Prospecting models of primary diamond deposits of the north of the East European Platform
Abstract
As a result of a comprehensive study of the geological structure and diamond presense of the northern part of the East European Platform, generalization of the data accumulated by various organizations in the USSR, the Russian Federation, and other states, three main prospecting models of primary diamond deposits have been identified and characterized: Karelian, Finnish, and Arkhangelsk. Geological, structural, mineralogical, and petrographic criteria of local prediction, as well as the features of the response of kimberlite and lamproite bodies in dispersion haloes and geophysical fields, are considered using known examples, including data on the developed M.V.Lomonosov and V.P.Grib mines. It is shown that the most complicated prospecting environments occur in the covered areas of the Russian Plate, where, in some cases, the primary diamond-bearing rocks are similar in their petrophysical properties to the host formations. The buried dispersion haloes of kimberlite minerals in the continental Carboniferous and Quaternary deposits are traced at a short distance from the sources. Differences in the prospecting features of magnesian (Lomonosov mine) and ferromagnesian (Grib mine) kimberlites are also shown. Conclusions about the diamond potential of the model objects of various types are given in this paper.
Introduction
The East European Platform (EEP) is a large diamond-bearing province, where two deposits (named after M.V.Lomonosov and V.P.Grib) are currently being developed. In recent years, about 20 % of Russian and 6-7 % of world diamonds have been mined from these deposits. They are located within the Karelian-Kola subprovince in the north of the EEP. The Karelian-Kola diamond subprovince has an area of over 1.5 million square kilometers and covers Arkhangelsk, Vologda, Murmansk, Leningrad, Pskov, and Novgorod regions, Karelia, and Finland. About 100 pipes, as well as more than 100 dikes, sills, and stockworks of kimberlites and lamproites, differing in diamond grades, have been found within this subprovince. Despite a considerable amount of prospecting work, no deposits were discovered after the discovery of the Grib pipe (in 1996). The current problem of the Russian diamond mining industry is to replenish the exhausting diamond reserves by new discoveries. This article is focused on prospecting models of primary diamond deposits, which are predicted within the territories of the northern part of the EEP characterized by different exploration evironments.
Geological structure of the territory and diamond presense
The East European platform with an Epikarelian crystalline basement consists of tectonic elements of different types, scales, and ages. In terms of minerageny, EEP is a diamond province, and its largest Archean megablocks (Karelian-Kola, Volga-Ural, and Voronezh-Ukrainian) are subprovinces. Among them, is the Karelian-Kola subprovince, where the vast majority of the known diamondiferous and potentially diamondiferous kimberlites and lamproites occur, including the Lomonosov and Grib mines, has the highest potential.
In the northern part of the EEP, two superorder geological structures are distinguished – the Baltic (Fennoscandian) shield and the Russian Plate. The first is a deeply metamorphosed folded platform basement, characterized by a complex block structure. In the southern and eastern directions, the basement rocks a slightly submerged under the sedimentary cover of the Russian Plate and its constituent Mocovskaya and Mezenskaya syneclises. There are three structural stages in the geolo-gical structure of the northern part of the EEP. The lower one is the Archean-Lower Proterozoic folded crystalline basement of the Platform. The middle one is composed of non-folded Riphean and Lower Vendian formations preserved in separate troughs and grabens. The upper stage is represented by shallow dipping Upper Vendian and Paleozoic sediments, which form a continuous cover of the Russian Plate.
Within the Karelian-Kola megablock (megacraton), where all the currently known manifestations of kimberlite and lamproite magmatism in the north of the EEP and areas promising for prospecting for diamond deposits are concentrated, three major geostructural units (blocks of the first order) are distinguished: Murmansk, Kola (Kola-Kuloi), and Karelian, each of them is characterized by its features of the geological structure and development in the Precambrian and Phanerozoic time. By deep faults they are divided into blocks of the Earth's crust (terrains) of higher orders (Fig.1), which differ from each other by the history of geological development, geophysical features, deep structure, the intensity of tectonic and thermal reworking, and, accordingly, by the prospects of discovery of diamond deposits.
In the history of the development of the Earth's crust of the Karelian-Kola subprovince, several major stages (tectonic megacycles) can be distinguished: Archean, Early Proterozoic (Karelian), Late Proterozoic, and Phanerozoic. During the tectonic regime of any of these megacycles, periods of tectonic and magmatic activity (TMA), associated with the intrusion of diamond-bearing magmatic rocks, are locally manifested. At present, five epochs of diamond-bearing magmatism have been reliably established in the north of the EEP: Early Proterozoic, Middle Riphean, Late Riphean, Late Riphean-Early Vendian, and Middle Paleozoic.
The Archean megacycle (>3500-2500 Ma) corresponding to the acron is considered a pre-Platform stage in the formation of mature continental crust. The Archean acron is characterized by single occurrences of diamond-bearing magmatism in the world [1-3]. Deposits of industrial importance have not been established among them. In theory, similar bodies could also be found within the Baltic Shield. Given the relatively good study and exposure of the Baltic Shield, we should recognize that prospecting for industrial diamond-bearing objects of the Archean age as unpromising.
The Early Proterozoic (Karelian) megacycle (2500-1650 Ma) corresponds to the relevant eon and is characterized by the proto-Platform evolution of the area. The Karelian epoch is separated from the previous one by a gap of about 100 Ma. Since the early Proterozoic, the territory has deve-loped as a cratonized area, while some independence in the development of its various parts has been preserved. The Kalevian stage is associated with the oldest epoch of kimberlite magmatism in the region, represented by the Kimozero diamond-bearing body, located within the Karelian craton and confined to the Onegozersky intracratonic trough. Currently, there are three datings of the Kimozero kimberlites, with considerable variation: 1986 Ma [4], 1920 Ma [5], and 1764 Ma [6]. The latest dating most likely reflects the age of the metamorphism. The most reliable dates are 1986 Ma and 1920 Ma. To date, no Early Proterozoic (Paleoproterozoic) commercial diamond-bearing magmatic bodies have been established in the world. At the same time, Paleoproterozoic metaconglomerates of the Vila Nova Group are being developed in Brazil, and in Ghana, coeval metasediments of the Birim Supergroup [8]. In the north of the EEP, Early Proterozoic diamond primary sources with economic grades can be discovered.
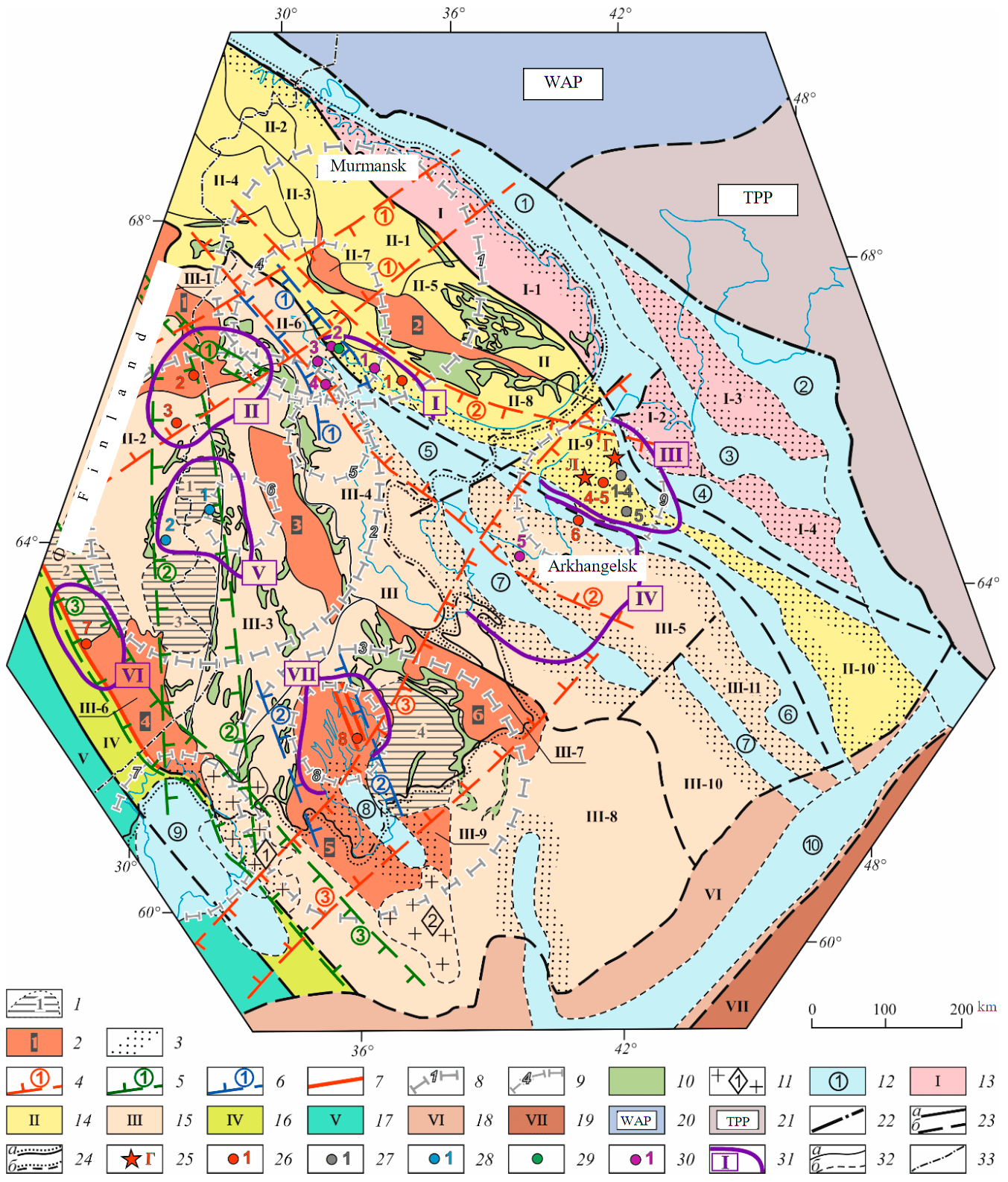
Fig.1. Structural and tectonic factors of diamondiferous magmatism distribution in the northern part of the East European Platform [7] 1-9 – favorable structural and tectonic factors of diamondiferous magmatism distribution (established and assumed). Determined: 1 – areas of early Archean (Saami) stabilization: Voinitsky (1), Isalmi (2), Tulossky (3), Vodlozersky (4); 2 – the main Early Proterozoic proto-rift structures: Kuolayarvinsky (1), Imandra-Varzugsky (2), Shombozero-Lekhtinsky (3), Outokumpu-Ladoga (4), Onegozersky (5), Vetrenoy belt (6); 3 – zones of edge dislocations of Riphean aulacogens; 4-6 – main zones of tectonic and magmatic activity, including ore-bearing and ore-controlling faults: 4 – Middle Paleozoic (Khibino-Botnia rift-1, Kovdorsko-Zimneberezhnaya-2, Ilmensko-Mezenskaya-3); 5 – Riphean (Sally-Tuttiyarvinskaya-1, Ladoga-Kuusamskaya-2) and Riphean-Vendian (Kuopiya-Sortavalskaya-3); 6 – Late Karelian (Kuzemsko-Kovdorskaya-1, Zaonezhskaya-2); 7 – large deep kimberlite-controlling faults; 8, 9 – ring structures (RS): 8 – with a diameter of up to 400 m (Kola-1, Kalevalskaya-2, Onegozerskaya–3), 9 – 100-200 km in diameter (Kandalaksha-4, Engozerskaya-5, Nyukozerskaya-6, Ladozhskaya-7, Zaonezhskaya-8, Zimneberezhnaya-9). Assumed: 10 – Late Archean greenstone belts; 11, 12 – unfavorable factors: 11 – massifs of rapakivi granites (areas of the Archean crust reworked in the Early Proterozoic): Ulyalegsko-Salminsky (1), Belozersky (2), 12 – aulacogenes, depressions filled with Riphean and Riphean-Lower Vendian deposits: Kildinsko-Svyatonosskaya step (1), Predtimansky (Mezen-Vychegodsky) marginal (pericratonic) trough (2), Safonovo-Ponoysky (3), Leshukonsky (4), Belomorsky (5), Keretsko-Pinezhsky (6), Onega-Dvinsky (7), and Onegozersky (8) aulacogenes, Pashsko-Ladoga (Ladoga) trough (9), Central Russian aulacogene (10); 13-19 – main structures of the basement in the northern part of the EEP: 13 – Murmansk geoblock (archon), I; 14 – Kola-Kuloi geoblock (archon), II; 15 – Karelian geoblock (archon), III; 16 – Savo-Ladoga-Rybinsk mobile belt, IV; 17 – Svecofennian geoblock, V; 18 – Central Russian folded belt, VI; 19 – Volga-Ural megablock (Sysolsky dome), VII; 20, 21 – platforms bordering the EEP: 20 –Western Arctic, 21 – Timan-Pechora; 22 – the boundary of the East European Platform; 23 – faults: a – established, b – assumed; 24 – the boundary of the sedimentary formations of the Russian plate: a – on the surface, b – under the waters; 25-30 – occurrences of kimberlites and related rocks: 25 – diamond mines (G – named after V.P.Grib, L – named after M.V.Lomonosov); 26 – kimberlite fields (with confirmed diamond content): 1 – Ermakovskoye (PZ2), 2 – Kuusamo North (RF3), 3 – Kuusamo South (RF3), 4, 5 – Chernoozerskoye, Zolotitskoye (PZ2), 6 – Chidvinsk-Izhmozerskoye (PZ2), 7 – Kaavi-Kuopio (RF3-V1), 8 – Kimozerskoye (KR21); 27 – kimberlite fields (with unidentified or poor diamond content): 1-4 – Melskoye, Megorskoye, Verkhotinskoye, Kepinskoye (PZ2), 5 – Suksomskoye (PZ2); 28 – lamproite-kimberlite fields (with confirmed diamond content): 1 – Kostomuksha (RF2), 2 – Kuhmo-Lentira (RF2); 29 – Poryegubskoe lamproite field (KR23) (with unknown diamond content); 30 – fields of rocks related to kimberlites (with unidentified diamond content): 1 – Umba olivine melilitites and ultrabasic foidites (PZ2), 2 North Kandalaksha alkaline picrites, melaanalcimites, picrodolerites (PZ2), 3 – Belie Shcheli – a field of picrites, ultrabasic foidites, and lamprophyres (PZ2), 4 – Gridino – picrite field (PZ2), 5 – Nenokskoe melilitites (PZ2); 31 – diamond regions: I – Terskyi Bereg, II – North Karelian, III – Zimnyi Bereg, IV – Arkhangelsk, V – West Karelian, VI – Central Finland, VII – North Onega; 32 – geological boundaries: a – reliable, b – assumed; 33 – the state border of the Russian Federation. The main basement blocks of the north of the EEP: I-1 – North-Kola, I-2 – Mezensky, I-3 – Nessko-Tylugsky horst, I-4 – Mezensky horst; II-1 – Central Kola, II-2 – Pechenga, II-3 – Upper Tuloma (Inari), II-4 – Laplandsky, II-5 – Keivsky, II-6 – Kolvitsky, II-7 – Imandra-Varzugsky, II-8 – Kola White Sea, II-9 – Zimneberezhny, II-10 – Poltinsko-Yelkibsko-Vashkinskaya uplift zone; III-1 – Kuolajarvinsky, III-2 – Fenno-Karelian, III-3 – Central Karelian, III-4 – Karelian White Sea, III-5 – Dvinsky, III-6 – Outokumpu-Ladoga, III-7 – Vetrenogo Poyasa, III-8 – South Karelian, III-9 – Onegozersky, III-10 – Onego-Kodimskoe uplift, III-11 – Arkhangelsky horst
The Late Proterozoic megacycle (1650 – about 600 Ma) covers the time interval from the Early Riphean to the Early Vendian (inclusive). This is the aulacogen phase of the platform stage of the geological evolution of the north of the Platform. The Riphean and Karelian epochs are separated by a period of tectonic and endogenous quiescence lasting about 100 million years. Within the limits of the Late Proterozoic megacycle in the north of the EEP, three epochs of diamondiferous magmatism have been reliably established: Middle Riphean, Late Riphean, and Late Riphean-Early Vendian. The Middle Riphean (Mesoproterozoic) epoch is characterized by rather high productivity in various provinces of the world. Its characteristic feature is the presence of deposits of both kimberlite and lamproite types. An example of deposits of the first type is: Lerala (Botswana) – 1333 Ma, Premier (South Africa) – 1180 Ma, and Salvador (Brazil) – 1150 Ma; the second one are: Argyle (Australia) – 1175 Ma and Majhgawan (India) – 1075 Ma [1].
More than 110 dikes of Middle Riphean (1200-1230 Ma) [9, 10] low diamondiferous kimberlites and lamproites have been found in the border area of the Russian Federation and Finland on an area of more than 10000 km2. Lamproite dykes, presumably of the Middle Riphean age, have been identified within the pericratonic trough of the Vetreny Belt (Lake Levushka region). The above data allow us to predict the discovery of commercial diamond-bearing objects in the territory of northern EEP, associated with the Middle Archean epoch of diamondiferous magmatism.
The Late Riphean and Late Riphean-Early Vendian (Neoproterozoic) epochs manifested quite widely worldwide. Kimberlite bodies of this period are known in Australia, Africa (Liberia), North America (USA, Canada), South America (Brazil, Venezuela), Greenland, and India. However, their productivity is significantly inferior to the previous Mesoproterozoic era. In the north of the EEP, in the Late Riphean, diamond-bearing kimberlite bodies of the Kuusamo South field (near the city of Kuusamo, Finland) were formed, the age of which is 758 Ma [11]. It is assumed that the formation of pipes and dikes of olivine lamproites of the Kuusamo North field (Finland) is associated with the same stage. The fields are about 40 km apart. Some bodies contain micro and macro diamonds. The intrusion of kimberlites of the Kaavi-Kuopio field, located in Southeastern Finland in the marginal part of the Karelian craton, is associated with the Late Riphean-Early Vendian epoch. Their age is 589-626 Ma [12]. About 30 bodies (pipes and dikes) were found within the field, most of which are diamond-bearing, but with diamond content not reaching the commercial level. Within the Russian part of the Baltic Shield, kimberlites and lamproites of the Late Riphean and Late Riphean-Early Vendian ages have not yet been identified, but the prospects of discovery of economic diamond-bearing objects of these epochs remain.
The Phanerozoic megacycle (<570-555 Ma) is a plate phase of the platform stage of the evolution of the northern part of the EEP. The Hercynian stage, covering the period from the Middle Devonian to the Late Permian, is of great importance in the history of the development of the region and diamond content. Tectonic processes of various types were manifested at this time in major part of the territory of the Karelian-Kola subprovince. The Middle Paleozoic (Late Devonian-Early Carboniferous) epoch has the highest diamond potential for both the East European and Siberian platforms. All known primary deposits in Russia are associated with it. During this period, within the Zimnyi Bereg and Arkhangelsk diamond regions, large-scale magmatic activity continues along with the formation of volcanic pipes of alkaline ultrabasic (kimberlites, melilitites) and basic (tholeiitic basalts) compositions; there are intrusions of melilitite volcanic pipes within the Onega Peninsula. At the same time, the formation of the Kandalaksha hypabyssal complex was completed. It is represented by kimberlite volcanic pipes and dikes of alkaline picrites, picrodolerites, picrites, lamprophyres, and carbonatites.
A characteristic feature of the Hercynian tectonic genesis in the region is the widespread deve-lopment of TMA zones represented by systems of close deep-seated faults that control occurrences of mantle magmatism (including alkaline-ultrabasic). Concerning regional basement structures, they often occupy a secular position, creating an autonomous structural plan. During this period, in addition to the Khibino-Botnichesky rift, the Kovdor-Zimneberezhnaya TMA zone was formed (Fig.1), which represents the shoulders of the Riphean White Sea aulacogen activated in the Middle Paleozoic, including its northern Keretsky-Pinezhskaya branch. It traces in a southeasterly direction for a distance of about 800 km from the area of Kovdor to the southeast to the Belomorsko-Kuloisky Plateau. Its width ranges from 80-90 to 200-250 km. Dikes of alkaline mafic and ultrabasic rocks of the Kandalaksha Bay, volcanic pipes of the Tersky Coast, including diamond-bearing kimberlites of the Ermakovskoe field, numerous bodies of kimberlites and related rocks of the Zimnyi Bereg region, including the mines named after. M.V.Lomonosov and V.P.Grib, are confined to this TMA zone.
Research methodology
The primary diamond deposits currently being mined in the world are represented by kimberlites or lamproites. The vast majority of them belong to kimberlites. It should be noted that in recent decades, along with these primary sources, other rocks containing diamonds have also become known. These include komatiites from Guyana [13] and Ghana [14-16], as well as lamprophyres from Canada [17, 18]. The last two types of rocks are not considered in the article, although it can be assumed that their diamond-bearing varieties can be discovered within the Karelian-Kola subprovince.
Diamond deposits in kimberlites and lamproites in areas with different types of prospecting environments differ in a set of features, which are the result of differences in the structure of the lithospheric mantle, which determines the features of the composition of the primary source, and the history of their geological and geomorphological evolution. The main criteria that distinguish diamondiferous bodies can be combined into several groups: geological; structural; mineralogical and petrographic; response of diamond primary sources in dispersion haloes of kimberlite minerals in overlying sedimentary rocks; geophysical.
Among the geological criteria, the morphology of the bodies and their sizes, the time of formation, the depth of the erosion, the thickness of the overlying sediments and their age, and the predictive and prospecting type of the pipe response in terrigenous diamond-bearing rocks [19], and the type of prospecting environment are taken into account. Until very recent years, diamond mines were associated only with pipe-shaped bodies. However, the complex of kimberlite dikes was discovered by N.P.Pokhilenko in the late 1990s near Snap Lake (Slave Craton, Canada), puts the dike type of deposits in the category of highly significant in terms of the economy [20]. Diamond-bearing dikes, veins, and stockworks are also known in South Africa, Sierra Leone, and Guinea.
The type of prospecting environment is determined by the structural position of the territory within the shield, plate, or their junction zone and a set of other geological features [21], which require the use of an appropriate set of prospecting methods. Three main types of environments are distinguished in the northern part of the EEP: Karelian, Onega, and Arkhangelsky.
Structural control of deposits involves the analysis of signs of local control, which include: ore-bearing faults coinciding with the long axes of pipes, zones of folds and faults of the kimberlite host basement (KHB), subsidence troughs of the near-pipe space, and complications of the structure of the bottom of the formations in the upper part of the KHB.
Types of rocks, associations of kimberlites indicator minerals, and parameters of diamond content should be distinguished among the mineralogical and petrographic criteria of diamond deposits in kimberlites and lamproites. Kimberlites and lamproites [22] are hypabyssal rocks that have no analogs among plutonic and volcanic rocks; they are combined within the hypabyssal class into one group divided into three families: lamprophyres, lamproites, and kimberlites (with a subfamily of related alkali feldspar-free picrites).
Among the kimberlites of the Siberian and East European provinces, three types of rocks are distinguished by composition: magnesian, ferromagnesian, and alkaline titan-magnesian (based on materials by A.D.Kharkiv et al.). Differences between the first two types of rocks in terms of petrochemical features are not significant. They differ more contrastingly in the content and composition of deep minerals: pyrope, picroilmenite, chrome spinel, and diamond. The magnesian group of kimberlites differs from the ferromagnesian one by slightly higher MgO and lower total iron content. Diamond deposits in Russia are found only in the first two types of rocks. On the territory of the Zimnyi Bereg region of the EEP, all pipes of the Lomonosov deposit belong to the magnesian type and only the Grib pipe belongs to the ferromagnesian one. Kimberlites are also subdivided into Groups I and II according to the composition of typical minerals [23]. Group II kimberlites (orangites) have mineralogical similarities with lamproites, although there are also differences that allow us to distinguish these rocks. Some rocks are similar in petrochemical, mineralogical, and geochemical characteristics to kimberlites of the II group and olivine lamproites. They are conditionally classified as rocks of intermediate composition K2L [24]. In general, lamproites are characterized by the same indicator minerals as kimberlites, but chromium spinel and chromium diopside are essentially predominant, while pyrope and picroilmenite are present in extremely small amounts. Manganese ilmenite is often noted as a characteristic mineral in lamproites. Rocks related to kimberlites and lamproites, accompanying them in some fields, and also forming separate fields, are alkaline picrites, minettes, and ultramafic lamprophyres.
The diamond grade in the primary sources is determined using small- or large-volume sampling data. The following grades of diamond content (ct/t) were used: poor (less than 0.05), low (0.05-0.25), medium (0.25-1.0), and high (more than 1.0). At the initial stage, a preliminary evaluation of the productivity of kimberlites, lamproites, and related rocks is carried out based on the interpretation of the chemical compositions of minerals based on the results of X-ray microprobe analysis, which is performed using various diagnostic diagrams that allow determining the belonging of indicator mine-rals to a certain paragenesis: pyropes [25, 26], picroilmenites [27-29], chrome spinels [25, 30, 31], and clinopyroxenes [27, 32, 33]. An important criterion for the diamond potential of kimberlites and related rocks is the P-T conditions of formation of their mantle melts, which can be reconstructed with the use of the evaluation of physical and chemical parameters (temperature, pressure, and oxidation-reduction degree) of crystallization of individual high-pressure minerals [34-36], peridotite [37, 38] and eclogite [39, 40] associations.
Primary sources of diamonds often reflect in sedimentary strata in dispersion haloes of indicator minerals of kimberlites, lamproites, and related rocks (KIMs). Pyrope, picroilmenite, chromium diopside, chromium spinel, and diamond are of the most significant practical interest in predictive and prospecting work. The dispersion haloes are characterized by higher KIMs contents relative to the background ones. The entire set of kimberlite minerals in terrigenous diamond-bearing rocks forms blankets or dispersion trains. According to the relationship with the sources, haloes of short (up to 5-10 km), moderate (up to 30-40 km), and long (tens and hundreds of kilometers) travel distance are distinguished. The closer to the primary source, the more slightly abraded KIMs are contained in the dispersion haloes. The degrees of abrasion (I-V) by A.D.Kharkiv [41] are used. The KIMs of the I degree (very good preservation) are unabraded grains. Very extensively abraded minerals belong to the V grade.
Geophysical features of diamond deposits are identified by magnetic, electromagnetic, and gravity survey and other methods. The indicative characteristics of prospecting objects are determined not so much by their petrophysical parameters as by their contrast concerning the host rocks. The physical properties of kimberlites and lamproites vary over a wide range but are still within a certain range of values, which allows several geophysical methods to be used in diamond prospecting in almost all regions of Russia and of the World. First of all, these are the increased magnetic properties of rocks. Another parameter is the reduced electrical resistance characteristic of most bodies. The average density values of kimberlites and lamproites are also, as a rule, reduced.
A set of the above techniques was used to characterize diamond deposit models, which were developed based on objects studied by the authors within different types of prospecting environments of the northern EEP. Speaking of deposit models, we are referring first of all to pipe-shaped bodies, which are their main morphological type. At the same time, it is quite probable that diamond-bearing dikes, veins, and stockworks will be found.
Results and discussion
In the northern part of the East European diamond province, primary diamond deposits can be identified within the territories of the Karelian (Baltic Shield), Arkhangelsk, and Onega (Russian Plate) types of prospecting environments. They are predicted within structures characterized by favorable structural, tectonic, mineralogical, facies dynamic factors, and features of the deep structure [7, 42, 43].
On the territory with the Karelian type of prospecting environment, diamond-bearing magmatic rocks of a wide age range, from Archean to Phanerozoic, can be found. Among the primary sources of diamonds, which may be potential deposits, in the territory of the Baltic Shield two main types (varieties) of bodies have been established – Kimozersky and Finnish. They differ in age, composition, level of erosion, and other features. Within the Russian Plate, the Arkhangelsk-type buried pipes stand out in covered areas.
We compare the Kimozero-type pipes (the first variety) with the Kimozero body (see Table). Among these pipes, deposits with low- or medium diamond grade can be identified. Bodies of this type can be represented by normal diatremes or narrow feeder channels and wide maar-shaped caps. Diamond-bearing kimberlites are distinguished by a peculiar composition of KIMs, among which chrome spinel and manganese ilmenite dominate, pyrope, and chrome diopside are present in small amounts. The body of Kimozero studied by the authors [7] is represented by a saucer-shaped deposit of very significant size (2.0 × 0.8 km) and narrow feeder channels, which brings the Kimozero complex closer to bodies of similar structure [44, 45], which are conditionally identified as the Saskatche-wan morphotype of kimberlites [46].
By analogy with them and based on some general petrographic features (the presence of pyroclastics and amoebic autoliths, rare fragments of the host strata, local phenomena of redeposition of deposited material), the Kimozero kimberlites are considered as products of subaerial volcanic emissions, which were ejected from the vents of a paleovolcano in several eruptive phases. After consolidation, the kimberlites underwent burial and alteration, including metamorphism. By analogy with other kimberlite bodies and taking into account that the studied complex is made up of formations that are commonly considered crater deposits, one can assume an extremely insignificant erosion of the body, measured only in tens of meters [19]. The particularity of the Karelian-Kola diamond subprovince lies partly in the fact that here, within the limits of proto-riftogenic troughs, very old-age low eroded kimberlite bodies are likely to be found. KIMs dispersion haloes are contained in the Quaternary basal moraine deposits and can be traced for a distance of a few kilometers from the source [8]. The transport of kimberlite minerals is possible under fluvial conditions and over moderate distances (a few tens of kilometers). In ancient potential diamond-bearing rocks, the highest probability of KIMs detecting is associated with paleo-delta deposits.
Prospecting models for primary diamond deposits in the north of the East European platform
Criteria |
Prospecting models |
|||
Kimozero |
Finnish |
Arkhangelsk |
||
Geological |
Morphology of bodies and sizes* |
Pipes (small, medium, large, unique) |
Pipes (small, medium), veins, dikes, stockworks |
Pipes (small, medium, large, unique), sills |
Age |
PR1 |
PR-PZ |
PZ2 |
|
Depth of erosion** |
Low and moderate |
Moderate (?) |
Low (crater facies preserved) |
|
Overlying sediments thickness (age) |
More often 0-20 m (Q), less often 20-60 m (Q), can reach |
More often 0-20 m (Q), less often 20-60 m (Q), can reach 200 m (Q) |
Up to 200 m (C, Q) |
|
Composition of rocks in the upper part of the KHB (age) |
Rocks of the crystalline basement (AR-PR1) |
Rocks of the crystalline basement (AR-PR1) |
Sandstones, siltstones, mudstones (PR2), terrigenous and carbonate rocks (PZ1) |
|
Predictive prospecting pipe type |
Slightly eroded bodies with |
Moderately eroded bodies with an areal KIM train; highly eroded bodied with a regional KIMs train |
Slightly eroded bodies with |
|
Type of prospecting |
Karelian |
Karelian, Onega |
Arkhangelsk, Onega |
|
Structural |
Local structures |
Zones of folded and discontinuous dislocations; deep faults |
Negative structures expressed in relief (often under lakes); deep-seated faults |
Cauldron depressions of the near-pipe space; complications of the structure of the base of the formations in the upper part of the KHB; ore-controlling faults (coinciding with the long axes of the pipes) |
Mineralogical and petrographic |
Rock types |
Kimberlites, lamproites |
Kimberlites, lamproites |
Kimberlites (magnesian |
Mineral associations of KIMs |
Pyrope-chrome spinel |
Pyrope-chrome spinel, |
Magnesian type: chromium diopside-pyrope-chrome spinel (up to 10-15 g/t – crater facies, up to |
|
Diamond grades (established and expected) |
Low, Medium |
Low, Medium |
Low, Medium, High |
|
Response in KIMs dispersion haloes |
Genetic types of haloes (age) |
Moraine, fluvioglacial, alluvial, deluvial, eluvial (Q); in the Proterozoic depressions are assumed to be deltaic (PR1) |
Moraine, fluvioglacial, alluvial, deluvial, eluvial (Q); |
Moraine, fluvioglacial, alluvial, deluvial, eluvial (Q); alluvial, deluvial, shoreface (C1-2); lacustrine crater depressions (D3-C1) |
The total distance of KIMs travel from primary sources |
Short, moderate |
Short, moderate, and long |
Short, moderate |
|
The content of KIMs of I-II degrees of abrasion in haloes of short travel distance (grains per sample) |
Hundreds |
Tens, hundreds, rarely thousands |
From pipes of magnesian kimberlites: the first grains per sample, less often tens; from pipes of ferromagnesian kimberlites: |
|
The size of the minera-logical anomaly according to distribution of KIMs of I-II degrees of abrasion: |
1 – not established; |
1 – within the pipe area and the nearest area; |
1 – within the pipe area and the nearest area; |
|
Criteria |
Prospecting models |
|||
Kimozero |
Finnish |
Arkhangelsk |
||
Response in geophysical fields |
Magnetic survey |
Positive anomalies from tens to 3000 nT, depending on the type of kimberlite |
Positive anomalies from tens to hundreds of nT. Anomalies of the similar intensity of non-kimberlite nature are widespread |
Positive anomalies from units to tens of nT. In the ground survey, anomalies from kimberlites of the magnesian type are often broken up into small positive or negative extremes. The kimberlites of the ferromagnesian type are more homogeneous in structure and composition, and the anomalies in ground surveys, as a rule, are not disintegrated |
Gravity survey |
Not detected |
Negative anomalies of varying intensity |
Not manifested |
|
Electromagnetic survey |
Apparent resistivity anomalies from a few to 1000 ohm-m (depending on the type of kimberlite) against the background of host rocks with |
Apparent resistivity anomalies from units to tens of ohm-m against the background of host rocks with resistivity up to thousands of ohm-m |
Anomalies of apparent resistivity of 10-150 ohm-m against the background of host rocks with a resistivity of up to 500 ohm-m |
* Pipe sizes: small – less than 10 000 m2, medium – 10 000-100 000 m2, large – 100 000-250 000 m2, unique – more than
250 000 m2 (according to V.M.Podchasov [47]). ** Depth of erosion: low – up to 200-250 m, moderate – up to 1000 m, high – more than 1000 m.
Narrow diatreme channels of the Kimozero occurrence with a surface diameter of about 30-40 m (Fig.2) break through both host dolerites and sheet-like kimberlite body. The channels are exposed on the surface in the form of isometric outcrops [7]. The section of rocks that make up the sheet-like deposit has a complex three-layer structure [6]. The upper horizon (15-20 m) is formed by carbonaceous kimberlite, and the middle (up to 10 m) magnetite-serpentine-tremolite (“magnetite”) kimberlites of variable thickness, characterized by high magnetite content. The lower part of the section of the sheet-like deposit (10-20 m) is composed of normal phlogopite-carbonate-serpentine kimberlite without obvious distinguishing features. All major types of these rocks are reflected in magnetic and electric fields. Moreover, each of them corresponds to well-defined chara-cteristics of the named fields, its own “geophysical image” [48]. The “magnetite” horizon is determined by an anomalously high magnetic field. The maximum anomaly amplitude of more than 3000 nT is observed in the right part of the shown profile, where it has the highest thickness and horizontal dimensions. This horizon is not consistent in area, and, accordingly, not the entire body is characterized by such a contrasting anomaly, but only its fragments. Apparently, it is this largest outcrop of the “magnetite” horizon in the northeastern part of the body that is recorded by airborne magnetic surveys with an anomaly with an intensity of about 340 nT. In terms of electrical resistance, the “magnetite” horizon is characterized by relatively high absolute values of ρk (about 1000 ohm-m), but against the background of host dolerites with a resistance of tens of thousands or more ohm-m, such objects can be considered conductors.
The “carbonaceous” horizon (pt 50-200, Fig.2) is characterized by an anomalously low electrical resistance of less than 10 ohm-m against the background of high-resistivity dolerites with a resistance of tens of thousands ohm-m. In the magnetic field, the “carbonaceous” horizon is relatively weak. However, when examined in detail, it still differs from dolerites by higher values and smoothed frequency response of the magnetic field: the dolerite – kimberlite boundary is detected in the pt 50 site. These details of the magnetic field were recorded using a nearly continuous survey with a measurement cycle of 1 sec, which, on average, corresponds to a survey step of about 1 m.
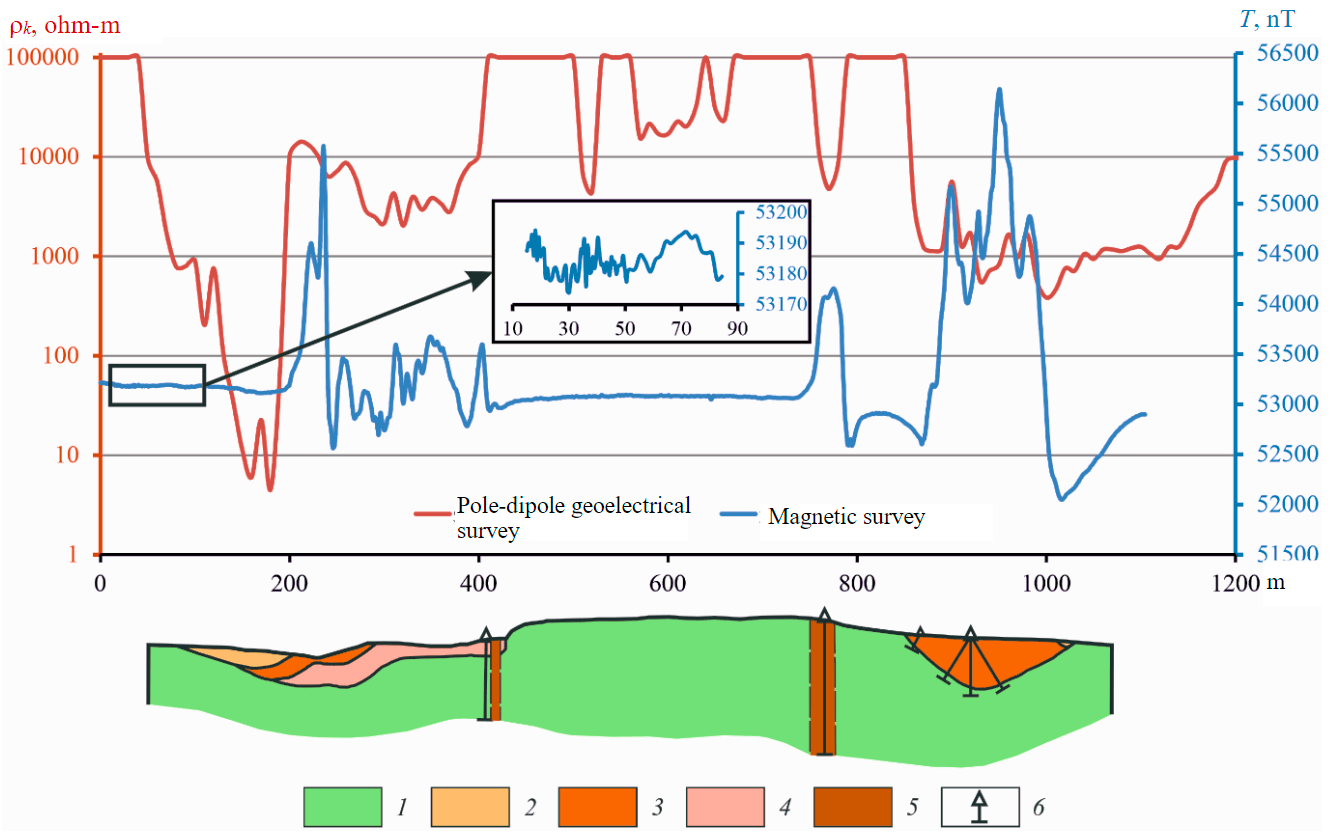
Fig.2. Prospecting model of the Kimozero type: geological and geophysical section through the Kimozero kimberlite body according to ground geophysical data 1 – gabbrodolerites; 2 – carbonaceous kimberlites; 3 – magnetite-serpentine-tremolite (“magnetite”) kimberlites; 4– phlogopite-carbonate-serpentine kimberlites; 5 – diatreme channels: phlogopite-serpentine porphyritic mica kimberlites and kimberlite breccias; 6 – boreholes
“Normal” kimberlite is characterized by a jagged magnetic field with sharp changes in values reaching up to ±500 nT. The electrical resistance, according to electrical prospecting data, is about 2000 ohm-m. Both characteristics make it possible to confidently distinguish this type of kimberlite against the background of host rocks. The feeder channels (pt 770 and pt 400, Fig.2) are distinguished by magnetic field anomalies with amplitudes of 1000 and 500 nT, respectively. One larger channel is also identified according to electrical resistivity survey data. The smaller one is located in the marginal region of low ρk values, but no local anomaly is observed, possibly due to its small size and/or the influence of the “normal” kimberlite that cuts through this channel. A detailed gravity survey within the Kimozero area was not carried out, but the morphology and small thickness of sheet-like bodies of kimberlites, as well as the small size of the feeder channels, do not allow the detection of local anomalies in the gravity field.
Pipes of the Finnish type (the second variety) can be found within the Baltic Shield and in the area of its junction with the Russian Plate. They are usually represented by small diatremes (up to a few hectares in size), such as bodies within the Kaavi-Kuopio, Kuusamo South, and Kuusamo North fields. They are composed of diamond-bearing kimberlites of group I of the KIM polymineral composition with a predominance of pyropes and picroilmenites, less often micaceous kimberlites of Group II of pyrope-chrome spinel composition or olivine lamproites. The pipes are cha-racterized by the absence of crater facies and a more significant erosion compared to the bodies of the Kimozero type.
Finnish-type bodies are characterized by KIMs dispersion haloes of different shapes and sizes in overlying Quaternary sediments. In formations of the basal moraine up to 15-20 m thickness, they are traced as unabraded or slightly abraded (I-II degrees) grains of kimberlite minerals at a distance of about 1.5-2.0 km from the primary source [49]. Shear moraines can bring KIMs to the surface. According to M.Tyni [50], some kimberlite pipes may not have a dispersion halo of short travel at all.
When KIMs are transported by glacial or river waters, dispersion trains up to 30 km long or more are formed. The contrast and extent of the halo depend primarily on the conditions of formation of the deposits overlying the object of interest and on the content of kimberlite minerals in the source rocks.
Known kimberlite and lamproite bodies of the Finnish type are usually characterized by positive anomalies in the magnetic field. The intensity of the anomalies varies in a wide range: from units to hundreds of nT. However, more intense anomalies than from kimberlites may be associated with rocks of greenstone belts and sills of gabbro. Thus, within the Kaavi-Kuopio kimberlite field, as well as in the Kuusamo South field, there are many anomalies comparable in amplitude or more intense than those from kimberlite pipes. In addition, the magnetic susceptibility of host rocks, represented mainly by granite gneisses or quartzites, can reach hundreds of 10–5 SI (data from the Geological Survey of Finland), which overlaps the range of changes in the magnetic susceptibility of diamondife-rous rocks. Despite these complicating factors, virtually all Finnish-type pipes have been found using magnetic surveys.
For example, in the Kuusamo South kimberlite field, when verifying magnetic anomalies, two kimberlite pipes (Kattaisenvaara and Kalettomanpuro) were discovered (Fig.3). The first, according to the airborne magnetic survey, is distinguished by an anomaly with a width of 180 m and an intensity of 600 nT (Fig.3, a). The Kalettomanpuro pipe consists of two bodies represented by kimberlites of Groups I and II (Fig.3, b). Rocks of the Group II are only slightly more magnetic than the host rocks and do not stand out as a separate magnetic body. According to Arctic Star (Canada), the olivine lamproites of the Kuusamo North field have magnetic, electromagnetic, and gravitational anomalous responses.
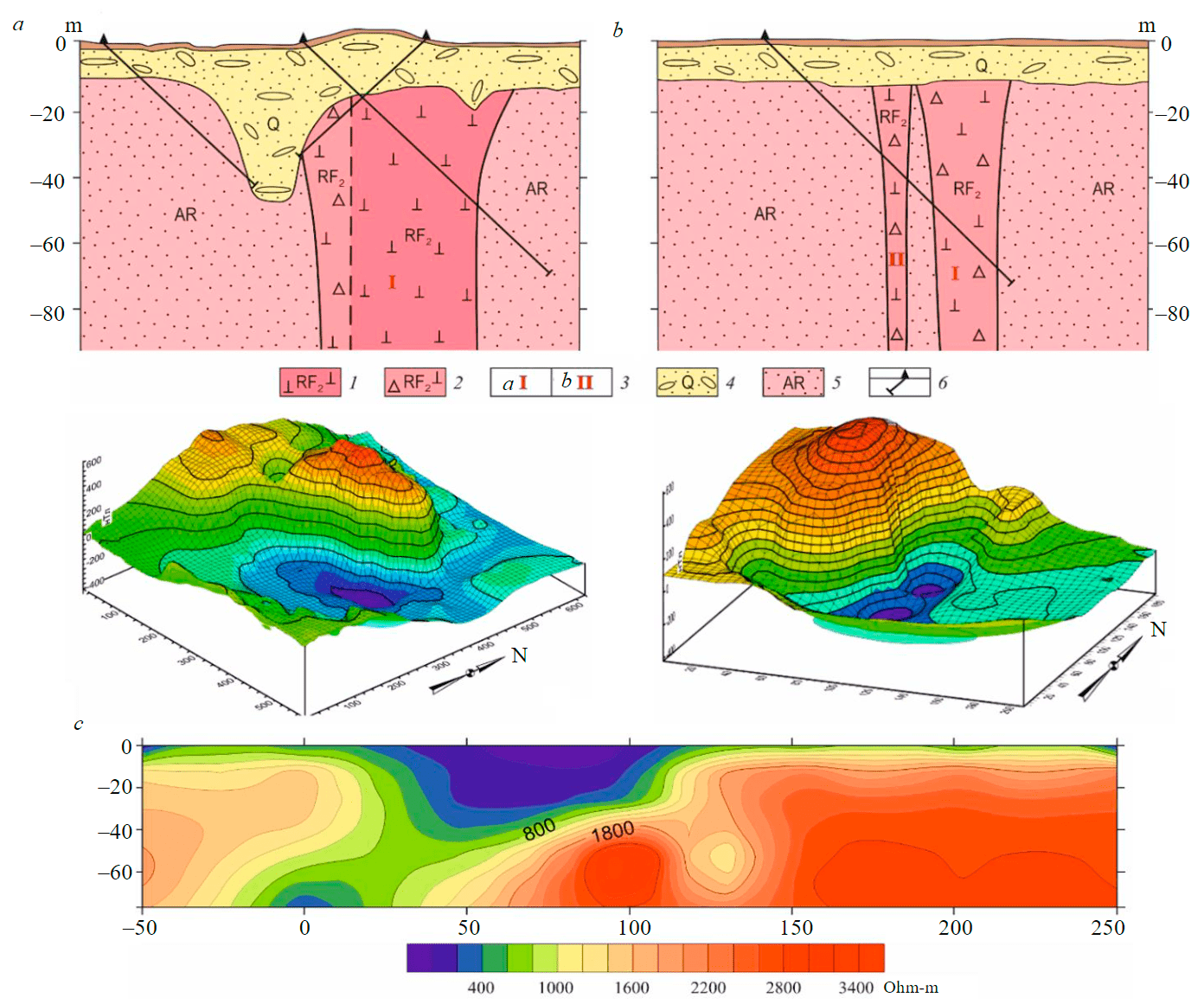
Fig.3. Finnish-type prospecting model: geological sections and magnetic field from ground survey data of the Kattaisenvaara (a) and Kalettomanpuro (b) pipes of the Kuusamo South field and geoelectric section of pipe N 21 of the Kaavi-Kuopio field (c) ([7, 11, 51], with supplements and data from Sunrise Diamonds [52]) 1, 2 – rocks composing the kimberlite pipe: 1 – hypabyssal kimberlite, 2 – kimberlite breccia; 3 – kimberlites: a – Group I, b – Group II; 4 – Quaternary glacial deposits; 5 – Archean gneisses; 6 – boreholes
In the Kuusamo North field, in 2005, European Diamonds Plc discovered olivine lamproites forming Black Wolf and White Wolf pipes within the area of magnetic basement rocks. It is intere-sting to note that the later discovered Grey Wolf pipe, based on the data of Arctic Star, is not detected in the magnetic field, but, compared to the other pipes, it is much more contrasting in the gravity field.
Studies by specialists from the Geological Survey of Finland have shown that the electrical resistivity of Archean rocks ranges from a few thousands to more than 30,000 ohm-m, with values in the first tens of thousands being the most common. At the same time, the resistivity of kimberlites, according to the [53], can vary from 5 to 700 ohm-m, where the maximum values are rare and typical for deep horizons. With all possible fluctuations in the electrical resistance of kimberlites, lamproites, and host rocks, there are multiple differences between Finnish-type pipes.
According to Yu.V.Korotkov (2011), who carried out work using the transient process method on several kimberlite pipes of the Kaavi-Kuopio field, they belong to the category of well-conductive relative to the host rocks (Fig.3, c). The results of these works are published in the report of the joint venture between Sunrise Diamonds and Nordic Diamonds [52]. Kimberlites are marked by a contrast anomaly, within which the electrical resistance is tens of times lower than that of the host rocks. At the same time, elements of the morphology of the kimberlite body are well detected both in plan view and in section.
Pipes of the Arkhangelsk type (the third variety) are predicted in the covered territories of the Russian Plate. In such areas, slightly eroded kimberlite bodies, accompanied by a local KIMs train in terrigenous diamond-bearing rocks can be discovered. In such environments, the formation of diamond placers considerably transported from primary sources is impossible, and the bodies themselves are distinguished very weakly both in the geophysical fields and in low-contrast concentrate haloes. The identification of kimberlite bodies and related rocks in such areas is very difficult and requires the use of a broad set of geological, geophysical, geochemical, and other methods. Typical examples of pipes of the Arkhangelsk type are the diamond mines named after. M.V.Lomonosov and V.P.Grib. Generalizing studies have established that economic diamond-bearing bodies are characterized by some features (see Table). Most often they are represented by buried pipes, consisting of a well-preserved diatreme and a crater part (Fig.4). The local structural control of the bodies is determined by the confinement to ore-bearing faults, coinciding with the long axes of the bodies, subsi-dence troughs, and the complexity of the structure of the suites base in the upper part of the KHB. The presence or absence of a well-defined crater in many pipes is explained not only by the peculiarities of their original structure but also by the depth of the erosion. It could be higher for bodies formed at the initial stage of the Middle Paleozoic tectonic and magmatic activity [54]. It is is well correlated with the presence of a blanket of kimberlite minerals, represented by grains of IV and V degrees of abrasion, most likely coming from primary sources at an early stage of intrusion.
The Arkhangelsk type of pipes is represented by ferromagnesian (mine named after V.P.Grib) and magnesian (mine named after M.V.Lomonosov) kimberlites. The KIMs contents in them differ by a factor of tens or hundreds. The diamond-bearing ore bodies of the ferromagnesian type are characterized by more contrasting continental dispersion haloes of short travel distance with higher contents of KIMs of I and II degrees of abrasion (Fig.4). Magnesian kimberlites are very weakly reflected in haloes, and even single unabraded and slightly abraded grains of high-pressure minerals may indicate the proximity of the source. Buried mineralogical anomalies from pipes of the Arkhangelsk type can be traced in the continental Carboniferous paleoenvironments at a distance of no more than 2 km from the source in the direction of transportation. The area of the mineralogical anomaly represented by the dispersion halo of KIMs of I and II degrees of abrasion varies from 1-2 to 16 km2. Primary sources can also appear in ancient shoreface haloes, but only if they are located near the pipe.
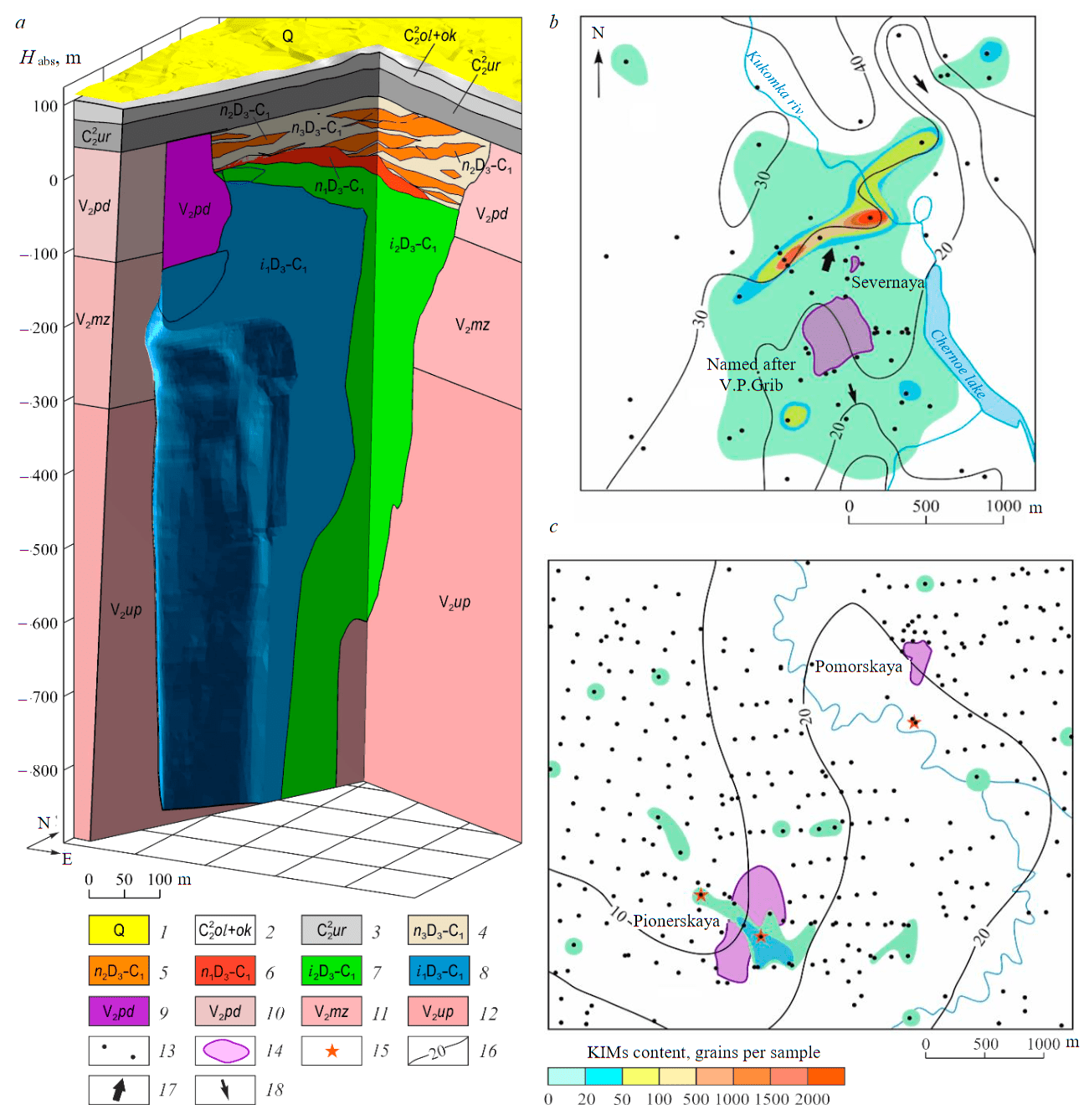
Fig.4. Section of a pipe of the Arkhangelsk type on the example of the Grib mine (a) and the structure of short travel distance haloes of KIMs (I-II degrees of abrasion) in continental Carboniferous deposits from kimberlite bodies of ferromagnesian (b) and magnesian varieties (c) ([7, 55], with supplements ) 1-3 – sediments covering the kimberlite pipe: 1 – Quaternary, 2 – Olmugsko-Okunevskaya suite, 3 – Urzugskaya and Voerechenskaya combined suites; 4-6 – rocks of the crater part: 4 – sandstones and tuff sandstones, 5 – tuff sandstones, tuffites and tuffs, 6 – tuffs, tuffites; 7, 8 – diatreme rocks (sequence of intrusion): 7 – autolithic kimberlitic breccia and porphyritic kimberlite (final), 8 – xeno-tuff breccia and tuff breccia (initial); 9 – zone of disintegrated sandstones; 10-12 – host rocks of the Late Proterozoic kimberlite–containing basement, Upper Vendian formations: 10 – Padunskaya, 11 – Mezenskaya, 12 – Ust-Pinezhskaya; 13 – places of concentrate sampling (from the borehole cores); 14 – kimberlite bodies; 15 – diamond occurences in heavy concentrate samples; 16 – isohypses of the reconstructed Middle Carboniferous relief, m; 17, 18 – directions of transportation of KIMs: 17 – in the Early Carboniferous and 18 – in the Middle Carboniferous time
Almost all known kimberlite bodies of the Arkhangelsk type were discovered during the verification of magnetic anomalies. According to airborne magnetic survey data, they are reflected, as a rule, by positive anomalies of the magnetic field of the so-called pipe type. So, kimberlite pipes of the Lomonosov deposit are manifested in the local component of the field (Fig.5). The amplitude of the values of the magnetic induction of kimberlite bodies in most cases lies in the range from units to tens of nT. With ground magnetic surveys, many anomalies acquire a complex shape, split into several extrema, making it difficult to determine the epicenter of the anomaly. If, for example, on the Lomonosov pipe, according to ground survey data, although it is displaced relative to the center of the pipe, it is still in its contour, then on the Arkhangelsk pipe, the maximum is confined to the marginal part of the body and goes beyond it (Fig.5). Significantly, negative values of the local magnetic field are observed over individual blocks of the body. There may be bodies completely composed of similar kimberlites with the corresponding reflection in a magnetic field. Therefore, during pipes (and deposits) of the Arkhangelsk type prospecting, a ground magnetic survey does not always solve the problem of detailing the airborne magnetic anomaly. Such examples are not unique, and in such cases, according to the results of ground works, the contours of the body and, accordingly, the points of boreholes can hardly be determined.
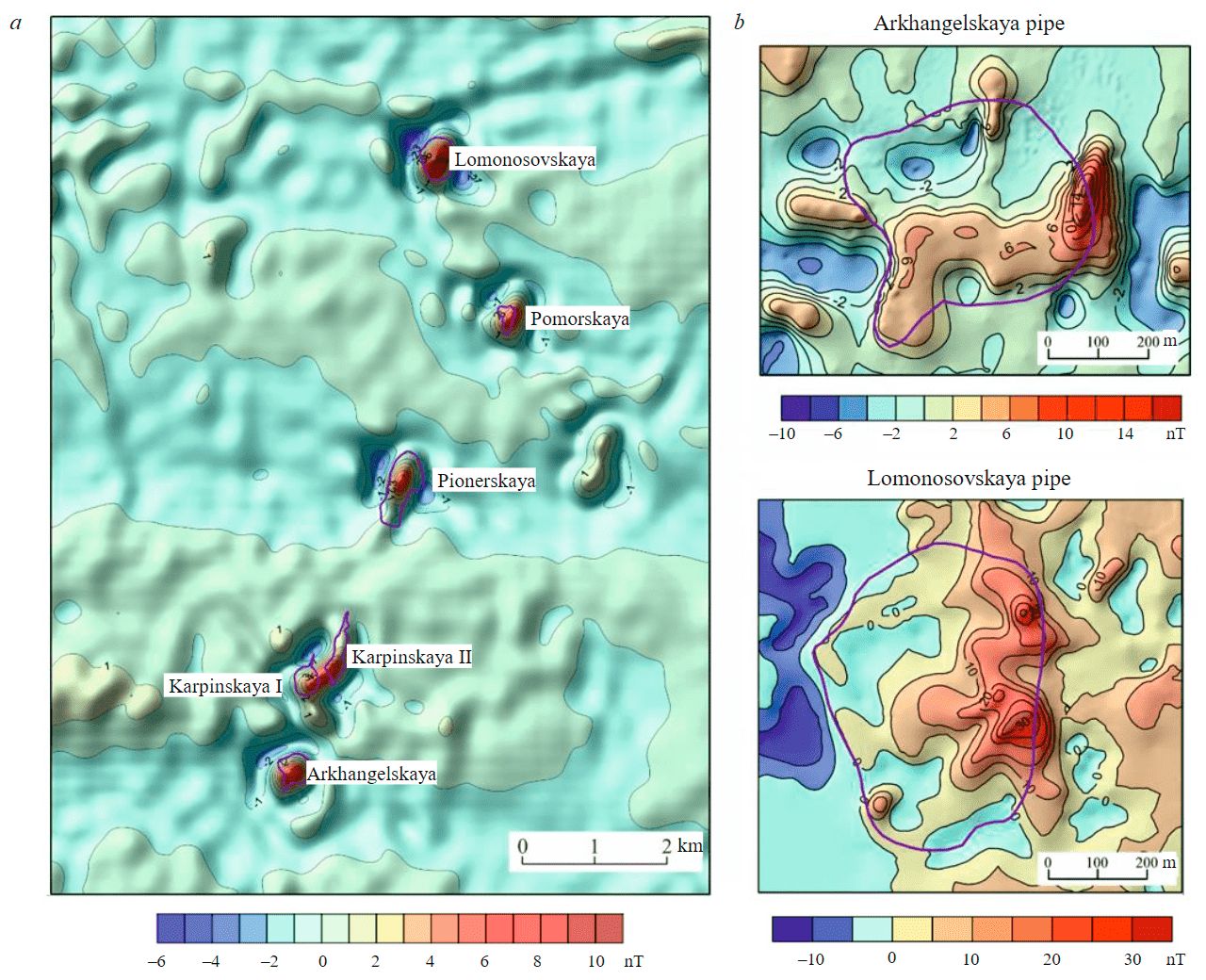
Fig.5. The local magnetic field in the area of the Lomonosov deposit according to aeromagnetic survey data (a) and magnetic field according to ground magnetic survey data on Arkhangelskaya and Lomonosovskaya kimberlite pipes (b)
Moreover, as a result of sorting out airborne anomalies only by ground magnetic survey, some of them may be generally recognized as unpromising and not worthy of further study, or verification boreholes will be set in the wrong place. A similar situation took place on the Grib pipe, where, according to ground magnetic survey data, in 1987, borehole N 906 was drilled 150 m from the pipe (Fig.6).
Subsequently, the Grib mine was discovered only in 1996 as a result of comprehensive revision works, and a 441/1 borehole, which intersected kimberlite, was set according to electromagnetic survey data [56]. At the same time, an airborne magnetic survey conducted in 1984-1985 recorded a low-intensity but quite recognizable positive anomaly above the pipe (Fig.6). The amplitude of the airborne anomaly values varies depending on the data processing technique but does not exceed 4 nT. In the magnetic field, according to ground magnetic survey data, two epicenters are distinguished. One of them with an amplitude of 15 nT coincides with the epicenter of the airborne magnetic anomaly and is located within the contour of the pipe. Another, with an amplitude of more than 30 nT, where the borehole No906 was drilled lies outside the body limits and does not appear according to airborne data. Let us assume that the second epicenter is caused by a near-surface object and is not connected with the pipe.
For a long time, magnetic survey remained virtually the only geophysical method for kimberlites prospecting in the Zimnyi Bereg diamond region. Statistics show [57] that in the early 1980s the efficiency of verifying magnetic anomalies on the Zimnyi Bereg territory was 20 %; in the 1980s – 12.5 %, in the 1990s – already less than 1 %, and in the first decade of the XXI century – less than 0.5 %. This is mainly because relatively contrasting magnetic field anomalies have already been identified and verified, and at present, the intensity of possible prospective anomalies is approaching the level of geological noise. In this regard, to increase the efficiency of searches, electrical prospecting is increasingly being introduced, in particular, the transient electromagnetic method (TEM). TEM works carried out on kimberlite pipes of the Zimnyi Bereg diamond region showed that kimberlites are characterized by contrasting conductivity anomalies. Examples are the geoelectric sections of the Pionerskaya (Fig.7, a) and Severnaya (Fig.7, b) pipes. Despite the small size of the Severnaya pipe (50×100 m), it is confidently distinguished by a conductivity anomaly according to the TEM data with a 50×50 m loop.
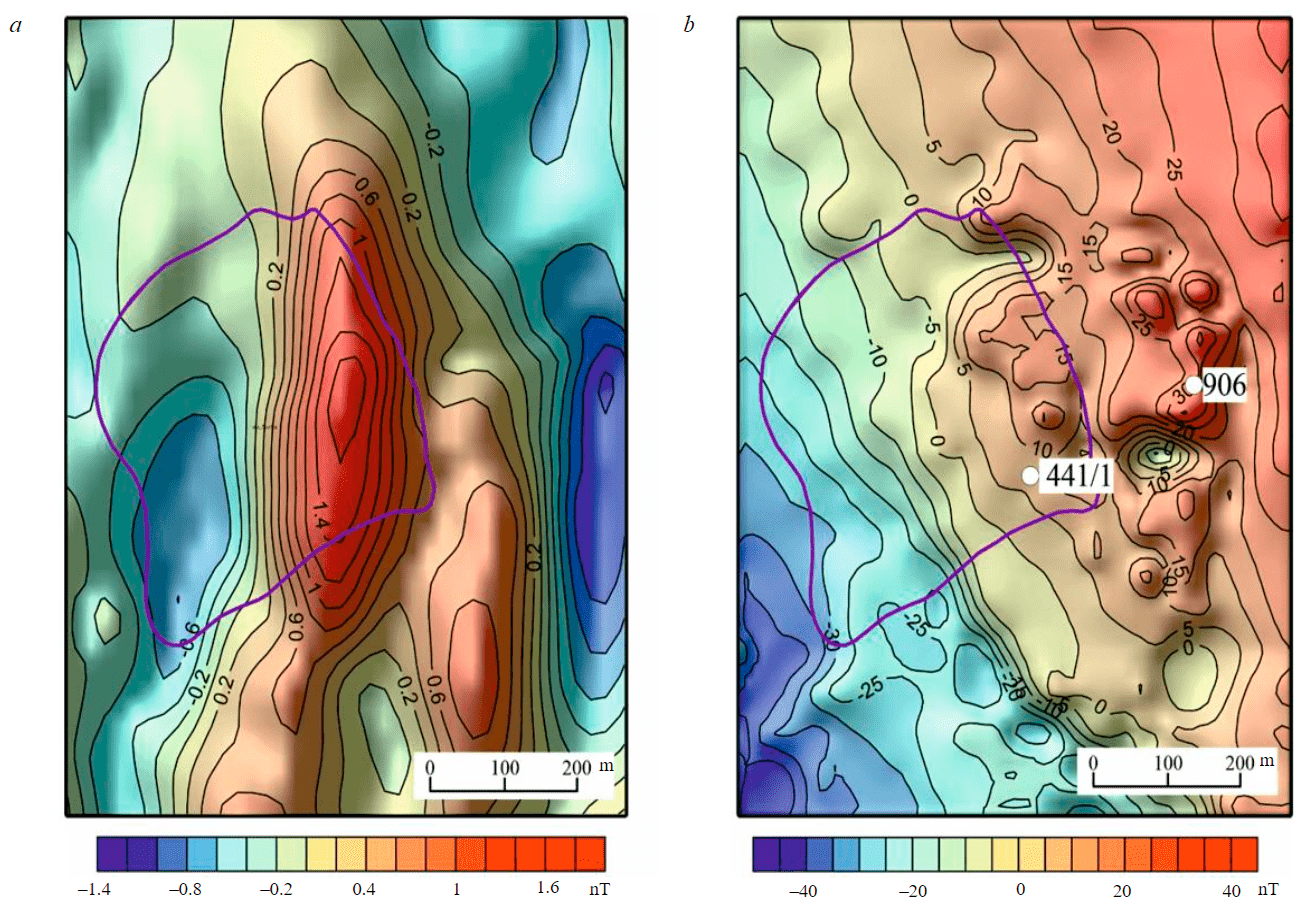
Fig.6. The magnetic field above the Grib kimberlite pipe according to airborne magnetic (a) and ground (b) surveys
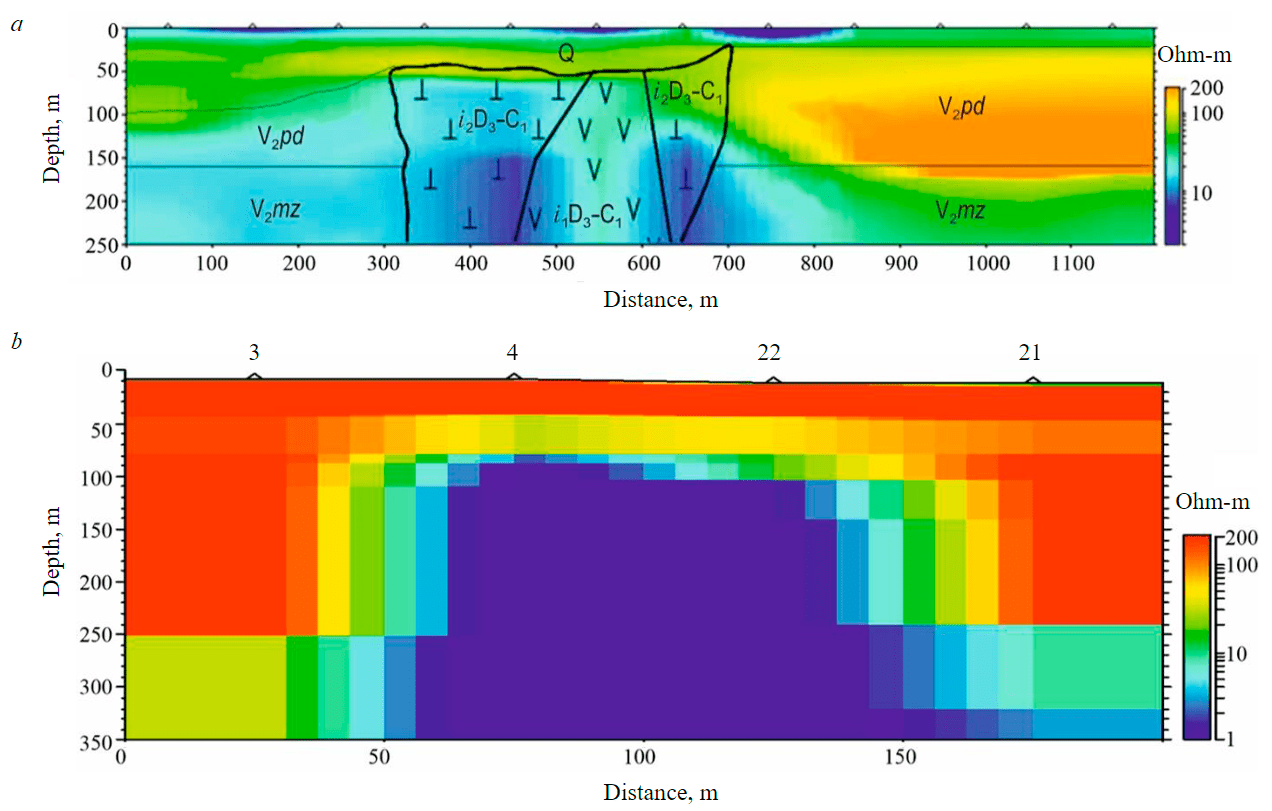
Fig.7. Geoelectric sections according to TEM data for the Lomonosovskaya (a), Severnaya (b) pipes ([7, 58], with supplements)
Features of the Arkhangelsk-type pipes in the geophysical fields are in good agreement with the distribution of petrophysical properties in kimberlites, host, and overlying formations. The results of the study of these rock properties are reflected in numerous companies reports and summarized in publications [59-61]. The analyzed results of these studies showed that Quaternary and Middle Carboniferous sediments overlying kimberlite pipes are characterized by a wide range of changes in physical properties. The average value of the magnetic susceptibility for them is 20-40·10–5 SI. However, there are areas with a general increased background of up to 100·10–5 SI, and in some interlayers, the values reach hundreds and even thousands of units SI 10–5. Kimberlite-hosting Vendian deposits are generally weakly magnetic: the magnetic susceptibility averages 25·10–5 SI.
Analysis and generalization of the available information on geophysical studies of boreholes and petrophysical features of kimberlites of magnesian and ferromagnesian types make it possible to reveal some of their differences. The rocks of the crater part of magnesian-type kimberlite pipes (for example, the Lomonosov deposit) are represented by tuffaceous sedimentary formations. Their magnetic susceptibility is, as a rule, higher than that of host rocks, and reaches 100 and more 10–5 SI units in some layers. The diatreme parts of the pipes are represented by tuff breccias and xeno-tuff breccias, characterized by increased magnetic susceptibility, averaging 80-100·10–5 units SI. Autolithic breccia is characterized by a lower value of χ = 40-60·10–5 SI. The magnetic susceptibi-lity of kimberlites of both phases tends to increase with depth. The maximum magnetic susceptibi-lity, reaching 2700·10–5 units SI is characterized by porphyritic kimberlites formed in supply channels. A good example of the reflection of the kimberlite properties in a magnetic field is the Lomonosovskaya pipe. Here, xeno-tuff breccias compose the southeastern, and autolithic breccias compose the northern and western parts of the pipe [62]. Therefore, according to ground magnetic survey data, the southeastern part of the pipe is characterized by higher magnetic signatures and the northwestern part by lower ones (see Fig.6). During the airborne magnetic survey, individual extrema that appear during ground surveys are integrated, and the influence of near-surface magnetic objects decreases, while deep-seated ones, on the contrary, increase. These factors, taking into account the increase in the magnetic susceptibility of kimberlites with depth, explain the more unambiguous reflection of pipes in the airborne magnetic survey data than in the ground one.
Pipes composed of ferromagnesian kimberlites differ in petrophysical parameters from magnesian ones. For example, according to the magnetization in the section of the Grib pipe crater, two rock strata are distinguished. The upper stratum up to 70 m thick is characterized by the predominance of rocks with a magnetization of 85-90·10–5SI, and only for separate layers of tuffs and tuffites its values reach 100-120–5 SI. The rocks of the lower strata are distinguished by increased magnetization up to 100-200·10–5 SI. The weathered autolithic kimberlite breccia in the contact zone with the rocks of the crater is characterized by a weak magnetization of 120-150·10–5 SI. Deeper in most boreholes, a block of kimberlites with magnetization values up to 1200·10–5 SI is distinguished. Below this block, its intensity decreases to 120-300·10–5 SI, and from a depth of 410-440 m (for individual boreholes) increases and reaches values of 2000-2500·10–5 SI, especially in the central part of the vent. The magnetic susceptibility of tuff and xeno-tuff breccias varies from 100 to 500·10–5 SI, the maximum values are characteristic of xeno-tuff breccias. The magnetization of tuff breccias is 100-250·10–5 SI.
According to the data of surface and airborne magnetic surveys, a positive magnetic anomaly stands out in the eastern part of the Grib pipe, composed of autolithic kimberlite breccia (see Fig.6). The western part of the pipe, represented by xeno-tuff breccias, does not appear in the magnetic field. This is probably due to both the lower magnetic susceptibility and the significant admixture of nonmagnetic material in the host rocks of the Vendian age. The content of xenoliths here reaches 40-50 %, the composition is dominated by mudstones and siltstones. Thus, despite the presence of rocks with high magnetic susceptibility in the Grib pipe, the pipe is weakly recognized in a magne-tic field. It can be explained by the small thickness and deep occurrence of such rocks.
Most of the ferromagnesian kimberlite pipes of the Kepinskoye field (Stepnaya, Solokha, 840, etc.) are composed of xeno- and tuff breccias. In a magnetic field, they are confidently distinguished by positive anomalies with intensity from a few to 10-15 nT, according to airborne magnetic survey data, and up to 30 nT, according to surface one. According to the results of the ground magnetic survey, unlike the pipes of the Lomonosov deposit, anomalies do not split, which is explained by their simpler structure and the homogeneity of the kimberlites that compose them.
The electrical characteristics of kimberlites of magnesian and ferromagnesian types are similar. Similar parameters also distinguish the host and overlying rocks. The electrical resistivity of kimberlites is 5-30 ohm-m, less often in individual blocks, usually deep, up to 100 ohm-m. Similar indicators of the host rocks of the Padunskaya suite vary from 70-200 ohm-m, in the upper part of the section to 5-30 ohm-m, in its lower part. A sharp increase in electrical conductivity is noted at depths from 100 to 250 m and is due to the upper boundary of highly mineralized groundwater. Thus, the greatest contrast between kimberlites and host rocks takes place in the upper part of the host rock section.
The average density of host rocks and kimberlites is almost the same (2.2-2.4 g/cm3). Lower values (2.0-2.2 g/cm3) distinguish overlying deposits. Therefore, it is not advisable to use a gravity survey to prospect for deposits of the Arkhangelsk type in these conditions.
Conclusions. An analysis of the geological, structural, mineralogical, and petrographic criteria for a local prediction, as well as a study of the response of kimberlite and lamproite bodies in dispersion haloes and geophysical fields, made it possible to identify and characterize the main models of primary diamond deposits in various prospecting environments in the north of the EEP. The most valuable mines are associated with the model of the Arkhangelsk type, developed on the examples of the known pipes of the Zimnyi Bereg region. At the same time, the study and generalization of data on the petrophysical features of kimberlites, host and overlying rocks, and low mineralogical signal, especially from some bodies of the magnesian series, makes it possible to assume the exis-tence of kimberlite pipes within the covered territories of the Russian Plate with no geophysical and mineralogical anomalies. It requires the development of new methods (structural, remote, geochemical, etc.) for their discovery. Based on the study of primary diamond-bearing bodies, their main features are formulated. These signs should be guided during diamond prospecting within the Karelian-Kola subprovince.
The authors are grateful to N.V.Antonova (ALROSA) and I.S.Galushkina (TsNIGRI), who provided illustrations for the article, as well as the colleagues from the Geological Survey of Finland (Dr. H.O’Brian, Dr. M.Lehtonen) and the Arctic Star Exploration of Canada (Buddy Doyle) for valuable advice and assistance in writing the paper.
References
- De Wit M. Identification of global diamond metallogenic clusters to assist. Conference Diamonds Source to Use, 1-3 March 2010, Johannesburg, Botswana. The Southern African Institute of Mining and Metallurgy, 2010, p. 15-38.
- Kopylova M.G., Afanasiev V.P., Bruce L.F. et al. Metaconglomerate preserves evidence for kimberlite, diamondiferous root and medium grade terrane of a pre-2.7 Ga Southern Superior protocraton. Earth and Planetary Science Letters. 2011. Vol. 312.
Iss. 1-2, p. 213-225. DOI: 10.1016/j.epsl.2011.09.057 - Tappe S., Smart K., Torsvik T., Massuyeau M. et al. Geodynamics of kimberlites on a cooling Earth: Clues to plate tectonic evolution and deep volatile cycles. Earth and Planetary Science Letters. 2018. Vol. 484, p. 1-14.
- Samsonov A.V., Larionova Yu.O., Salnikova E.B. et al. Isotopic geochemistry and geochronology of Paleoproterozoic metakimberlites of the Kimozero occurrence (Central Karelia). Materialy IV Vserosciiskoi konferentsii “Izotopnye sistemy i vremya geologicheskikh protsessov”, 2-4 iyunya 2009, Sankt-Peterburg. St. Petersburg, 2009, p. 158-161 (in Russian).
- Priyatkina N., Khudoley A.K., Ustinov V.N., Kullerud K. 1.92 Ga kimberlitic rocks from Kimozero, NW Russia: Their geochemistry, tectonic setting and unusual field occurrence. Precambrian Research. 2014. Vol. 249, p. 162-179.
DOI: 10.1016/j.precamres.2014.05.009 - Ushkov V.V. Kimozero occurrence of diamond-bearing kimberlites in the Onega structure. Geologiya i poleznye iskopaemye Karelii. 2001. N 3, p. 94-98 (in Russian).
- Ustinov V.N., Neruchev S.S., Zagainyi A.K. et al. Diamonds in the north of the East European Platform. St. Petersburg: Nauka, 2021, p. 410 (in Russian).
- Ustinov V.N. Terrigenous diamond-bearing rocks of the Siberian, East European, and African platforms. St. Petersburg: Nauka, 2015, p. 531 (in Russian).
- O’Brien H.E., Phillips D., Spencer R. Isotopic ages of Lentiira – Kuhmo – Kostomuksha olivine lamproite – Group II kimberlites. Bulletin of the Geological Society of Finland. 2007. Vol. 79, p. 203-215.
- Belyatskii B.V., Nikitina L.P., Savva E.V., Levskii L.K. Isotopic signatures oflamproite dikes on the Eastern Baltic shield. Geochemistry International. 1997. Vol. 35, p. 575-579 (in Russian).
- O’Brien H., Bradley J. New kimberlite discoveries in Kuusamo, northern Finland. 9th International Kimberlite Conference, Frankfurt, Germany. IKC, 2008. Vol. 9. A00346.
- O`Brien H.E., Tyni M. Mineralogy and geochemistry of kimberlites and rocks from Finland. 7th International Kimberlite Conference. Cape Town, Republic of South Africa. Red Roof Design, 1999. Vol. 2, p. 643-645.
- Capdevila R., Arndt N., Letendre J., Sauvage I.F. Diamonds in volcaniclastic komatiites from French Guiana. Nature. 1999. Vol. 399 (6735), p. 456-458. DOI: 10.1038/20911
- Canales D.G. The Akwatia diamond field, Ghana, West Africa: source rocks: Unpubl. MSc thesis, New Mexico inst. Mining and Technilogy. Socorro, New Mexico, 2005, p. 143.
- Davis D.W., Hirdes W., Schaltegger U. U-Pb age constraint on deposition and provenance of Birimian and gold-bearing Tarkwaian sediments in Ghana, West Africa. Preсambrian Research. 1994. Vol. 67. Iss. 1-2, p. 89-107. DOI: 10.1016/0301-9268(94)90006-X
- Gueye M., Seigemund S., Wemmer K. et al. New evidence for an early Birimian evolution in the West African Craton: an example form Kedougou-Kenieba inlier, southeast Senegal. South African Journal of Geology. 2007. Vol. 110. N 4, p. 534-551.
DOI: 10.2113/gssajg.110.4.511 - Sablukov S.M., Kaminskii F.V., Sablukova L.I. Ancient diamond-bearing igneous rocks of non-kimberlite type.Trudy V Mezhdunarodnogo seminara “Problemy istochnikov glubinnogo magmatizma i plyumy”, 02-04 iyunya 2009, Sankt-Peterburg, Rossiya. Irkutsk – Petropavlovsk-Kamchatskii: Izd-vo Instituta geografii SO RAN, 2005, p. 116-134 (in Russian).
- Kaminsky F.V., Sablukov S.M., Sablukova L.I. Diamondiferous Archaean lamprophyres with komatiitic affinities from the Wawa area, Ontario, Canada. 8th International Kimberlite Conference. Victoria, Canada, 2003, p. 123. DOI: 10.29173/ikc3248
- Ustinov V.N. Prediction and prospecting types of reflection of buried kimberlite fields in terrigenous diamond-bearing rocks. Journal of Mining Institute. 2009. Vol. 183, p. 149-159 (in Russian).
- Pokhilenko N.P., Sobolev N.V., Yefimova E.S. et al. Crystalline Inclusions in Diamonds from Kimberlites of the Snap Lake Area (Slave Craton, Canada): New Evidences for the Anomalous Lithospheric Structure. Doklady Earth Sciences. 2001. Vol. 380, p. 806-811 (in Russian).
- Ustinov V.N., Antaschuk M.G., Zagainy A.K. et al. Prospects of Diamond Deposits Discovery in the North of the East-European Platform. Ores and metals. 2018. N 1, p. 11-26 (in Russian).
- Petrographic Codex. Magmatic and metamorphic formations. Ex. ed. O.A.Bogatikov. St. Petersburg: VSEGEI, 1995, p. 128 (in Russian).
- Mitchell R.H. Compositional variation of micas in kimberlites, orangeites, lamproites and lamprophyres. 6th International Kimberlite Conference. Novosibirsk, Russia. 1995. P. 390-392.
- Mahotkin I.L. Petrology of Group 2 kimberlite – olivine lamproite (K2L) from the Kostomuksha area, Karelia, N.W. Russia. 7th International Kimberlite Conference. 1998, p. 529-531. DOI: 10.29173like2793
- Sobolev N.V. Depth inclusions in kimberlites and the problem of the composition of the upper mantle. Novosibirsk: Nauka, 1974, p. 264 (in Russian).
- Gurney J.J., Helmstaedt H., Moore R.O. A review of the use and application of mantle mineral geochemistry in diamond exploration. Pure and Applied Chemistry. 1993. Vol. 65. N 12, p. 2423-2442. DOI: 10.1351/pac199365122423
- Ilupin I.P. New data on the typomorphism of chromium diopside from kimberlites. Prirodnye assotsiatsii, osobennosti sostava i svoistva mineralov-sputnikov almaza. Proceedings TsNIGRI. 1988. Vol. 229, p. 7-9 (in Russian).
- Moore A.E. A model for the origin of ilmenite in kimberlite and diamond: implications for genesis of the discrete nodule (magacryst) suite. Contributions to Mineralogy and Petrology. 1987. Vol. 95, p. 245-253. DOI: 10.1007/BF00381274
- Schulze D.J., Anderson P.F.N., Carter Hearn Jr.B., Hetman C.M. Origin and significance ofilmenite magacrysts from kimberlite. International Geology Review. 1995. Vol. 37. Iss. 9, p. 780-812. DOI: 10.1080/00206819509465427
- Mitchell R.H. Kimberlites: mineralogy, geochemistry, and petrology. New York: Plenum Press, 1986. 442 р.
- Smith C.B., Lucas H., Hall A.E., Ramsay R.R. Diamond prospectivity and indicator mineral chemistry: a western Australian perspective. Fifth International Kimberlite Conference. Minas Gerais, Brazil, CPRM, 1991. Vol. 2/91, p. 380-382.
- Ramsay R.R., Tompkins L. The geology, heavy mineral concentrate, mineralogy, and diamond propectivity of the Boa Esperanca and Cana Verde pipes, Corrego D’anta, Minas Gerais, Brazil. Fifth International Kimberlite Conference. Minas Gerais, Brazil. CPRM, 1991. Vol. 1B, p. 329-345.
- Ramsay R.R., Edwards D., Taylor W.R. et al. Compositions of garnet and spinel from the Aries diamondiferous kimberlite pipe, Central Kimberley Block, Western Australia – implications for exploration. Journal of Geochemical Exploration. 1994. Vol. 51. Iss. 1, p. 59-78. DOI: 10.1016/0375-6742(94)90005-1
- Creighton S. A semi-empirical manganese-in-garnet single crystal thermometer. Lithos. 2009. Vol. 112. Suppl. 1, p. 177-182. DOI: 10.1016/j.lithos.2009.05.011
- Ryan C.G., Griffin W.L., Pearson N.J. Garnet geotherms: pressure-temperature data from Cr-pyrope garnet xenocrysts in volcanic rocks. Journal of Geophysical Research. 1996. Vol. 101. Iss. B3, p. 5611-5625. DOI: 10.1029/95JB03207
- Ashchepkov I.V., Pokhilenko N.P., Vladykin N.V. et al. Reconstruction of mantle sections beneath Yakutian kimberlite pipes using monomineral thermobarometry. Metasomatism in Oceanic and Continental Lithospheric Mantle. London: Geological Society, 2008. Vol. 293, p. 335-352. DOI: 10.1144/SP293.15
- Brey G.P., Kolher T. Geothermobarometry in Four-phase Lherzolites II. New Thermobarometers, and Practical Assessment of Existing Thermobarometers. Journal of Petrology. 1990. Vol. 3. Iss. 6, p. 1353-1378. DOI: 10.1093/petrology/31.6.1353
- Achterbergh E., Griffin W.L., Stiefenhofer J. Metasomatism in mantle xenoliths from the Letlhakane kimberlites: estimation of element fluxes // Contributions to Mineralogy and Petrology. 2001. Vol. 141, p. 397-414. DOI: 10.1007/s004100000236
- 39. Ellis D.G., Green D.H. An experimental study of the effect of Ca upon garnet-clinoperoxene Fe-Mg exchange equilibria. Contributions to Mineralogy and Petrology. 1979. Vol. 71, p. 13-22. DOI: 10.1007/BF00371878
- Simakov S.K. Physical and chemical conditions of formation of diamond-bearing eclogite parageneses in rocks of the upper mantle and crust. Magadan: Severo-Vostochnyi nauchnyi tsentr DVO RAN, 2003, p. 187 (in Russian).
- Kharkiv A.D. Mineralogical bases of diamond deposits prospecting. Moscow: Nedra, 1978, p. 136 (in Russian).
- Zagainyi A.K., Ustinov V N., Zhuravlev V.A. Structural and tectonic factors of kimberlite and lamproite magmatism in the northwest of the East European Platform. Geologiya almaza – nastoyashchee i budushchee (geologi k 50-letnemu yubileyu g. Mirnyi i almazodobyvayushchei promyshlennosti Rossii). Voronezh: Voronezhskii gosudarstvennyi universitet, 2005, p. 79-86 (in Russian).
- Mikoev I.I., Zagainyi A.K. Features of the deep structure and structural and tectonic prerequisites for the localization of diamond-bearing magmatites of the North Onegozero kimberlite region. Problemy prognozirovaniya i poiskov mestorozhdenii almazov na zakrytykh territoriyakh: Materialy konferentsii, posvyashchennoi 40-letiyu YaNIGP TsNIGRI AK “ALROSA”, 18-20 marta 2008, Mirnyi, Rossiya. Yakutsk: Izd-vo Yakutskogo nauchnogo tsentra Sibirskogo otdeleniya RAN, 2008, p. 93-97 (in Russian).
- Fieremans M.R., Fieremans C.L. Diamond in its primary rocks with special reference to the diamond deposits of Mbujimayi, East Kasai, Zaire. Bulletin Society Geology. 1992. Vol. 101, p. 9-39.
- Mainkar D., Lehmann B., Haggerty S.E. The crater-facies kimberlite system of Tokapal, Bastar District, Chhattisgarh, India. Lithos. 2004. Vol. 76. Iss. 1-4, p. 201-217. DOI: 10.1016/j.lithos.2004.04.024
- Berryman A.K., Scott Smith B.H., Jellicoe B.C. Geology and diamond distribution of the 140/141 kimberlite, Fort à la Corne, central Saskatchewan, Canada. Lithos. 2004. Vol. 76, p. 99-114. DOI: 10.1016/j.lithos.2004.04.031
- Podchasov V.M., Minorin V.E., Bogatykh I.Ya. et al. Geology, Forecasting, Methods of Prospecting, Evaluation and Exploration of Diamond Deposits. Book 1: Primary deposits. Yakutsk: Izd-vo Sibirskogo otdeleniya RAN, 2004, p. 548 (in Russian).
- Mikoev I.I. Ground Geophysical Surveys in Kimberlite Bodies Prospecting: Results and Efficiency in Various Prospecting Environments. Ores and Metals. 2018. N 3, p. 40-46 (in Russian). DOI: 10.24411/0869-5997-2018-10005
- Lehtonen M., Marmo J. Glacial dispersion study of kimberlitic material in Quaternary till from the Lahtojoki pipe, eastern Finland. 8th International Kimberlite Conference: Long Abstract. 2003, p. 1-5. DOI: 10.29173/ikc2989
- Tyni M. Diamond prospecting in Finland. A review. Geological Survey of Finland. 1997, p. 789-791.
- Ustinov V., O’Brien H., Lukianova L., Peltonen P. Diamond-bearing kimberlites of northern Europe. 9th International Kimberlite Conference, Frankfurt, Germany, 2008. N IKC-A-00112. DOI: 10.29173/ikc3357
- Sunrise Resources Plc. Relinquishment Report: Lapinluhta 1. URL (accessed 20.02.2021).
- Reed L.E., Witherly K.E. 50 Years of Kimberlite Geophysics, A Review. Proceedings of Exploration 07: Fifth Decennial International Conference on Mineral Exploratio. 9-10 September 2007, Toronto, Canada. Exploration, 2007, p. 679-689.
- Larchenko V.A., Stepanov V.P., Minchenko G.V., Pervov V.A. Age of igneous rocks, ore-bearing strata and Middle Paleozoic reservoirs of the Zimneberezhny diamond-bearing region (Arkhangelsk diamond-bearing province). Geologiya almaza – nastoyashchee i budushchee (geologi k 50-letnemu yubileyu g. Mirnyi i almazodobyvayushchei promyshlennosti Rossii). Voronezh: Voronezhskii gosudarstvennyi universitet, 2005, p. 322-347 (in Russian).
- Pendelyak R.N., Morozov A.V., Mogutova V.A. Geological structure of the V.Grib pipe and its display features in geophysical fields. Otechestvennaya Geologia. 2019. N 5, p. 53-59 (in Russian). DOI: 10.24411/0869-7175-2019-10038
- Meshcheryakov M.G., Golovin N.N., Verichev E.M. Discovery, evaluation and exploration of the diamond deposit named after. V.Grib Gornyi zhurnal. 2014. N 3, p. 22-26 (in Russian).
- Stognii V.V., Korotkov Yu.V. Prospecting for kimberlite bodies by the transient electromagnetic method. Novosibirsk:
Malotirazhnaya tipografiya 2D, 2010, p. 121 (in Russian). - Applied Electromagnetic Research (AEMR). URL (accessed 12.02.2021).
- Abramov V.Y. Petrophysical characteristics of geological-geophysical models of kimberlite pipes of the Archangelsk' kimberlite province. RUDN Journal of Engineering Research. 2008. N 1, p. 70-76 (in Russian).
- Zinchuk N.N., Bondarenko A.T., Garat M.N. Petrophysics of kimberlites and host rocks. Moscow: Nedra, 2002, p. 695
(in Russian). - Kutinov Yu.G., Chistova Z.B. Hierarchical series of occurrences of alkaline-ultrabasic magmatism in the Arkhangelsk diamondiferous province. Their reflection in geological and geophysical materials. Arkhangelsk: Pravda Severa, 2004, p. 283 (in Russian).
- Verzhak V.V. Geological structure, composition, conditions of formation, and exploration methodology of the M.V.Lomo-nosov diamond deposit: Avtoref. dis. … kand. geol.-mineral. nauk. Moscow: Moskovskii gosudarstvennyi universitet im. M.V.Lomonosova, 2001, p. 27 (in Russian).