Improving the reliability of 3D modelling of a landslide slope based on engineering geophysics data
- 1 — Ph.D., Dr.Sci. Professor Saint Petersburg Mining University ▪ Orcid
- 2 — Ph.D. Associate Professor Saint Petersburg State University ▪ Orcid
- 3 — Engineer-Geologist of the II Category ”Promterra” Ltd. ▪ Orcid
- 4 — Ph.D. Chief Specialist JSC “Polymetal Engineering” ▪ Orcid
Abstract
Landslides are among the most dangerous geological processes, posing a threat to all engineering structures. In order to assess the stability of slopes, complex engineering surveys are used, the results of which are necessary to perform computations of the stability of soil masses and assess the risks of landslide development. The results of integ-rated geological and geophysical studies of a typical landslide slope in the North-Western Caucasus spurs, composed of clayey soils, are presented. The purpose of the work is to increase the reliability of assessing the stability of a landslide mass by constructing a 3D model of the slope, including its main structural elements, identified using modern methods of engineering geophysics. Accounting for geophysical data in the formation of the computed 3D model of the slope made it possible to identify important structural elements of the landslide, which significantly affected the correct computation of its stability.
Introduction
Assessment of the operational reliability of constructed and designed engineering structures on slopes is based on the concept of the detailed structure and stress-strain state of the soil mass, as well as the identification of factors influencing the formation and development of landslide processes, and indicators characterizing the stability of slopes [1].
The degree of danger of slopes for construction is determined on the basis of engineering geological models that characterize the probable position of the main structural elements of landslide displacements, the spatial distribution of the stress-strain state, strength and physical properties of the landslide mass [2-4]. In this case, particular importance should be given to the detail studies of soil strata [5-7]. Increasing the detail of studies enable to identify factors that significantly affect the assessment of a landslide slope stability. These are the presence of lenses and thin beds, to which sliding surfaces can be confined, complex topography of the landslide bed, numerous fractures that cut through the landslide body [1, 8]. Such a requirement necessitates a reasonable interpolation of data on the geological structure within the interwell space. The high-quality interpolation of geological interfaces with the identification of local structural elements in the landslide mass is hindered by a significant distance between wells. The probable missing of the structural elements causing landslide displacements is high. In addition, the quality of interpolation is significantly affected by the conditions of work on the excavation of engineering geological workings. Thus, often due to the complexity of drilling on steep slopes, engineering geological wells are replaced by pits, which,
despite their advantages, will not allow reaching the required depth of research. Therefore, the distance between wells in the line can reach 100 m (Fig.1). Correct geological interpolation with a rare network of wells and mine workings, considering all the factors affecting the stability of a landslide slope, is possible using modern methods of engineering geophysics, which allow obtaining almost continuous 2D geological and geophysical sections. Increasing reliability of the slope stability assessment is also facilitated by the construction of 3D models identifying the main landslide elements, including the localization of zones of weakened soils, and the determination of the spatial distribution of physical mechanical properties in the soil mass.
According to the current regulatory documentation (SP 420.1325800.2018), it is recommended to perform engineering geological computations of slope stability in a 2D setting for a line oriented along the most likely direction of landslide displacement, along the maximum slope of the surface. However, the 2D problem formulation method does not consider possible displacements that can occur outside the estimated line plane. The impact of various factors, including the results of human engineering and economic activities, the heterogeneous geological structure of the landslide mass, as well as the specific topography of the slope surface, can cause a complex nature of the field of the stress-strain state of the soil mass and create a landslide displacement trajectory which does not correspond to the estimated line of the slope [9, 10].
Analysis of a 3D slope model allows to obtain a pattern for a more probable landslide displacement of soils and, accordingly, to improve the accuracy of assessing the stability of soil masses. Compared to flat 2D models, 3D models give a more complete and correct idea of the structure of the main deformable horizon based on the spatial shape and position of displacement planes, lines of landslide fractures, separation walls, protrusion ramparts, and landslide terraces within the emerging landslide cirque [11, 12]. These data make it possible to determine the spatial distribution of the slope stability factor, considering the 3D approximation of its structure. To develop a more adequate 3D model and increase the reliability of the landslide slope stability assessment, it is necessary to use integrated geoscientific information about its detailed geological structure.
To calculate slope stability using a 3D model, the Slide3 software (Canadian company Rocscience Inc [13]) was used, which approximates a soil mass by 3D computed blocks. When pas-sing from 2D to 3D formulation of the problem by the limit equilibrium method, the concept of describing the shape of the sliding surface completely changes [14]. For example, a round-cylindrical sliding surface in a 2D problem formulation is synthesized as spherical or ellipsoidal when passing to a 3D model. In the 3D problem, when calculating the stability, the limiting balance of the holding and shear forces in two orthogonal directions is considered. The vertical load defines the perpendi-cular and tangential forces acting on the basis of each computed block of the model. The values of the forces that shear and oppose the displacement determine the resulting stability factor for the estimated 3D sliding surface [13, 15]. In general, modelling in a 3D setting makes it possible to obtain more correct slope stability characteristics in comparison with the 2D approach [14, 16].
Recently, electrical tomography (ET) and seismic tomography (ST) have been widely used to study landslides, providing the most complete and detailed information on the geological and geophysical properties of landslide structures [17]. Unlike 1D methods of studying a slope using wells and pits, modern 2D geophysical technologies make it possible to obtain an almost continuous section of a landslide slope with an indication of the main structural elements of the landslide mass and to localize weakened zones associated with the formation of fractures and increased soil moisture [18-20].
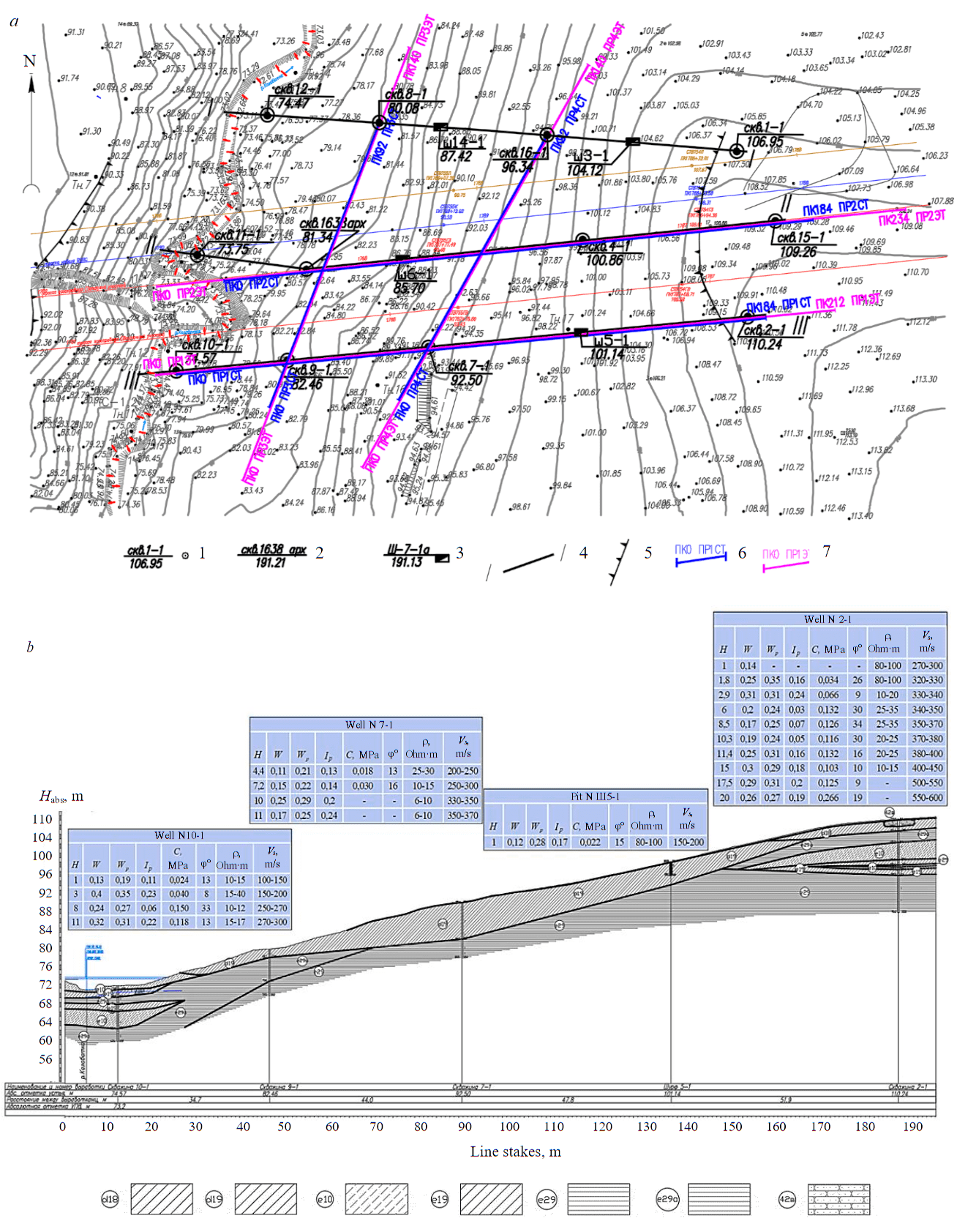
Fig.1. Layout plan of engineering geological workings and geophysical lines (a) and engineering geological section along line III-III (b) 1 – geological well, its number, abs. head mark, m; 2 – archived geological well, its number, abs. head mark, m; 3 – pit, its number, abs. head mark, m; 4 – line and number of engineering geological section; 5 – boundary of the long-term stabilized landslide according to the engineering geological surveys; 6 – seismic tomography lines; 7 – electrical tomography lines
Methodology
The target of 2D and 3D modelling is a landslide slope on the northeastern low of the Northwestern Caucasus. The landslide is genetically related to the watercourse formation. The landslide basis is the riverbed (Fig.1, b). The average slope of the surface is 11-15°. The landslide separation walls and its sides are not clearly traced due to the dense vegetation and turfiness of the slope. The surface of the sliding tongue is smoothed and eroded by a temporary stream in the channel part. The topography in the upper part of the slope is more gentle, and closer to the watercourses, its inflection is observed, leading to an increase in the slope to 15-25°. In this area, there are weakly turfed thrust edges, drunken forest, and in some places even shallow gullies. On the landslide slope, geomorphological elements are weakly expressed.
The geological section of the studied landslide area (Fig.1, b) is represented mainly by the Quaternary deposits [21]. The upper part of the section to a depth of 8 m is composed of deluvial loams, represented by the following lithological varieties: yellow-brown heavy sandy hard loam (d18); light grey heavy sandy hard loam with interlayers of weakly cemented sandstone to 3-5 mm (d19). Loams overlie the following varieties of eluvial clays: clay from dark brown to light grey, light silty hard, with vegetation residues (e29); bluish-grey light silty semi-hard dense clay (e29a). In the upper and lower intervals of the slope, there are lenses of light grey hard dense sandy loam of eluvial genesis with nodules of carbonates and shells to 10 % (e10) and yellow-brown heavy sandy hard loams (e19). Well 2-1 in the upper part of the section uncovered a lens of dark yellow, low-strength, weakly ferruginous, weathered, weakly cemented sandstone (42c).
According to the engineering geological studies, an increase in the moisture content and plasti-city of clayey soils with depth is noted, while the consistency index is mainly below zero. At the boundary of loams and underlying eluvial clays in some engineering geological workings in the central part of the section, an increase in moisture can be traced. The consistency index in this zone reaches 0.22, and the moisture values approach the limit of plasticity as much as possible [22].
As a result of field and laboratory studies of soils in a landslide mass, strength characteristics were determined according to the scheme of an unconsolidated level (see table). The values of strength properties increase with depth, which is typical for landslide slopes [14, 23, 24].
Physical and mechanical properties of soils
Engineering |
Cohesion C, kPa |
Angle of internal |
Soil density at natural moisture ρ, g/cm3 |
Dry strength Rdry, MPa |
Strength in the |
d19 |
23 |
16 |
1.8 |
– |
– |
d18 |
40 |
15 |
1.7 |
– |
– |
e10 |
131 |
32 |
1.95 |
– |
– |
e19 |
31 |
15 |
1.89 |
– |
– |
e29 |
112 |
16 |
1.94 |
– |
– |
e29a |
101 |
15 |
1.9 |
– |
– |
42в |
– |
– |
1.93 |
23.46 |
16.8 |
The engineering geological data obtained in wells and pits, together with the results of electrical and seismic tomography studies, made it possible to develop a 3D model of the landslide slope, as well as to analyse the effect on the estimated stability of the landslide slope in local areas of weakened rocks of the landslide mass, distinguished using geophysical methods.
Analysing the signs of manifestation of landslide activity on the slope under consideration (landslide topography, weakly turfed thrust edges, drunken forest), one can confidently assert the formation of a landslide and the active landsliding. As practice shows, in the presence of these signs, the slopes are in a state close to the limiting equilibrium. The stability factors of such slopes, as a rule, do not exceed 1.1.
Results of computation of slope stability on the basis of engineering geological surveys
Guided by the information on the composition, moisture content, and properties of the landslide slope soils, obtained from the engineering geological surveys, a computed 3D engineering geological model of the landslide slope was developed. The computations presented in this article are performed as an example without considering possible seismic effects. For a comparative analysis of the 3D and 2D modelling, computational engineering geological models of the landslide slope were built along parallel lines II-II and III-III.
Fig.2, a shows the results of 3D modelling, performed only on the basis of engineering geological surveys in the Slide3 software package. Coloured outlines on the 3D model surface characterize the spatial distribution of the estimated factor of slope stability [25], the colour scale shows changes in the values of this factor in the range from 2.72 to 6.00. The minimum slope stability factor is 2.72. The obtained factor values make it possible to assess the slope as a whole as stable. The most unstable area, highlighted in red in the 3D model, is in the central part of the slope, where wells 9-1, 1638 арх, 7 are marked, which penetrated a layer of weak loam d19, the thickness of which in these wells varies from 2 to 7 m.
Computation of the stability factor for the 2D model of the landslide slope along line III-III, which crosses the weakened zone, was performed using the Morgenstern – Price method. This method has the best convergence in iterative computations, enabling to calculate the stability factor and build a prism of possible collapse most accurately. The initial data for modelling (Fig.3, a) were only materials of engineering geological studies [26, 27]. The section of the 2D slope model shows the result of searching for the most probable sliding surface with the minimum value of the stability factor, calculated using the Slide2 software [28, 29]. The estimated value of the stability factor was 2.42, which is somewhat lower than the value of Kstab computed for a spherical surface using the Slide3 software (see Fig.2, a). It should be noted that Kstab value along the sliding surface, obtained on the parallel line II-II, was 2.81, which, on the contrary, is somewhat higher than the value computed for the 3D slope model.
Based on the practice of studying landslide processes, as well as the assumption that the slope, which has all the signs of landslide activity, as a rule, has Kstab in the range to 1.1, it can be concluded that Kstab obtained as a result of 2D and 3D computations, are overestimated.
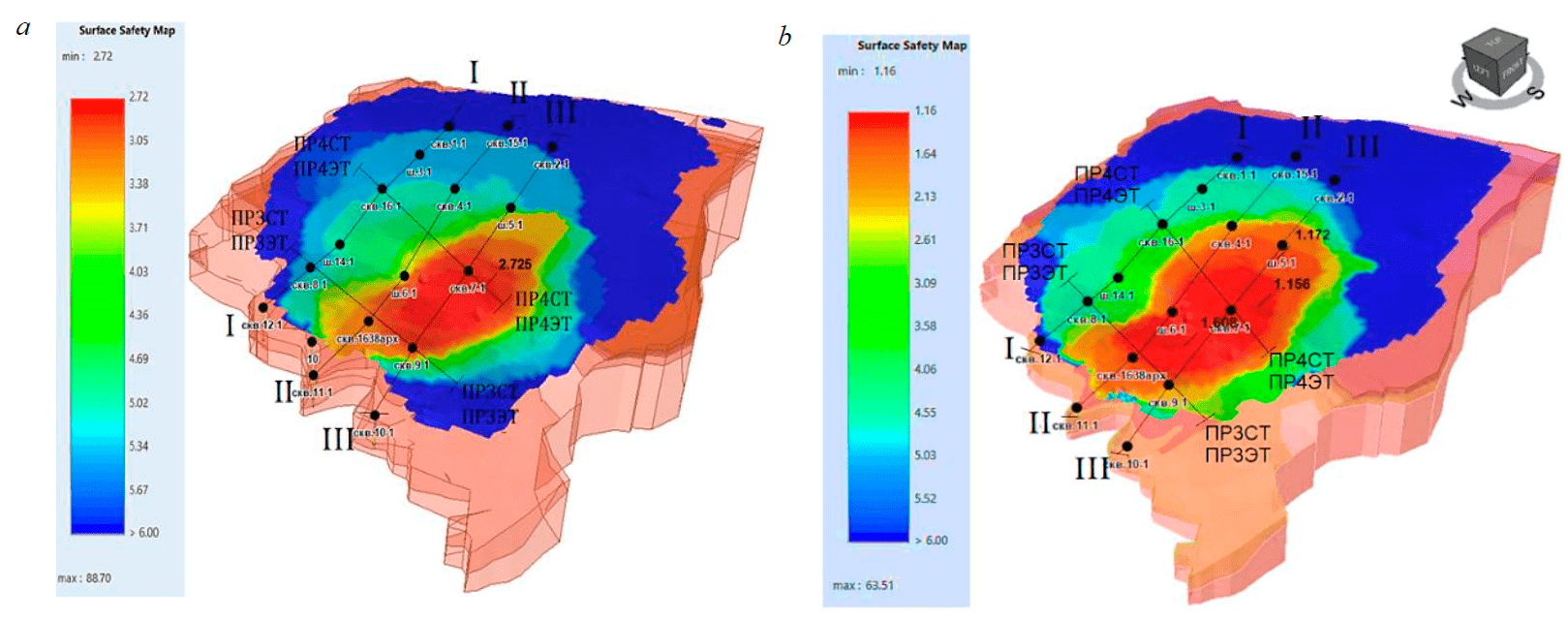
Fig.2. 3D model of the distribution of the stability factor of a landslide slope in the Slide3 software based on the engineering geological surveys (a) and integrated geological and geophysical studies (b)
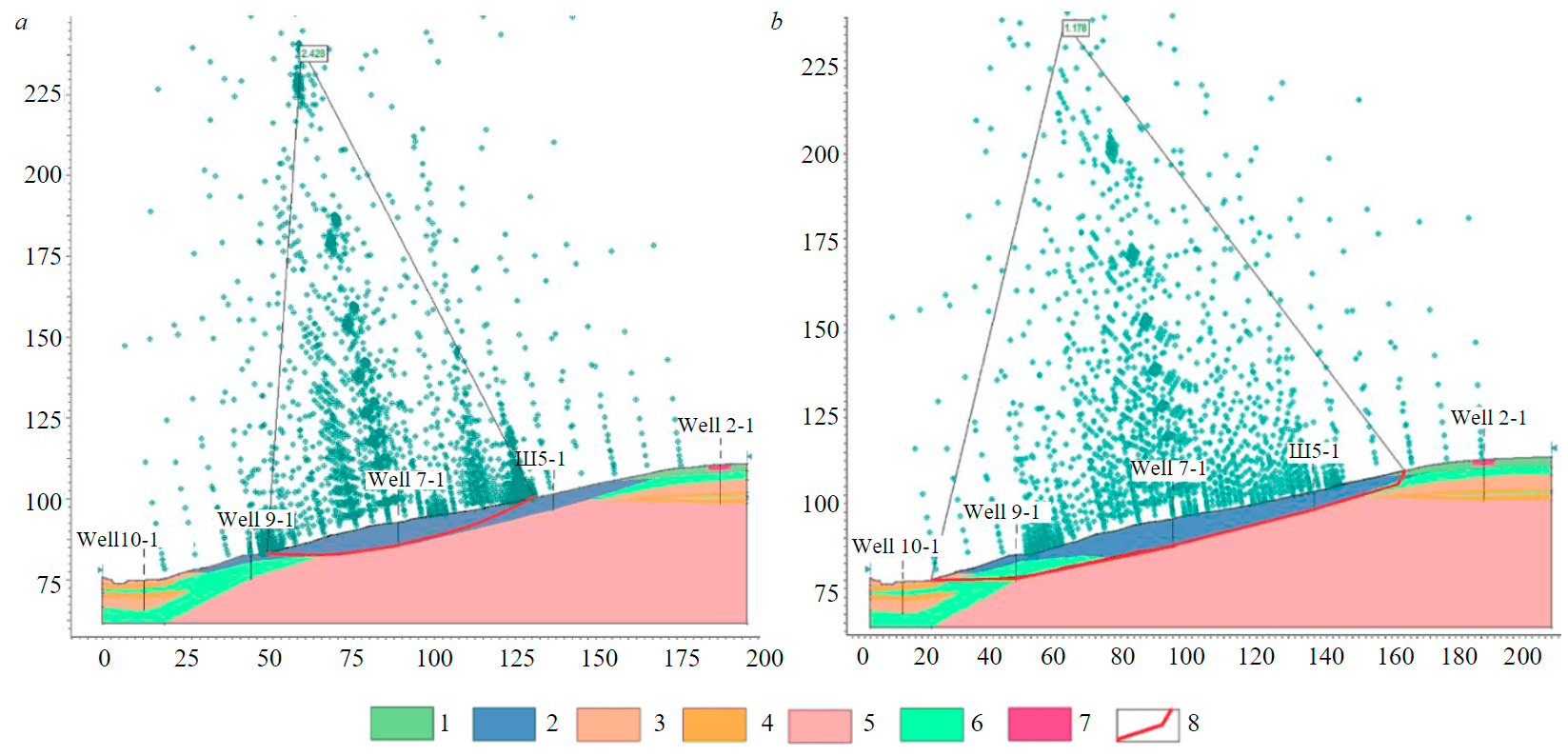
Fig.3. 2D computation of the stability factor of the landslide slope along line III-III according to the drilling materials (a) and integrated geological and geophysical studies (b) [30] Engineering geological elements: 1 – d18; 2 – d19; 3 – е10; 4 – e19; 5 – e29; 6 – e29a; 7 – 42в; 8 – sliding surface zones and failure fractures
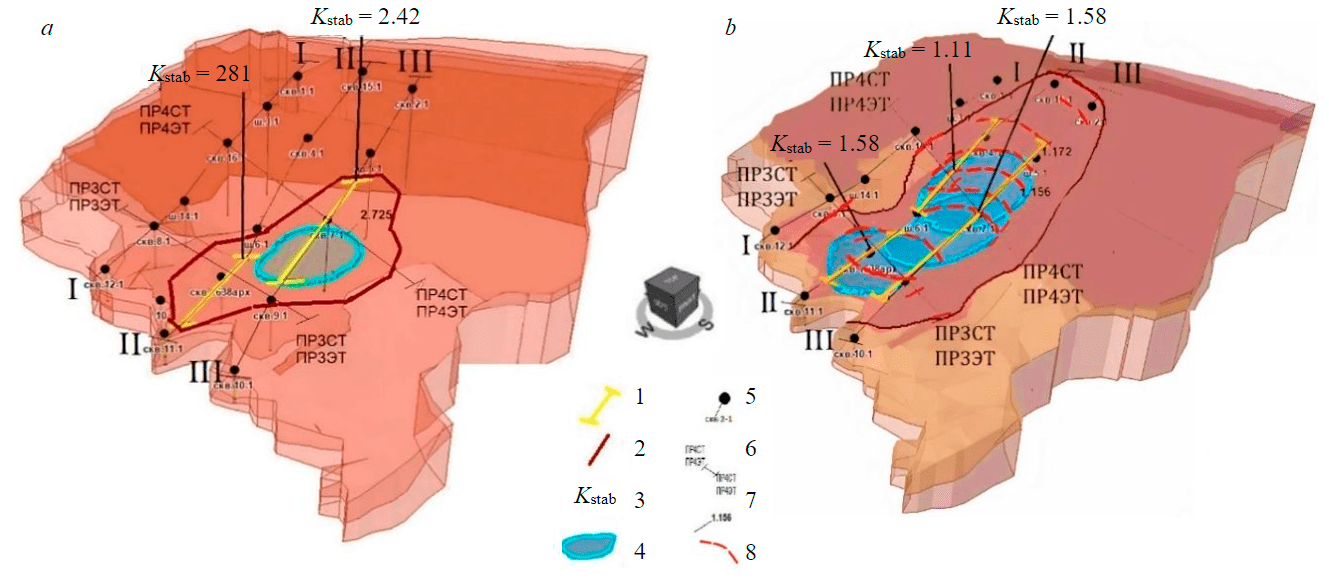
Fig.4. Generalized 3D model of a landslide-prone area according to engineering geological surveys (a) and integrated geological and geophysical studies (b) 1 – projection of a 2D sliding surface computed in Slide2; 2 – boundary of the area with the greatest landslide hazard; 3 – stability factor obtained in 2D computations; 4 – 3D sliding surface of a landslide; 5 – engineering geological workings; 6 – seismic and electrical tomography lines; 7 – stability factor obtained in 3D computations; 8 – landslide fractures
zone identified in the 3D model. In this case, the most probable landslide displacement along the spherical surface is of a more local nature.
Considering the known relationships between the dynamic shear modulus and the value of the specific cohesion C of clayey rocks, it should be considered that a decrease in the values of the velocity of shear waves Vs in clayey soils indicates a decrease in their strength due to disintegration and destruction of structural bonds [22]. This indicates the development of landslide displacements and the occurrence of stress-strain zones in the soil mass. For these reasons, the clayey rocks that make up the landslide body lose their structural strength and fractures develop in them [16, 31, 32].
On seismic tomographic sections, these processes are reflected in the form of near-vertical local zones characterizing the position of landslide fractures. As the practice of studying landslide mass shows, the values of specific cohesion C and the angle of internal friction φ in these zones can decrease to 50 % compared to undisturbed soils [26, 33].
The recent years were devoted to a comprehensive geophysical study of landslides of predominantly asequential type on the northern slopes of the Northwestern Caucasus [22, 26, 34]. As investigations showed, the main geophysical methods in the study of plastic landslides are ET and ST on S-waves. The combination of these geophysical methods makes it possible to confidently identify in the section of a plastic landslide such important structural elements as landslide fractures, which manifest in the form of local near-vertical regions with reduced values of specific electrical resistivity (SER) and Vs.
Tomographic geophysical studies of the slopes were carried out using standard methods of observation and data processing.
Electrical tomography observations were made using the Schlumberger installation and the Skala-48 multi-electrode electrical exploration station. The electrodes were placed along the streamer with a step of 3 m. The ET data were processed by the 2D inversion method using the Res2Dinv software, v.4.01.35. Inversion ensured the selection of SER values in a 2D grid model of the medium.
Seismic observations were carried out using the ELLISS-3 seismic station. The seismic receivers were installed with an interval of 2 m. The seismic source points were located every 4 m with offsets for half the length of the seismic receivers. Seismic tomography processing was performed in the XTomoDPU and XTomo software.
The spatial resolution on the surface was 1.5 m for electrical and 1 m for seismic tomography observations.
The results of the performed electrical tomography studies showed the high efficiency of this method for a detailed study of the heterogeneous structure of the landslide, as well as assessing changes in the composition and state of clayey soils (Fig.5, a). The analysis of the obtained electrical tomography sections of the landslide slope makes it possible to clearly trace the changes in humidity, which cause changes in the strength parameters of finely dispersed soils. In the near-surface parts of the electrical tomography sections, there are local zones with reduced values of the specific electrical resistivity of dispersed soils. These areas are associated with a local increase in humidity in places where landslide fractures form [22, 26].
Seismic tomography sections of the landslide slope characterize the 2D distribution of shear wave velocities Vs [26]. Due to the disintegration of clayey soils of landslide mass, in the studied seismic tomography sections of S-waves, areas with reduced values of the Vs velocities distribution are distinguished, corresponding to such important structural elements as fractures in the landslide body (Fig.5, b) [22, 35, 36].
In addition to studying electrical and seismic tomography longitudinal sections, it is also necessary to consider the structure of transverse geological and geophysical sections. In the transverse ET and ST lines, there are also local areas with reduced values of SER and Vs, corresponding to the position of landslide fractures. Differences in the form of local Vs and ρ anomalies in the longitudinal and transverse sections were established. On longitudinal geotomographic sections, these anomalies and, accordingly, landslide fractures have an inclination in the direction of slope dip, and on transverse sections, a multidirectional nature of their near-vertical occurrence is observed (Fig.6).
The slope of fractures in the longitudinal and transverse sections decreases with depth and is directed towards the extended area of disintegrated rocks of the landslide body. These differences can be explained by the fact that in the longitudinal lines, the ET and ST sections make it possible to identify landslide failure fractures, and in the transverse lines, shear fractures bordering the landslide body on both sides.
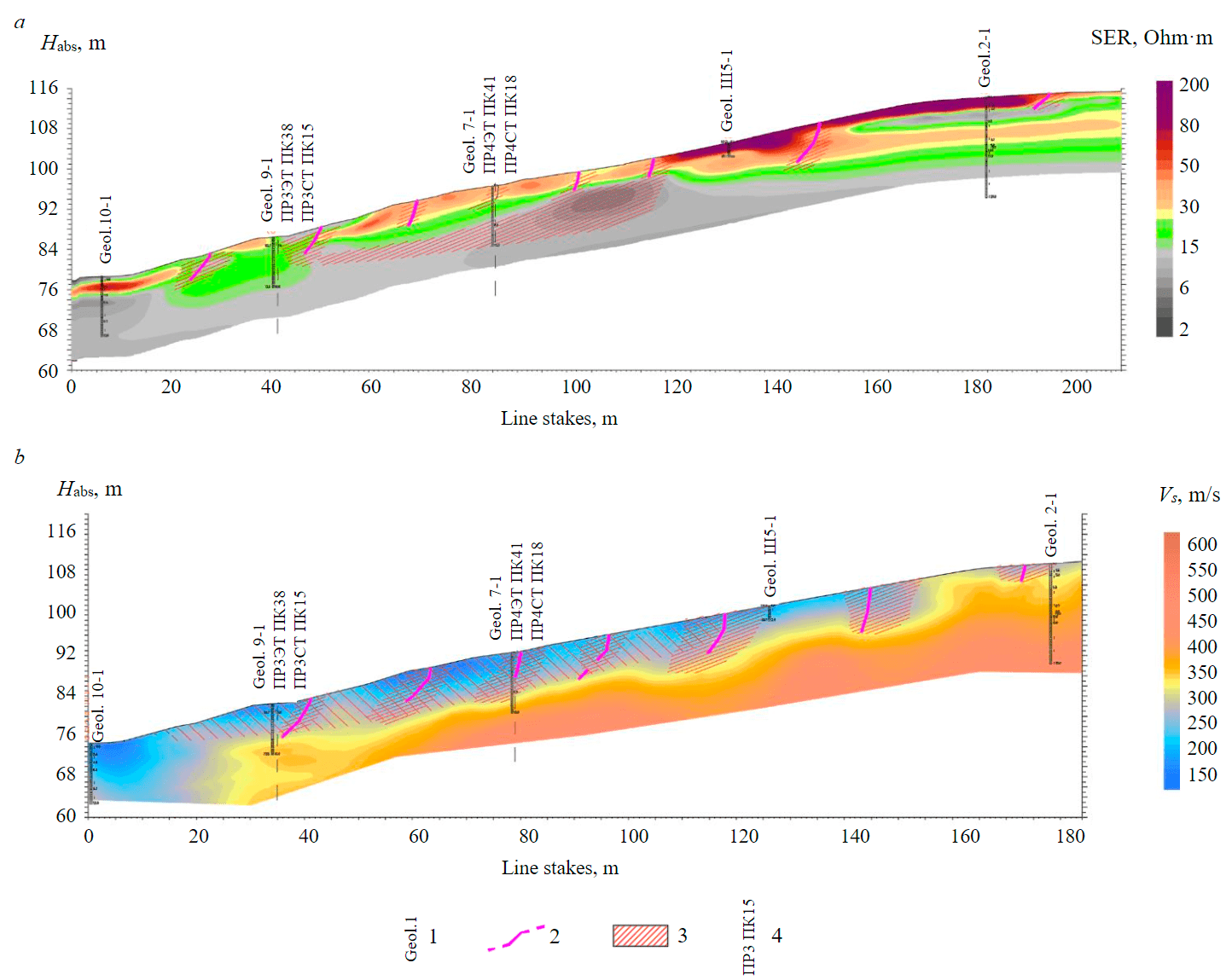
Fig.5. Electrical tomography (a) and velocity seismic tomography sections of shear waves Vs (b) along the longitudinal line III-III [26, modified] 1 – well number; 2 – supposed landslide fractures; 3 – areas with reduced values of SER and Vs, indicating an increase in soil moisture and a decrease in the specific cohesion value; 4 – intersections of geophysical lines
Geotomographic study of the landslide slope, together with the engineering and geological surveys, made it possible to perform a comprehensive interpretation [22, 37]. Based on its results, the spatial position of the lithological boundaries between engineering geological workings was corrected, zones of weakened soils confined to the main structural elements of the landslide were identified, and changes in the physical and mechanical properties of soils in these zones were assessed.
According to the integrated geological and geophysical interpretation (Fig.6), the sliding surface zone of a landslide is mainly confined to the weakening zone at the boundary of dispersed soils with various genesis, grain size, and consistency [34]. According to the classification of F.P.Savarensky, the studied landslide can be attributed to a mixed structural type, which has elements of both asequential and consequent types.
The main structural elements of the landslide were identified according to the joint analysis of ET, ST, and engineering geological sections, considering signs that indicate the disintegration of clayey soils and a decrease in their strength [22]. The results of electrical and seismic tomographic studies also made it possible to identify fractures of various landslide cycles in the landslide slope section.
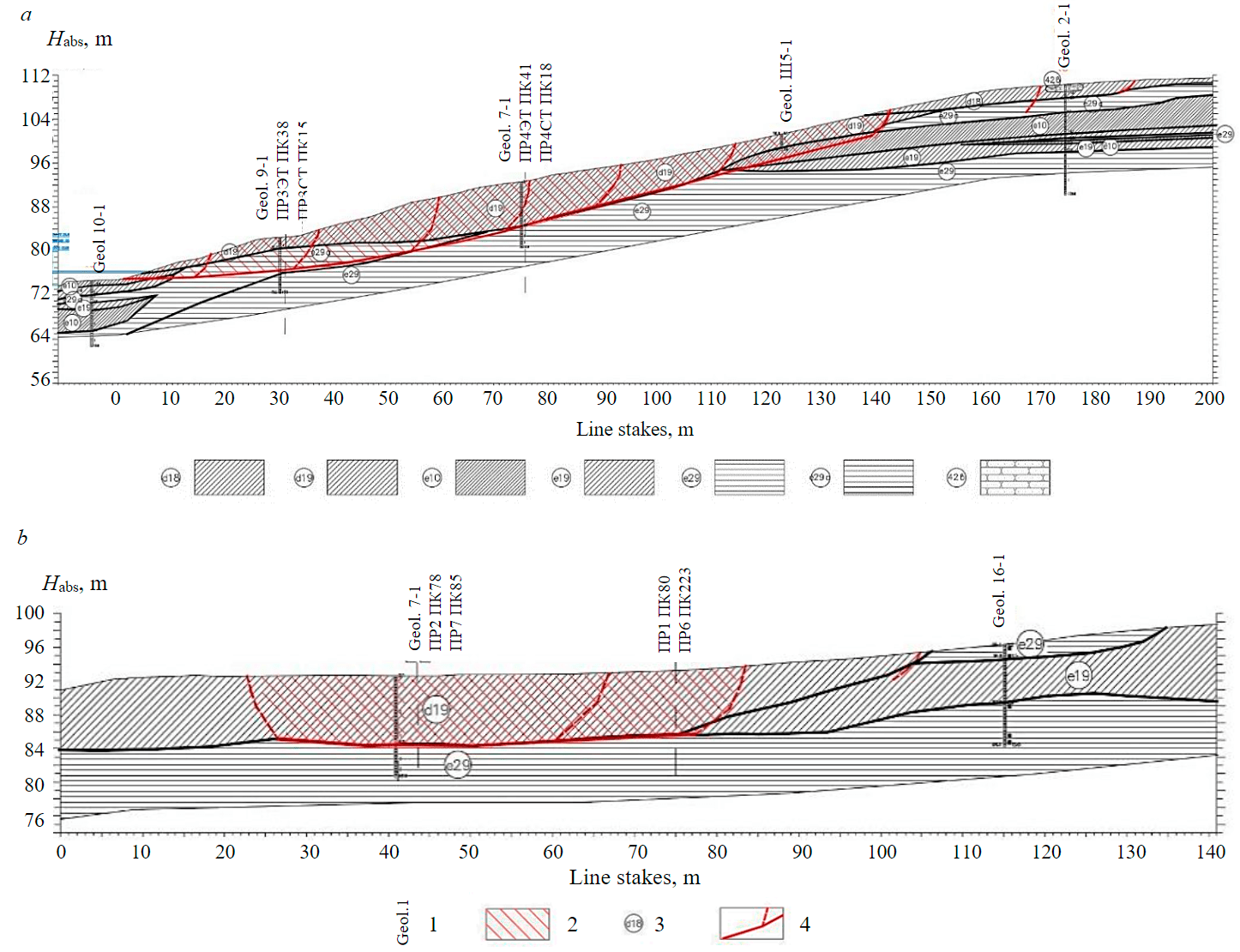
Fig.6. Geological and geophysical sections along the longitudinal III-III (a) and transverse line ПР4 (b) [22, 26, modified] 1 – well number; 2 – mechanically destroyed rocks that make up the landslide mass; 3 – number of engineering geological element; 4 – supposed zones of the sliding surface and landslide fractures
Thus, the results of an integrated geological and geophysical interpretation make it possible to introduce into the 3D model of the landslide slope the refined position of lithological boundaries, as well as zones with weakened disintegrated soils related to such important structural elements as the landslide body and landslide fractures. The introduction of these additional data into the 3D computational model of a landslide makes it possible to significantly refine the developed model and obtain more reliable slope stability properties [38-40].
Results of computation of slope stability on the basis of integrated geological and geophysical studies
Considering the available information about the properties of the soils in the landslide mass, obtained from the data of engineering and geological surveys, as well as additional information about the landslide slope structure, extracted from the materials of geophysical studies, an improved 3D engineering geological model of the landslide slope was formed. Information about the change in the physical and mechanical parameters of soils in weakened zones was taken based on an analysis of the available correlation dependences of the seismic properties of clayey rocks on their strength, as well as electrical and seismic tomography materials together with the results of engineering and geological surveys.
The introduction of additional geophysical information significantly affected the structure of the stress-strain state field of the landslide mass, which changed the area, outlines, and position of the mass most susceptible to landslide displacement (“red” zone). This area noticeably increased (see Fig.2, b), leading to a change in the value of the landslide stability factor, which decreased by more than two times. According to the zonal distribution of the stability factor, in some parts of the slope Kstab reaches values of 1.16, which makes it possible to classify the slope according to SP 420.1325800.2018 as conditionally stable.
Additional geophysical information in the 3D model of the landslide slope adjusted the determination of the position of the most probable sliding surfaces and the computation of the mass stability on these surfaces. As a result of the search for the most probable spherical sliding surfaces, the Slide3 software generated three surfaces located sequentially along the slope (see Fig.4, b). The values of the stability factors for these surfaces were 1.17; 1.16; 1.60. Individual spherical sliding surfaces are located with an overlap of about 20-25 m, which forms a single glacier-like landslide body elongated downdip. A significant decrease in Kstab after the introduction of additional geophysical information is due to the presence of a large number of weakened zones (landslide fractures) in the landslide body. This is especially noticeable in its central part.
Computation of slope stability in the Slide2 software using the Morgenstern – Price method also showed a decrease in the slope stability factor after introducing additional geophysical information. Fig.3, b shows the result of the computation in the Slide2 software for line III-III. Considering the zones of weakened soils and adjusting the position of lithological boundaries according to geophysics, Kstab value was 1.18.
The results of a 2D computation for two parallel lines II-II and III-III, considering the position of the zones with weakened soils confined to the main landslide elements, obtained from the materials of geological and geophysical interpretation, were plotted on the 3D model surface (see Fig.4, b). The analysis of 2D and 3D models with additional geophysical information showed the similar position of sliding surfaces on the slope. In this case, the stability factors have similar values.
Discussion
The results of 2D and 3D computer modelling, performed considering the ST and ET data, made it possible to determine the outline of the soil mass area subject to the greatest landslide hazard. The area is elongated along the slope and includes both the zones of the most probable sliding surfaces and landslide fractures, including fractures of various landslide cycles. An analysis of the glacier-like shape of the landslide structure showed that the landslide displacement will occur not only along but also across the slope. The introduction of additional geophysical information made it possible to obtain stability factors that are characteristic of slopes and indicate the presence of landslide activity. It should be noted that the failure fractures in the landslide mass, most clearly traced in the ET and ST data, correspond to the upper parts of spherical sliding surfaces.
Thus, 3D landslide modelling data confirm the expediency of using electrical and seismic tomography technologies to identify local areas of weakened soils associated with landslide fractures and to obtain more accurate estimates of the landslide slopes stability.
Conclusions
The results of 2D and 3D modelling based on engineering geological information make it possible to obtain close values of the landslide slope stability factors. However, a 3D model has undoubted advantages, as it gives a more complete pattern of the spatial distribution of the landslide body within the slope.
Unlike 1D slope studies from well drilling data, modern 2D geophysical technologies provide almost continuous sections of the landslide slope, characterizing the detailed structure of the main elements in the landslide and areas of weakened rocks. Considering integrated geophysical information obtained from longitudinal and transverse lines when performing a 3D computation of the soil masses stability enables to get the most complete pattern of a possible landslide displacement.
Accounting for geophysical information about the position of landslide failure and shear fractures bordering the landslide body in 3D modelling leads to increased reliability in assessing the stability factor of the landslide slope. In the considered case, the landslide slope, after considering the integrated geophysical information in the model computations, was reclassified from stable to conditionally stable.
Geophysical information about local weakened zones significantly changes the ideas about the structure of the sliding surface of a landslide. 3D model of the landslide, based only on engineering geological information, identified a single sliding surface, and with the involvement of engineering geophysics data, a glacier-like landslide structure appeared. The revealed more complex structure of the landslide indicates a multi-phase landslide displacement, which occurs not only along the line of maximum slope, but also across the slope.
Thus, additional geophysical information about the position of lithological boundaries and the localization of zones of weakened disintegrated soils of a landslide mass introduced into the computed 3D model significantly increases the reliability of assessing the landslide slope stability.
When designing construction works within a slope, an integrated geological and geophysical 3D model of a landslide slope provides making more informed decisions to ensure the operational reliability of structures built on it.
References
- Sowers G.B., Sowers G.F. Introductory Soil Mechanics and Foundations. New York: Macmillan, 1970, p. 544.
- Fedorenko E.V. Method for computing stability by reducing strength characteristics. Transport Rossiiskoi Federatsii. 2013. N 6 (49), p. 24-26 (in Russian).
- Cloutier C., Locat J., Couture R., Jaboyedoff M. The anatomy of an active slide: the Gascons rockslide, Québec, Canada. Landslides. 2016. Vol. 13, p. 241-258.
- Tiwari B., Sassa K., Bobrowsky P.T., Takara K. Understanding and Reducing Landslide Disaster Ris. Cham: Springer, 2021. Vol. 4, p. 644.
- Pavlovich A.A., Korshunov V.A., Bazhukov A.A., Melnikov N.Y. Estimation of Rock Mass Strength in Open-Pit Mining. Journal of Mining Institute. 2019. Vol. 239, p. 502-509. DOI: 10.31897/PMI.2019.5.502
- Pospehov G., Goloborodko A. Landslides Within River Valleys in the Southern Part Predportoviy Lowlands in Conditions of Intensive Development. Engineering and Mining Geophysics 2019 15th Conference and Exhibition, 22-26 April 2019, Gelendzhik, Russia. European Association of Geoscientists & Engineers, 2019. DOI: 10.3997/2214-4609.201901707
- Pospekhov G.B., Goloborodko A.A. Engineering geological support for the protection of the Pavlovsky Park area from landslides. Estestvennye i tekhnicheskie nauki. 2018. N 1 (115), p. 70-73 (in Russian).
- Stability of Slopes and Underground Excavations: Proceedings of Indian Geotechnical Conference 2020. Singapore: Springer, 2022. Vol. 3, p. 372. DOI: 10.1007/978-981-16-5601-9
- Kutepov Yu.I., Kutepova N.A., Vasileva A.D. External Dump Stability Substantiation and Monitoring in Kuzbass. Mining Informational and Analytical Bulletin. 2019. N 4, p. 109-120 (in Russian). DOI: 10.25018/0236-1493-2019-04-0-109-120
- Ponomarenko M.R., Kutepov Y.I. Mining Complexity Assessment to Substantiate Deformation Monitoring at Open Pit Mines. Journal of Mining Science. 2021. Vol. 57, p. 986-994. DOI: 10.1134/S1062739121060119
- Kutepova N.A., Kutepov Yu.I., Kudashov E.S., Daniliev S.M. Strength of Phosphogypsum Mixed With Nepheline Slime in Construction of Embankments of Gypsum Ponds. Mining Informational and Analytical Bulletin. 2020. N 10, p. 67-78 (in Russian). DOI: 10.25018/0236-1493-2020-10-0-67-78
- Kutepov Yu. I., Kutepova N. A., Vasileva А. D., Mukhina A. S. Engineering geological and ecological concerns in operation and reclamation of high slope dumps at open-pit mines in Kuzbass. Mining Informational and Analytical Bulletin. 2021. N 8, p. 164-178 (In Russian). DOI: 10.25018/0236_1493_2021_8_0_164
- Slide3 – 3D Limit Equilibrium Slope Stability Overview: Help to the Rocscience Slide 3. URL: https://www.rocscience.com/help/slide3/tutorials (accessed 12.04.2022).
- Fomenko I.K., Zerkal O.V., Gorobtsov D.N. State-of-the-art tools in quantifying slope stability. Inzhenerno-geologicheskie zadachi sovremennosti i metody ikh resheniya: Materialy dokladov nauchno-prakticheskoi konferentsii, 13-14 aprelya 2017, Moskva, Rossiya. Moscow: Geomarketing, 2017, p. 94-101 (in Russian).
- Kaya A., Ümit M. Midilli Slope stability evaluation and monitoring of a landslide: a case study from NE Turkey. Journal of Mountain Science. 2020. Vol. 17, p. 2624-2635. DOI: 10.1007/s11629-020-6306-x
- Cody E., Draebing D., McColl S. et al. Geomorphology and geological controls of an active paraglacial rockslide in the New Zealand Southern Alps. Landslides. 2020. Vol. 17, p. 755-776. DOI: 10.1007/s10346-019-01316-2
- Daniliev S.M., Danilieva N.A. Perspective Electrical Exploration the Dams of Gypsum Accumulator in the Framework for Geotechnical Monitoring. Engineering and Mining Geophysics 2019 15th Conference and Exhibition, 22-26 April 2019, Gelendzhik, Russia. European Association of Geoscientists & Engineers, 2019. DOI: 10.3997/2214-4609.201901716
- Burlutsky S.B., Taninkova V.S., Glazunov V.V. Refinement of Landslide Slope Stability Assessment Using Geophysical Survey Data. Engineering Survey. 2020. N 3, p. 58-67 (in Russian). DOI: 10.25296/1997-8650-2020-14-3-58-67
- Bounemeur N., Benzaid R., Atoub S.Geological, Hydrological and Geotechnical Impact for the Landslides Susceptibility in Mila Basin (Northeast Algeria). Research Developments in Geotechnics, Geo-Informatics and Remote Sensing: Proceedings of the 2nd Springer Conference of the Arabiane Journal of Geosciences (CAJG-2), 25-28 November 2019, Sousse, Tunisia. Springer, 2022, p. 11-15. DOI: 10.1007/978-3-030-72896-0
- Sassa K., Nagai O., Solidum R. et al. An integrated model simulating the initiation and motion of earthquake and rain induced rapid landslides and its application to the 2006 Leyte landslide. Landslides. 2010. Vol. 7, p. 219-236. DOI: 10.1007/s10346-010-0230-z
- Landslide Science and Practice. Ed. by C.Margottini, P.Canuti, K.Sassa. Vol. 5: Complex Environment, Springer, 2013, p. 354.
- Burlutskii S.B. Physical geology models of landslide slopes based on electrical and seismic tomography: Avtoreferat dis. ... kand. geol.-mineral. nauk. St. Petersburg: Sankt-Peterburgskii gornyi universitet, 2015, p. 22 (in Russian).
- Gallotti G., Tinti S. A New Approach for Landslide Modeling: Application to the Scilla 1783 Tsunamigenic Landslide, South Italy. Pure and Applied Geophysics. 2020. Vol. 177, p. 3563-3576. DOI: 10.1007/s00024-020-02546-5
- Liang Wang, Xue Zhang, Filippo Zanibon et al. Mathematical Optimization Problems for Particle Finite Element Analysis Applied to 2D Landslide Modeling. Mathematical Geosciences. 2021. Vol. 53, p. 81-103. DOI: 10.1007/s11004-019-09837-1
- Bar N., McQuillan A. 3D Limit Equilibrium Slope Stability Analysis for Anisotropic and Faulted Rock Masses in Australian Coal and Iron Ore Mines. 10th Asian Rock Mechanics Symposium, 29 October – 3 November, 2018, Singapore. OnePetro, 2018. N ISRM-ARMS10-2018-127.
- Burlutskiy S.B., Glazunov V.V. Identification Peculiarities of Landslide Structural Elements with the Use of a Two-Dimensional Seismic-Geoelectric Model Synthesized According to Electrotomographic and Seismotomographic Research Data. Engineering Survey. 2017. Vol. 6-7, p. 94-107 (in Russian). DOI: 10.3997/2214-4609.201700410
- Mandal S., Mondal S. Geoinformatics and Modelling of Landslide Susceptibility and Risk. An RS & GIS-based Model Building Approach in the Eastern Himalaya. Cham: Springer, 2019, p. 223. DOI: 10.1007/978-3-030-10495-5
- Shuvalova R.A., Burlutsky S.B., Glazunov V.V., Zhdanov S.V. Landslide Slope Stability Estimation by the Geotechnical and Geophysical Data. Engineering and Mining Geophysics 2021, 26-30 April 2021, Gelendzhik, Russia. European Association of
Geoscientists & Engineers, 2021. DOI: 10.3997/2214-4609.202152022 - Shu-wei Sun, Bo Pang, Jia-bing Hu et al. Characteristics and mechanism of a landslide at Anqian iron mine, China. Landslides. 2021. Vol. 18, p. 2593-2607. DOI: 10.1007/s10346-021-01671-z
- Slide2 User Guide Rocscience Inc., Toronto. URL: https://www.rocscience.com/help/slide2/tutorials (accessed 22.04.2022).
- Göktepe F., Keskin I. A Comparison Study between Traditional and Finite Element Methods for Slope Stability Evaluations. Journal of the Geological Society of India. 2018. Vol. 91, p. 373-379. DOI: 10.1007/s12594-018-0864-3
- Izotova V., Petrov D., Pankratova K., Pospehov G. Research of Acoustic Characteristics and Physical and Mechanical Pro-perties of Quaternary Soils. Engineering and Mining Geophysics 2020 16th Conference and Exhibition, 14-18 September 2020, Perm, Russia. European Association of Geoscientists & Engineers, 2020. DOI: 10.3997/2214-4609.202051130
- Hussain G., Singh Y., Bhat G.M. et al. Geotechnical Characterisation and Finite Element Analysis of Two Landslides along the National Highway 1-A (Ladakh Region, Jammu and Kashmir). Journal of the Geological Society of India. 2019. Vol. 94, p. 93-99. DOI: 10.1007/s12594-019-1272-z
- Burlutskiy S., Glazounov V. Separation of the Structural Elements of the Landslide Based on Seismic-Geoelectric Model of Landslide Slopes. 13th Conference and Exhibition Engineering Geophysics 2017, 24-28 April 2017, Kislovodsk, Russia. European Association of Geoscientists & Engineers, 2017. DOI: 10.3997/2214-4609.201700410
- Fan X., Yang F., Siva Subramanian S. et al. Prediction of a multi-hazard chain by an integrated numerical simulation approach: the Baige landslide, Jinsha River, China. Landslides. 2020. Vol. 17, p. 147-164. DOI: 10.1007/s10346-019-01313-5
- Boualla O., Mehdi K., Fadili A. et al. GIS-based landslide susceptibility mapping in the Safi region, West Morocco. Bulletin of Engineering Geology and the Environment. 2017. Vol. 78 (4). DOI: 10.1007/s10064-017-1217-y
- Kumar N., Verma A.K., Sardana S. et al. Comparative analysis of limit equilibrium and numerical methods for prediction of a landslide. Bulletin of Engineering Geology and the Environment. 2018. Vol. 77, p. 595-608. DOI: 10.1007/s10064-017-1183-4
- Brideau M.-A., McDougall S., Stead D. et al. Three-dimensional distinct element modelling and dynamic runout analysis of a landslide in gneissic rock, British Columbia, Canada. Bulletin of Engineering Geology and the Environment. 2012. Vol. 71,
p. 467-486. DOI: 10.1007/s10064-012-0417-8 - Reyes-Carmona C., Galve J.P., Moreno-Sánchez M. et al. Rapid characterisation of the extremely large landslide threatening the Rules Reservoir (Southern Spain). Landslides. 2021. Vol. 18, p. 3781-3798. DOI: 10.1007/s10346-021-01728-z
- Graber A., Santi P., Meza Arestegui P. Constraining the critical groundwater conditions for initiation of large, irrigation-induced landslides, Siguas River Valley, Peru. Landslides. 2021. Vol. 18, p. 3753-3767. DOI: 10.1007/s10346-021-01767-6