Feasibility study of using cogeneration plants at Kuzbass coal mines
- 1 — Ph.D. Senior Researcher T.F.Gorbachev Kuzbass State Technical University ▪ Orcid
- 2 — Ph.D. Researcher T.F.Gorbachev Kuzbass State Technical University ▪ Orcid
- 3 — Technician T.F.Gorbachev Kuzbass State Technical University ▪ Orcid
- 4 — Researcher T.F.Gorbachev Kuzbass State Technical University ▪ Orcid
Abstract
The paper considers the problem of reducing greenhouse gas emissions in the process of coal mining during the coal mine methane utilization in power supply systems. An algorithm to form recommendations for the implementation of CMM generation is presented. A simulation model for one of the Kuzbass coal mines was developed in the PowerFactory software application. The simulation model considers the uneven nature of the power consumption of mining equipment. As a result of modeling, daily power consumption profiles and voltage levels in the coal mine power supply system were determined before and after the implementation of the proposed measures. Based on the results, the technical and economic effects was estimated, which consisted in reducing the direct and indirect carbon footprint, electricity and capacity fees. It has been established that the cost of carbon dioxide emission quotas significantly affects the investment attractiveness of cogeneration projects. Based on the results, recommendations are given to stimulate the development of small generation in coal mines.
Introduction
According to the Russian Coal Industry Development Program to 2035, the competitiveness of Russian coal companies will increase due to the technological development of the coal industry: modernization and technical re-equipment; implementation by coal companies of high-performance technologies, digital technologies in the processes of coal mining and processing. In accordance with the Order of the Government of the Russian Federation N 1144-r dated by 11th of May 2022, large investments are aimed at creating a set of technologies that increase the efficiency of coal mining and processing. Serious challenge for the development strategy of the coal industry in Russia is the need to “decarbonize coal mining” to compensate for the negative effects of greenhouse gas emissions into the environment. “Greening of national energy consumption” is one of the possible solutions aimed at reducing the carbon footprint of finished products by increasing the share of “clean” energy sources in the energy balance of a coal mine.
In accordance with the Strategy of social and economic development of the Russian Federation with the low level of greenhouse gas emissions until 2050* among the measures aimed at implementing the strategy, the following are noted: development of distributed generation; creation of additional sources of power generation; development and implementation of coal mine methane (CMM) utilization technologies. Coal mining enterprises provide 11 % of anthropogenic methane emissions [1]. According to [2] anthropogenic methane accounts for about 18 % of global greenhouse gas emissions into the atmosphere, and coal mine methane accounts for up to 12 % of global anthropogenic methane emissions [3]. About 70 % of CMM is emitted by the ventilation systems of coal mines. In Russia, the underground method of coal mining determines 85 % of emissions from the methane coal industry [4]. Some researchers note that methane emissions from minerals may be 60-150 % higher than current estimates [5, 6]. Although these studies do not consider coal mines separately, they show how underestimated methane emissions from mining are. The prospects for extracting methane in mines of the Russian Federation are limited due to its insufficient representation as an internal source of growth in the profitability of coal production [7].
There is also an economic side to the issue of CMM utilization. The countries of Europe and Asia are actively discussing the introduction of a carbon border tax, the main task of which is to attract additional investments to solve the problem of global climate change [8, 9]. The carbon border tax stimulates investments aimed at improving the energy efficiency of production [10]. The object of taxation is the volume of greenhouse gas emissions that accompanies production. European producers are already incurring costs of a similar nature to the carbon border tax. Since 2005, the European Union Emissions Trading System (EU ETS) has been operating. The average price of futures per unit of carbon dioxide emission reduction (EU ETS) for 2022 averaged 80-84 euro [11], while in Russia the possibility of introducing such mechanism is only being discussed [12].
Developing of in-home generating capacities (for the utilization of CMM from the degassing system of a coal mine) provides energy flexibility at the coal mining enterprise, which, along with the possibility of using load-regulated consumers and energy storage devices [13], makes it possible to reduce the cost of coal production [14]. The relevance and necessity of integrated implementation of technologies and principles of distributed generation in the conditions of enterprises of the mineral resource complex are substantiated in [15]. It will also allow attracting additional investments: to ensure the stability of the elements of the degassing system [16]; to develop the blockchain platforms [17]; to participate in the provision of demand management services (with an increase in renewable energy production) [18, 19] and other energy flexibility services [20, 21].
The development of this area requires the development of the electricity market, considering the specifics of the energy transition [22]. Thus, it is expedient to consider the introduction of a CMM utilization system for organizing its own distributed generation at a coal mining enterprise.
The problem statement
CMM and other associated gases were considered by many researchers as an energy source. In [23], a methodology for assessing the economic and environmental prospects of CMM utilization was formulated. The proposed methodology was applied to assess the prospects of this approach for Australian mines, conclusions were drawn about a significant reduction in methane emissions into the atmosphere and the economic effect of its combustion. The study [24] considers the prospects for CMM generation in India. 18 mines were analyzed and as a result, approximate payback periods for projects (3.5-6 years) were obtained, except for three unprofitable cases, which shows the high potential of such projects. The paper [25] analyzes 30 projects in China aimed at reducing CMM emissions into the atmosphere, 16 of which involve the use of methane as an alternative energy resource.
An overview of the possibilities of generation at oil production facilities using natural or associated gas is given in [26, 27]. The article considers the optimization of a cogeneration system with a binary cycle – electrical and thermal energy, with the possibility of creating additional capacities with a small need for heat. Such a system may be promising for coal mines using CMM as a primary energy carrier. The issues of diversifying the economic activity of coal mining enterprises based on cogeneration are widely disclosed in [28]. The expediency of creating mine energy complexes using methane-air mixture extracted by underground degassing wells has been proved. The work [29] is devoted to the issues of using CMM to provide electricity and heat supply to a data processing center created on the basis of a coal mine infrastructure. There are also studies [3, 30], aimed at improving the efficiency of using CMM with an efficiency close to 95 % when using gas piston units operating on a cogeneration or trigeneration cycle. The publication [31] considers the idea of joint use of CMM and biogas. It is shown that in this case the payback period will be about two years. The utilization of ventilation methane with a concentration of less than 3.5 % [32, 33] is very promising (in China, its utilization can produce up to 2610 GW∙h/year [34]).
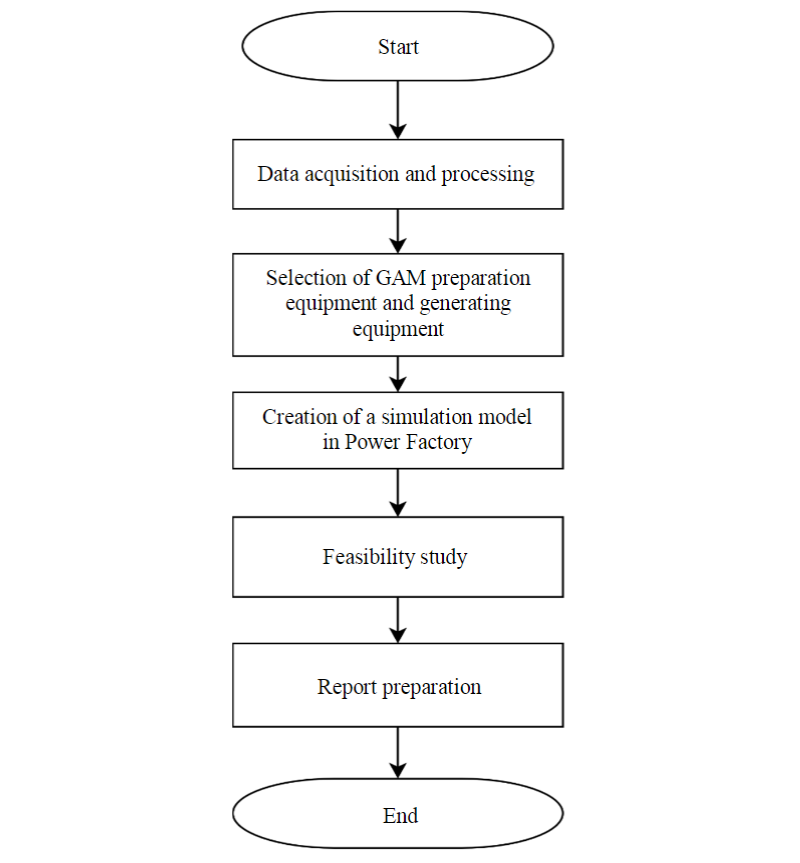
Fig.1. Formation of recommendations for the implementation of distributed energy facilities based on simulation modeling
Nevertheless, the issue of introducing CMM utilization in the conditions of Kuzbass coal mines has not been sufficiently studied and does not allow us to draw conclusions about the advisability of introducing cogeneration at the stages of construction and re-equipment of coal mines.
The efficiency of power generation based on CMM and its inclusion in the power supply system of a coal mine are evaluated using a simulation model developed in the DIgSILENT PowerFactory software application.
Methodology
The feasibility study of introducing distributed generation on the CMM was carried out according to the algorithm (Fig.1), which includes the following steps:
- Data acquisition and processing. At this stage, interaction with the enterprise is carried out, the following data is requested: equipment parameters; electrical load and generation profiles (in the presence of distributed energy facilities); information about the technological process (if it needs to be considered when forming recommendations); data on tariffs for electricity (contract on electricity supply); power supply scheme. The data is processed, the equivalent circuit parameters are calculated, which are used when creating a model in PowerFactory.
- Selection of gas-air mixture (GAM) preparation equipment and generating equipment. The composition of the generating equipment is selected based on information about the characteristics of the methane-air mixture transported by the coal mine degassing system. At CMM low concentration (less than 3.5 %), it is necessary to consider the installation of equipment for CMM purification and enrichment using adsorption and membrane technologies.
- Creation of a simulation model in PowerFactory using the received data. To generate a consumption profile for a shearer and a scraper conveyor, we used the results from studies [35, 36]. Finally, the model is verified using actual measurements of load current and voltage levels.
- Feasibility study. Two software modules have been developed: for the electricity cost calculation and the financial and economic model construction. The feasibility study also considers the reduction of the carbon footprint in the process of CMM utilization (Fig.2) and the reduction of electricity consumption.
- Report preparation. The report contains information on the issue of the proposed recommendations effectiveness.

Fig.2. Considered option for CMM utilization [37]
Results and discussion
Results and discussion. The considered mine operates around the clock. The power supply system of the considered coal mine includes the following power facilities: power transformers (transformers of main step-down substation (2 ´ 16 MVA), underground mine substations (1250-3150 kVA); high-voltage and low-voltage cable network; shearer (cutting drive 2 ´ 825 kW and combine feed 2 ´ 150 kW); crusher (crusher drive 1 ´ 400 kW); reloader (reloader drive 1 ´ 400 kW); belt conveyor (belt conveyor drive 3 ´ 500 kW); generalized load (underground mine substations, auxiliary power, ventilation, heater); reactive power compensation devices placed on the surface.
Estimation of CMM generation
CMM power generation depends on the potential amount of energy contained in cubic meter of the GAM, which ventilates from the mine. Indicative methane capture level is about 16.3 m3/min, methane concentration – 44 %.
The calorific value of the GAM can be obtained with the use of D.I.Mendeleev’s formula
where LCVi – lower heating value of methane, MJ/m3; ai – share of the i-gas in the GAM; Pg – useful heat which is equivalent to electric power generation, kW; R – gas yield, m3; 0.28 – percentage of energy contained in methane that can be converted into effective power.
Data on the scope of GAM issuance and methane concentrations (Fig.3) used to estimate electricity generation are shown in Fig.3.
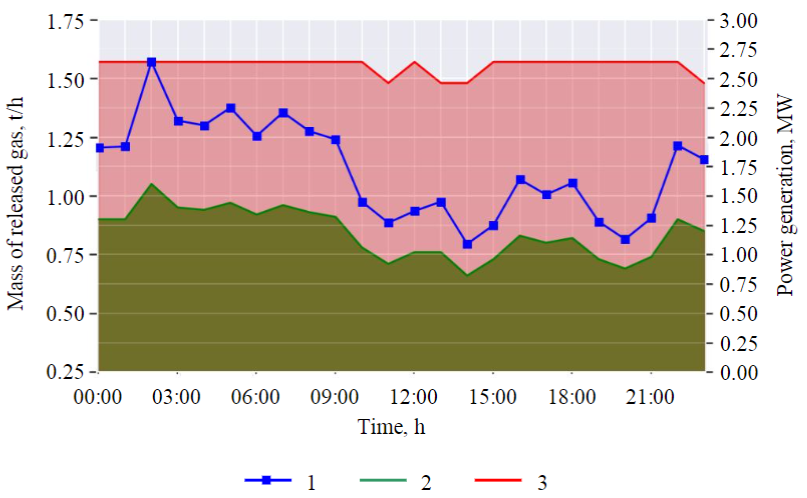
Fig.3. Daily production of GAM and CMM, daily profile of CMM generation 1 – generated power; 2 – mass of CH4 emissions; 3 – mass of GAM emissions

Fig.4. Simulation model of the power supply system of a coal mine in power system analysis software application PowerFactory (EPDS – electric power distribution station, UPDS – underground power distribution station, TS – transformer substation, EPSG – explosion-proof switchgear) 1 – GPU; 2 – coal mine extraction area; 3 – main step-down substation
To transfer GAM consumption into the mass of the evolved gas, the formula is used
where V – GAM flow rate, m3/min; ρ – GAM density, kg/m3; s – GAM concentration, %.
Based on the calculated CMM generation profile, it can be concluded that the average energy production for CMM will not exceed 2.4-2.6 MW. Based on the nature of the fuel (natural gas) and the operation mode which implies the loading of generating equipment within 30-80 % in 95 % operating time, a gas-piston power plant of the block-container type AG-3000S-T400-3RN was chosen. The AG-3000 includes 10 gas piston units (GPUs) with a rated capacity of 315 kW for a rated voltage of 0.4 kV. The methane-air mixture is supplied through the main pipeline to 10 generator sets. In the simulation model, a gas-piston power plant is implemented using two GPU models, the parameters of which are equal to the parameters of five parallel GPUs. Two step-up transformers 6/0.4 kV are used to output GPUs’ power.
At the modelling stage, only the normal mode was considered (Fig.4). In this case, the calculations were performed for each hour based on a real schedule of electrical loads for one of the Kuzbass coal mines (Fig.5).

Fig.5. The considered coal mine daily load profile. Power, consumed from the external grid, without CMM generation (1) and with CMM generation (2) Pg, Pl – electrical energy, generated and consumed from the external grid
Results of the implementation of the CMM utilization system: maximum load of substation transformers is reduced by 9.51 % (from 69.23 to 59.72 %); minimum voltage level at underground power distribution station N 5 is increased by 0.83 % (from 6.12 to 6.17 kV).
Electrical energy cost calculation
Electrical energy and power costs are calculated for the 4th price category based on estimated daily demand profiles, considering the medium voltage level and maximum load power being 10 MW at least. Tariffs are taken, according to PAO “Kuzbassenergosbyt”. Electrical power cost is calculated, considering peak, and planned peak load hours, according to AO “ATS” and AO “SO UPS”, respectively. A power and energy cost calculation module is developed, based on the data mentioned above. The option to sell the power to the external power grid is not considered because the power demand of the coal mine is always higher than its CMM generation.
Carbon footprint estimation
Carbon footprint estimation. CO2 emissions from stationary fuel combustion are calculated using default methods, based on different sources, sources groups, or organization in total:
where j – fuel type, used in combustion; n – amount of fuel type, used within a period (in this paper only methane is considered – n = 1); FCj year – j-fuel consumption over a year, t (thousand m3, tons of conventional fuel or TJ); EFCO2j year – CO2 emissions coefficient of j-fuel combusting over a year, which is determined by methane concentration, carbon dioxide density and moles of carbon, created after combustion, t; OFj year – j-fuel oxidation factor (taken equal 1 for gaseous fuels).
Indirect carbon footprint is estimated according to a carbon dioxide emission, which is presented on the ATS website [38],
where Pcons j – power, consumed from the external grid over an hour j; E(CO2 j) plan – planned CO2 emissions coefficient, t/MW∙h.
The effect of CMM utilization is calculated by the following expression:
where ECO2 year – emissions level after applying CMM generation, t; E(CO2 year) util – emissions level before applying CMM generation, t.
Quota payment reduction is estimated by the following expression:
where ETSyear – greenhouse gases emission payment; PETSCO2 – CO2 emissions payment (16 rub./t); PETSCH4 – CH4 emissions payment (108 rub./t).
In quota payment reduction calculation, it is necessary to consider that, while CMM utilization, the GPU creates 2.75 kg of CO2 for 1 kg of methane [39].
Economic profit estimation
In terms of income, the economic model considers the following: electrical energy payment reduction, emissions payment reduction, and heating cost reduction due to generation of GPU thermal power (estimated, based on coal buying cost, which combustion power is equal to heating power of the GPU). Heat outcome over a year is 12971.7 Gcal. In terms of expenses, the economic model considers the following: capital investments for buying GPUs and operational costs, which include regular maintenance, oil replacement, and major overhaul. In total, capital investments, incomes, and operating expenses are 165750; 52489; 13282 thousand rub.
A result of the net present value (NPV) calculation is a payback period of five years, considering the discount rate as 7.5 % (taken, based on the author’s forecast of refinancing rate at the end of the 2022). A detailed list of technical and economic indicators is shown in the Table 1 and 2, respectively. For the calculation of electrical power cost the following tariffs are taken: power capacity – 804110.8 rub./MW·month; services for energy transmission – 794057.7 rub./MW·month.
Table 1
Technical indicators comparison over a year
Technical indicators |
Without CMM generation |
With CMM generation |
Total effect |
Total grid electrical power consumption, MW∙h |
93921.04 |
78834.26 |
15086.78 |
Average electrical power, purchased from external grid, MW |
11.4 |
9.8 |
1.6 |
Average electrical power, transmitted from external grid, MW |
11.6 |
10.0 |
1.6 |
GPU heat generation, Gcal |
0.0 |
12971.2 |
12971.2 |
Direct CO2 emissions in atmosphere ECO2, t |
16270 |
36827.9 |
20557 |
Direct CH4 emissions in atmosphere ECH4, t |
7475.28 |
0 |
–7475.28 |
Indirect CO2 emissions in atmosphere ECO2, t |
84.9 |
71.3 |
–13.6 |
Table 2
Economic indicators comparison over a year
Economic indicators, thousand rub. |
Without CMM generation |
With CMM generation |
Total effect |
Electrical energy cost |
135521,1 |
113926,1 |
21595,0 |
Electrical power cost |
220170,7 |
189341,2 |
30829,5 |
Indirect carbon footprint payment, by tariff: |
|||
RU |
0,0 |
0,0 |
0,0 |
EU |
148770,9 |
124873,5 |
23897,5 |
Direct carbon footprint payment, by tariff: |
|||
RU |
954,0 |
589,2 |
364,8 |
EU |
286200,0 |
176760,0 |
109440,0 |
Operational costs |
0 |
–13282,0 |
–13282,0 |
Total economic effect, by tariff: |
|||
RU |
365645,2 |
303856,5 |
39207,3 |
EU |
790661,8 |
604899,3 |
172480,5 |
On Fig.6 NPV dynamic is shown for the different values of CO2 emissions tariffs. Data shows that CO2 emission tariff affects a project’s investment attractiveness greatly: the current tariff gives a payback period of 5.2 years; in the case of emissions trading mechanism integrating (analogically to EU countries) payback period could be lowered to 1.2 years.
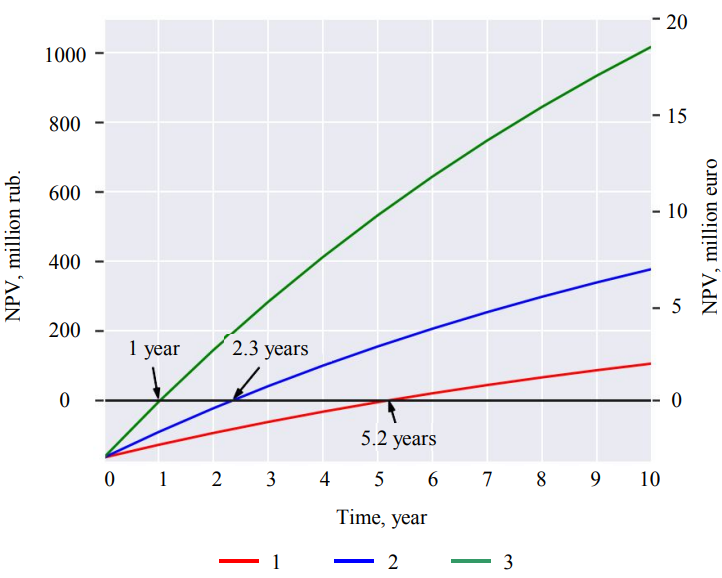
Fig.6. NPV change profile for different CO2 emission tariffs Tariffs: 1 – 16; 2 – 1440; 3 – 4800 rub./t CO2
Conclusions
The global trend of decarbonization of production and the inevitability of the energy transition pose a threat to the sustainability of the coal mining industry.
In this regard, it is required to study various options for reducing the carbon footprint of finished products. One of the options is the construction of facilities for the CMM utilization.
To evaluate the effect of GPU introduction, a simulation model was developed that takes into account the hourly change in consumption and generation profiles, depending on the concentration and volume of methane inflow from a coal mine.
The simulation results showed that the introduction of CMM generation reduces the payment for electricity, while minimizing the amount of carbon dioxide emissions. With the implementation of the mechanisms of the “green economy” when calculating the fee for CO2 emissions, the investment attractiveness of such projects can increase by four times. However, to achieve such a state, serious work is required to form a national market for “quotas for emissions” of greenhouse gases into the atmosphere.
Global trends in the transition to carbon-free and low-carbon energy encourage enterprises to use distributed generation. In this case, the choice of the optimal strategy for the energy development of a coal mining enterprise is especially important.
The developed simulation model allows for further research of the coal mine power supply system in terms of assessing measures for the introduction of distributed generation on CMM.
References
- Global Non-CO2 Greenhouse Gas Emission Projections & Mitigation Potential: 2015-2050. URL: https://www.epa.gov/global-mitigation-non-co2-greenhouse-gases/global-non-co2-greenhouse-gas-emission-projections (accessed 27.07.2022).
- Climate Change 2022: Mitigation of Climate Change. URL: https://www.ipcc.ch/report/ar6/wg3/ (accessed 28.07.2022).
- Yuan-Ping Cheng, Lei Wang, Xiao-Lei Zhang. Environmental impact of coal mine methane emissions and responding strategies in China. International Journal of Greenhouse Gas Control. 2011. Vol. 5. Iss. 1, p. 157-166. DOI: 10.1016/j.ijggc.2010.07.007
- Tailakov O.V., Zastrelov D.N., Utkaev E.A. et al. Prospects of the Coal Mine Methane Utilization. Bulletin of the Kuzbass State Technical University. 2012. N 6 (112), p. 62-66 (in Russian).
- Schwietzke S., Sherwood O.A., Bruhwiler L.M.P. Upward revision of global fossil fuel methane emissions based on isotope database. Nature. 2016. Vol. 538, p. 88-91. DOI: 10.1038/nature19797
- Miller S.M., Wofsy S.C., Michalak A.M. Anthropogenic emissions of methane in the United States. Proceedings of the National Academy of Sciences of the United States of America. 2013. Vol. 110. Iss. 50, p. 20018-20022. DOI: 10.1073/pnas.1314392110
- Dzhioeva A.K., Brigida V.S. Spatial non-linearity of methane release dynamics in underground boreholes for sustainable mining. Journal of Mining Institute. 2020. Vol. 245, p. 522-530. DOI: 10.31897/PMI.2020.5.3
- Vaughan A.EU’s carbon tax will apply globally. New Scientist. 2021. Vol. 251. Iss. 3344, p. 18. DOI: 10.1016/S0262-4079(21)01276-8
- 9. Kiss T., Popovics S. Evaluation on the effectiveness of energy policies – Evidence from the carbon reductions in 25 countries. Renewable and Sustainable Energy Reviews. 2021. Vol. 149. N 111348. DOI: 10.1016/j.rser.2021.111348
- Dorsey-Palmateer R., Niu B. The effect of carbon taxation on cross-border competition and energy efficiency investments. Energy Economics. 2020. Vol. 85. N 104602. DOI: 10.1016/j.eneco.2019.104602
- EU Carbon Permits – 2022 Data – 2005-2021 Historical – 2023 Forecast – Price – Quote. URL: https://tradingeconomics.com/commodity/carbon (accessed 26.06.2022).
- Balashov M.M. The Impact of Carbon Regulation Mechanisms on the Development of Industry in the Russian Federation. Strategic decisions and risk management. 2020. Vol. 11. N 4, p. 354-365 (in Russian). DOI: 10.17747/2618-947X-2020-4-354-365
- Ustinov D.A., Sychev Ya.A., Kovalchuk M.S. et al. Development Trends of Industrial Energy Storage Units for Sustainable Development of Mineral Complex Facilities. Russian Mining Industry. 2021. N S5-2, p. 89-96 (in Russian).
- Nepsha F.S., Krasilnikov M.I., Perevalov K.V. Application of the Digital Platform for Building Intelligent Power Supply Management Systems for the Mining Industry. Avtomatizaciya i IT v energetike. 2021. N 5 (142), p. 26-34.
- Abramovich B.N., Sychev Yu.A., Ustinov D.A. Efficiency of distributed energy in the conditions of the mineral resource complex. Promyshlennaya energetika. 2019. N 5, p. 8-16.
- Brigida V.S., Golik V.I., Dmitrak Y.V., Gabaraev O.Z. Ensuring Stability of Undermining Inclined Drainage Holes During Intensive Development of Multiple Gas-Bearing Coal Layers. Journal of Mining Institute. 2019. Vol. 239, p. 497-501. DOI: 10.31897/PMI.2019.5.497
- Zhukovskiy Y.L., Semenyuk A.V., Alieva L.Z., Arapova E.G. Blockchain-based Digital Platforms to Reduce the Carbon Footprint of Mining. Mining Informational and Analytical Bulletin. 2022. N 6-1, p. 361-378 (in Russian). DOI: 10.25018/0236_1493_2022_61_0_361
- Lashmar N., Wade B., Molyneaux L., Ashworth P. Motivations, barriers, and enablers for demand response programs: A commercial and industrial consumer perspective. Energy Research & Social Science. 2022. Vol. 90. N 102667. DOI: 10.1016/j.erss.2022.102667
- 19. Ratner S.Chapter 10 – Smart grids in Russia: status, barriers, and prospects for development. Recent Advances in Renewable Energy Technologies. London: Academic Press, 2022, p. 377-402. DOI: 10.1016/B978-0-12-823532-4.00010-0
- Villar J., Bessa R., Matos M. Flexibility products and markets: Literature review. Electric Power Systems Research. 2018. Vol. 154, p. 329-340. DOI: 10.1016/j.epsr.2017.09.005
- Degefa M.Z., Sperstad I.B., Sæle H. Comprehensive classifications and characterizations of power system flexibility resources. Electric Power Systems Research. 2021. Vol. 194. N 107022. DOI: 10.1016/j.epsr.2021.107022
- Mitrova T., Melnikov Y. Energy transition in Russia. Energy Transit. 2019. Vol. 3. N 73-80. DOI: 10.1007/s41825-019-00016-8
- Meybodi M.A., Behnia M. Australian coal mine methane emissions mitigation potential using a Stirling engine-based CHP system. Energy Policy. 2013. Vol. 62, p. 10-18. DOI: 10.1016/j.enpol.2013.07.040
- Hummel J.A., Ruiz F.A., Kelafant J.R. Quantifying the benefits of coal mine methane recovery and use projects: Case study on the application of in-mine horizontal pre-drainage boreholes at gassy coal mines in India and the optimization of drainage system design using reservoir simulation. Environmental Technology & Innovation. 2018. Vol. 10, p. 223-234. DOI: 10.1016/j.eti.2018.03.003
- Uddin N., Blommerde M., Taplin R., Laurence D. Sustainable development outcomes of coal mine methane clean development mechanism Projects in China. Renewable and Sustainable Energy Reviews. 2015. Vol. 45, p. 1-9. DOI: 10.1016/j.rser.2015.01.053
- Bogdanov I.A., Veprikov A.A., Kasyanova A.N., Morenov V.A. Increase of Energy Efficiency of Electrotechnical Complexes of Cogeneration Plants for Power Supply of Objects of Oil And Gas Enterprises. International Research Journal. 2017. N 12-5 (66), p. 59-63 (in Russian). DOI: 10.23670/IRJ.2017.66.144
- Shklyarskiy Ya. E., Salov R.A. Improvement in Efficiency of Power Plants Based on Associated Petroleum Gas. News of the Tula state university. Technical sciences. 2017. N 12-2, p. 484-492 (in Russian).
- Yutyaev A.E., Belyaev V.V., Agafonov V.V. Cogeneration of Resource-Saving Technologies in Coal Mining. Mining Informational and Analytical Bulletin. 2013. N 6, p. 69-74 (in Russian).
- Varnavskiy K., Nepsha F., Kostomarov R., Chen Q.G. Underground hard-coal mine as a hosting facility for a data processing center infrastructure. XX Conference of PhD Students and Young Scientists, 14-16 October 2020, Poland. IOP Conference Series: Earth and Environmental Science. 2021. Vol. 684. N 012015. DOI: 10.1088/1755-1315/684/1/012015
- 30. Karacan C.Ö., Ruiz F.A., Cotè M., Phipps S. Coal mine methane: A review of capture and utilization practices with benefits to mining safety and to greenhouse gas reduction. International Journal of Coal Geology. 2011. Vol. 86. Iss. 2-3, p. 121-156. DOI: 10.1016/j.coal.2011.02.009
- Feifei Yin, Baisheng Nie, Yueying Wei, Shuangshuang Lin. Co-Production System Based on Lean Methane and Biogas for Power Generation in Coal Mines. Atmosphere. 2022. Vol. 13. Iss. 5. N 803. DOI: 10.3390/atmos13050803
- Xinxin Wang, Fubao Zhou, Yihan Ling et al. Overview and Outlook on Utilization Technologies of Low-Concentration Coal Mine Methane. Energy Fuels. 2021. Vol. 35. Iss. 19, p. 15398-15423. DOI: 10.1021/acs.energyfuels.1c02312
- Kaledina N.O., Malashkina V.A. Industrial extraction of methane in operating coal mines by the complex system “Ventilation-degassing”. Mining Informational and Analitical bulletin. 2006. N S5, p. 413-425.
- Fubao Zhou, Tongqiang Xia, Xinxin Wang et al. Recent developments in coal mine methane extraction and utilization in China: A review. Journal of Natural Gas Science and Engineering. 2016. Vol. 31, p. 437-458. DOI: 10.1016/j.jngse.2016.03.027
- Voronin V.A., Nepsha F.S. Simulation of the electric drive of the shearer to assess the energy efficiency indicators of the power supply system. Journal of Mining Institute. 2020. Vol. 246, p. 633-639. DOI: 10.31897/PMI.2020.6.5
- Voronin V.A., Nepsha F.S. Modelling and Simulation of Scraper Face Conveyor Electric Drive. 2020 Ural Smart Energy Conference (USEC), 13-15 November 2020, Ekaterinburg, Russia. IEEE, 2020, p. 63-67. DOI: 10.1109/USEC50097.2020.928120237
- Amidpour M., Man M.H.K. Cogeneration and Polygeneration Systems. London: Academic Press, 2020, p. 406.
- Carbon dioxide emission factor for the First Synchronous Zone of the UES of Russia AO “ATS”. URL: https://www.atsenergo.ru/results/co2 (accessed 05.08.2022).
- Savitenko M.A., Rabkov B.A. Hydrogen application for power generation: ecological issues. Turbiny i dizeli. 2021. N 1, p. 10-16.