Deep structure, tectonics and geodynamics of the Sea of Okhotsk region and structures of its folded frame
- 1 — Ph.D., Dr.Sci. Head of Department Saint Petersburg Mining University ▪ Orcid ▪ Elibrary ▪ Scopus ▪ ResearcherID
- 2 — Postgraduate Student Saint Petersburg Mining University ▪ Orcid
- 3 — Ph.D., Dr.Sci. Professor Saint Petersburg Mining University ▪ Orcid ▪ Elibrary
- 4 — Ph.D. Senior Lecturer Saint Petersburg Mining University ▪ Orcid ▪ Elibrary ▪ Scopus ▪ ResearcherID
Abstract
The use of the zonal-block model of the earth's crust for the construction of regional tectonic schemes and sections of the earth's crust based on a complex of geological and geophysical data makes it possible to consider the resulting maps and sections as tectonic models. The main elements of such models are blocks with an ancient continental base and interblock zones formed by complexes of island arcs, an accretionary prism, or oceanic crust. The developed geotectonic model of the Sea of Okhotsk region reflects the features of the deep structure, tectonics, and geodynamics. The Cimmerian Novosibirsk-Chukotka, Verkhoyansk-Kolyma, Kolyma-Omolon, and Amur folded regions and the Alpides of the Koryak-Kamchatka and Sakhalin-Sikhote-Alin folded regions are developed along the northern, western, and southern boundaries of the Sea of Okhotsk megablock with a continental crust type. From the east, the megablock is limited by oceanic basins and island arcs.
Introduction
The research area covers the geostructures of the transition zone from the Eurasian continent to the Pacific oceanic plate. The formation of geostructures in the region is associated with the manifestation of Mesozoic and Cenozoic subduction processes, and collisional and shear attachment of island arcs and microplates to the margin of the Eurasian continent. Paleoplates (megablocks with continental-type crust), suture (interblock) zones, island arcs and active continental margins, deep-water trenches, and oceanic basins are distinguished here. Within the study region, there are areas of Cimmerian folding: Novosibirsk-Chukotka, Verkhoyansk-Kolyma, Kolyma-Omolon, Amur, and Okhotsk-Chukotka. Alpine orogenic structures are represented by the Sikhote-Alin, Sakhalin, and Koryak-Kamchatka folded areas. The Japanese, Kuril-Kamchatka, and Aleutian subduction zones are modern convergent structures. The oceanic depressions of the Sea of Japan, the Sea of Okhotsk, and the Bering Sea are mapped in the region.
In connection with the solution of a wide range of problems of mineral resource use, the Far Eastern region has long been studied with a wide range of geological, geophysical, and remote sensing methods.
Until the 1970-1980s, within the framework of the fixist ideology, the Sea of Okhotsk region was considered a zone of transition from the Asian continent to the Pacific Ocean [1, 2]. The first tectonic map of the region at a scale of 1:2500000, made within the framework of the tectonics of lithospheric plates, was developed by a team of authors of the Russian Academy of Sciences, edited by N.A.Bogdanovа [3]. A great contribution to the study of the deep structure was made by the works of I.P.Kosminskaya, S.M.Zverev, Yu.V.Tulina, I.S.Berzon, and other employees of the Institute of Physics of the Earth RAS, carried out jointly with the Institute of Marine Geology and Geophysics. The studies were based on deep seismic sounding data obtained from 1957 to 1969. Since the 80s of the 20th century, the Dalmorneftegeofizika trust has been conducting seismic surveys at a higher technical level, during which the block structure of the acoustic basement was established, large sedimentary basins were delineated, interregional correlation and stratification of the sedimentation basins of Sakhalin, Northern Okhotsk and Western Kamchatka were carried out.
Deep seismic soundings in Kamchatka were carried out by S.T.Balesta, V.K.Utnasitny, V.V.Mishin, S.P.Belousov, N.I.Pavlenkova, et al. A wide range of specialized studies of the region was carried out by Japanese, Chinese, European, and North American scientists [4-6].
Problem statement
Extensive factual material has been accumulated on the territory of the region under consideration, which allows performing geotectonic constructions on a new scientific and methodological level. The results of the State Geological Mapping at a scale of 1:1000000 (GGK-1000) performed under the scientific and methodological supervision of FSUE “VSEGEI” are of fundamental importance for the solution of the set objectives. The GGK-1000 database includes materials of gravity and magnetic surveys at a scale of 1:200000 and calculations of their transformations, results of specialized remote sensing, and geomorphological and geochemical studies [7-9]. The data of bathymetric surveys, seismic (DSS and CDP seismic reflection method), seismic tomography, geothermal, magnetotelluric surveys, geological mapping, deep-sea drilling, geoacoustic profiling, etc. are used [10-12].
The most detailed set of modern geological and geophysical surveys was applied by “Sevmorgeo” in 2010 along the geophysical reference profiles “2-DV-M” and “1-OM”, crossing the Okhotsk Sea water area [13, 14]. The complex surveys included onboard gravimagnetic observations, airborne gravimetric and aeromagnetometric surveys, gas geochemical studies, seismic exploration of DSS and CDP seismic reflection methods, and surface seismoacoustic profiling.
In 2010-2021 in the Sea of Okhotsk, only prospecting and exploration work was carried out in licensed areas. It can be assumed that the regional stage of research in this region is nearing completion. However, despite a significant amount of geological and geophysical research carried out in the Sea of Okhotsk and adjacent territories, the question about the stages of formation of the main tectonic structures of the region, characteristic of the successively changing geodynamic conditions, remains debatable. In this regard, it is necessary to develop a geological and structural framework of the region, reflecting the characteristic features of the structure of the basement and sedimentary cover in lateral and radial dimensions. An important step toward creating such a framework is the development of a zonal-block model of the Earth's crust structure based on the study of the appearance of simulated structures in the geophysical fields [15, 16].
Methodology
To analyze the regularities of the spatial distribution of fields and their subsequent interpretation, the regional and local components of the gravity and magnetic fields, the total horizontal (module) and vertical gradient of the gravity field, the slope of the derivative (tilt transformation), the pseudo gravity field and artificial pseudo-relief maps were calculated.
When zoning the territory according to the complex of geophysical fields, classification algorithms with pattern recognition without training were used. In the course of the research, classifications of potential fields implemented in the COSCAD 3D software package were applied. Additionally, the classification of fields was performed using the algorithm of D.S.Zelenetsky in the automated data management system “Air”. In the course of a qualitative interpretation of potential fields and their transformants, blocks, interblock zones, volcanic belts, faults were identified and their geotectonic interpretation was made (Fig.1, a).
To build an interpretive section of the region, a section of the composite profile A-B was chosen, the position of which is shown in Fig.1, a. Since the 2-DV-M profile (length 1700 km) is purely marine, in order to develop a unified deep structure model illustrating the structures of the sea and adjacent land, the composite profile was extended to the adjacent part of the mainland.
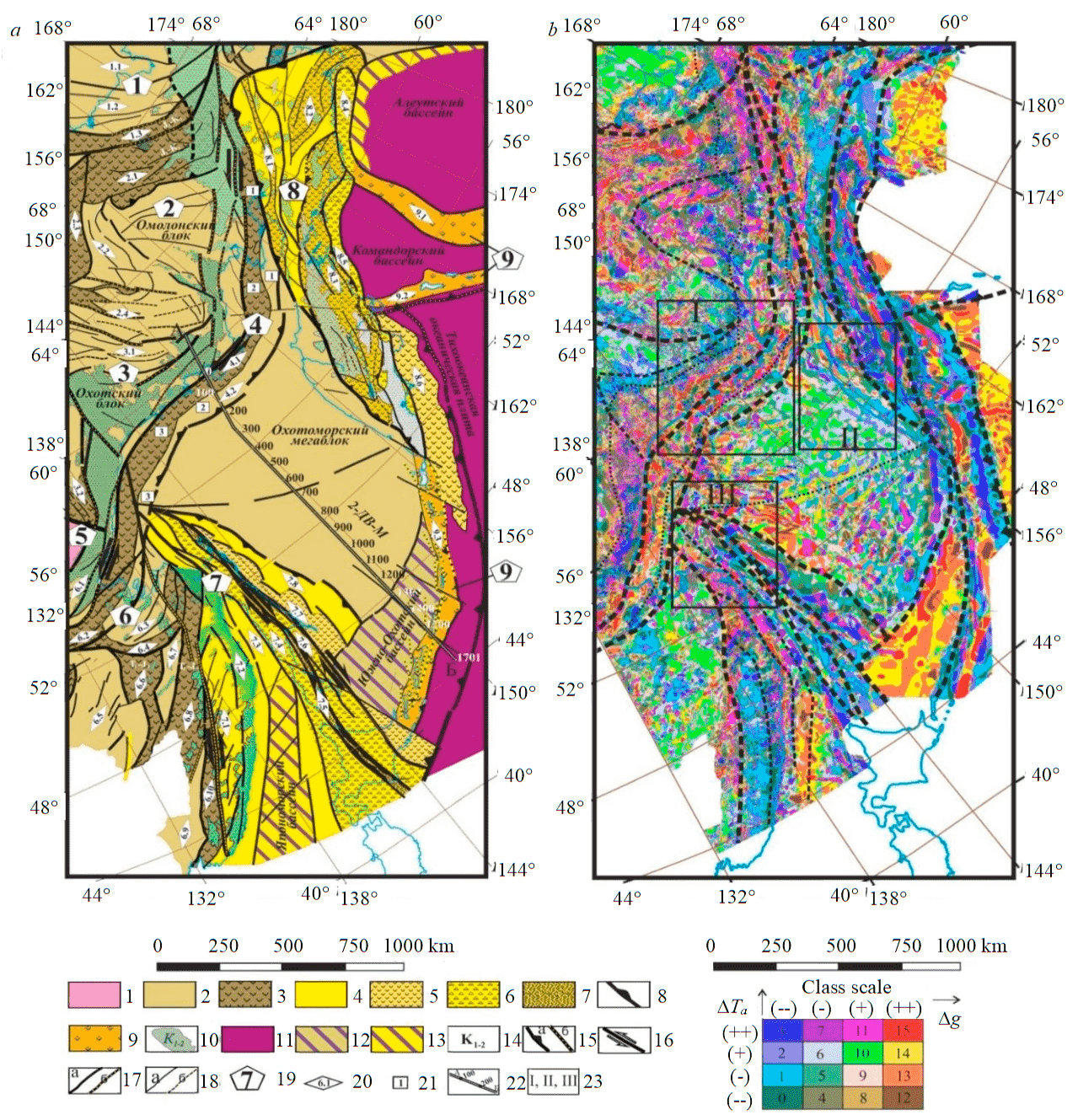
Fig.1. Tectonic zoning of the consolidated crust of the Sea of Okhotsk region and structures of its folded framing (a); standard-free classification of the local component of potential fields (b) (ΔTa is the anomalous magnetic field; Δg is the gravity field) 1-11 – structural and material complexes of the consolidated crust: 1 – ancient platforms; 2, 3 – Cimmerian folded areas (FA): 2 – blocks; 3 – interblock (suture) megazones; 4-6 – Alpine FA: 4 – blocks; 5, 6 – interblock (suture) megazones (5 – composed of poorly defined volcanic sedimentary island-arc complexes, 6 – filled with accretionary prism formations); 7 – ophiolites; 8 – subduction zone; 9 – complexes of modern island arcs; 10 – complexes of active continental margins; 11 – oceanic crust complexes; 12, 13 – crustal complexes of transitional continental-oceanic type: 12 – on Cimmerian basement, 13 – on Alpine basement; 14 – age of complexes of interblock zones; 15-18 – faults: 15 – boundaries of folded areas (a – thrust, b – other); 16 – regional shifts; 17 – boundaries of structural zones (a – thrust; b – other), 18 – secondary faults (a – thrust; b – other); 19 – index of the main geostructures; 20 – structure index; 21 – structure segment index; 22 – line of the composite geological and geophysical section A-B (Fig.2); 23 – the boundaries of the contours for Fig.3-5, respectively Index of the regional structures: 1 – Novosibirsk-Chukotka folded area: 1.1 – Rauchanskaya, 1.2 – West Chukotka, 1.3 – Yuzhno-Anyuiskaya; 2 – Kolyma-Omolon folded area: 2.1 – Oloi, 2.2 – Prikolimo-Omolon, 2.3 – Polusnenskaya, 2.4 – Uyandina-Yasachnenskaya; 3 – Verkhoyansk-Kolyma folded area: 3.1 – Adyga-Suntarskaya, 3.2 – Yuzhno-Verkhoyansk; 4 – Okhotsk-Chukotka collision-active marginal folded area: 4.1 – Okhotsk-Chukotka suture zone (1 – Murgalsky, 2 – Yuzhno-Taigonossky, 3 – Tauisky), 4.2 – marginal fold-thrust belt (1 – Shelikhov, 2 –Primagadansky, 3 – Bilibinsky); 5 – Aldan-Stanovoi shield of the Siberian platform; 6 – Amur collisional-active marginal folded area: 6.1 – Stanovaya, 6.2 – Amur-Okhotsk, 6.3 – Nimelenskaya, 6.4 – Ulbanskaya, 6.5 – Bureinskaya, 6.6 – Badzhalskaya, 6.7 - Gorinskaya, 6.8 – Nizhne-Amurskaya, 6.9 – Khankaiskaya, 6.10 – Samarka zone; 7 – Sakhalin-Sikhote-Alin collisional-active marginal folded area: 7.1 – Central Sikhote-Alin, 7.2 – East Sikhote-Alin, 7.3 – Tatar trough, 7.4 – West Sakhalin, 7.5 – Merey, 7.6 – East Sakhalin, 7.7 – East Sakhalin-Nemuro, 7.8 – East Sakhalin trough; 8 – Koryak-Kamchatka accretionary-collisional active marginal folded area: 8.1 – West Koryak, 8.2 – Central Koryak, 8.3 – Olyutorskaya, 8.4 – Khatyrskaya, 8.5 – Vetlovsko-Govenskaya, 8.6. – Kronotskaya zone; 9 – island arcs: 9.1 – Shirshova, 9.2 – Aleutsko-Komandorskaya, 9.3 – Kurilskaya
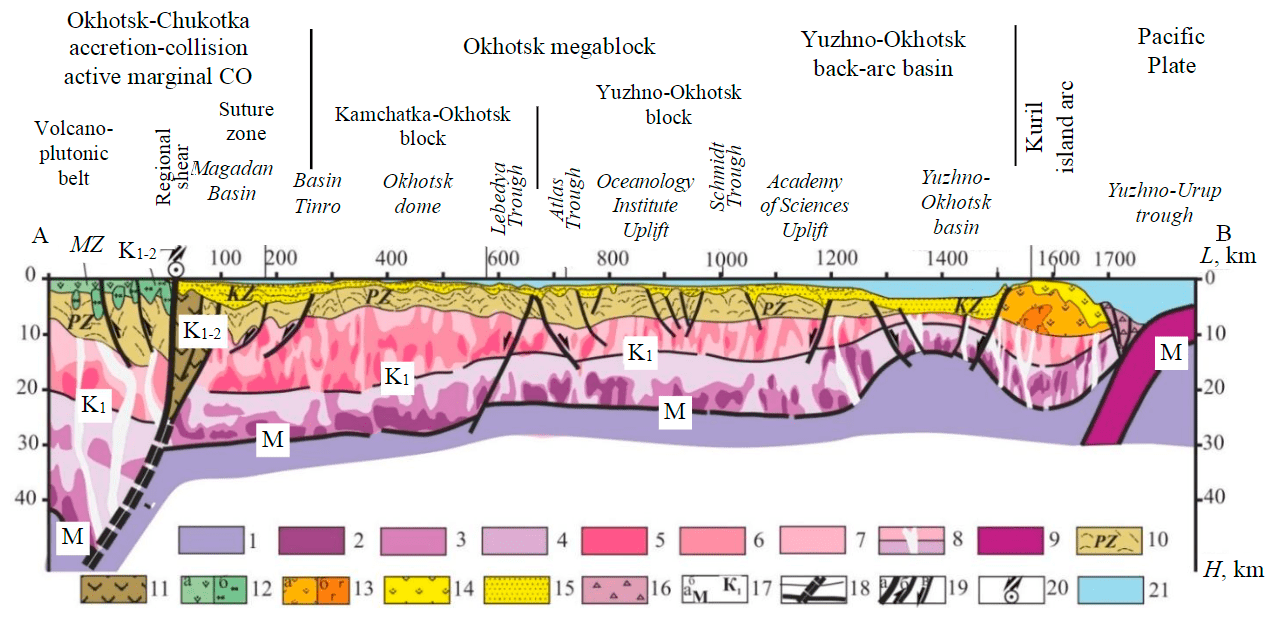
Fig.2. Deep geological and geophysical section along the composite seismic line A-B 1-16 – structural and material units of the lithosphere: 1 – upper mantle; 2-4 – lower crust, differentiated by the reflection parameter (2 – high, 3 – increased, 4 – medium); 5-7 – upper crustal (granite-gneiss) layer, differentiated by the reflection parameter (5 – high, 6 – increased, 7 – medium); 8 – areas of reduced reflection of the crystalline crust; 9 – oceanic crust; 10 – deformed sediments of the consolidated basement; 11 – complexes of ancient island arcs and accretionary prisms within suture zones; 12 – sedimentary-volcanogenic complexes of the active continental margin and platform cover (a) and intrusive massifs (b); 13 – weakly lithified sedimentary-volcanic complexes (a) and intrusive massifs of the island arc (b); 14 – recent sediments and island arc volcanic rocks; 15 – sedimentary complexes of the platform cover; 16 – accretionary prism complexes; 17 – boundaries of the radial layering of the earth's crust, including the base of the earth's crust (M) (a) and the granite-gneiss layer (K1) (b); 18 – deep channel of the Okhotsk-Chukotka suture zone; 19 – discontinuous disturbances (a – global, b – regional, c – secondary); 20 – mark of the global left-sided shear zone; 21 – layer of sea water
To construct a geological and geophysical section along the 2-DV-M geotraverse, the published seismic section of the CDP seismic reflection method the calculated velocity section in this section [14], and the seismic section in terms of “reflectivity” made using the ArcMap software package [15] were used.
For depth mapping of structures along the composite section, the inverse problem of gravity exploration in COSCAD 3D was solved. The constructed pseudo-density sections in combination with seismic data made it possible to estimate the distribution of structural and compositional complexes of the earth's crust in the section of the composite profile (Fig.2).
A complex tectonic interpretation of the heterogeneous actual materials used is successfully carried out within the framework of the zonal-block model of the earth's crust. Within the framework of this methodological approach, the most important elements of the developed model of the earth's crust are blocks, which are taken as conditionally stationary areas of geophysical parameters, and for interblock zones – areas of change in the structure of geophysical fields. The term “block” in the tectonic interpretation of the complex of geophysical data corresponds to the continental parts of the paleoplates; interblock zones are sutures of collisional orogens, rift zones, and regional strike slips [16]. Interblock zones appear as narrow, gently dipping deep channels with a wedge-shaped synform in the upper crust.
A powerful tool of the current stage of research is the geodynamic analysis of geological and geophysical data. To model structural and compositional inhomogeneities of the earth's crust, a parametric description of structures – tectonotypes, formed in various geodynamic settings (structural, material, and morphological parameters of their deep structure, features of sedimentation, magmatism, metamorphism, and appearance in geophysical fields) was used. Significant progress in the recognition of types of deep structures is associated with the use of modern technologies of “pattern recognition with learning” based on the involvement in the construction of the analogs study results.
Tectonic maps (schemes) and geological and geophysical sections of the earth's crust and upper mantle reflect the patterns of distribution of the main structural and material units of the earth's crust in the Sea of Okhotsk region as a result of geodynamic processes of the Cimmerian and Alpine orogenic cycles.
Discussion
On the compiled tectonic scheme of the study area, Late Cimmerian structures of the Novosibirsk-Chukotka, Kolyma-Omolon, Verkhoyansk-Kolyma, and Amur collisional folded regions are distinguished. The Okhotsk-Chukotka collision-active folded region occupies a border position with the Sea of Okhotsk continental megablock. Along the northern and southwestern flanks of the Sea of Okhotsk megablock, the structures of the Koryak-Kamchatka and Sakhalin-Sikhote-Alin accretionary-collisional active marginal folded areas develop. On the eastern flank of the study area, the island arcs Shirshov, Aleutsko-Komandorskaya, and Kuril, as well as the Pacific oceanic basin and back-arc oceanic basins – the Commander and Aleutian, Yuzhno-Okhotsk (see Fig.1, a) are mapped. These structures are confidently manifested in potential geophysical fields and on the map of the non-standard classification of potential fields, calculated on the basis of the integration of maps of anomalous magnetic and gravitational fields (Fig.1, b) and on the deep geological and geophysical section (Fig.2). As a rule, for the largest geotectonic structures, the same type of manifestation in the potential fields is established [17].
Cimmerian structures
The northern and western flanks of the research area are occupied by the Novosibirsk-Chukotka, Verkhoyansk-Kolyma, and Kolyma-Omolonsk folded regions.
The Novosibirsk-Chukotka collisional region is represented by structures of the Northeastern block with continental-type crust and the Yuzhno-Anyui suture zone. The Northeastern block is characterized by homogeneous isometric gravitational and magnetic fields; The Anyui zone is distinguished by a system of longitudinal linear anomalies.
The northeastern block, which includes the Rauchan and Western Chukotka zones [18], is the southern margin of the Hyperborea continental plate deformed by Late Cimmerian folding. The formations of the ancient crystalline crust correspond to the Grenville or Baikal basement [19]. Permian-Triassic-Lower Jurassic terrigenous deposits occur with some unconformity in the Paleozoic and are intensely deformed before the Late Jurassic. The Upper Jurassic and Lower Cretaceous form molassoid superimposed depressions on the coast. The degree of dislocation of deposits increases towards the southeast. The formations of the Okhotsk-Chukotka volcanic-plutonic belt overlap the eastern flank of the considered fragment of the megastructure.
The formation of the Yuzhno-Anyui suture zone is associated with the closure of the Late Jurassic-Early Cretaceous Yuzhno-Anyui paleoocean. The section of the zone is composed of Permian ophiolites unconformably overlain by Berriasian-Valanginian terrigenous flysch and Late Jurassic-Early Cretaceous accretionary prism complexes. The collisional orogeny belongs to the Early-Late Cretaceous and manifests itself in the form of intermountain sedimentary basins and volcano plutonic areas [18]. The formations of the eastern flank of this collisional orogen are overlain by the Early-Late Cretaceous complexes of the Okhotsk-Chukotka volcano plutonic belt.
The Kolyma-Omolon folded area (FA) within the study area is represented by the Early Precambrian continental Omolon block, framed by the Oloy, Polusna and Uyandina-Yasachna suture zones.
Gneisses, amphibolites, granites, and migmatites of Archean age, metamorphites, ultramafic rocks, and crystalline schists of primary sedimentary origin of Early Proterozoic age are mapped in the crystalline basement high in the central part of the Omolon block and on its flanks (Prikolimo-Omolon zone). The overlying shelf carbonate-terrigenous (Paleozoic) and terrigenous (Mesozoic) formations are weakly deformed. Within the suture zones, formations of Paleozoic and Mesozoic island arcs accreted in the Middle Jurassic are mapped.
In potential geophysical fields, the homogeneous Omolon block is framed by linear anomalies of suture zones. The eastern boundary of the block with the structures of the Okhotsk-Chukotka volcanogenic belt is contrasted by a sharp change in the character of the fields.
The Verkhoyansk-Kolyma folded region is represented in the study area by the Adyga-Suntar and Yuzhno-Verkhoyansk suture zones framing the Okhotsk continental block. The formation of the folded area is associated with the successive attachment of the Okhotsk, Omolon, and Indigiro-Alazeya paleoplates to the margin of the Siberian paleo plate.
The Okhotsk block has, presumably, an ancient Early Precambrian basement, as indicated by U-Pb zircon dating [20]. Archean gneisses, crystalline schists, amphibolites, Early Proterozoic shales, Riphean, Paleozoic and Mesozoic shelf carbonate-terrigenous and terrigenous complexes have been mapped within its boundaries. Sedimentary complexes are folded and broken by faults at the end of the Jurassic and the beginning of the Cretaceous. Characterized by large plutons of granitoids, the formation of which occurred simultaneously with tectonic deformations.
An analysis of the spatial distribution of gravity and magnetic fields shows that the Okhotsk block is part of a large paleo plate with continental-type crust. In potential fields, the range of stationary field values in the central part of the block is framed by linear zones on its periphery. Its southeastern border with the Okhotsk-Chukotka suture zone is contrasted by a sharp change in the field infrastructure. A less clear picture of the zonation of geophysical fields in comparison with the Omolon block suggests that the Okhotsk massif is to a greater extent deformed by Cimmerian fold-thrust structures. Cretaceous volcanic formations widely developed in the region are manifestations of an active continental margin.
Shear dislocations of the Arctic-Asian regional shear zone are developed along the boundary of the Omolon and Okhotsk blocks (see Fig.1, a). The latter is traced from the Gakkel Ridge through the Chersky Ridge by neotectonic reverse faults with an amplitude of up to 2.0-3.1 km. The northern branch of these dislocations extends to the Shelikhov Bay of the Sea of Okhotsk and further into the Aleutian subduction zone [21]. By analogy with the North American San Andres zone, V.S.Imaev (2019) considers this structure as a classical transform dislocation that changes its morphological and kinematic type along the strike: the tensile strains of the Gakkel oceanic ridge on the continent are transformed into a left-sided regional shear.
The Okhotsk-Chukotka accretionary-collisional active marginal folded area was formed as a result of successive manifestations of various geodynamic settings: subduction structures of the Late Mesozoic Okhotsk-Chukotka volcanic belt of the active continental margin and the Okhotsk-Chukotka collisional orogen, which was formed in the area of the collisional junction of the Eurasian and Okhotsk paleocontinents. For FA, a clear linear zoning of fields is characteristic. The western flank of the region, which corresponds to the area of development of the Okhotsk-Chukotka volcanic belt, is characterized by high values of the magnetic field and low values of the gravitational field.
The Okhotsk-Chukotka volcanic belt was formed in the Early-Late Cretaceous epoch under the conditions of subduction of the lithospheric plate under the edge of the Eurasian continent. Near the day surface, its position is marked by the Okhotsk-Chukotka suture zone and its deep channel, experiencing a general westward dip (Fig.2). In the reference section, the volcanic rocks of the belt, underlain by
deformed sediments of the Mesozoic and Paleozoic ages, overlie the Okhotsk block with continental-type crust up to 50 km thick. The section shows a lower crustal layer (up to 22 km) with velocities of 6.5-6.8 km/s and an upper crustal layer 16-18 km thick with velocities of 6.2-6.4 km/s [13, 22].
The Okhotsk-Chukotka collisional orogen was formed as a result of the collision of the Sea of Okhotsk and the Eurasian Paleolithic. This process marked the regression of the subduction zone, the completion of magmatism in the Okhotsk-Chukotka volcanic belt, the formation of the Okhotsk-Chukotka suture zone and fold-thrust structures of the orogen.
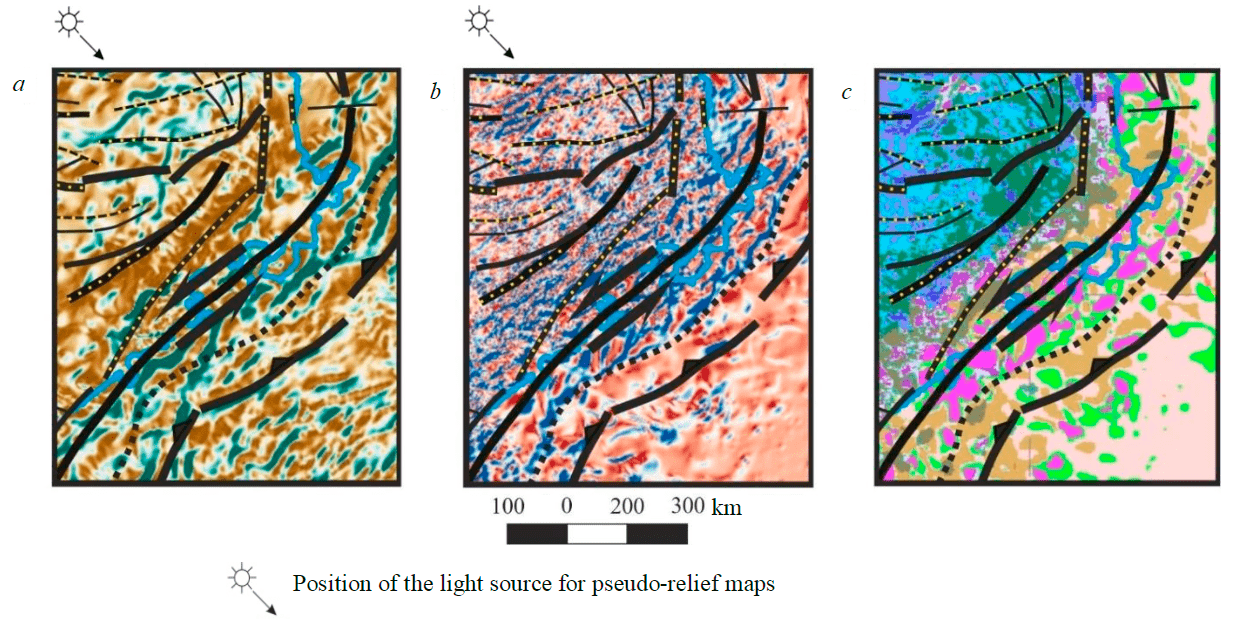
Fig.3. Manifestation of the structures of the Okhotsk-Chukotka accretionary-collisional active marginal folded area in geophysical fields: a – gravitational field pseudo-relief; b – pseudo-relief of the magnetic field; c – standard-free classification of potential fields (the position of the area corresponds to inset I in Fig.1, b) See the scale of classes in Fig.1
Mapping of the Okhotsk-Chukotka suture zone is based on tracking in the geophysical fields of the North Murgal and Yuzhno-Taigonos segments of this zone (see Fig.1). Late Paleozoic passive marginal complexes, volcanogenic-terrigenous formations of accretionary belts (Barremian Stage), and volcanogenic Late Permian-Early Cretaceous complexes of developed island arcs, as well as a gabbro-peridotite association of oceanic rifts and abyssal plains of the ocean floor, are distinguished here. In the central part of the zone (Tauy segment), andesite-basalt and gabbro-diorite-granodiorite associations of island arcs (Lower Cretaceous) and accretionary belts are mapped [17]. In potential fields, the zone corresponds to linear positive anomalies of the gravitational field (Fig.3, a); in the magnetic field, there is a clear linear zoning with numerous high-amplitude positive local anomalies (Fig.3, b). The area of development of anomalies makes it possible to map the boundaries of this suture zone along its entire length. The zone is confidently traced on the map of potential field classes (Fig.3, c). The presence of accretionary prism complexes and island arcs indicates the development of an ensimatic island arc in the Early Cretaceous.
In the crustal section (see Fig.2, survey markers 0-100 km) the zone is modeled in the form of a wedge-shaped structure with a narrow deep channel dipping in the western direction. This suture structure is manifested in the section by a sharp change in the thickness of the crust from 50 to 30 km.
A regional shear zone develops along the western flank of the suture. The eastern flank of this left-lateral strike-slip zone develops in a stretching environment that determines the configuration, thickness, and filling of the Cenozoic sedimentary basins. In the north of the Sea of Okhotsk, the sutural zone is overlapped by the Magadan Depression, which has a considerable thickness with horizontally persistent layers. In the deep section, the Moho boundary is elevated in comparison
with the adjacent sections of the profile [16, 26].
The existence of a strike-slip component along the western flank of the Okhotsk-Chukotka suture zone subsequently led to the initiation of pull-apart rifts and horsts. Riftogenic subsidence of the earth's crust led to the formation of the Shantar, Kashevarovsky, Lisyansky, Yamsko-Tauysky, Gizhiginsky, Magadansky, Shelikhovsky, and Penzhinsky basins along the margin of the Eurasian continent. The average thickness of the cover in the basins reaches 10 km.
The Amur folded region (AFR) was formed at the end of the Mesozoic during the closure of the Mongolian-Okhotsk segment of the Pacific Ocean during a long-term collisional juncture of the Siberian, Sino-Korean continents and smaller microplates with the initiation of the structures of the Mongolian-Okhotsk suture, the Amur-Okhotsk and Ulban zones, the Lower Amur and Samarka suture zones, as well as the deformed margins of the Siberian and Sino-Korean paleocontinents (the eastern flank of the Stanovoi megablock [23], the Bureinskii and Khankaiskii massifs).
The spatial distribution of potential fields reflects the position of blocks with isometric fields and interblock zones with linear field morphology.
The Stanovoi megablock has a continental-type crust with a thick granite metamorphic layer (more than 25 km) and an increased density of the lower crust. As a result of Mesozoic collisional processes against the background of the ancient Archean crust, granitoid intrusive massifs are laid within its boundaries. In the Late Jurassic – Early Cretaceous, a volcano-plutonic complex of the active continental margin formed on the southeastern flank of the megablock.
The Amur-Okhotsk and Ulban zones are the borders of the Siberian and Sino-Korean continents. These zones are characterized by an imbricated-thrust structure of deformations and a heterogeneous material composition: fragments of the oceanic crust, accretionary prism complexes, and rift formations of the Mesozoic and Cenozoic ages. In the cross sections of the Rubtsovsk – Nevelsky Cape profile, the zone appears as a thick accretionary wedge sloped toward the Siberian Plate [16].
The Lower Amur suture zone is filled with Paleozoic-Lower Mesozoic basalts, accretionary prism diabases, and oceanic siliceous-argillaceous complexes. These formations are intensively dislocated by numerous discontinuous faults.
The basement of the Bureinskii and Khankaiskii blocks includes Archean and Early Proterozoic complexes of deeply metamorphosed rocks. The extensive Bureinskii block is manifested by the isometric potential field anomalies (see Fig.1, b). Collisional granitoids of Paleozoic and Mesozoic ages are widely shown here. Paleozoic and Mesozoic terrigenous-carbonate complexes of passive margins and volcanogenic complexes of Late Paleozoic active margins are distinguished.
An important conclusion about the history of the development of the region can be drawn from the fact of the spatial correlation of the Amur-Okhotsk (AO) and Okhotsk-Chukotka suture zones of the accretionary-collisional active marginal folded area of the same name. The map of the non-standard classification of fields (Fig.1, b) shows that linear anomalies of classes 14 and 15 (anomalously high values of the gravitational field, high and elevated values of the magnetic field) extend without distortion from the Okhotsk-Chukotka to the Amur-Okhotsk zone.
The Sea of Okhotsk megablock with a crustal thickness of about 25-30 km is located in the waters of the Sea of Okhotsk [24]. Its upper crust (granite-gneiss layer) has longitudinal wave velocities of 5.7-6.3 km/s and a lower crust of 6.5-6.9 km/s. The thickness of the crust decreases in the direction from the continent to the Kuril Islands, which is presumably associated with the impact of the subduction zone and the manifestation of the mantle diapir [22].
On the daytime surface, its northeastern flank is exposed only within the West Kamchatka zone. The discovery of numerous zircons dating from the Archean to the Early Cretaceous, which underwent regional metamorphism in the Late Cretaceous 77 Ma [25], indicates the continental type of the megablock crust [26]. Its ancient consolidated basement [26] is overlain by the Late Cretaceous-Cenozoic cover, the thickness of which, determined from geophysical data, varies within 1-12 km [27]. Lower and Upper Cretaceous granitoids and felsic volcanic rocks have been mapped on basement uplift [28, 29].
The megablock is characterized by an isometric spatial distribution of geophysical fields, which in the boundary zones with adjacent folded areas acquire elements of linear zoning (Fig.1, b). The megablock is divided by a deep fault into two blocks, the deep structure of which differs significantly (Fig.2). The northern Kamchatka-Okhotsk block is characterized by relatively shallow depths and is manifested by alternating weakly elevated gravitational and magnetic fields. Its deep section has an increased thickness of up to 30 km (Fig.2, survey markers 0-650). The upper part of the section is composed of a layer of ancient sediments and a Cenozoic cover. The South-Okhotomorsk block is distinguished by a sign-variable gravitational field with an overall elevated magnetic field value. It is characterized by a reduced thickness of the earth's crust of up to 25 km with a regular increase in the velocity of elastic waves towards the South Okhotsk trough (Fig.2, survey markers 650-1500). This phenomenon is associated with the fact that the Earth's crust becomes more basic as the depth of the subduction zone decreases [22]. The positions of possible channels of deep fluids inflow into the Earth's crust are modeled by areas of reduced reflectivity (Fig.2).
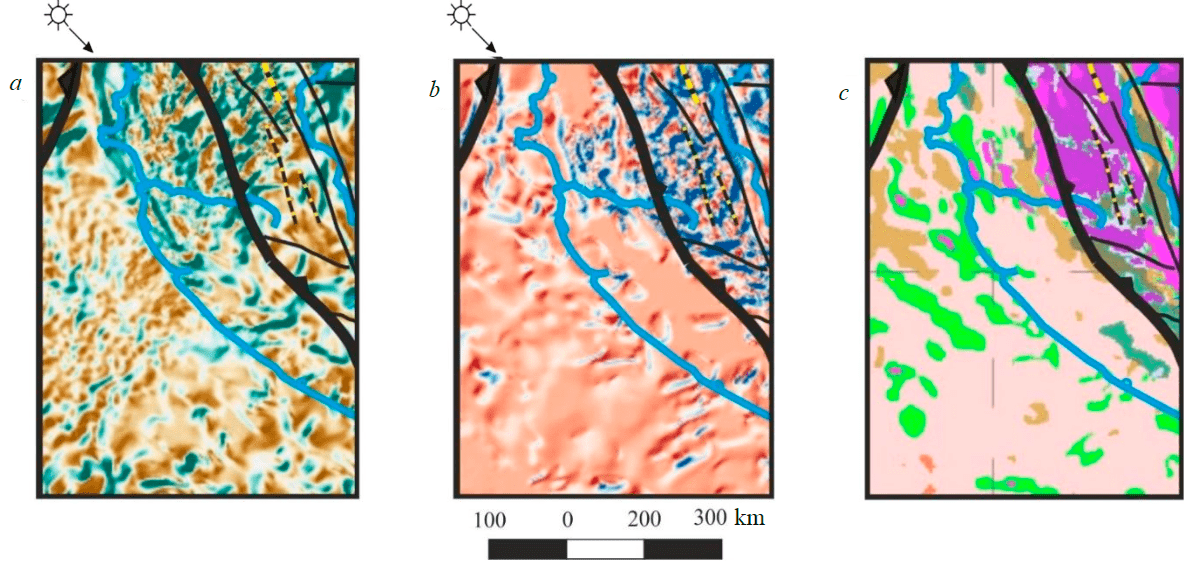
Fig.4. Manifestation of the structures of Western Kamchatka and the northern part of the Sea of Okhotsk megablock in geophysical fields: a – gravity field pseudo-relief; b – pseudo-relief of the magnetic field; c – standard-free classification of potential fields (the position of the area corresponds to inset II in Fig.1, b) See Fig.1 and 3 for class scales and symbols, respectively
The depressions within the Sea of Okhotsk block are filled with Late Miocene-Pliocene sediments. In the water area, the North Okhotsk arch, the Central Okhotsk and South Okhotsk uplifts, as well as a number of troughs (North Okhotsk, TINRO, Kashevarova, Makarova, etc.) and the Deryugin and South Okhotsk depressions are distinguished [26].
There is a well-founded opinion about the attachment of the Okhotsk Sea megablock to the margin of the Eurasian continent about 100 Ma ago. Further, it moved in a northwestern direction from the South China block along the border of the paleocontinent, forming a left-handed tectonic zone. The collision process ended in the Late Cretaceous about 77 Ma ago [30], and with Sakhalin in the Paleogene [31].
The West Kamchatka zone has a section of the earth's crust that is radically different from other structures in Kamchatka. This zone is laid on an ancient continental base. In the scientific literature several versions of the origin of this base are put forward. A number of researchers believe that this is a separate West Kamchatka microcontinental block [32-34]. Others suggest that the West Kamchatka zone is part of the Sea of Okhotsk megablock [25, 26, 35], which is supported by the authors of this article. This is evidenced by the character of the manifestation of the zone in potential geophysical fields of the same type with the northern flank of the Sea of Okhotsk block (Fig.4). As in the northern part of the Sea of Okhotsk megablock, slightly elevated gravitational and reduced magnetic fields are observed here, which confirms that its crust is of continental type. At the same time, the infrastructure of geophysical fields with elements of longitudinal zonality of individual linear gravity and magnetic anomalies indicates the presence of Alpine tectonic deformations.
Terrigenous complexes of the continental slope from the Upper Cretaceous to the Eocene are mapped on the day surface within the western flank of the zone [18, 36]. The oldest rocks here are represented by the Upper Mesozoic highly metamorphosed rocks of the median complex, consisting of granulites, amphibolites, migmatites and granites, which are exposed in the southern part of the Sredinny Ridge. The youngest phase of metamorphism and felsic granitic magmatism occurred in the Early Eocene 47-55 Ma ago [25]. These processes are associated with the obduction of the Olyutorsky island-arc complex and the oceanic crust to the margin of the West Kamchatka block. The accretion time in the south of Kamchatka belongs to the interval of 55-50 Ma [36].
Within the Sredinny massif, clastic zircons of a non-metamorphosed basement were found, the study of which gives the Archean (2900-2500 Ma), Early Proterozoic (1700-2100 Ma), Ordovician-Early Jurassic (460-175 Ma) and Late Jurassic-Early Cretaceous (150-96 Ma) age [25]. These facts also support the conclusion about the continental type of the crust of the West Kamchatka zone.
Alpine structures
The Koryak-Kamchatka accretionary-collisional active marginal area was formed in the course of collisional and active marginal orogenic processes. In its section, microcontinents with ancient continental crust, Cretaceous ophiolites, formations of Late Mesozoic and Cenozoic accretionary prisms, and island arcs are distinguished. The latter have been attached to the margin of the Eurasian continent since the Cretaceous [18]. This geostructure develops as a volcanic arc of the active continental margin associated with the subduction of the Pacific Plate along the Kamchatka Trench. Four structural zones are traditionally distinguished in the basement of Kamchatka: West Kamchatka, Olyutorskaya, Vetlovsko-Govenskaya, and Kronotskaya island-arc (see Fig.1, a). Their formation is associated with the successive attachment of the Olyutorskaya and Kronotskaya island arcs, the displacement of the subduction zone towards the Pacific Ocean, and the formation of volcanic belts and forearc troughs, which are confidently mapped on various tectonic schemes [34, 36]. These structures are contrasted in the potential fields (Fig.1, b). In the Koryak part of the folded area, the most contrasting anomalous zones correspond to the position of the suture zones. Within Kamchatka, the fields reflect a clear longitudinal zonality of the main structures.
The Olyutorskaya island-arc zone is composed of island arc formations and Upper Cretaceous–Lower Paleogene ophiolites. Island-arc formations are represented by basalts, andesites, tuffs, volcaniclastic sandstones, siltstones, flints, and jaspers. Ophiolites are represented by peridotites, gabbro, and diabases. They have a zircon age of ~76-72 Ma. Obduction of the Olyutor arc to the West Kamchatka block in the northeast direction occurred 55-50 Ma ago in the south of Kamchatka and up to ~45 Ma ago in the north [26, 36, 37]. The eastern flank of the Olyutorskaya zone structurally overlaps the Paleogene accretionary prism of the Vetlovsko-Govenskaya zone. Obducted ophiolite covers of the Olyutorskaya zone in Koryakia and Eastern Kamchatka have been noted.
The Vetlovsko-Govenskaya zone is mapped to the east of the Olyutorskaya zone. Their northwest-dipping boundary thrust consists of serpentinite tectonic melange containing ultrabasic rocks, gabbro, and sediments. The zone is composed of formations of the Paleogene accretionary prism accumulated during subduction of the oceanic lithosphere (with northwestern dip) that occurred from the middle Eocene to Pliocene [36].
The Kronotskaya island-arc zone, mapped on the eastern flank of the Kamchatka Peninsula, is traced by a set of geophysical data in the Pacific Ocean (Fig.1, b). Its linear manifestations in the magnetic and gravitational fields are very similar to those of the Kuril island arc. It is assumed that the Kronotskaya Island Arc evolved from the Late Cretaceous (~85 Ma) to the Middle Eocene (~40 Ma). Its attachment to the eastern outskirts of Kamchatka dates back to the Late Miocene. The upper age limit for the collision of the arc with the continent is estimated from Miocene-Pliocene volcanic rocks (10-5 Ma) [36-38].
Oceanic and island-arc structures
Within the northwestern part of the Pacific Ocean and the marginal basins of the Sea of Japan, the Sea of Okhotsk, and the Bering Sea, the Japanese, Kuril-Kamchatka, and Aleutian subduction zones and island arcs develop. The subduction zones are separated from the Eurasian or North American continental plates by the Aleutian, Commander, and South Kuril oceanic basins, as well as active and inactive volcanic arcs.
The Aleutian Basin with oceanic-type crust corresponds to the largest deep-water basin of the Bering Sea. Taking into account the anomalously high values of the gravitational and magnetic fields (Fig.1, b), the conclusion of the authors of “Tectonics of Asia” (2021) about the crust of the “back-arc basin” of this structure, as well as the Commander Basin, requires more detailed clarification. The structure as a whole is manifested by anomalously high values of gravitational and magnetic fields (Fig.1, b). Judging by the localization of positive magnetic anomalies against the background of a reduced gravitational field in the western marginal parts, we can assume a transitional continental-oceanic type of the Earth's crust.
The formation of the crust in the central part of the basin is associated with the spreading of the seabed, which determines the formation of strip magnetic anomalies here [39, 40]. The age estimates of these anomalies have been obtained; they range from ~128 to 138 Ma. A number of studies justified their correspondence to chrons 34 (~84 Ma) and 32 (~71 Ma). It has been established that amphibolites, metagabbro, volcanic and sedimentary rocks of the oceanic crust, ranging in age from the Upper Cretaceous to the Paleocene, underwent intense deformation [41].
These facts suggest that the upper crust of the basin margins has a somewhat different type of crust from the rest of the oceanic part of the Aleutian Basin.
The Shirshov Ridge located to the south, which is considered a remnant of the ensimatic island arc, morphologically manifests itself as an underwater ridge with a length of about 550 km. The western flank of the ridge adjoins the mainland of Asia at Cape Olyutorsky. The 40Ar/39Ar dating of island-arc rocks of the Shirshov Ridge gave an age of 65-69 Ma [42, 43].
The Commander Oceanic Basin is characterized by the development of oceanic magnetic anomalies, the age of which is estimated at intervals of 10 and 20 Ma. The age of the basalt rock from the bottom of the basin is estimated as 9.3±0.8 Ma [44].
The Aleutian Island Arc separates the Bering Sea marginal basin from the Pacific Ocean one. The Pacific Plate is dipping northward under the North American Plate, forming the classic ensimatic island arc zoning from a deep trench to a back-arc basin. The 40Ar/39Ar isochron age of the island arc is 46.3±0.9 Ma for andesites and 46.2±1.5 Ma for tholeiitic basalts of the western Aleutian Islands. The K/Ar age of the amphiboles in the amphibolites from the oceanic complex is 47±5 Ma, the age of zircons is 72±1.4 Ma. The beginning of subduction in the Aleutian zone dates back to ~50 Ma ago [45].
The Kronotskaya intra-oceanic island arc, mapped on the eastern flank of the Kamchatka Peninsula and its coastal oceanic zone, began to form ~85 Ma ago. Its collision with Kamchatka dates back to ~10-5 Ma [26, 36]. The boundaries of this structure in the coastal zone of Kamchatka are drawn according to the features of manifestation in potential geophysical fields: intense positive linear anomalies of the gravitational and magnetic fields against the background of an area of increased values of the magnetic and reduced gravitational fields (Fig.1, b).
The Kuril Island Arc, which formation was caused by the subduction of the Pacific Plate under the Sea of Okhotsk megablock of the Eurasian continent, stretches from Kamchatka to the Japanese Islands. This arc developed from the Late Oligocene to the Early Miocene. The oldest igneous rocks date back to the early Oligocene [45, 46]. The results of dredging of underwater ridge rocks indicate that the modern Kuril arc was built on the crust of the paleo-arc of East Sakhalin-Nemuro. The development area of the island arc is outlined by the same features of manifestation in potential fields as the Kronotskaya island arc (Fig.1, b).
Profile 2-DV-M crosses the island arc in the area of Urup Island (Fig.2, survey markers 1500-1700). The seismic section is characterized by a complex structure of numerous seismic boundaries undergoing deformations and faults, and a large spread of velocities. Its thickness is 23 km, increasing on the Vityaz Ridge to 25 km. The section is dominated by the lower crustal layer up to 13-15 km thick with a longitudinal wave velocity of 6.5-7.1 km/s and a density of 2.75-3.05 g/cm3. The upper crustal layer is 2-6 km thick with a density of 2.65-2.85 g/cm3 at a velocity of 3.3-6.4 km/s. Above it, weakly lithified Meso-Cenozoic sedimentary-volcanogenic complexes (velocity 3.3-5.2 km, density 2.40-2.60 g/cm3) and Cenozoic loose sediments with P-wave velocities of 2.3-2.7 km /s and densities of about 2.0 g/cm3[47]. According to these data, it can be concluded that the section of the earth's crust is of a continental type.
The Kuril ensialic island arc borders the Yuzhno-Okhotsk Basin in the west along a system of complex normal faults with a strike-slip component. Its eastern boundary is the Kuril-Kamchatka deep-sea trench.
The South Okhotsk Basin, 750 km long and 300 km wide, was formed 23 to 9 Ma ago. According to the studies of I.P.Kosminskaya and her followers, it is characterized by an oceanic (suboceanic, meaning close to oceanic) type of the earth's crust. The basin is located in the back-arc region of the Kuril Island Arc in the southern part of the Sea of Okhotsk. Multicomponent measurements carried out in the basin using bottom seismographs made it possible to use converted waves to estimate the velocity characteristics of the layers of the earth's crust. It was calculated that the velocity values in the upper consolidated crust of the basin (Vp = 5.50-5.80 km/s, Vp/Vs = 1.74-1.76 km/s) correspond to the characteristics of the granite-metamorphic layer of the continental crust [48]. A small thickness (2.5-3.5 km) of the layer may indicate the formation of a structure in the geodynamic setting of a typical back-arc rifting on the eastern flank of the continental megablock of the Sea of Okhotsk.
These data were the basis for the conclusion that the crust is close in its parameters to the upper continental crust. Despite the reduced thickness of the consolidated part, the Earth's crust of this structure retains a thin upper crust. The deep section along the 2-DV-M profile shows the development of a thin (up to 10 km) crystalline crust overlain by a Cenozoic mantle up to 3-5 km thick. The deep part of the section is penetrated by a series of sub-vertical zones of reduced reflectivity, which may manifest heat and mass transfer channels (Fig. 2).
A similar type of the “back-arc basin” crust, substantiated by a wide range of deep seismic studies, gravity, and magnetic data, heat flow, and seismology, was also determined for the Sea of Japan [44].
The Kuril-Kamchatka deep-water trench at the border of the island arc with the Pacific Plate is localized at a depth of more than 10 km. Its width varies from 50 to 100 km, and the inclination of the sides of the trench is estimated at an average of 7°. The slopes of the trench are complicated by faults, tectonic ledges, and steps [47]. The thickness of precipitation in the area of the Urup Island is 2 km. The Moho boundary is mapped at depths of 13-18 km.
In 1946, A.N.Zavaritsky established the existence of a focal zone of earthquake sources, falling towards the continent at an angle of about 40° to a depth of 700 km. Where the focal zone approaches the Earth's surface at a distance of 100-150 km, an island arc develops. In the zone of the Pacific plate oceanic crust dipping into the mantle, elements of an accretionary prism have been formed.
The Sakhalin-Sikhote-Alin collisional-active marginal folded area was formed as a result of the late Mesozoic accretion of the Pacific oceanic plate under the Sakhalin margin of Eurasia (see Fig.1). In the course of subduction, the island arc joins and the Cenozoic active continental margin develops. During this period, the Late Cretaceous-Paleogene volcano-plutonic belt was formed in the Sikhote-Alin. The manifestation of tension stress in the rear zone of the active margin is associated with the initiation of the rift of the Tatar Strait, which split the Anyui continental plate into the western (Sikhote-Alin) and eastern (Sakhalin) parts.
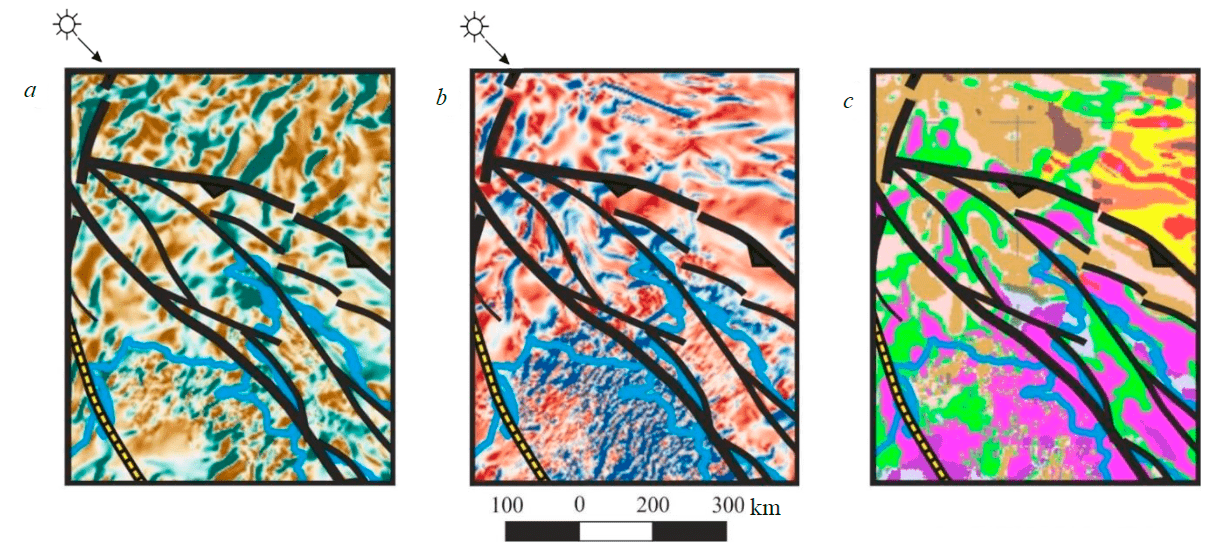
Fig.5. Manifestation of the structures of the North Sakhalin - Sikhote-Alin collision-active marginal folded area in geophysical fields: a – gravity field pseudo-relief; b – pseudo-relief of the magnetic field; c – non-standard classification of potential fields (the position of the area corresponds to inset III in Fig.1, b) See Fig.1 and 3 for class scales and symbols, respectively
The Central Sikhote-Alin block with continental-type crust is characterized by a two-layer structure of the crystalline basement. The most ancient complexes of metamorphic rocks are exposed within the Khor and Anyui ledges. In the north, in the Baladek ledge, Archean anorthosites and Proterozoic granite-gneisses are mapped. The lower volcanic-sedimentary layer has velocity parameters indicating its volcanic composition. The structure of the upper stratigraphic levels of the Earth's crust is due to tectonic and magmatic processes on the Late Cretaceous-Paleogene active margin of Hokkaido and Sakhalin [49].
The East Sikhote-Alin volcanic-plutonic belt was formed in the middle of the Late Cretaceous. It is composed of thick (up to 6 km) felsic and intermediate effusive rocks with a wide development of ignimbrites in the Paleogene, but in the Neogene and Early Pleistocene, they were replaced by andesite-basalts and basalts. Intrusions from gabbro and diorites to granites and alkaline granites are closely associated with volcanic rocks. The belt is characterized by positive linear anomalies of the gravitational and magnetic fields against the background of isometric positive anomalies of the magnetic field and reduced values of the gravitational field (Fig.5). All Cretaceous and Paleogene volcanic rocks are folded into simple brachymorphic folds, and only Neogene-Quaternary basalts lie horizontally.
The Tatar zone is an intracontinental rift structure about 50 km wide, overlain by a thick (up to 8-10 km) Mesozoic-Cenozoic sediments formation. The rift is separated from the adjacent structures of the Sikhote-Alin and Western Sakhalin by deep faults. The throw and thrust fault dislocations of the eastern flank of the rift are steeply inclined (up to 50-80°) to the west. In the basement, according to seismic data, a granite metamorphic layer is modeled with a longitudinal wave velocity of 5.8-6.2 km/s. An increased heat flow and a reduced velocity of longitudinal waves along the Moho surface (7.4-7.6 km/s) correspond to a radical reduction in the crust thickness. The rift is the northern continuation of the spreading center located in the deep-water basin of the Sea of Japan. It is believed that spreading processes, accompanied by eruptions of basaltic lavas, continued 25-15 Ma ago [30]. From the middle of the Oligocene to the Miocene in the Tatar Strait, the earth's crust was stretched as a result of rifting, accompanied by the processes of basalt volcanism.
As a whole, Sakhalin is characterized by anomalously high values of the magnetic field at low values of the gravitational field, which confirms the conclusion about the continental type of the section of the Earth's crust with obstruction of ophiolites, island arc complexes, and accretionary prisms under conditions of collision with the margin of Eurasia. Field analysis shows that the southwestern part of the Sea of Okhotsk plate is deformed by Alpine folding and should be included in the studied folded area.
The West Sakhalin zone is considered as the northern flank of the belt of central Hokkaido. The occurrence of accretionary wedge formations here is associated with subduction of the oceanic plate beneath the Asian margin. The Aptian-Paleocene turbidite strata of the West Sakhalin Belt correlate with forearc deposits of the Sorachi-Ezo Belt [50].
The zone of the Merey ophiolite suture of South Sakhalin is considered as the northern continuation of the Idonnappu zone and the Sorachi-Ezo belt of Hokkaido Island. The Sorachi-Ezo Belt consists of Middle Jurassic and Cretaceous accretionary complexes, ophiolites, and metamorphic rocks unconformably overlain by Cretaceous to Paleocene marine sediments. Ophiolites and accretionary complexes of the Idonnappu suture zone form the eastern margin of the Sorachi-Ezo belt. In the northern direction, this suture zone, according to geological and geophysical data, can be traced to the ophiolite complex of the Schmidt Peninsula in the far north of Sakhalin.
The East Sakhalin zone, which is a northward continuation of the Hidaki zone of Hokkaido Island, is made up of rocks of the accretionary complex (from the Middle Cretaceous to the Paleocene), metamorphic rocks, and ophiolite mélange with tectonic layers of Permian-Late Cretaceous rocks [17, 50]. Their current position is associated with the westward obduction of the East Sakhalin island arc.
The East Sakhalin – Nemuro (Hokkaido) zone was established along the eastern flank of the East Sakhalin zone. On the Tonino-Anivsky and Terpeniya peninsulas, formations of the Upper Cretaceous-Paleogene intra-oceanic island arc are mapped [50]. It is separated from Eurasia by a regional shift.
The East Sakhalin trough was formed as a result of the collision of the East Sakhalin island arc with the Sea of Okhotsk Plate 50-38 Ma ago. It is assumed that as a result of the Paleogene accretion of the Sea of Okhotsk geoblock and Sakhalin, structures of northeastern Japan and Sakhalin inclined to the west were formed. A characteristic feature of the outer zones is their location on the same continental crust as the basement of the adjacent platform.
Disjunctive dislocations
The main tectonic dislocations mark the boundaries of folded regions of different ages in the region. In most cases, they have thrust kinematics, for example, the northern and western boundaries of the Koryak-Kamchatka folded area with the Cimmerian structures of the Kolyma-Omolon folded area and the Sea of Okhotsk megablock. The thrust character of edge dislocations is assumed for the eastern flank of the Okhotsk-Chukotka and northern flanks of the Sakhalin-Sikhote-Alin folded regions. Thrust faults are modeled at the boundaries of suture zones and blocks of almost all folded regions of the area.
The region under consideration is characterized by a wide manifestation of shear dislocations. The most contrasting regional strike-slip boundary is the Arctic-Asian seismic belt, which extends from the Gakkel Ridge into the Laptev Sea through the Chersky Ridge, the Sea of Okhotsk, and the Kamchatka Peninsula towards the Aleutian subduction zone. This left-lateral strike-slip structure is considered as the boundary between the North American and Eurasian lithospheric plates. Its occurrence and isolation dates back to the beginning of the Cenozoic and is due to the opening of the Eurasian Basin. In the study area (see Fig.1), the zone is traced along the boundary of the Kolyma-Omolon and Verkhoyansk-Kolyma folded regions as a system of subparallel linear dislocations, which are contrastingly manifested in geophysical fields and modern relief. Further, against the background of submeridional structures of the Okhotsk-Chukotka and Koryak-Kamchatka folded regions, the dislocation zones are traced up to the Aleutian deep-water trench.
The southern branch of the Arctic-Asian strike-slip zone presumably intersects the area of tectonic deformations of the western flank of the Okhotsk-Chukotka volcanic belt and closes on the Okhotsk-Chukotka regional left-hand strike-slip. The latter can be traced within the continent (Murgal and South Taigonos zones) and the Sea of Okhotsk (Tauy zone).
The Okhotsk-Chukotka regional left-hand strike-slip, which develops along the western flank of the Okhotsk-Chukotka suture zone, is confidently traced by a set of geophysical methods by changing the infrastructure of the gravitational and magnetic fields (Fig.1, 3). The northern flank of the Mongol-Okhotsk suture is characterized by the development of left-handed strike-slip dislocations, which have a neotectonic manifestation.
The Central Sikhote-Alin strike-slip is interpreted as a regional left-hand strike-slip shifting the Amur structure by 200-250 km. In the lateral dimension, the system of feathering dislocations reaches a width of 100 km and is well manifested in geophysical fields.
The Sakhalin-Hokkaido dextral strike-slip zones were formed after the obduction of the East Sakhalin-Nemuro island arc in the Late Eocene. In parallel, the Tym-Poronai fault develops, crossing from north to south the central part of Sakhalin, the Tatar Strait, and the western part of the Sea of Okhotsk. According to Fournier et al. (1994), right-hand displacements that developed from the Miocene along shear faults in the East Sakhalin Mountains reached at least 50 km.
Conclusions
The Sea of Okhotsk region is a transition zone from the Eurasian continent to the Pacific margin. Within its limits, structures of Cimmerian and Alpine age are distinguished. Based on potential field data using various recalculation algorithms, geological and seismic materials, a new geotectonic model was developed that reflects the features of the deep structure, tectonics and geodynamics of the Sea of Okhotsk region and its framing. The key elements of the presented model are blocks with an ancient continental base and interblock zones filled with complexes of island arcs, an accretionary prism, and oceanic crust.
The central structure of the region is the Sea of Okhotsk megablock with continental-type crust. On its northwestern flank, the Okhotsk-Chukotka suture zone was first delineated. The development of the regional Late Cretaceous Okhotsk-Chukotka strike-slip fault and the wide distribution of feathering dislocations led to the formation of extension and compression structures (grabens and horsts) and related sedimentary Cenozoic basins.
The developed geological and structural scheme of the region is considered as the basis for creating maps of tectonic and oil and gas geological zoning and performing a formalized forecast at the regional level.
References
- Alekseev V.I. Deep structure and geodynamic conditions of granitoid magmatism in the Eastern Russia. Journal of Mining Institute. 2020. Vol. 243, p. 259 (in Russian). DOI: 10.31897/PMI.2020.3.259
- Ivolga E.G., Manilov Y.F. Structure Of The Lithosphere In The Zone Of The Continent–Ocean Transition In The Southern Part Of The Russian Far East: Evidence From The Density Modeling. Russian Journal of Pacific Geology. 2019. Vol. 13. N 6, p. 493-509. DOI: 10.1134/S1819714019060034
- Tectonic map of the Sea of Okhotsk region. Scale 1:2500000. Explanatory note. Мoscow: Federalnaya sluzhba geodezii i kartografii Rossii, 2000, p. 193 (in Russian).
- Seton M., Müller R.D., Zahirovic S. et al. Global continental and ocean basin reconstructions since 200 Ma. Earth-Science Reviews. 2012. Vol. 113. Iss. 3-4, p. 212-270. DOI: 10.1016/j.earscirev.2012.03.002
- Vaes B., van Hinsbergen D.J.J., Boschman L.M. Reconstruction of subduction and back‐arc spreading in the NW Pacific and Aleutian Basin: Clues to causes of Cretaceous and Eocene plate reorganizations. Tectonics. 2019. Vol. 38. Iss. 4, p. 1-47. DOI: 10.1029/2018TC005164
- Yong-Tai Yang. An unrecognized major collision of the Okhotomorsk Block with East Asia during the Late Cretaceous, constraints on the plate reorganization of the Northwest Pacific. Earth-Science Reviews. 2013. Vol. 126, p. 96-115. DOI: 10.1016/j.earscirev.2013.07.010
- Movchan I., Yakovleva A., Movchan A., Shaygallyamova Z. Early assessment of seismic hazard in terms of Voronezh massif-Moscow Depression contact. Mining of Mineral Deposits. 2021. Vol. 15, p. 62-70. DOI: 10.33271/mining15.03.062
- Alekseev V.I., Marin Yu.B. A Unique Find of Rhenium-bearing Wolframite in Ongonites of the Russian Far East. Doklady Earth Sciences. 2018. Vol. 478. Iss. 2, p. 250-252. DOI: 10.1134/S1028334X18020216
- Talovina I.V., Krikun N.S., Yurchenko Yu.Yu., Ageev A.S. Remote sensing techniques in the study of structural and geotectonic features of Iturup Island (the Kuril Islands). Journal of Mining Institute. 2022. Vol. 254, p. 158-172 (in Russian). DOI: 10.31897/PMI.2022.45
- Telegin A.N. Possibilities of seismic exploration for crystalline basement study. Journal of Mining Institute. 2017. Vol. 223, p. 30-36. DOI: 10.18454/PMI.2017.1.30
- Movchan I.B., Yakovleva A.A. Experience of qualitative and quantitative interpretation of nonpotential geofields with surface and deep morphostructural reconstructions on the example of unica ore province (Kareljya, Russia). International Journal of Mechanical Engineering and Technology. 2017. Vol. 8. Iss. 12, p. 926-932.
- Grigorev G.S., Salishchev M.V., Senchina N.P. On the applicability of electromagnetic monitoring of hydraulic fracturing. Journal of Mining Institute. 2021. Vol. 250, p. 492-500 (in Russian). DOI: 10.31897/PMI.2021.4.2
- Pavlenkova N.I., Kashubin S.N., Gontovaya L.I., Pavlenkovа G.A. Deep structure and geodynamics of the Sea of Okhotsk region. Regional Geology and Metallogeny. 2018. N 76, p. 70-82 (in Russian).
- Sakulina T.S., Kalenich A.P., Atakov A.I. et al. Geological model of the okhotsk sea region according to basic profiles 1-OM AND 2-DW-M. Prospect and protection of mineral resources. 2011. N 4, p. 36-43 (in Russian).
- Egorov A.S., Vinokurov I.Yu., Telegin A.N. Scientific and Methodical Approaches to Increase Prospecting Efficiency of the Russian Arctic Shelf State Geological Mapping. Journal of Mining Institute. 2018. Vol. 233, p. 447-458 (in Russian). DOI: 10.31897/PMI.2018.5.447
- Egorov A.S., Prischepa O.M., Nefedov Y.V. et al. Deep Structure, Tectonics and Petroleum Potential of the Western Sector of the Russian Arctic. Journal of Marine Science and Engeenering. 2021. Vol. 9. Iss. 3. N258. DOI: 10.3390/jmse9030258
- Movchan I.B., Yakovleva A.A. Wave analogies in the quantitative interpretation of potential fields. International Journal of Advanced Trends in Computer Science and Engineering. 2020. Vol. 9. N2, p. 1793-1799. DOI: 10.30534/ijatcse/2020/136922020
- Map of tectonic zoning of Russia. Scale 1:5000000. Brief explanatory note. Мoscow: Geokart, 2000 (in Russian).
- Khain V.E. Large-scale cyclicity in the tectonic history of the Earth and its possible causes. Geotektonika. 2000. N 6, p. 3-18 (in Russian).
- Khudolei A.K., Reinbird R., Stern R. et al. New data on the Riphean tectogenesis in the North-East of Russia. Tectonics, geodynamics and processes of magmatism and metamorphism. Materials of the XXXII Tectonic Meeting, February 2-5, 1991, Мoscow, Russia. Vol. II. Geos, 1999, p. 261-264.
- Imaev V.S., Imaeva L.P., Koz'min B.M. Geodynamic Features of the Seismotectonic Structures in the Central Part of the Chersky Zone (North-East of Russia). Arctic and Subarctic Natural Resources. 2019. Vol. 24, N 2, p. 5-20 (in Russian). DOI: 10.31242/2618-9712-2019-24-2-1
- Kashubin S.N., Petrov O.V., Shokalsky S.P. et al. Deep crustal structure in northeastern eurasia and its continental margins. Geodynamics & Tectonophysics. 2021. Vol. 12. N 2, p. 199-224. (in Russian). DOI: 10.5800/GT-2021-12-2-0521
- Ageev A., Egorov A., Krikun N. The principal characterized features of earth’s crust within regional strike-slip zones. Advances in Raw Material Industries for Sustainable Development Goals. London: CRC Press, 2020, p. 78-83.
- Piskarev A.L., Butsenko V.V., Poselov V.A., Savin V.A. Deep Structure of the Crust Beneath the Sea of Okhotsk Inferred From 3D Seismic Density Modeling. Oceanology. 2012. Vol. 52. N 3, p. 411-421. DOI: 10.1134/S0001437012030095
- Bindeman I.N., Vinogradov V.I., Valley J.W. et al. Archean protolith and accretion of crust in Kamchatka: SHRIMP dating of zircons from Sredinny and Ganal Massifs. The Journal of Geology. 2002. Vol. 110. N 3, p. 271-289. DOI: 10.1086/339532
- Konstantinovskaya E., Brown D., Ryan P. Early Eocene Arc–Continent Collision in Kamchatka, Russia: Structural Evolution and Geodynamic Model. Frontiers in Earth Sciences. 2011. Vol. 4, p. 247-277. DOI: 10.1007/978-3-540-88558-0_9
- Kharakhinov V.V. Sedimentary (oil and gas bearing and potentially oil and gas bearing) systems of the Sea of Okhotsk region: geodynamic arrangement. Geologiya nefti i gaza. 2020. N 1, p. 81-99 (in Russian). DOI: 10.31087/0016-7894-2020-1-81-99
- Lelikov E.P., Pugachev A.A. Granitoid Magmatism of the Japan and Okhotsk Seas. Petrology. 2016. Vol. 24. N 2, p. 196-213. DOI: 10.1134/S0869591116020053
- Parfenov L.M., Natal'in B.A. Mesozoic tectonic evolution of northeastern Asia. Tectonophysics. 1986. Vol. 127. Iss. 3-4, p. 291-304. DOI: 10.1016/0040-1951(86)90066-1
- Pan Zhao, Alexandrov I., Jahn B.M., Ivin V. Timing of Okhotsk sea plate collision with Eurasia plate: Zircon U‐Pb age constraints from the Sakhalin Island, Russian Far East. Journal of Geophysical Research: Solid Earth. 2018. Vol. 123. Iss. 9, p. 8279-8293. DOI: 10.1029/2018JB015800
- Maruyama S., Isozaki Y., Kimura G., Terabayash M. Paleogeographic maps of the Japanese Islands: plate tectonic synthesis from 750 Ma to the present. Island Arc. 1997. Vol. 6. Iss. 1, p 121-142. DOI: 10.1111/j.1440-1738.1997.tb00043.x
- Bogdanov N.A., Chekhovich V.D. On the collision of the West Kamchatka and Sea of Okhotsk plates. Geotektonika. 2002. N 1, p. 72 (in Russian).
- Filatova N.I. New data on the tectonic position of the Mesozoic rocks of Western Kamchatka in the structures of the Middle Cretaceous orogenic belt of East Asia. Doklady Earth Sciences. 2014. Vol. 455. N 5, p. 556-561 (in Russian). DOI: 10.7868/S0869565214110164
- Chekhovich V.D., Sukhov A.N., Palandzhyan S.A., Kononov M.V. Geodynamics of the Northwestern Sector of the Pacific Mobile Belt in the Late Cretaceous-Early Paleogene. Geotectonics. 2009. Vol. 43. N 4, p. 283-304. DOI: 10.1134/S0016852109040049
- Parfenov L.M., Badarch G., Berzin N.A. et al. Summary of Northeast Asia geodynamics and tectonics. Stephan Mueller Special Publication Series. Vol. 4, p. 11-33. DOI: 10.5194/smsps-4-11-2009
- Shapiro M.N., Solov'ev A.V. Formation of the Olyutorsky-Kamchatka Foldbelt: a Kinematic Model. Russian Geology and Geophysics. 2009. Vol. 50. N 8, p. 668-681. DOI: 10.1016/j.rgg.2008.10.006
- Tsukanov N.V., Palechek T.N., Soloviev A.V., Savelyev D.P. Tectonostratigraphic Complexes Of the Southern Kronotskii Paleoarc (Eastern Kamchatka): Structure, Age, And Composition. Russian Journal of Pacific Geology. 2014. Vol. 8. N 4, p. 233-246. DOI: 10.1134/S1819714014040083
- Silaev V.I., Karpov G.A., Anikin L.P. Mineral Phase Paragenesis in Explosive Ejecta Discharged by Recent Eruptions in Kamchatka and the Kuril Islands. Part 1. Diamonds, Carbonaceous Phases, and Condensed Organoids. Journal of Volcanology and Seismology. 2019. Vol. 13, p. 323-334. DOI: 10.1134/S0742046319050051
- Cooper A.K., Marlow M.S., Scholl D.W., Stevenson A.J. Evidence for Сenozoic crustal extension in the Bering Sea region. Tectonics. 1992. Vol. 11. Iss. 4, p. 719-731. DOI: 10.1029/92TC00214
- Scholl D.W. Viewing the tectonic evolution of the Kamchatka-Aleutian (KAT) connection with an Alaska crustal extrusion perspective. Volcanism and Subduction: The Kamchatka Region. 2007. Vol. 172, p. 3-35. DOI: 10.1029/172GM03
- Ogg J.G. Geomagnetic polarity timescale. Chapter 5. The geologic timescale. 2020, p. 159-192. DOI: 10.1016/B978-0-12-824360-2.00005-X
- Grebennikov A.V., Khanchuk A.I. Geodynamics and magmatism of Pacific-type transform margins: main theoretical aspects and discriminant diagrams. Tikhookeanskaya geologiya. 2021. Vol. 40. N 1, p. 3-24 (in Russian). DOI: 10.30911/0207-4028-2021-40-1-3-24
- Sukhov A.N., Chekhovich V.D., Lander A.V. et al. Age of the Shirshov Submarine Ridge Basement (Bering Sea) based on the Results of Investigation of Zircons using the U-Pb Shrimp Method. Doklady Earth Sciences. 2011. Vol. 439. N 1, p. 926-932. DOI: 10.1134/S1028334X11070178
- Layer P., Scholl D., Newberry R. Ages of igneous basement from the Komandorsky Islands, FarWestern Aleutian Ridge. AGU Fall Meeting Abstracts. 2007, p. 44-52.
- Schellart W.P., Jessell M.W., Lister G.S. Asymmetric deformation in the backarc region of the Kuril arc, northwest Pacific: New insights from analogue modeling. Tectonics. 2003. Vol. 22. Iss. 5, p. 1047-1064. DOI: 10.1029/2002TC001473
- Martynov A.Y., Martynov Y.A. Pleistocene Basaltic Volcanism Of Kunashir Island (Kuril Island Arc): Mineralogy, Geochemistry, And Results Of Computer Simulation. Petrology. 2017. Vol. 25. N 2, p. 206-225. DOI: 10.1134/S0869591117020035
- Proshkina Z.N., Kulinich R.G., Valitov M.G. Structure, Matter Composition, and Deep Structure of the Oceanic Slope of the Central Kuril Islands: New Evidence. Russian Journal of Pacific Geology. 2017. Vol. 11. N 6, p. 436-446. DOI: 10.1134/S1819714017060045
- Kashubin S.N., Petrov O.V., Rybalka A.V. et al. Earth's crust model of the South-Okhotsk Basin by wide-angle OBS data. Tectonophysics. 2017. Vol. 710-711, p. 37-55. DOI: 10.1016/j.tecto.2016.11.021
- Kemkin I.V., Khanchuk A.I., Kemkina R.A. Accretionary prisms of the Sikhote-Alin orogenic belt: composition, structure and significance for reconstruction of the geodynamic evolution of the Eastern Asian margin. Journal of Geodynamics. 2016. Vol. 102, p. 202-230. DOI: 10.1016/j.jog.2016.10.002
- Zharov A.E., Bragin N.Yu., Rechkin A.N. The Cretaceous-Lower Paleogene Stratigraphy Of Accretionary Rock Complexes In The Tonino-Aniva Peninsula Of Southern Sakhalin. Stratigraphy and Geological Correlation. 2005. Vol. 13. N 1, p. 80-98.