.G.S., Olson G.J., Olson S.B. Acid leaching of a copper ore by sulphur-oxidizing microorganisms. Hydrometallurgy. 2014. Vol. 147-148, p. 223-227. DOI: 10.1016/j.hydromet.2014.05.019
- Klyuev R.V., Bosikov I.I., Mayer A.V., Gavrina O.A. Comprehensive Analysis of the Effective Technologies Application to Increase Sustainable Development of the Natural-Technical System. Sustainable Development of Mountain Territories. 2020. N 2, p. 283-290 (in Russian). DOI: 10.21177/1998-4502-2020-12-2-283-290
- Bosikov I.I., Klyuev R.V., Gavrina O.A. Analysis of geological-geophysical materials and qualitative assessment of the oil and gas perspectives of the Yuzhno-Kharbizhinsky area (Northern Caucasus). Geology and Geophysics of Russian South. Vol. 11, p. 6-21 (in Russian). DOI: 10.46698/VNC.2021.36.47.001
- Bosikov I.I., Mazko A.I., Mayer A.V. A comprehensive evaluation of the productive formation collector of the Kanevskoye field. Oil and Gas Studies. 2021. N 3, p. 25-36 (in Russian). DOI: 10.31660/0445-0108-2021-3-25-36
- Bosikov I.I., Klyuev R.V., Silaev I.V. Comprehensive analysis and assessment of Prospective gold-ore zones using modern geophysical methods. Geology and Geophysics of Russian South. 2022. Vol. 12. N 2, p. 89-102 (in Russian).
DOI: 10.46698/VNC.2022.32.98.007 - Litvinenko V.S. Digital Economy as a Factor in the Technological Development of the Mineral Sector. Natural Resources Research. 2020. Vol. 29, p. 1521-1541. DOI: 10.1007/s11053-019-09568-4
- Nassani A.A., AldakhilM., Zaman K. Ecological footprints jeopardy for mineral resource extraction: Efficient use of energy, financial development and insurance services to conserve natural resources. Resources Policy. 2021. Vol. 74. N 102271. DOI: 10.1016/j.resourpol.2021.102271
- Zhong Li, Renjun Xi, Yi Wu, Junliang Yuan. Progress and prospect of CNOOC's oil and gas well drilling and completion technologies. Natural Gas Industry B. 2022. Vol. 9. Iss. 2, p. 209-217. DOI: 10.1016/j.ngib.2021.08.020
- Xizhe Li, Zhenhua Guo, Yong Hu et al. High-quality development of ultra-deep large gas fields in China: Challenges, stra-tegies and proposals. Natural Gas Industry B. 2020. Vol. 7. Iss. 5, p. 505-513. DOI: 10.1016/j.ngib.2020.09.008
- Zengkai Liu, Qiang Ma, Xuewei Shi et al. A dynamic quantitative risk assessment method for drilling well control by integrating multi types of risk factors. Process Safety and Environmental Protection. 2022. Vol. 167, p. 162-172. DOI: 10.1016/j.psep.2022.08.066
- Jun Liu, Jianxun Wang, Xiaoqiang Guo et al. Investigation on axial-lateral-torsion nonlinear coupling vibration model and stick-slip characteristics of drilling string in ultra-HPHT curved wells. Applied Mathematical Modelling. 2022. Vol. 107, p. 182-206. DOI: 10.1016/j.apm.2022.02.033
- Sudharsan J., Khare S.K. Role of nanocomposite additives in well bore stability during shale formation drilling with water based mud – A comprehensive review. Materials Today: Proceedings. 2022. Vol. 62. Part 12, p. 6412-6419. DOI: 10.1016/j.matpr.2022.04.009
- Shingala J., Vora N., Shah M. Emerging the dual string drilling and dual coil tubing drilling technology in a geothermal well applications. Petroleum. 2021. Vol. 8. Iss. 1, p. 102-109. DOI: 10.1016/j.petlm.2021.03.007
- Dao-Bing Wang, Fu-Jian Zho, Yi-Peng Li et al. Numerical simulation of fracture propagation in Russia carbonate reservoirs during refracturing. Petroleum Science. 2022. DOI: 10.1016/j.petsci.2022.05.013
- Martyushev D.A., Ponomareva I.N., Filippov E.V., Li Yuwei.Formation of hydraulic fracturing cracks in complicated carbonate reservoirs with natural fracturing. Bulletin of the Tomsk Polytechnic University, Geo Assets Engineering. 2022. Vol. 333. N 1, p. 85-94 (in Russian). DOI: 10.18799/24131830/2022/1/3212
- Kunpeng Zhang, Bing Hou, MianChen et al. Fatigue acid fracturing: A method to stimulate highly deviated and horizontal wells in limestone formation. Journal of Petroleum Science and Engineering. 2022. Vol. 208. Part B. N 109409. DOI: 10.1016/j.petrol.2021.109409
- Liang Zhang, ZhiPing Li, Feng Peng Lai et al. Integrated optimization design for horizontal well placement and fracturing in tight oil reservoirs. Journal of Petroleum Science and Engineering. 2019. Vol. 178, p. 82-96. DOI: 10.1016/j.petrol.2019.03.006
- Khan R.A., Awotunde A.A. Determination of vertical/horizontal well type from generalized field development optimization. Journal of Petroleum Science and Engineering. 2018. Vol. 162, p. 652-665. DOI: 10.1016/j.petrol.2017.10.083
- Huang L.A., Peijia Jiang, Xuyang Zhao et al. Modeling Study of the Productivity of Horizontal Wells in Hydrocarbon-Bearing Reservoirs: Effects of Fracturing Interference. Geofluids. 2021. Vol. 2021. N 2168622. DOI: 10.1155/2021/2168622
- Wuguang Li, Hong Yue, Yongpeng Sun et al. Development Evaluation and Optimization of Deep Shale Gas Reservoir with Horizontal Wells Based on Production Data. Geofluids. 2021. Vol. 2021. N 4815559. DOI: 10.1155/2021/4815559