Iron ore beneficiation technologies in Russia and ways to improve their efficiency
- Ph.D., Dr.Sci. Associate Professor Ural State Mining University ▪ Orcid
Abstract
Increasing the efficiency of crushing circuits is associated with a decrease in the particle size of finely crushed ore and the use of dry magnetic separation of crushed ore. Reducing grinding costs is achieved by using drum mills jointly with mills of other designs. The use of automation systems, slurry demagnetization, technologies with staged concentrate separation, and beneficiation and fine screening in a closed grinding cycle lead to a reduction in grinding costs. The main industrial technology for improving the quality of concentrate is its additional beneficiation using regrinding, fine screening, flotation, and magnetic-gravity separators. Increasing the integrated use of iron ore raw materials is associated with an increase in the yield of iron concentrate and the production of hematite concentrate during the beneficiation of hematite-magnetite ores and ilmenite concentrate during the beneficiation of titanomagnetite ores. Incremented concentrate yield is possible by using magnetic separators with an increased magnetic induction up to 0.25-0.5 T in the first stages of beneficiation. To obtain hematite and ilmenite concentrates, combined technologies can be used, including fine screening, high-gradient magnetic, gravity, flotation, and electrical separation.
Introduction
In Russia, three commercial types of ores are mainly mined and beneficiated: magnetite (ferruginous) quartzites; skarn magnetite; titanomagnetite ores. Iron ores are divided into commercial types according to the genetic type of deposits and material composition.
Magnetite quartzites have an increased content of Fe (more than 25 %) and SiO2 (more than 35 %). The latter leads to an increased content of SiO2 (more than 5-6 %) in concentrates, which reduces their metallurgical value, despite the higher content of Fe in concentrates (65-67 %) compared to those obtained from other ores. Ferruginous quartzites are characterized by a large amount of hematite in the ore. The beneficiation circuit includes mainly three stages of grinding. Self-grinding is used at the Lebedinsky Mining and Beneficiation Plant (GOK). Desludging is always used due to increased sludge formation during grinding. Increasing the integrated use of iron ore raw materials is especially important in the beneficiation of ferruginous quartzites. It is associated with the production of a second iron (hematite) concentrate.
Skarn magnetite ores have a decreased content of SiO2 (less than 30-35 %) and an increased content of Fe (more than 20 %). Concentrates (62-66 % Fe) are characterized by a low content of SiO2 (less than 6 %), which increases their metallurgical value. With a significant amount of other useful minerals (not Fe minerals), they are extracted into separate products, which increases the integrated use of iron ore raw materials. For example, Kovdorsky GOK produces apatite and baddeleyite concentrates. It is possible to obtain copper concentrate (Vysokogorsky GOK). The beneficiation circuit is simple; desludging is generally not used. Circuits with two stages of grinding can be applied. Crushed stone is produced from dry magnetic separation (DMS) tailings.
Titanomagnetite ores have a decreased content of Fe (15.5-16 %) and SiO2 (less than 35 %) and an increased content of TiO2 and V2O5 [1]. Concentrates (61.2-62.3 %), sinter, and pellets are characterized by low content of SiO2 (less than 4-5 %) and high content of V2O5, which increases their metallurgical value. Titanium contained in concentrates (2.5-3.0 % TiO2) should be classified as a detrimental impurity. Its significant amount leads to a violation of the removal of slag from the blast furnace, since titanium is a refractory element. Vanadium is extracted from concentrate processing products using pyrometallurgical and hydrometallurgical processes. The beneficiation circuit is simple, with three stages of grinding; desludging is not used. Crushed stone is produced from DMS tailings (Fig.1).
Iron ores contain minerals with detrimental impurities S, P, Cu, As, Zn, Pb, Na2O, and K2O. Basically, these minerals are removed to the tailings (except for highly magnetic pyrrhotite) and do not reduce the metallurgical value of iron concentrate.
The main economic mineral from which steel is obtained in Russia is magnetite and its variety titanomagnetite with isomorphic impurities of titanium and vanadium. Magnetite is a highly magnetic mineral, so the main method of its beneficiation is magnetic separation in a weak field with induction from 0.16 to 0.25-0.5 T [2, 3]. Other beneficiation methods can be used: flotation and gravity separation [4, 5], as well as fine hydraulic screening [6, 7], in which the undersize product is characterized by an increased iron content.
The work is designated to analyse and classify the main technical and engineering solutions aimed at improving the efficiency of iron ore beneficiation circuits, and their possible implementation using domestic equipment. Many technologies are implemented at industrial enterprises.
Methodology
The study target is the technology of iron ores beneficiation and ways to improve its efficiency. Research methods were the analysis and generalization of information obtained from literary scientific sources and by performing experiments in industrial and laboratory conditions. The results were obtained mainly during the beneficiation of titanomagnetite ore from the Gusevogorskoye deposit. Standard beneficiation circuits for the main types of iron ores do not fundamentally differ. The difference lies in the use of certain technologies, such as improving the concentrate quality (for ferruginous quartzites) or the use of slurry demagnetization (mainly for skarn and titanomagnetite ores). Therefore, the main part of technical and engineering solutions is applicable to all types of iron ores.
The test results were obtained using industrial (Kachkanarsky GOK, average sampling results) and laboratory equipment (samples taken in 2004-2021 were studied). The latter was used for crushing, grinding, granulometric analysis, magnetic (in weak, strong, and variable magnetic fields), gravity (screw separation and table concentration), and electrical separation.
Standard circuit of magnetite ores beneficiation
Beneficiation of all iron ores is carried out according to the general circuit, including several stages of grinding and separation of a non-magnetic product (tailings) using wet magnetic separation (WMS) after each stage of grinding (Fig.1). This reduces the load on the process equipment from the first to the last operation in the circuit.
Most combines use finely crushed ore (less than 25 mm) DMS to separate some of the gangue into tailings prior to the most energy-intensive grinding operations. As a rule, crushed stone is produced from the non-magnetic DMS product after size classification, which is an additional commercial product of GOK. At some combines, DMS is used with an ore particle size of –300+0 mm after the first stage of crushing (Kostomukshsky and Kimkano-Sutarsky GOK) or with a particle size of –80+0 mm after the second stage of crushing (Goroblagodatskoye Mining Administration).
Performance indicators of magnetite ores beneficiation are determined by the material composition of the ore from a particular deposit and magnetite content in the ore. The concentrate yield directly depends on magnetite content in the ore. Magnetite concentrate is obtained after the last stage of grinding and final WMS operations. Iron content in the concentrate is determined mainly by the content of –0.071 (–0.045) mm class in it. The final particle size of middlings grinding before the last WMS stage is from 70-80 (–0.071 mm class) to 95-98 % (–0.045 mm class). Most GOKs apply three stages of grinding to obtain the required quality of the concentrate due to the greater opening of magnetite grains at a smaller concentrate particle size. The required size of the concentrate particle is also determined by its further metallurgical processing. If pellets are produced from the concentrate, then its size should be at least 92 % of the –0.071 mm class. In the production of sinter, the concentrate particle size may be higher.
Iron content in the concentrate directly depends on iron content in magnetite and the grain size of magnetite. Iron extraction into concentrate depends on the ratio of magnetite iron to total (Femagn/Fe), which varies from 0.5 to 0.85 at the developed deposits in Russia. The higher the values of Femagn and Femagn/Fe in the ore, the higher the performance indicators of iron concentrate production.
The main beneficiation machines at the plants are drum magnetic separators for dry and wet beneficiation (PBM). With an increased formation of sludge during ore grinding, magnetic slug catchers are used. For crushing, grinding, and dewatering, standard equipment applied in the beneficiation of ores of other metallic and non-metallic minerals is used. If magnetite from a particular deposit is characterized by an increased coercive force, then demagnetization is applied before classification, fine screening, and filtration.
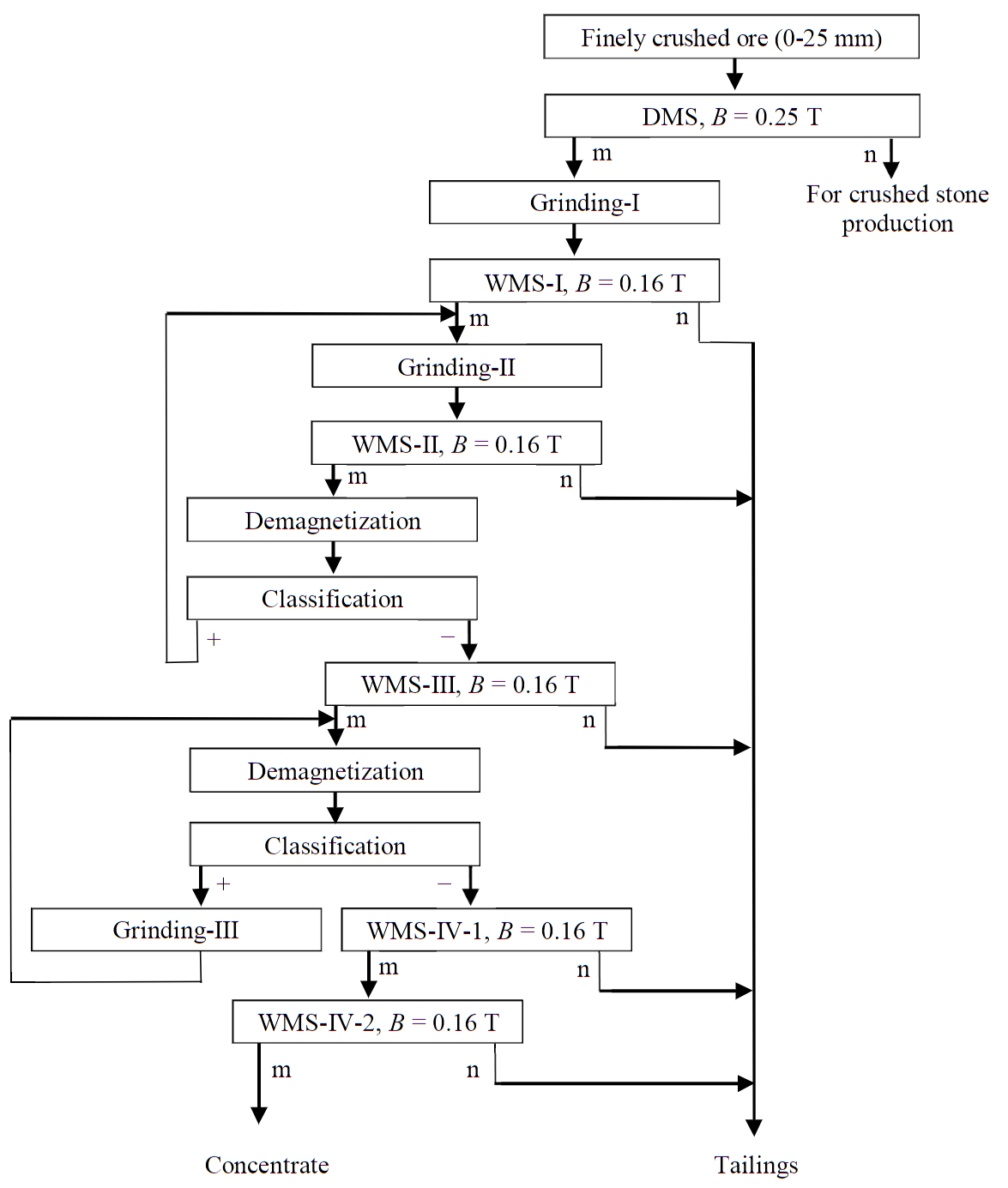
Fig.1. Beneficiation of titanomagnetite ore at the Kachkanarsky GOK (m – magnetic product; n – non-magnetic product)
Discussion of the results
Increasing the efficiency of crushing circuits is associated mainly with two directions: with a decrease in the cost of ore crushing and a decrease in the particle size of finely crushed ore. Both directions contradict each other, since a decrease in the particle size of finely crushed ore always leads to an increase in the unit cost of crushing the ore. The reduction in crushing costs (without reducing the particle size of finely crushed ore) is mainly due to the use of more productive crushers and a wider use of DMS after the first and second crushing stages. Removal of part of the rocks using DMS reduces the load on subsequent crushing operations.
Specific power consumption for crushing and grinding 1 ton of titanomagnetite ore (Kachkanarsky GOK, data of 2012), kWh/t: crushing (stage I) – 0.195, crushing and screening (stages II-III) – 0.767, total – 0.962, grinding (stage I, MSTs) – 3.123, grinding (stages II-III, MSTs) – 7.458, total – 10.581. At the Kachkanarsky GOK, ore is crushed to a particle size of P95 = 30 mm without the use of a closed crushing cycle. Data are given only for the main equipment (crushers, screens, and mills) without auxiliary equipment. Grinding energy costs are 11 times higher than crushing energy costs, so it is more profitable to increase crushing costs if this leads to the reduction in subsequent grinding costs.
One way to reduce grinding costs is to reduce the particle size of finely crushed ore. For this, closed cycles of crushing at the third stage are mainly used, which make it possible to reduce the maximum particle size of finely crushed ore to 10-14 mm according to standard circuits.
Innovative solutions to reduce the particle size of finely crushed ore include the use of high-pressure grinding rolls (HPGR) as an additional fourth stage of crushing [8, 9]. This method allows to reduce the maximum particle size of finely crushed ore to 6-12 mm. DMS can be used both on ore after traditional crushing, and on the product crushed in the HPGR.
According to the Stoilensky GOK, the use of HPGR as the fourth stage of crushing makes it possible to increase the processing unit productivity by 12-15 % and reduce the specific power consumption by 2 %. A technical and engineering solution enabling to reduce the particle size of finely crushed ore should be attributed to solutions that increase the efficiency of crushing circuits, since this reduces the cost of subsequent grinding.
Increasing the efficiency of the DMS technology is mainly associated with the application of DMS after different crushing stages, the use of several DMS operations, and the choice of magnetic induction depending on the size of the feed particles [10, 11].
Dry magnetic separation can be used already after the first stage of crushing, if there are lean rock pieces in the crushed ore that can be removed into tailings. The same applies to crushed products after the second and third crushing stages. The maximum removal of tailings at the crushing stage can significantly reduce the cost of subsequent grinding. At a number of enterprises, DMS tailings after classification are used to obtain additional highly profitable products, crushed stone (Vysokogorsky and Kachkanarsky GOKs, Bogoslovskoye Mining Administration).
If the finely crushed ore has a particle size of more than 10-12 mm, then in order to increase the DMS efficiency, a circuit with screening and separate beneficiation of small and large products should be used. The higher the feed particle size, the greater should be the magnetic induction of the separators.
Performance indicators of finely crushed ore DMS increase when using double-drum separators, in which a circuit is implemented with the main and control operations. In this case, in the first operation, separation in a decreased magnetic field (induction less than 0.1-0.14 T) should be used, in the second, separation in a higher magnetic field (B = 0.16-0.25 T).
Improving the efficiency of grinding circuits
Energy consumption for grinding reaches 50-60 % of the energy consumption of the beneficiation plant. Therefore, increasing the efficiency of grinding circuits associated with reducing energy costs when obtaining a product with the required particle size is an urgent task. This task is solved by grinding technologies associated with the use of various equipment: HPGR (finely crushed ore particle size reduction), semi-autogenous grinding mills (SAG mills), ball mills with increased productivity, vertical mills (“Vertimilll”). Increasing the efficiency of grinding circuits is also achieved by automated operation of closed-cycle equipment (mill, pump, hydrocyclone), demagnetizing the feed of classifying equipment, the use in a closed grinding cycle of beneficiation operation (WMS) and fine screening instead of classifiers in a closed grinding cycle. The efficiency of grinding circuits is increased by using a technology with a staged separation of not only tailings, but also the concentrate after grinding.
The use of mills of various designs and automation systems for the operation of equipment in grinding circuits
The question on the effective use of mills of various designs in the circuits of grinding iron ores can be referred to as a debatable one. A typical answer is the assertion that the choice of applied grinding circuits and mills of various designs depends on the physical and mechanical properties of the ore and its material composition, which determines the possibility of separating tailings using DMS before grinding.
The results presented in scientific papers point to an increase in the efficiency of grinding circuits when using HPGR [12], SAG mills [13, 14], “Vertimill” [15], and equipment automation systems [16, 17] in closed grinding cycles. [18] concludes that the projects of new operating plants for the beneficiation of copper-bearing ores mainly use the HPGR technology (for hard ores) and SAG mills (for ores with medium and soft hardness). However, it was noted in [18] that for brittle itaberite (ferruginous quartzites) ore in the project of an iron ore combine (Minas Rio, Brazil), the HPGR technology followed by ball milling was used.
The use of HPGR, SAG and Vertimill mills does not exclude the use of ball mills and technology applying only rod and ball mills. For iron ores, the fundamental issue is the possibility of using DMS to remove large lump tailings before grinding, especially if additional products (crushed stone) can be obtained from them. If DMS allows reducing the costs of subsequent grinding due to the removal of large lump tailings, then the grinding technology applying SAG mills should not be used. The use of HPGR and “Vertimill” does not lead to the exclusion of DMS from the performance circuit, so they may be more promising in the circuits of iron ore preparation compared to the SAG mills. This conclusion is confirmed by the results of testing various circuits for grinding itaberite ore (Brazil), given in [9].
The final decision on the application of grinding technology using HPGR, SAG mills and “Vertimill” mills, along with ball grinding, should be made based on the results of a performance comparison of possibilities.
The use of an automated system mill – pump – hydrocyclone enables to stabilize the operation of the equipment by controlling their modes. Table 1 shows the results of testing and operation of the Cavex – Warman hydrocyclone unit (Kachkanarsky GOK, 2007). The classification process control system in the hydrocyclone 650CVX led to an increase in the classification efficiency from 41.1 to 56.32 % with a significant decrease in the –0.071 mm class content in sands (by 5.8 %). The circulating load decreased by 21 % and the possibility of increasing the productivity of the processing unit by 6-8 % was shown.
Table 1
Comparative tests of hydrocyclones 650CVX and HC-710 at the second grinding stage
Feed |
Overflow |
Sands |
Classification |
||||
Content, % |
Yield, % |
Content, % |
Content, % |
||||
–71 µm |
solid |
–71 µm |
solid |
–71 µm |
solid |
||
Hydrocyclone unit “HC-710 – 8GR-8 pump” |
|||||||
24.0 |
43.8 |
21.92 |
58.2 |
18.0 |
14.4 |
73.3 |
41.10 |
Automated hydrocyclone unit “650CVX – Warman pump” |
|||||||
21.0 |
40.8 |
24.65 |
58.9 |
16.2 |
8.6 |
81.1 |
56.32 |
Application of beneficiation operation (WMS) in a closed grinding cycle
This technical and engineering solution makes it possible to remove non-overground rock minerals from the closed grinding cycle and reduce the circulating load. Basically, this circuit is used only in the beneficiation of magnetite ores, which is associated with the high performance of PBM magnetic separators. The condition for the applicability of the technology is a high yield of WMS tailings from the cycle feed. For example, at the Kachkanarsky GOK, the use of WMS at the second grinding stage in a closed cycle (Fig.1) makes it possible to remove 40-50 % of the tailings from the closed cycle feed with a particle size of 35-45 % of the –71 µm class.
The use of slurry demagnetization
Slurry demagnetization is used to destroy magnetite floccules before classification, fine screening, and filtration, which leads to an increase in the efficiency of these processes [19]. Table 2 shows the comparative results of applying demagnetization before classification in hydrocyclones HC-710 in a closed grinding cycle of the second stage (Kachkanarsky GOK, 2005).
Table 2
Results of slurry demagnetization before classification [19]
Overflow yield |
–71 µm class content, % |
Classification |
||
in feed |
in overflow |
in sands |
||
Demagnetizer RA-4 |
||||
22.4 |
26.5 |
65.0 |
15.4 |
44.2 |
Without demagnetization |
||||
27.7 |
36.2 |
54.2 |
29.3 |
21.6 |
Demagnetization before classification in a closed grinding cycle makes it possible to obtain a finer overflow and increase the classification efficiency (Table 2).
The use of screens in a closed grinding cycle instead of hydrocyclones
The main purpose of using such a technical and engineering solution is to reduce the volume of grinding equipment by reducing the grinding stages or reducing the number of mills in the stages. This significantly reduces grinding costs.
Table 3 shows the results of testing Derrick 2SG40-60W-5STK sizer screens with 0.1 mm shale sneakers, which showed the possibility to reduce the grinding stages from three to two (Kachkanarsky GOK, 2007). A two-stage grinding circuit is possible when using two screens with one mill MShTs-3600´5000. The tests performed using one Derrick 2SG40-60W-5STK sizer screen with a large shale sneaker size (0.15 mm) showed the possibility to use a two-stage grinding circuit only if the productivity of the processing unit is 10-15 % lower than planned, which is unacceptable for an industrial enterprise [20].
Table 3
Performance balances of circuits with three and two grinding stages
Product |
Yield, % |
Content, % |
Fe extraction, % |
|
Fe |
–71 µm |
|||
Circuit with three grinding stages using classification in hydrocyclones |
||||
Concentrate |
16.51 |
62.70 |
93.20 |
66.57 |
Tailings |
83.49 |
6.23 |
– |
33.43 |
Ore |
100.00 |
15.55 |
– |
100.00 |
Circuit with two grinding stages using fine screening |
||||
Concentrate |
16.36 |
62.80 |
68.10 |
66.41 |
Tailings |
83.64 |
6.21 |
– |
33.59 |
Ore |
100.00 |
15.47 |
– |
100.00 |
The disadvantages of using screens in a closed grinding cycle include a decrease in the productivity of the beneficiation plant (with an insufficient number of screens) or an increase in investment (large number of screens) and operating costs (increased wear of screens) with a constant plant productivity. This can lead to reduced economic efficiency from reducing the volume of grinding equipment.
Reduction in the number of grinding stages from three to two due to fine screening was achieved by obtaining concentrate of the required quality with a larger particle size. The content of –71 µm class in the concentrate decreased from 93.2 to 68.1 % (Table 3). This will not be a disadvantage when producing concentrate for agglomeration. If the combine produces concentrate for pelletizing, then the technology under consideration may not be in demand. In world practice, iron concentrate is additionally grinded in the HPGR to provide it with the required particle size for pelletizing [21, 22].
Staged separation of the concentrate before the last stage of grinding
The use of a typical circuit for grinding and beneficiation of iron ores in comparison with other ores makes it possible to reduce the cost of grinding due to the staged removal of tailings after each stage of grinding and beneficiation, including from the grinding stages (Fig.1). Staged separation of not only tailings, but also concentrate is possible. Such a technology is always considered if it is possible to isolate concentrate (sinter ore) from finely crushed rich iron ore using DMS.
The feed at the last grinding stage of magnetite ores beneficiation circuits contains a significant amount of open grains of magnetite (titanomagnetite). Table 4 shows the results of fractionation by size of the WMS-III magnetic product (feed of the last grinding stage) of the Kachkanarsky GOK beneficiation plant. Fractionation results show that more than 50 % of the feed at the last grinding stage is the finished concentrate. Therefore, it is possible to reduce the amount of middlings fed to the third grinding stage by isolating a portion of the finished concentrate before the last grinding stage. The use of technology with staged concentrate separation allows reducing the volume of grinding equipment for the last grinding stage.
Table 4
Results of fractionation according to the particle size of the WMS-III middlings
Size class, mm |
Yield, % |
Content, % |
Distribution of iron, % |
+0.16 |
4.25 |
45.37 |
3.37 |
−0.16+0.1 |
19.58 |
48.96 |
16.74 |
−0.1+0.071 |
24.03 |
56.14 |
23.55 |
−0.071+0.045 |
27.29 |
60.86 |
29.00 |
−0.045+0 |
24.85 |
63.02 |
27.34 |
Total −0.071+0 |
52.14 |
61.88 |
56.34 |
Total |
100.00 |
57.27 |
100.00 |
The circuit with the staged concentrate separation applied at the beneficiation plant of the Kachkanarsky GOK (Fig.1) is shown in Fig.2. The following can be used as beneficiation methods and devices for the staged concentrate separation: drum magnetic-gravity separators with a modified bath [23]; drum separators with an alternating magnetic field [24, 25]; MGS magnetic-gravity separators of a cylindrical type with a vertical direction of movement of the separated products [26]; fine screening [27]; gravity separation (screw separation) [5].
Performance indicators of concentrate N 1 separation increase when using combined circuits: fine screening – magnetic separation of the undersize product or screw separation – magnetic separation of the heavy product.
Table 5 shows the results of testing the circuit with the staged separation of concentrate during the beneficiation of ore from the Gusevogorskoye deposit (Kachkanarsky GOK, Fig.2). Comparison of the results obtained under industrial conditions showed the advantage of fine screening for the staged separation of concentrate compared with using drum magnetic-gravity separators. Concentrate N 1 yields were 50.8 and 22.2 % using fine screening and magnetic-gravity separators, respectively. Comparison of the results obtained in laboratory conditions showed the advantage of separation in an alternating magnetic field for the staged separation of concentrate compared with using a combined circuit with screw and magnetic separation. Concentrate N 1 yields were 52.25 and 43.14 % when using separation in an alternating magnetic field and gravity-magnetic separation, respec tively. It should be noted that the results obtained in laboratory conditions are always higher than those obtained in industrial conditions. Therefore, the most promising for industrial application are practices with staged concentrate separation using a combined circuit with fine screening and magnetic separation of the undersize product (Table 5).
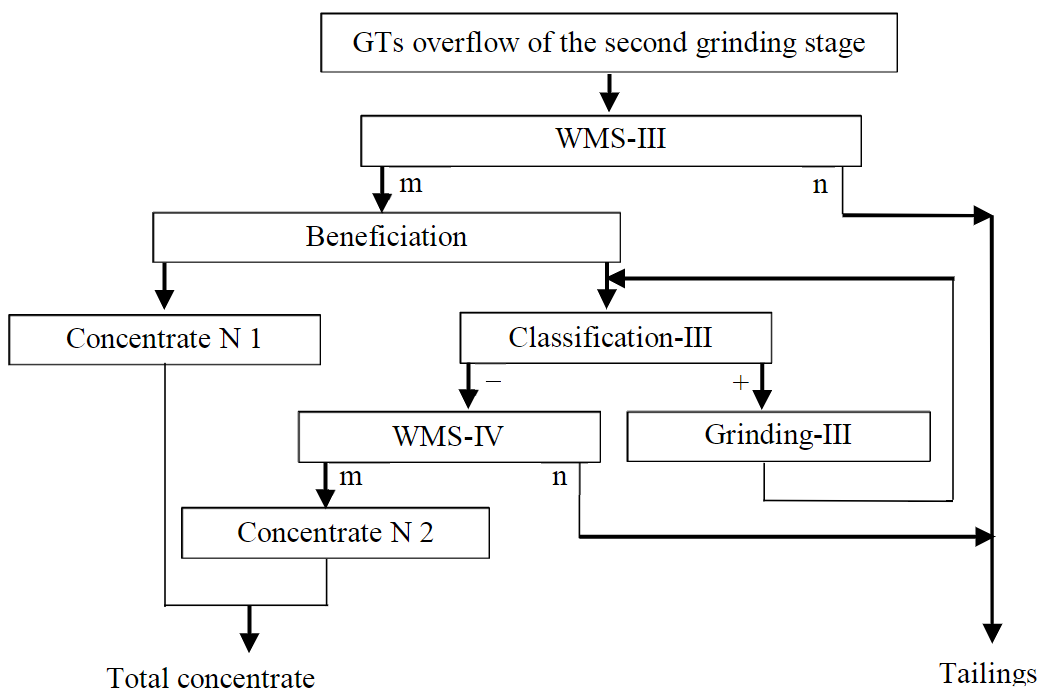
Fig.2. Staged concentrate dressing
Table 5
Results of testing the beneficiation methods and devices for staged separation of concentrate from WMS-III middlings
Product |
Yield for |
Content, % |
Fe extraction |
|
Fe |
−71 µm |
|||
Drum magnetic-gravity separator (industrial testing) |
||||
Concentrate N 1 Middling (non-magnetic) |
22.2 77.8 |
61.8 58.6 |
59.4 55.8 |
23.1 76.9 |
Feed |
100.0 |
59.3 |
56.6 |
100.0 |
Screening (0.15 mm) and magnetic separation (industrial testing) |
||||
Concentrate N 1 Middling (oversize) |
50.8 49.2 |
62.0 53.9 |
79.9 33.6 |
54.3 45.7 |
Feed |
100.0 |
58.0 |
57.1 |
100.0 |
Drum separator with alternating magnetic field (laboratory tests) |
||||
Concentrate N 1 Middling (non-magnetic) |
52.25 47.25 |
61.51 49.53 |
65.02 49.13 |
57.61 42.39 |
Feed |
100.0 |
55.79 |
57.43 |
100.0 |
Screw and magnetic separation (laboratory tests) |
||||
Concentrate N 1 |
43.14 |
61.51 |
– |
46.94 |
Middling of screw separation and non-magnetic |
56.86 |
52.75 |
– |
53.06 |
Feed |
100.00 |
56.53 |
– |
100.00 |
The results of industrial tests of the staged concentrate separation using fine screening at the Kachkanarsky GOK showed that this technology makes it possible to reduce the volume of mills at the third stage by 25-33 % or increase the productivity of the beneficiation plant by 10 % [27]. With the same productivity of the plant and the volume of the mills at the third stage, the use of staged extraction of concentrate makes it possible to increase the extraction of iron into concentrate by 0.5-1 %.
When using the staged concentrate separation, the −71 µm class content in the separated concentrate N 1 increases in comparison with the beneficiation operation feed (Table 5). However, the −71 µm class content in the total concentrate of the beneficiation plant will decrease, since concentrate N 1 will not go to grinding at the last stage. Therefore, the technology with staged concentrate separation is promising for beneficiation plants that produce concentrate for agglomeration. If the performance calculations show the high efficiency of this technology for plants producing concentrate for pelletizing, one can consider regrinding of iron concentrate in a HPGR to ensure the required particle size of the concentrate for pelletizing [21, 22].
An increase in the processing plant productivity leads to an increase in the amount of marketable products. If the technology used is applied without increasing the volume of grinding equipment, then this leads to an increase in the economic performance of the mining and beneficiation plant. To solve this task, technical and engineering solutions are used, mainly associated with a decrease in the particle size of finely crushed ore and an increase in the grinding circuits efficiency.
Reducing the particle size of finely crushed ore will always lead to an increase in the productivity of the ore beneficiation plant. With a decrease in the particle size of finely crushed ore, the yield of DMS tailings will grow, which will increase the productivity of the mills of the first and partially second grinding stages. Reducing the particle size of finely crushed ore will increase the cost of crushing, which will always be lower than the cost of ore grinding. The use of such technology should be considered if the iron content in the DMS tailings will not exceed the iron content in the WMS tailings. Otherwise, the extraction of iron into concentrate will decrease.
The use of almost all of the described technologies (except for the use of screens in a closed grinding cycle), leading to an increase in the efficiency of grinding circuits, makes it possible to increase the beneficiation plant productivity without reducing the concentrate quality.
If screens are used as classifiers in a closed grinding cycle, then in order to increase the plant productivity, it is necessary to increase the number of screens (increase investment and operating costs). This will lead to an increase in the concentrate particle size, which may be unacceptable in the production of concentrate for pelletizing. Therefore, the replacement of hydrocyclones with screens in a closed cycle to increase the beneficiation plant productivity must be justified by the feasibility study of possibilities. For example, in [28], it was concluded that it is necessary to replace screens in a closed grinding cycle with hydrocyclones to increase the plant productivity, and it was proposed to use screens in the final operations to improve the concentrate quality.
To improve the concentrate quality, technologies with additional beneficiation of the run-of-mine (crude) concentrate of the beneficiation plant are mainly used. The use of technologies for additional beneficiation of crude concentrate always leads to an increase in iron losses with tailings and a decrease in concentrate yield. The decision to use technologies to improve the concentrate quality is dictated by economics. If the increase in the company's profit associated with an increase in the price of high-quality concentrate exceeds the losses associated with a decrease in the amount of concentrate produced and an increase in its cost, then this technology is always used.
The following technologies enable to improve the concentrate quality.
- Regrinding and magnetic separation of run-of-mine concentrate. The technology is implemented at the Lebedinsky GOK and makes it possible to obtain a “superconcentrate” with iron content of more than 69 % and silicon dioxide of less than 3% for the out-of-blast production of iron and steel (for metallization) [29].
- Reverse flotation of crude concentrate. This technology became widespread [4, 30]. In Russia, flotation is used to improve the concentrate quality at the Mikhailovsky GOK and makes it possible to obtain a “superconcentrate” with iron content of 69 % and silicon dioxide of 3 % for the out-of-blast production of iron and steel [31].
- Fine screening. The technology is applied at Kostomukshsky and Lebedinsky GOKs. The use of fine screening is due to the increased content of iron in fine grades compared to large grades of concentrate particle size [32, 33].
- Magnetic-gravity separation in MGS separators designed by the Mining Institute of the KSC RAS. MGS separators are used by the Kostomukshsky and Olenegorsky GOKs [33].
- Separation in an alternating magnetic field [24, 34]. This technology is not used in the industry, as industrial separators with a variable magnetic field are under development.
The disadvantage of all technical and engineering solutions for the additional beneficiation of crude concentrate is the production (except for high-quality concentrate) of a hard-to-beneficiate middling. Additional beneficiation of such a middling leads to the production of tailings with a high iron content and an increase in iron losses in the whole beneficiation plant.
Increasing the integrated use of raw materials is relevant for any mineral raw materials [35]. An increase in the integrated use of iron ore raw materials is possible by expanding the yield of magnetite concentrate, extracting iron into concentrate, and obtaining a second iron (hematite) concentrate when beneficiating ferruginous quartzites or a second titanium-containing (ilmenite) concentrate when beneficiating ilmenite-titanomagnetite ores. When beneficiating titanomagnetite ores, it is also possible to obtain ilmenite concentrate.
Increasing the yield of magnetite concentrate and the extraction of iron into concentrate is mainly achieved by using technologies with staged extraction of concentrate [27] or by using drum separators with systems of permanent magnets with increased energy (Nd- Fe-B) at the first stages of beneficiation (DMS and WMS-I) [36, 37]. The use of separators with increased magnetic induction (0.25-0.5 T) can lead to a decrease in the iron content in the concentrate. According to the industrial tests at the Kachkanarsky GOK of wet magnetic separation (WMS-I) at the first stage, there was an increase in the concentrate yield by 2 rel.% and iron extraction into concentrate by 1.1 rel.% [3]. Iron content in the concentrate decreased from 61.2 to 60.7 %. Therefore, trying to significantly increase the yield of magnetite concentrate without reducing its quality will not work. The magnetite iron content in the total beneficiation tailings decreased from 0.53 to 0.42 % (by 20 rel.%), which is a high indicator. The achieved indicators were obtained during the beneficiation of lean titanomagnetite ore from the Gusevogorskoe deposit (15-16 % Fe).
During the beneficiation of magnetite and ferruginous quartzites, magnetite is extracted into the concentrate. Hematite is mainly transferred to tailings of wet magnetic separation in a weak field. This is mainly due to the very thin dissemination of hematite (less than 0.05 mm) and its reduced magnetic properties. To extract hematite, combined technologies are developed [38], including high-gradient magnetic separation in a strong field [39], gravity [40], and flotation separation [41, 42]. The use of fine screening is promising [43]. Dry magnetic separation of hematite is possible in separators with permanent magnets made of Nd-Fe-B [44]. For enterprises processing ferruginous quartzites (Mikhailovsky and Kimkano-Sutarsky GOK), the introduction of technologies for producing hematite concentrate is a particularly urgent task. Fig.3 shows a laboratory-tested combined magnetic-gravity circuit for obtaining hematite concentrate from WMS tailings (non-magnetic product) of the circuit for obtaining magnetite concentrate from ore of the Sutarskoe deposit. Hematite in the ore is represented by scaly-shaped grains (mostly less than 0.05 mm in size). High-gradient separation was performed with a magnetic induction of 0.4 (main operation) and 0.6 T (control separation). Gravity separation was carried out on a sludge concentration table “MOLM”. As a result of beneficiation, hematite concentrate with iron content of 62.3 % at a yield of 4 % (from ore) was obtained [43].
Ilmenite concentrate is produced from the non-magnetic WMS product in the circuit for obtaining titanomagnetite concentrate during the beneficiation of ilmenite-titanomagnetite ores. To obtain ilmenite concentrate, combined technologies are used, including high-gradient magnetic separation in a strong field [45], gravity [40] and flotation [46, 47], or electrical separation [48]. The use of thin screening is promising. When beneficiating titanomagnetite ores, ilmenite concentrate is also produced from the non-magnetic WMS product of the circuit for obtaining titanomagnetite concentrate. The use of a combined magnetic-gravity-electric circuit made it possible to obtain ilmenite concentrate from the ore of the Gusevogorskoe deposit under laboratory conditions with a titanium dioxide
content of 48.66 % at a yield of 0.13 % (of the ore). The circuit (Fig.3) was used, with additional beneficiation of the heavy product on the laboratory electric separator ELKOR-2.
Application of domestic equipment in iron ore beneficiation circuits
Almost all of the listed technical and engineering solutions aimed at increasing the efficiency of iron ore beneficiation can be implemented using equipment from domestic manufacturers.
High-performance and reliable equipment for crushing, screening, and grinding is produced by “Uralmashzavod”, “Rudgormash”, “Tyazhmash”. Equipment not produced in Russia includes cone crushers for medium and fine crushing with automatic continuous adjustment of the opening (Sandvik, Krupp, MetsoGP). However, the experience of operating these crushers at iron ore enterprises revealed the shortcomings of crushers associated with an increase in repair costs, so a number of enterprises (Kostomukshsky, Stoilensky, and Vysokogorsky GOK) replaced this equipment with “Uralmashzavod” crushers or suspended their implementation (Kachkanarsky GOK). Domestic enterprises do not produce vertical mills “Vertimill” and HPGR. The main advantage of the “Vertimill” mills is the smaller weight and occupied volume. However, the operating costs for grinding in “Vertimill” and ball mills do not differ significantly, and mills are not used at Russian iron ore GOKs. Ore preparation technologies that cannot be implemented with the help of domestic equipment include only the use of HPGR. However, closed third stage crushing cycles using modern “Uralmashzavod” crushers make it possible to achieve a finely crushed ore particle size of less than 15 mm (at Vysokogorsky GOK, a size of −13+0 mm was achieved). The use of HPGR at the fourth stage of crushing makes it possible to achieve an ore particle size of less than 6 mm. Such a solution may turn out to be unprofitable due to the high operating costs when using HPGR. Therefore, the lack of domestic developments in the field of HPGR is not problematic.
Automated systems mill – pump – hydrocyclone and demagnetizing devices are produced by many enterprises, for example, “Turbonasos” and NPK “Mekhanobr-tekhnika”. Hydraulic screens for fine screening are produced by NPO “Pnevmash” (analogous to Derrick screens).
Drum magnetic separators for dry and wet beneficiation with a weak (0.16 T) and increased magnetic field (0.25 T) are produced by the “Rudgormash” plant. The production of high-performance separators with an increased drum length PBM-P-150/360 was mastered. Equipment for gravity separation is produced by “Mashzavod Trud” and NPK “Spirit”. Flotation machines, including those with increased volume (RIF-300Ts), are produced by NPO “RIVS”. Electrical separators are manufactured by NPK “Mekhanobr-tekhnika” and “Russkaya Korona”. Industrial separators with a variable magnetic field have not been developed in Russia and other countries. MGS separators should be attributed to domestic innovative developments.
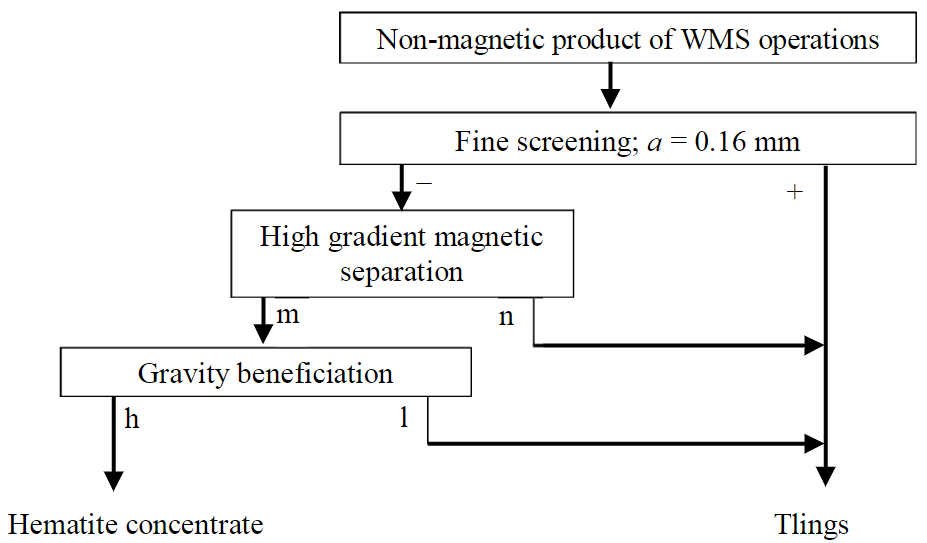
Fig.3. Obtaining hematite concentrate from ferruginous quartzites (h – heavy product; l – light product)
High-gradient magnetic separators with increased productivity are not produced by domestic enterprises due to their lack of demand. In Russia, ilmenite-titanomagnetite ores are not processed, and ferruginous quartzites are mainly processed only to obtain magnetite concentrate; therefore, separators with a low productivity of up to 10 t/h are manufactured (NPK “Mekhanobr-tekhnika”) for another type of mineral raw materials. High-gradient magnetic separators were developed back in the USSR; if the need arises for them, then the domestic industry will begin to produce them.
Russia also produces the main laboratory equipment to study the dressability of iron ores and develop the circuits for their beneficiation, for example, NPK “Mekhanobr-tekhnika”.
Specific enterprises producing equipment are given as an example. The information is taken from the official websites of enterprises. Many types of equipment are made by other companies, so mining and beneficiation plants have alternative possibilities in choosing an equipment supplier. This enables to talk about the possibility of implementing technologies that improve the efficiency of iron ore beneficiation using domestic equipment.
Conclusion. The growth in the efficiency of iron ore beneficiation technologies is mainly associated with an increase in the efficiency of grinding technologies, an increase in the quality of concentrates and the integrated use of raw materials. The application of any innovative technologies should be justified by the results of a feasibility study.
The efficiency of grinding circuits is increased by reducing the particle size of finely crushed ore, the use of crushed ore DMS and ball grinding in drum mills along with mills of other designs. Increasing the efficiency of grinding circuits is achieved by automation systems for the operation of closed-cycle equipment, using WMS in a closed grinding cycle, demagnetization of feed for classifying equipment, using fine screening instead of classifiers in a closed grinding cycle, and technology with staged concentrate separation after the last grinding stage.
For beneficiation of iron ores, in addition to magnetic beneficiation, it is possible to use flotation, gravity, and magnetic-gravity separation, fine hydraulic screening, separation in an alternating magnetic field and separators with increased magnetic induction at the first stages of beneficiation. Many technologies are applied in industrial enterprises. The use of combined beneficiation circuits makes it possible to improve the quality of iron concentrate and the integrated use of raw materials, associated with an increase in the concentrate yield and the production of hematite concentrate from hematite-magnetite ores and ilmenite concentrate from titanomagnetite ores.
Technical and engineering solutions to improve the efficiency of iron ore beneficiation are feasible using domestic equipment.
References
- Gabdulkhakov R.R., Rudko V.A., Pyagay I.N. Methods for modifying needle coke raw materials by introducing additives of various origin (review). Fuel. 2022. Vol. 310. N 122265. DOI: 10.1016/j.fuel.2021.122265
- Vaisberg L.A., Dmitriev S.V., Mezenin A.O. Controllable magnetic anomalies in mineral processing technologies. Gornyi zhurnal. 2017. N 10, p. 26-32 (in Russian). DOI: 10.17580/gzh.2017.10.06
- Pelevin A.E., Sytykh N.A. Increased Magnetic field induction Separators in Titanium Magnetite Ore Processing. Obogashchenie rud. 2020. N 2, p. 15-20 (in Russian). DOI: 10.17580/or.2020.02.03
- Zhuravleva E.S., Chanturiya E.L. Evaluation of the possibility of using electrochemical technology for the preparation of waters and reagents to improve the technological parameters of processing of unoxidized ferruginous quartzites. Chernye metally. 2018. N 5, p. 6-9 (in Russian).
- Prokopyev S.A., Pelevin A.E., Napolskikh S.A., Gelbing R.A. Staged screw separation of magnetite concentrate. Obogashchenie rud. 2018. N 4, p. 28-33 (in Russian). DOI: 10.17580/or.2018.04.06
- Vaisberg L.A., Korovnikov A.N., Trofimov V.A. Innovative re-equipment of screening circuits (to commemorate the 100th anniversary of the Mekhanobr Institute). Gornyi zhurnal. 2017. N 1, p. 11-17 (in Russian). DOI: 10.17580/gzh.2017.01.02
- Moraes M.N., Galery R., Mazzinghy D.B. A review of process models for wet fine classification with high frequency screens. Powder Technology. 2021. Vol. 394, р. 525-532. DOI: 10.1016/j.powtec.2021.08.078
- Rodriguez V.A., Barrios G.K.P., Bueno G., Tavares L.M. Investigation of Lateral Confinement, Roller Aspect Ratio and Wear Condition on HPGR Performance Using DEM-MBD-PRM Simulations. Minerals. 2021. Vol. 11. Iss. 8. N 801. DOI: 10.3390/min11080801
- Salaza J.S., Santos N.S.L., Tavares L.M. Holistic Pre-Feasibility Study of Comminution Routes for a Brazilian Itabirite Ore. Minerals. 2021. Vol. 11. Iss. 8. N 894. DOI: 10.3390/min11080894
- Tereshchenko S.V., Shibaeva D.N., Kompanchenko A.A., Alekseeva S.A. Research of the influence of material composition and size of iron quartzites of the Olenegorsk deposit on the results of dry magnetic separation. Obogashchenie rud. 2020. N 6, p. 15-20
(in Russian). DOI: 10.17580/or.2020.06.03 - Shibaeva D.N., Tereshchenko S.V. Studies on the Possibility of Pre-Concentration of Low-Iron Ores of the Kovdor Deposit. Obogashchenie rud. 2019. N 5, p. 24-28 (in Russian). DOI: 10.17580/or.2019.05.05
- Rodrigues A.F.V., Delboni H., Powell M.S., Tavares L.M. Comparing strategies for grinding itabirite iron ores in autogenous and semi-autogenous pilot-scale mills. Minerals Engineering. 2021. Vol. 163. N 106780. DOI: 10.1016/j.mineng.2021.106780
- Roux J.D., Steinboeck A., Kugi A., Craig I.K. Steady-state and dynamic simulation of a grinding mill using grind curves. Minerals Engineering. 2020. Vol. 152. N 106208. DOI: 10.1016/j.mineng.2020.106208
- Ziolkowski L., Roux J.D., Craig I.K. Optimizing grinding mill performance using extremum seeking control. IFAC-PapersOnLine. 2021. Vol. 54. Iss. 11, p. 43-48. DOI: 10.1016/j.ifacol.2021.10.048
- Rocha D., Spiller E., Taylor P., Miller H. Predicting the product particle size distribution from a laboratory vertical stirred mill. Minerals Engineering. 2018. Vol. 129, p. 85-92. DOI: 10.1016/j.mineng.2018.09.016
- Tereshchenko S.V., Shibaeva D.N., Kompanchenko A.A., Alekseeva S.A. Research of the influence of material composition and size of iron quartzites of the Olenegorsk deposit on the results of dry magnetic separation. Gornyi zhurnal. 2016. N 5, p. 94-96
(in Russian). DOI: 10.17580/gzh.2016.05.14 - Botha S., Roux J.D., Craig I.K. Hybrid non-linear model predictive control of a run-of-mine ore grinding mill circuit. Minerals Engineering. 2018. Vol. 123, p. 49-62. DOI: 10.1016/j.mineng.2018.04.016
- Baranov V.F. Designs of new operating copper processing plants: process types, equipment selection, industry trends. Obogashchenie rud. 2021. N 1, p. 44-52 (in Russian). DOI: 10.17580/or.2021.01.08
- Pelevin A.E. Effects of magnetic flocculation on iron-bearing ore concentration. Obogashchenie rud. 2021. N 4, p. 15-20
(in Russian). DOI: 10.17580/or.2021.04.03 - Pelevin A.E., Sytykh N.A. Titanomagnetite Ore Two-Stage Grinding Circuit Tests. Obogashchenie rud. 2018. N 2, p. 13-18
(in Russian). DOI: 10.17580/or.2018.02.03 - Campos T.M., Bueno G., Barrios G.K.P., Tavares L.M. Pressing iron ore concentrate in a pilot-scale HPGR. Part 1: Experimental results. Minerals Engineering. 2019. Vol. 140. N 105875. DOI: 10.1016/j.mineng.2019.105875
- Campos T.M., Bueno G., Barrios G.K.P., Tavares L.M. Pressing iron ore concentrate in a pilot-scale HPGR. Part 2: Modeling and simulation. Minerals Engineering. 2019. Vol. 140. N 105876. DOI: 10.1016/j.mineng.2019.105876
- Pelevin A.E., Sytykh N.A. Iron Concentrate Stage Separation by Means of Drum Magnetic Separator with Modified Separating Bath. Obogashchenie rud. 2016. N 4, p. 10-15 (in Russian). DOI: 10.17580/or.2016.04.02
- Pelevin A.E. Improving magnetite concentrate quality in an alternating magnetic field. Obogashchenie rud. 2019. N 6, p. 19-24 (in Russian). DOI: 10.17580/or.2019.06.04
- Karmazin V.V., Andreev V.G., Palin I.V. et al. Development of Equipment for the Technology of Full-Stage Beneficiation of Magnetite Quartzite. Gornyi zhurnal. 2010. N 12, p. 85-89 (in Russian).
- Opalev A.S., Birukov V.V., Scherbakov A.V. Stadial Obtaining Of The Magnetite Concentrate During The Development Of Power Resource-Saving Technology Of Benefication Of Ferruginous Quartzites On JSC “Olcon”. Mining Informational and Analytical Bulletin. 2015. N 11, p. 60-62 (in Russian).
- Pelevin A.E., Sytykh N.A. Fine hydraulic screening for staged separation of titanium-magnetite concentrate. Obogashchenie rud. 2021. N 1, p. 8-14 (in Russian). DOI: 10.17580/or.2021.01.02
- Palaniandy S., Halomoan R., Ishikawa H. Tower Mill circuit performance in the magnetite grinding circuit – The multi-component approach. Minerals Engineering. 2019. Vol. 133, р. 10-18. DOI: 10.1016/j.mineng.2018.12.019
- Nemykin S.A., Kopanev S.N., Mezentseva E.V., Okunev S.M. Iron concentrate production with the increased content of useful component. Gornyi zhurnal. 2017. N 5, p. 27-31 (in Russian). DOI: 10.17580/gzh.2017.05.05
- Pattanaik A., Rayasam V. Analysis of reverse cationic iron ore fines flotation using RSM-D-optimal design – An approach towards sustainability. Advanced Powder Technology. 2018. Vol. 29. Iss. 12, p. 3404-3414. DOI: 10.1016/j.apt.2018.09.021
- Ismagilov R.I., Baskaev P.M., Ignatova T.V., Shelepov E.V. The prospects for expanding the iron ore mineral and raw material base through the processing of oxidized ferruginous quartzite of the Mikhailovskoe deposit. Obogashchenie rud. 2020. N 3, p. 19-24 (in Russian). DOI: 10.17580/or.2020.03.04
- Ismagilov R.I., Kozub A.V., Gridasov I.N., Shelepov E.V. Case Study: Advanced Solutions Applied by JSC Andrei Varichev Mikhailovsky GOK to Improve Ferruginous Quartzite Concentration Performance. Russian Mining Industry. 2020. N 4, p. 98-103. DOI: 10.30686/1609-9192-2020-4-98-103
- Opalev A.S., Khokhulya M.S., Fomin A.V., Karpov I.V. Creation of innovative technologies for production of high-quality iron concentrate production in the North West of Russia. Gornyi zhurnal. 2019. N 6, p. 56-60 (in Russian). DOI: 10.17580/gzh.2019.06.17
- Korchevenkov S.A., Aleksandrova T.N. Preparation of standard iron concentrates from non-traditional forms of raw material using a pulsed magnetic field. Metallurgist. 2017. Vol. 61, р. 375-381. DOI: 10.1007/s11015-017-0503-z
- Chanturia V.A. Scientific substantiation and development of innovative approaches to integrated mineral processing. Gornyi zhurnal. 2017. N 11, p. 7-13 (in Russian). DOI: 10.17580/gzh.2017.11.01
- Pelevin A. E., Tsypin E. F., Koltunov A. V. Komlev S. G. High-intensity magnetic separators with permanent magnets. News of the Higher Institutions. Mining Journal. 2001. N 4-5, p. 133-136 (in Russian).
- Yakubailik E.K., Ganzhenkob I. M., Butovb P. Yu., Kilin V.I. Reduce the Loss Iron in the Wet Separation in High Fields. Journal of Siberian Federal University. Engineering & Technologies. 2016. Vol. 9. N 8, p. 1302-1310 (in Russian).
- Nikolaeva N.V., Aleksandrova T.N., Afanasova A.V., Chanturiya E.L. Mineral and technological features of magnetite-hematite ores and their influence on the choice of processing technology. ACS Omega. 2021. Vol. 6. N 13, р. 9077-9085. DOI: 10.1021/acsomega.1c00129
- Kuskov V.B., Lvov V.V., Yushina T.I. Increasing the recovery ratio of iron ores in the course of preparation and processing. CIS Iron and Steel Review. 2021. Vol. 21. N 1, р. 4-8. DOI: 10.17580/cisisr.2021.01.01
- Nienaber E.C., Auret L. Experimental modelling and plant simulation of spiral concentrators: Comparing response surface methodology and extended Holland-Batt models. Minerals Engineering. 2019. Vol. 141. N 105833. DOI: 10.1016/j.mineng.2019.105833
- Safari M., Hoseinian F.S., Deglon D. et al. Investigation of the reverse flotation of iron ore in three different flotation cells: Mechanical, oscillating grid and pneumatic. Minerals Engineering. 2020. Vol. 150. N 106283. DOI: 10.1016/j.mineng.2020.106283
- Shrimali K., Atluri V., Yan Wang et al. The nature of hematite depression with corn starch in the reverse flotation of iron ore. Journal of Colloid and Interface Science. 2018. Vol. 524, р. 337-349. DOI: 10.1016/j.jcis.2018.04.002
- Pelevin A.E. Production of hematite concentrate from hematite–magnetite ore. Mining Informational and Analytical Bulletin. 2020. N 3-1, p. 422-430 (in Russian). DOI: 10.25018/0236-1493-2020-31-0-422-430
- Tripathy S.K., Singh V., Murthy Y.R. et al. Influence of process parameters of dry high intensity magnetic separators on separation of hematite. International Journal of Mineral Processing. 2017. Vol. 160, р. 16-31. DOI: 10.1016/j.minpro.2017.01.007
- Jianwu Z., Luzheng C., Ruoyu Y. et al. Centrifugal high gradient magnetic separation of fine ilmenite. International Journal of Mineral Processing. 2017. Vol. 168, p. 48-54. DOI: 10.1016/j.minpro.2017.09.005
- Degodya E.Yu., Shavakuleva O.P. Elaboration of a technology for production conditional ilmenite concentrate by enrichment of titanium-magnetite ores. Ferrous Metallurgy. Bulletin of Scientific, Technical and Economic Information. 2019. Vol. 75. N 5, p. 572-576
(in Russian). DOI: 10.32339/0135-5910-2019-5-572-576 - Shaojun B., Pan Y., Zhan D. et al. New insights into lead ions activation for microfine particle ilmenite flotation in sulfuric acid system: Visual MINTEQ models, XPS, and ToF–SIMS studies. Minerals Engineering. 2020. Vol. 155. N 106473. DOI: 10.1016/j.mineng.2020.106473
- Yang Xing, Wang Haifeng, Peng Zhen et al. Triboelectric properties of ilmenite and quartz minerals and investigation of triboelectric separation of ilmenite ore. International Journal of Mining Science and Technology. 2018. Vol. 28. Iss. 2, p. 223-230. DOI: 10.1016/j.ijmst.2018.01.003