Scientific substantiation and development of innovative processes for the extraction of zirconium and rare earth elements in the deep and comprehensive treatment of eudialyte concentrate
- Ph.D., Dr.Sci. Member of the Russian Academy of Sciences Academician N.V.Melnikov Research Institute of Comprehensive Exploration of Mineral Resources, RAS ▪ Orcid
Abstract
Based on a package of modern analysis methods, the influence of various acids and energy effects on the morphology, elemental composition, structural and chemical transformations of the mineral surface, and the efficiency of eudialyte concentrate leaching was studied. The mechanism and the optimal conditions and specific features of the destruction of eudialyte and rock minerals and the extraction of zirconium and REE under the influence of various acids, powerful nanosecond pulses, dielectric barrier discharge, electrochemical processing, mechanochemical activation and ultrasound were revealed. The mechanism of formation and the optimal conditions for the dispersion of silica gel, depending on the methods and parameters of energy effects, was theoretically and experimentally substantiated. A combined three-stage circuit of nitric acid leaching of eudialyte concentrate with ultrasonic treatment of the suspension, providing 97.1 % extraction of zirconium and 94.5 % REE, were scientifically substantiated and tested. The conditions for the selective deposition of zirconium and REE were theoretically and experimentally substantiated.
Introduction
Most rare and rare earth metals are the basis for the structural materials in electronics, space industry, green energy, and other products of the defence complex [1, 2].
Production of rare and rare earth elements is difficult due to their low content in ores, their presence in a dispersed state in other minerals, and the complex material composition of rare metal raw materials [3-5]. Such production should be organized considering world practice and the prospects for creating a complete processing cycle from extraction to industrial use [6-8].
Process flows for obtaining rare and REE (La – lanthanides, Y, Sc) from ores for each type of raw material are individual and usually include the following successive stages: obtaining metal concentrates using flotation and gravity-magnetoelectric enrichment circuits; decomposition of concentrates (roasting, fusion, leaching); obtaining pure chemical metal compounds (crystallization, sorption, extraction, ion exchange, chemical precipitation, etc.) and further metals (cementation, electrolysis, etc.) [9, 12]. Due to the similarity of REE properties, their selective separation is a complex processing problem [13-15]. Modern REE separation circuits are based on liquid extraction and ion exchange, as well as on selective oxidation with oxygen, chlorine, hydrogen peroxide and reduction with zinc, zinc and sodium amalgams [16-19].
In Russia, eudialyte ores of the Lovozerskoye deposit can be effectively involved in treatment for the production of rare and REE (reserves of Zr oxides – 300 Mt, REM – 60-70 Mt) [20, 21].
Eudialyte ores containing 25-27 % eudialyte, 52 % feldspar, loparite and nepheline, 20 % aegirine can be treated according to combined flotation-gravity-magnetoelectric enrichment circuits to obtain eudialyte and loparite concentrates [22, 23]. The resulting eudialyte concentrates at minimal radioactivity contain oxides of Si (54 %), Zr (11-13 %), REE (1.8-2.5 %), Hf (0.21-0.27 %), Ta (0.06-0.1 %), Nb and Ti (0.6-1 %), Sr (0.95-1.48 %) [24, 25].
The existing technologies for extracting zirconium and rare earth elements from eudialyte concentrates are based on their multistage decomposition by acids and alkalis [25, 26]. Due to the high content of silicon in the concentrate composition, a silica gel is formed in the acid leaching solution. The silica gel sorbs valuable components, significantly reducing the extraction of zirconium and REE into productive solutions and hindering their further filtration and extraction-sorption treatment [27-29].
In recent years, the solution to this problem has been achieved through the preliminary treatment of mineral suspensions with powerful energy effects (electrochemical treatment, ultrasound, treatment with high-intensity electromagnetic fields, mechanical activation, etc.), which provide both a modification of the chemical structure and morphological properties of eudialyte, destruction of silica gel, and intensification of leaching [30-32].
Methodology
Eudialyte concentrate had the following composition, %: ZrO2 – 11.5; REE – 2.5; SiO2 – 44.47; Na2O – 10.5; K2O – 1.37, TiO2 – 3.29; Fe2O3 – 5.2; Cl – 0.97; CaO – 5.42; SrO – 2.63; Al2O3 – 6.9; MnO – 1.66; SrO – 1.95; Nb2O5 – 0.94; MgO – 0.2; BaO – 0.15; other – 0.35. General view of the concentrate and particles of eudialyte are shown in Fig.1.
The concentrate also contained 10 % feldspars, nepheline, aegirine and their intergrowths, about 3 % twinned crystals of loparite-(Ce), golden-brown crystals of lamprophyllite. Secondary zeolites (natrolite, gonnardite) are noted in accessory quantities.
The concentrate was loosened with various acids and alkalis in a TOP120 INDUSTRIE autoclave and in a reactor that uses electrochemical, thermal, and ultrasonic effects on the mineral suspension [30].
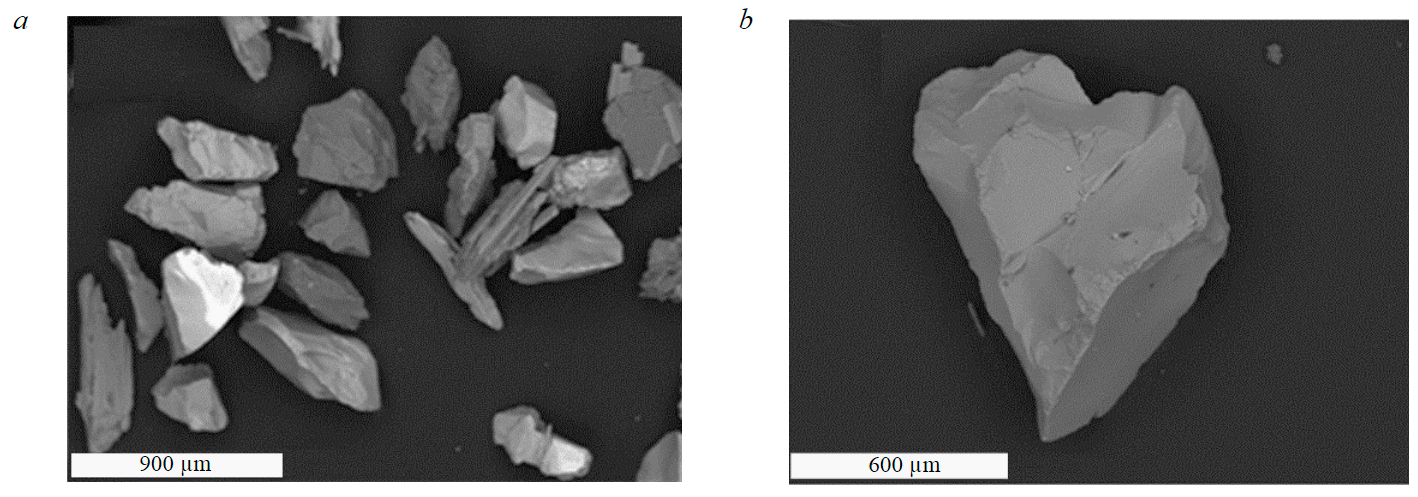
Fig.1. Micrographs of concentrate (a) and individual grains (b) of eudialyte
The sample material was ground in a MSHL-1 laboratory ball mill to a particle size of less than 40 µm. Preliminary mechanical activation (MA) of eudialyte concentrate samples was made in
a planetary centrifugal mill (PCM) brand LAIR-0.015 (NITU MISiS). Concentrate was treated with high intensity electromagnetic fields (high-altitude electromagnetic pulses, HEMP) and low-temperature plasma – dielectric barrier discharge (LTP-DBD) on experimental laboratory stands (IPKON RAS, “NPP FON” LLC). Technical parameters of HEMP: electric field intensity of the electrode system, E up to 107 V/m; pulse duration τp ~ 10-150 ns; pulse repetition frequency V = 100-1000 Hz; pulse voltage amplitude U up to 70 kV. Dielectric barrier discharge treatment parameters (LTP-DBD): duration of the pulse leading edge 250-300 ns; pulse duration 8 µs; voltage amplitude 20 kV; pulse repetition rate 16 kHz; treatment time 30 s; distance between electrodes 5 mm.
The suspension was treated by ultrasound using a disperser (MEF-15, MELFIZ) with a power of 600 W, operating frequency of 22±1.65 kHz, and oscillation amplitude of 25±5 μm.
The chemical and phase composition of the productive solutions of leaching, cake and silica gel was studied by inductively coupled plasma mass spectroscopy (ICP-MS) (ICPE-9000, “Shimadzu”), X-ray fluorescence analysis (XRF) (ARL ADVANT'X, “Thermo Scientific”), X-ray phase analysis (XRF) (diffractometer ADP-2, NPO “Burevestnik”). The morphology and elemental composition of the mineral surfaces were studied by scanning electron microscopy (SEM-EPMA) using a LEO 1420VP microscope equipped with an Oxford INCA Energy “Carl Zeiss” energy-dispersive microanalyzer. To record the IR spectra of eudialyte mineral particles, an IR-Affinity Fourier spectrometer “Shimadzu” and a DiffuseIR diffuse reflection attachment “PikeTechnologies” were used. X-ray photoelectron spectra of the surface of eudialyte particles were recorded using a Versa Probe II “ULVAC-PHI” spectrometer.
Discussion
Acid leaching of eudialyte concentrate
According to SEM-EPMA data, eudialyte, nepheline, and lamprophyllite react differently to acids, and the degree of dissolution (destruction) of their surface depends on the type of acid used. The shape of the particles of potassium feldspar, loparite, and aegirine does not change under the influence of acids.
According to the effectiveness of exposure to nitric acid, three groups of concentrate minerals were distinguished: highly soluble eudialyte and nepheline; partially leachable lamprophyllite and aqueous aluminosilicates K and Na; practically insoluble aegirine, loparite, and feldspars (skeleton aluminosilicates K, Na and Ca). XPS and IR spectroscopy showed that the dissolution of eudialyte concentrate with nitric acid occurs due to the destruction of minerals structure, destruction of the silicate skeleton, transition of Al, Na, and Mn cations and silicon into solution. In this case, silicon forms gel-like phases of SiO2∙nH2O silica gel type.
Under the influence of hydrochloric acid solution, the surface areas of eudialyte grains are actively dissolved with the penetration of the leaching solution into the bulk of the grains and the appearance of a series of deep, vertical, parallel cracks (Fig.2, a). Sulphuric acid (Fig.2, b) dissolves eudialyte more actively and evenly both from the surface and from the volume of particles, forming stable crystals of gypsum and celestite on the surface of undissolved minerals (Fig.3).
The most significant changes in the structural and chemical properties of the eudialyte surface are observed under the influence of a hydrochloric acid solution: the symmetry in the IR spectra changes and the spectral profile develops in the region of stretching vibrations of the Si-O-Si bonds (800-1350 cm–1) in the mineral structure. The revealed changes in the characteristics of the spectral curves are a consequence of the mineral skeleton distortion due to the removal of Na, Ca, Mg, etc. cations from the structural motifs of eudialyte.
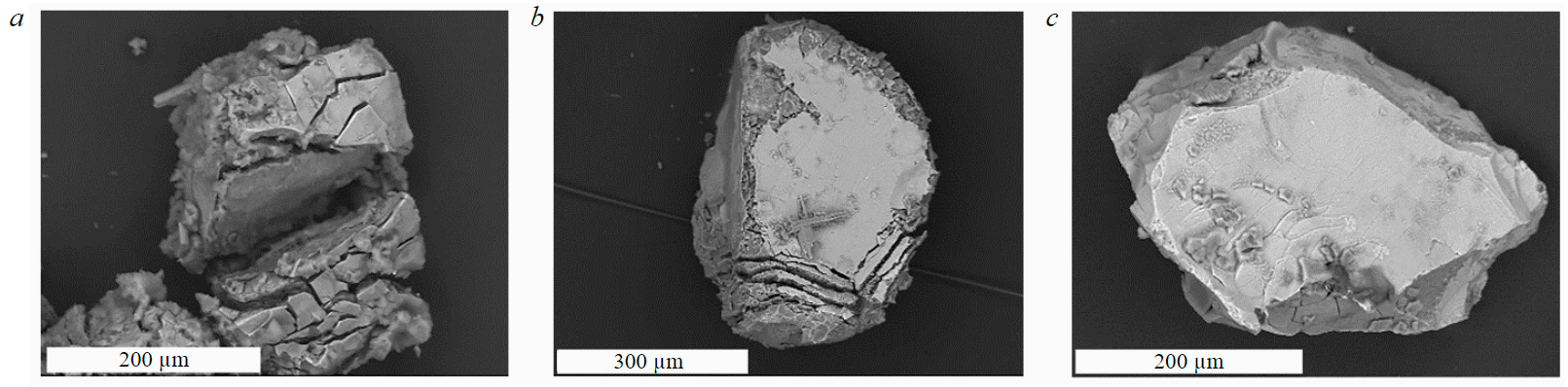
Fig.2. Eudialyte particles after leaching in (a) hydrochloric, (b) sulphuric, and (c) nitric acids
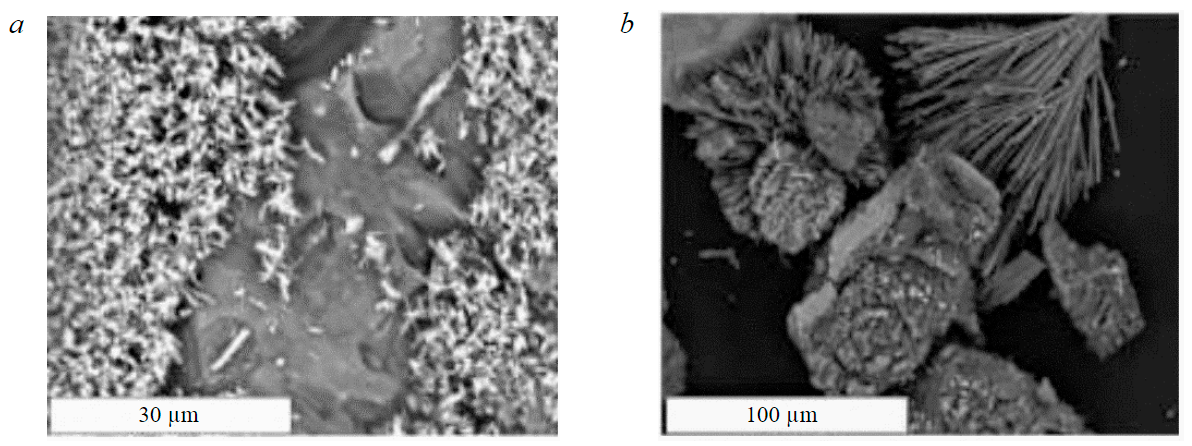
Fig.3. Celestine SrSO4 (a) and gypsum CaSO4·2H2O (b) formed on the surface of eudialyte concentrate mineral particles during leaching with sulphuric acid
XPS analysis of the elemental composition of the main elements of the eudialyte particles surface revealed its effective destruction, accompanied by the transfer of cations that are part of the mineral into solution: Al, Na, Ca, Mg, K, Ti, Mn, Fe, Sr, Zr. In descending order of the concentration of cations on the surface of the “original sample” of eudialyte, the action of acids can be arranged in the series HNO3 → H2SO4 → HCl. A decrease in the concentration, at.%, was revealed: Al – 2-28 times, Na – 3.6-61, Ca, Mg and Mn – 2.5-5, Ti – 3-9, Zr – 3-12 (Table 1).
Table 1
Composition of the surface layer of eudialyte particles (X-ray photoelectron spectroscopy data)
Acid |
Elemental composition, at. % |
ΣMe/Si |
||||||||||||||
N |
O |
S |
F |
Na |
Mg |
Ca |
Al |
Si |
K |
Ti |
Mn |
Fe |
Sr |
Zr |
||
No treatment |
0.6 |
60.0 |
n/t |
0.6 |
12.2 |
0.5 |
0.5 |
2.8 |
14.8 |
0.4 |
0.9 |
0.5 |
0.7 |
0.4 |
1.2 |
1.36 |
NHO3 |
1.9 |
66.2 |
n/t |
0.1 |
3.4 |
0.2 |
0.2 |
1.4 |
19.2 |
0.2 |
0.6 |
0.2 |
0.5 |
0.2 |
1.2 |
0.42 |
H2SO4 |
0.3 |
69.2 |
2.2 |
< 0.1 |
0.9 |
0.1 |
0.1 |
0.1 |
23.1 |
0.1 |
0.3 |
0.2 |
0.2 |
0.5 |
0.1 |
0.11 |
HCl |
0.2 |
70.2 |
n/t |
0.1 |
0.2 |
< 0.1 |
< 0.1 |
< 0.1 |
24.6 |
n/t |
0.1 |
< 0.1 |
0.1 |
n/t |
0.4 |
0.05 |
XRF established active dissolution of eudialyte concentrate by hydrochloric and sulphuric acids. The most effective dissolution of the eudialyte concentrate (the sample weight is reduced by 76 % of the initial value) and, accordingly, the maximum total extraction of Zr (91.5 %) and REE (82.5 %) is provided by sulphuric acid. When treated with nitric acid, 56 % of the mass of the concentrate passes into the solution with the extraction of Zr 76.9 % and REE 79.6 %, and in the case of using hydrochloric acid, 46 % of the mass of the concentrate passes into the solution with the extraction of Zr 83.91 % and REE 83.6 %.
The following content of silica gel in the solid precipitate of concentrate leaching was established: 45-65 % for nitric acid, 64 % for sulphuric acid, and 70.5 % for hydrochloric acid. These results are in good agreement with the volumes of silica gel in leaching solutions.
The highest volume percentage of silica gel after five days of settling is observed when using sulphuric acid – 63 %, for nitric acid – 55 %, hydrochloric acid – 48 %. After centrifugation of the solutions, the compaction of silica gel increases in 1.65-1.85 times.
Acid leaching of silicates [33, 34] revealed the interaction of silicic acid with sulphate ions through hydrogen bonds and with metal ions, for example, Al3+, according to the reactions presented in Fig.4. Based on these reactions, one can assume the formation of a large volume of silica gel with minimal losses of Zr in the case of using sulphuric acid (Fig.4, a) and higher losses of zirconium and other highly charged cations, characterized by a high ratio of charge to ionic radius, in the case of nitric acid (Fig.4, b).

Fig.4. Interactions of silicic acid molecules with sulphate ions: a – through hydrogen bonds; b – with metal ions (for example, Al3+)
Analysis of the elemental composition of productive leaching solutions established that the absolute (from the feed) losses of Zr and REE associated with silica gel during sulphuric acid leaching are about 26.9 and 70.2 %, respectively, while during nitric acid leaching, Zr and REE losses are 41.0 and 44.2 %, and the use of hydrochloric acid leads to a significant reduction in the loss of Zr and REE to 8.3 and 18.8 %.
The low losses of zirconium and REE during leaching with hydrochloric acid are determined by the minimum volume of the formed gel, which is probably due to the active transition into the solution of Ti cations interacting with silicic acid molecules, limiting the rate of polycondensation (Table 2). However, it is problematic to recommend the use of hydrochloric acid leaching in industrial conditions due to the high aggressiveness of hydrochloric acid to structural materials.
Table 2
Losses of valuable components with silica gel and their concentration in productive solutions
Acid |
Losses with gel, % |
Concentration, g/dm3 |
||
Zr |
ΣREM |
Zr |
ΣREM |
|
H2SO4 |
29.38 |
85.05 |
4.59 |
0.222 |
HCl |
9.94 |
22.47 |
5.04 |
0.999 |
HNO3 |
53.39 |
55.56 |
2.35 |
0.494 |
HNO3 (US) |
32.25 |
35.03 |
3.12 |
0.637 |
High losses of REE as a result of using sulphuric acid as a leaching agent are due to the precipitation of rare earths and titanium from the productive solution in the form of sulphates. Fig.5, 6 show lamellar rhombic intergrowths of crystals presumably REE2(SO4)3 and well-faceted cubic crystals of double calcium and titanium sulphates CaTi(SO4)3. The size of crystals of newly formed sulphates is 20 μm or less, which leads to the capture of a significant mass fraction of these phases by the silica gel, determines the high loss of REE in it and, accordingly, the low (12.3 %) extraction of ∑REE into the productive solution.
In addition, the X-ray phase analysis of silica gel samples during leaching of eudialyte concentrate with H2SO4, HCl, and HNO3 solutions revealed nano-fragments of the main minerals of the concentrate, as well as a number of newly formed weakly crystallized phases, such as sodium nitrate, sodium chloride, and gypsum.
Losses of zirconium and REE with gel are 29.4 and 85.1 % for sulphuric acid, 53.4, 55.6 % for nitric acid, and 9.9, 22.5 % for hydrochloric acid (Table 2). In [30], it was proposed to isolate valuable components from the cake by washing with water and precipitating Na2CO3.
After the removal of the gel by centrifugation, the relative losses of valuable components from the total extraction into the gel and solution were calculated (Table 2). The relative loss of valuable components with silica gel during leaching with nitric acid can be explained by the active sorption of Zr and REE cations due to their high charge.
The results of eudialyte concentrate leaching with nitric acid are close to those obtained with hydrochloric acid leaching of Zr 76.9 % and REE 79.6 %. In addition, compared with sulphuric acid, the loss of REE with silica gel is reduced in 1.6 times from 70.2 to 44 %.
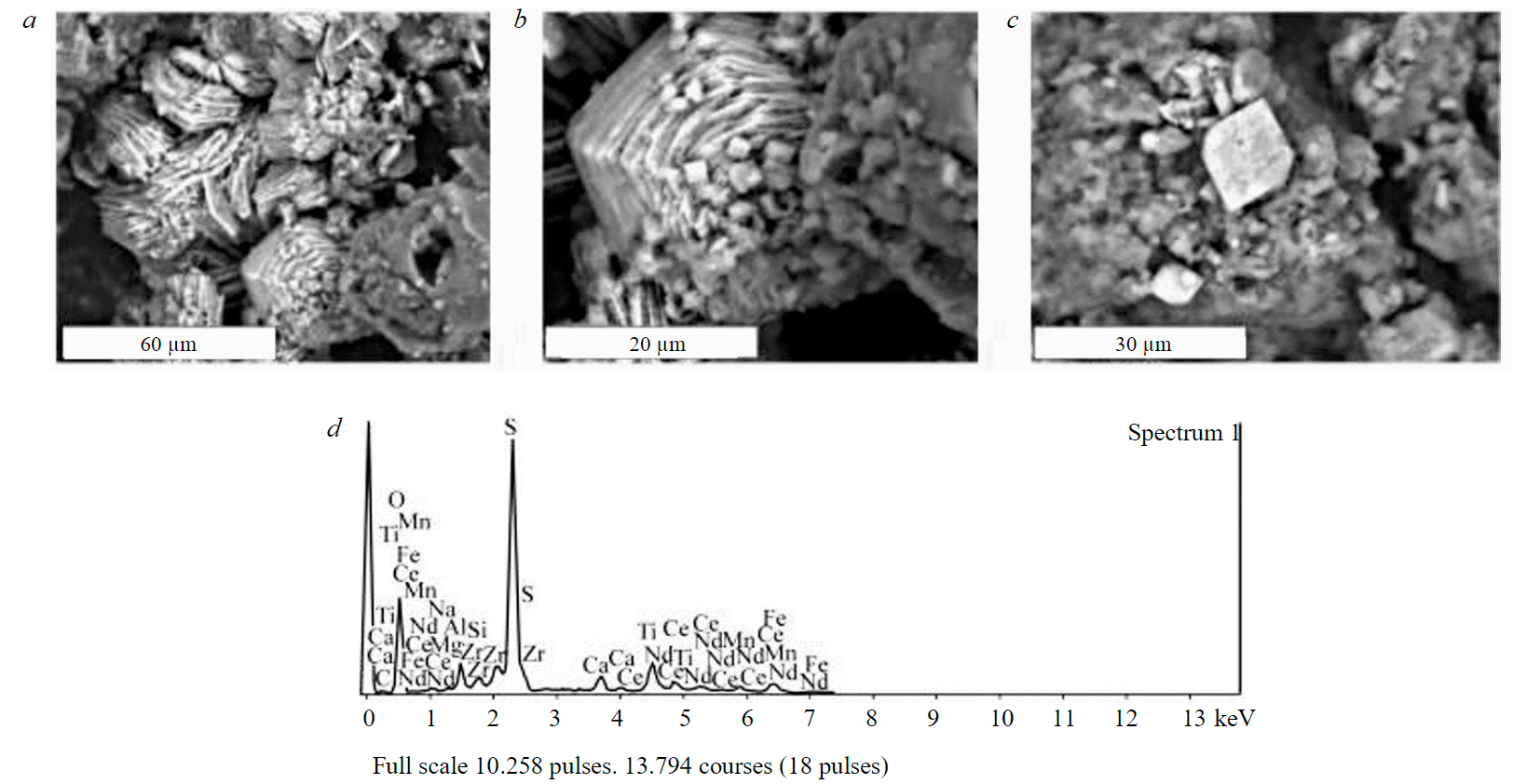
Fig.5. Crystals (a), intergrowths (b, c) and energy dispersive spectrum (d) of REE2(SO4)3
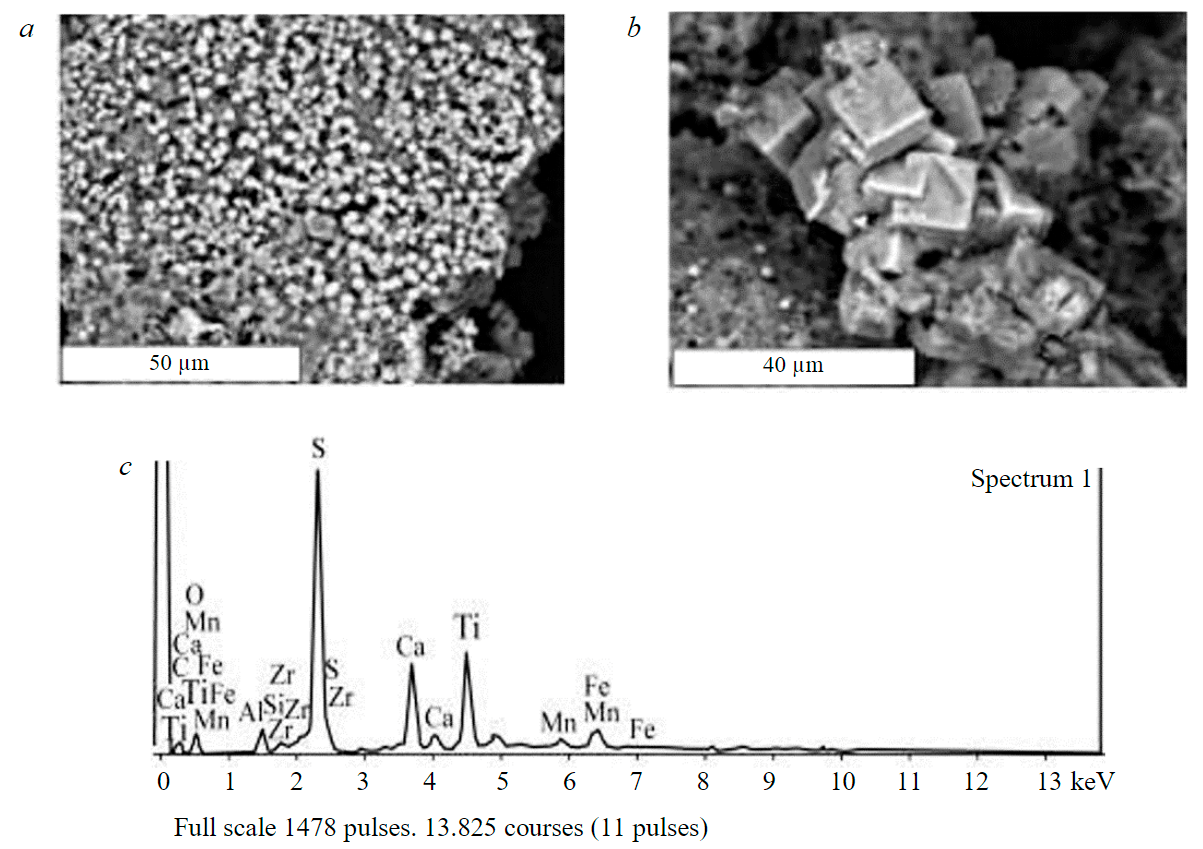
Fig.6. Crystals (a, b) and energy dispersive spectrum of CaTi (SO4)3 (c)
Thus, it was established that the use of nitric acid is the most economically and technologically feasible, since it provides high qualitative and quantitative indicators of eudialyte concentrate leaching and a reduction in REE losses with silica gel, and therefore further studies were carried out using nitric acid.
Energy effects on structural and chemical properties, phase composition of the main minerals of eudialyte concentrate and the efficiency of nitric acid leaching
Previous studies established the possibility of using energy effects for directed modification of the surface properties of minerals, selective disintegration, and intensification of loosening refractory concentrates [21, 35]. This work studied the energy impacts (ultrasound, low-temperature plasma, high power magnetic pulses, and electrochemical treatment) and preliminary mechanical activation (standard grinding and mechanical activation) of eudialyte concentrate on morphology, textural and structural features, material composition, formation intensity (destruction) of silica gel and the efficiency of valuable components extraction.
According to the data of X-ray phase and mineralogical analysis, eudialyte is the predominant phase in the studied samples of the feed unground concentrate. Lamprophyllite and loparite are present in a subordinate amount. Single signs of aegirine, nepheline, feldspars are noted [30].
After leaching of the feed concentrate under all modes, a decrease in the intensities of the main diagnostic peaks of eudialyte is observed, indicating its partial dissolution.
The study of the minerals surface using atomic force microscopy revealed the formation of a precipitate of silica gel on the surface of eudialyte as a result of exposure to nitric acid. The volume of silica gel on the surface of eudialyte decreases under energy impacts, mainly ultrasound (Fig.7, c). The surface of aegirine, feldspars, and loparite is not significantly affected by nitric acid and ultrasonic treatment.
Electrochemical treatment of the suspension leads to a significant reduction in the surface area of the gel-coated eudialyte. In terms of elemental composition, the gel-free areas of the mineral surface correspond to the original eudialyte; however, the quantitative content of ∑REE decreases significantly and is below the detection limit of an energy-dispersive spectrometer.
Ultrasonic treatment of the suspension promotes the removal of silica gel from the eudialyte surface and active cracking of the mineral grains, up to their complete destruction.
After leaching with preliminary MA, a decrease in the peak intensities of eudialyte and nepheline on the X-ray patterns of the samples is observed, confirming their active dissolution and amorphization of the surface. For aegirine and minerals of the feldspar group, these changes are less noticeable [24].
Consequently, all considered energy effects cause the formation of an inhomogeneous morphologically complex surface of particles, the destruction and removal of silica gel from the surface of minerals.
The ICP-MS analysis of the concentrate leaching cake established that the electrochemical treatment of the suspension makes it possible to increase the extraction of zirconium and ∑REE by 5.1-3.6 %. Ultrasonic treatment due to effective cleaning of the eudialyte surface from silica gel increases the extraction of ∑REE and zirconium by 28.9-43.9 % (Table 3).
Preliminary grinding of the concentrate to –40 µm class provides an increase in the extraction of REE and zirconium by 34.1-40.9 %. Mechanical activation of eudialyte concentrate leads to an increase in the extraction of REE and zirconium by 35.0-44.9 %. Ultrasonic treatment of the suspension after mechanical activation reduces the extraction by 3.6-5.7 %, which is due to the formation of an amorphous phase that absorbs ions and ionic groups from the solution.
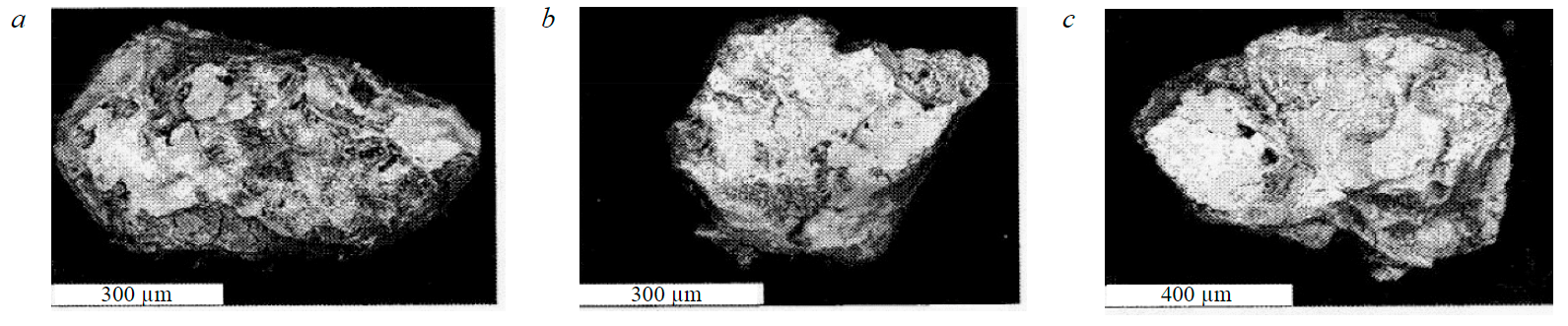
Fig.7. Eudialyte surface: a – after leaching; b, c – after leaching with electrochemical and ultrasonic treatment
Table 3
Extraction of zirconium and ∑REE depending on the type of energy impact
Type of grinding |
Energy impacts |
Extraction, % |
|
Zr |
∑REE |
||
No grinding |
No treatment |
12,5 |
18,2 |
No grinding |
Electrochemical treatment |
17,6 |
21,8 |
No grinding |
Ultrasonic treatment |
56,4 |
47,1 |
Standard grinding |
No treatment |
53,4 |
52,3 |
Standard grinding |
Electrochemical treatment |
75,8 |
64,8 |
Standard grinding |
Ultrasonic treatment |
76,8 |
68,5 |
Mechanical activation |
No treatment |
57,4 |
53,2 |
Mechanical activation |
Ultrasonic treatment |
53,8 |
47,5 |
High power electromagnetic pulses (HEMP, DBD) also have a significant destructive effect on the structural state of the eudialyte surface, which causes the activation of dissolution of mineral particles in nitric acid and increases the efficiency of acid leaching of eudialyte concentrate, which was evaluated on the basis of cake chemical analysis and calculation of leaching indicators considering the material balance (Table 4).
Table 4
Influence of parameters of high power electromagnetic pulse effects on the efficiency of leaching eudialyte concentrate
Treatment |
Extraction of elements, % |
Total extraction of REM, % |
|||||
Zr |
Ce |
Hf |
La |
Nd |
Y |
||
No treatment |
86.6 |
87.5 |
84.9 |
86.2 |
88.4 |
89.7 |
87.7 |
HEMP (30 s) |
89.9 |
88.4 |
89.9 |
87.2 |
89.9 |
92.4 |
89.5 |
HEMP (60 s) |
90.9 |
88.2 |
90.7 |
85.7 |
90.1 |
92.8 |
89.4 |
HEMP (90 s) |
90.2 |
87.6 |
89.5 |
85.8 |
89.4 |
92.3 |
88.9 |
DBD (30 s) |
90.6 |
87.9 |
89.4 |
85.9 |
89.9 |
92.8 |
89.2 |
In general, pre-treatment with HEMP made it possible to increase the extraction of zirconium into the productive leaching solution by 3.4-4.3 %; when using DBD, the extraction increased by 4.0 %. The maximum increase in the recovery of zirconium (4.3 %) was achieved as a result of concentrate treatment with HEMP for ttr = 60 s, which is comparable to the result obtained with a short-term (ttr = 30 s) action of a barrier discharge (4.1 %). The increase in the total extraction of rare earth elements was 1.2-1.8 %.
Consequently, the maximum intensification of the nitric acid leaching of the eudialyte concentrate is achieved by combining the preliminary grinding of the concentrate to –40 µm class with ultrasonic treatment of the suspension. This is explained by an increase in the specific surface area of eudialyte, cleaning of grains from amorphous phases (silica gel), and formation of additional inhomogeneities and defects on the surface of minerals, contributing to their more active dissolution.
Rational parameters of nitric acid leaching of eudialyte concentrate under conditions of energy impacts
To assess the most significant factors of energy impacts that affect the extraction of valuable components during nitric acid leaching of eudialyte concentrate, screening experiments were carried out using a mathematical model (Pluckett – Berman plan) [7].
Analysis of the obtained experimental data showed that the most significant factors affecting the extraction of zirconium are ultrasound, temperature, leaching time, grinding. Thus, the dependence of Zr extraction into a productive solution on the duration of ultrasonic impacts on a mineral suspension is described by a linear equation and reaches maximum values only with constant ultrasonic treatment of the suspension. Ultrasonic treatment of the suspension provides an increase in the extraction of zirconium by 8.5 %, ∑REE by 4.3 %, while reducing losses with silica gel to 29.4 %.
The increase in zirconium extraction is due to the fact that eudialyte particles intensely crack and destroy during the ultrasonic treatment of the suspension. In addition, the ultrasonic treatment in leaching the eudialyte concentrate prevents the formation of silica gel, leading to a decrease in its volume by 8.2 % from 55 to 46.8 vol.% Electrochemical treatment reduces the volume of silica gel by 3.5 % from 55 to 51.5 %.
Preliminary exposure to LTP does not lead to a significant decrease in the volume of the silica gel forming during leaching. The volume of the gel after settling is 51.4-55.9 vol.%. The total extraction of Zr and ∑REE slightly increases by 1-2.5 %, and their losses with silica gel decrease by 4.5-6 %.
Due to the high operating costs for concentrate grinding and the formation of a large amount of silica gel during leaching finely ground concentrate, pre-grinding the concentrate before the acid leaching process is impractical.
At the first stage of technology development, a two-stage process of eudialyte concentrate leaching was tested, which makes it possible to increase the extraction of zirconium by 9.6% (from 76.9 to 86.5), REE by 1.7 % (from 79.6 to 81.3). The total volume of the resulting silica gel increases by 10.3 % (from 55 to 65.3), which, accordingly, leads to an increase in the loss of Zr from 41 to 51.9 %, REE from 44.2 to 50.8 %. A further increase in the number of leaching stages from two to three provides a slight increase in the extraction of Zr and ΣREE into the productive solution: for Zr by 4.6 % from 86.5 to 91.1, for ΣREE by 1.9 % from 81.3 to 83.2, while the total volume of silica gel increases to 69.7 %. The data obtained (Table 5) confirm the presence in the undissolved residue after the third leaching stage of eudialyte concentrate under optimal conditions of an insignificant part of eudialyte, as well as feldspars, aegirine and loparite, practically insoluble in nitric acid, and, accordingly, the inexpediency of increasing the duration of nitric acid leaching of eudialyte concentrate over 3 hours (three stages).
Table 5
Mass fraction of elements in the feed (N 1) eudialyte concentrate and after the third stage of its acid leaching (N 2)
Concentrate |
Si |
ZrO2 |
TiO2 |
∑SrO, BaO, Ca |
Mn |
Ta2O5 |
Nb2O5 |
∑REM |
H2O |
Other |
N 1 |
22.7 |
8.34 |
3.99 |
5.43 |
1.42 |
0.074 |
0.94 |
2.50 |
0.38 |
47.79 |
N 2 |
27.0 |
3.15 |
4.83 |
2.56 |
0.53 |
0.13 |
1.45 |
3.13 |
2.71 |
48.36 |
Due to the fact that the most intensive dissolution of eudialyte and, accordingly, the formation of silica gel occurs at the first and second stages of leaching, the work explored the possibility of reducing the loss of valuable components by reducing the duration of the first two stages while increasing the duration of the third leaching stage (while maintaining the overall duration of the process). Reducing the 1st and 2nd stages of leaching from 60 to 40 min and increasing the 3rd stage to 100 min leads to an increase in the extraction of Zr by 5.1 % (from 91.1 to 96.2), REE by 9.6 % (from 83.2 to 92.8). The volume of the silica gel increases by only 1.6 %, while the losses of Zr and REE remain at the same level (41.5 and 44.7 %, respectively). Additional ultrasonic treatment at the 1st stage of leaching contributes to an increase in the extraction of Zr by 0.9 % (from 96.2 to 97.1), REE by 1.7 % (from 92.8 to 94.5) and a significant reduction in the volume of the forming silica gel (from 56.6 to 47 %), which leads to a reduction in the loss of valuable components to 31.4 and 33.8 %, respectively.
Thus, the developed three-stage process of nitric acid leaching of eudialyte concentrate with ultrasonic treatment of the suspension at the first stage is optimal and provides the extraction of zirconium up to 97.1 % and REE up to 94.5 %.
Extraction of zirconium and REE from productive solutions of eudialyte concentrate leaching
In the existing processes for the treatment of productive solutions of REE, both classical reactions of selective precipitation and modern methods based on complexation and ion exchange with various types of reagents (tributyl phosphate, quaternary ammonium salts, carboxylic acids, etc.) are used to isolate and selectively separate valuable components.
Since the concentrations of zirconium and REE in productive solutions of eudialyte concentrate leaching, as a rule, do not exceed 1-10 g/dm3, a study was made of the possible successive chemical precipitation of zirconium and REE from the productive solution. This method, which is characterized by process simplicity and low operating costs, makes it possible to obtain Zr and REE precipitates that are easily soluble in slightly acidic solutions and suitable for further treatment. The REE concentration in the initial productive solution is presented in Table 6.
Table 6
REE concentrations in the initial productive solution
Element |
Concentration, mg/dm3 |
Element |
Concentration, mg/dm3 |
Sc |
0.4 |
Gd |
23.1 |
Y |
138.0 |
Tb |
4.1 |
La |
76.7 |
Dy |
23.2 |
Ce |
182.6 |
Ho |
4.8 |
Pr |
22.5 |
Er |
12.9 |
Nd |
66.1 |
Tm |
2.0 |
Sm |
20.1 |
Yb |
11.1 |
Eu |
6.7 |
Lu |
1.6 |
Separation of Zr and REE with concomitant neutralization of productive solutions was carried out in two stages. At the first stage, nitric acid neutralisation with the release of Zr with Al and Fe admixtures was ensured by increasing the pH values of the solution from 0.74 to 4.8-5.0 using calcium carbonate. At the second stage, precipitation of REE was achieved by increasing the pH of the solution to 6.0-6.4 using sodium carbonate and sodium hydroxide. The reagents were supplied and the solution was mixed for 2 h. The precipitates were separated from the solutions by centrifugation at a relative centrifugal force (RCF) of a laboratory centrifuge of at least 500 g for 15 min, then the precipitates were washed with distilled water and dried until the mass stabilized.
An analysis of the dependence of Zr, Al, Fe extraction into a solid precipitate on the pH values of the medium under isothermal conditions (20 °C) showed that the efficiency of elements precipitation grows with an increase in pH, and at a pH value of 4.0, zirconium is extracted into precipitate by 99.9 %, aluminium by 100 %, iron by 43.8 %. It was found that the extraction of other admixtures (Na, Mn, Sr, Ti, Mg and K) does not exceed 15-35 %, and the total loss of rare earth elements is about 8 %.
A further increase in pH to values of 4.8-5.0 leads to a sharp increase in REE losses up to 40 % due to co-precipitation with Zr, which is probably associated with the onset of their precipitation in the form of carbonates. It is advisable to carry out subsequent chemical precipitation of rare-earth compounds using more soluble compounds, such as sodium carbonate and sodium hydroxide (Table 7).
Results of the second stage of the experiment showed that at a specific consumption of sodium carbonate of 6 g/dm3 (pH 6.1), the maximum extraction of 95.5 % REE into the solid phase is achieved. When the pH value of the productive solution is increased to 6.0 with sodium hydroxide, the maximum extraction of REE into the precipitate is 59.1 %, which confirms the extraction of a significant amount of REE into the solid phase in the form of carbonates.
Table 7
Extraction of REE into precipitate depending on the pH of the solution and type of reactant
Reactant |
Na2CO3 |
NaOH |
||
Consumption, g/dm3 |
3.0 |
6.0 |
9.0 |
0.01 |
pH |
6.0 |
6.1 |
6.4 |
6.0 |
REE extraction, % |
90.8 |
95.5 |
95.2 |
59.1 |
Thus, the analysis of the research results confirmed the high efficiency of chemical sequential precipitation of zirconium and REE from a productive solution of nitric acid leaching of eudialyte concentrate.
An increase in the pH value of the initial productive solution at the 1st stage of precipitation with calcium carbonate to 4.0 ensures the extraction of zirconium into the solid phase of 99.95 % with the associated extraction of admixture metals: aluminium – 100 %, iron – 43.8 %. In this case, the total loss of REE with zirconium product does not exceed 8 %. The subsequent increase in the pH value of the productive solution with sodium carbonate to 6.1 ensures the extraction of REE into the solid phase of 87.5 %, with a total extraction from the solution of 95.5 %.
Conclusion
A package of modern methods of optical, analytical electron, scanning probe microscopy, IR-Fourier spectroscopy, XPS, and other methods of physicochemical analysis enabled to obtain new scientific data on the morphology, structure, chemical and phase composition of the surface of minerals, the formation mechanism and destruction of silica gel. Efficient methods were developed for the selective separation of zirconium and rare earth elements from productive solutions of acid leaching of eudialyte concentrate.
References
- Kryukov V.A., Tolstov A.V., Samsonov N.Yu. Strategic importance of rare earth metals in the world and in Russia. Vserossiiskii ekonomicheskii zhurnal. 2012. N 2 (11), p. 5-16 (in Russian). DOI: 10.30680/ECO0131-7652-2012-11-5-16
- Samsonov Н., Semyagin И. World and Russian Rare Earth Market Survey. ECO Journal. 2018. N 44(2), p. 45-54 (in Russian). DOI: 10.30680/ECO0131-7652-2014-2-45-54
- Chanturia V.A. Scientific substantiation and development of innovative approaches to integrated mineral processing. Gornyi Zhurnal. 2017. N 11, p. 7-13 (in Russian). DOI: 10.17580/gzh.2017.11.01
- Goodenough K.M., Schilling J., Jonsson E. et al. Europe’s Rare Earth Element Resource Potential: An Overview of REE Metallogenetic Provinces and Their Geodynamic Setting. Ore Geology Reviews. 2016. Vol. 72. Part 1, p. 838-856.
DOI: 10.1016/j.oregeorev.2015.09.019 - Johnsen O., Ferraris G., Gault R. et al. The nomenclature of eudialyte-group minerals. The Canadian Mineralogist. 2003. Vol. 41. N 3, p. 785-794. DOI: 10.2113/gscanmin.41.3.785
- Khokhulya M.S., Gerasimova L.G., Nikolaev A.I. New Technological Solutions for Preparing and Processing Perovskite. Trudy Kolskogo nauchnogo tsentra RAN. 2018. Vol. 9. N 2-1, p. 196-200 (in Russian). DOI: 10.25702/KSC.2307–5252.2018.9.1.196-200
- Cohen J., Cohen P., West S.G., Aiken L.S. Applied Multiple Regression/Correlation Analysis for the Behavioral Sciences. 3rd Edition. New York: Routledge, 2002, p. 536. DOI: 10.4324/9780203774441
- Rastsvetaeva R.K., Chukanov N.V., Pekov I.V. et al.New data on the isomorphism in eudialyte-group minerals. 1. Crystal chemistry of eudialyte-group members with Na incorporated into the framework as a marker of hyperagpaitic conditions. Minerals. 2020. Vol. 10. Iss. 7. N 587. DOI: 10.3390/min10070587
- Mudruk N.V., Korovina Yu.V., Nikolaev A.I., Safonova L.A. Extraction of Rare-Earth Elements from the Solutions after Perovskite Concentrate Decomposition by Nitric Acid. Trudy Kolskogo nauchnogo tsentra RAN. Vol. 9. N 2-1, p. 323-327 (in Russian). DOI: 10.25702/KSC.2307–5252.2018.9.1.323-327
- Balinski A., Wiche O., Kelly N. et al. Separation of rare earth elements from contaminants and valuable components by in situ precipitation during the hydrometallurgical processing of eudialyte concentrate. Hydrometallurgy. 2020. Vol. 194. N 105345. DOI: 10.1016/j.hydromet.2020.105345
- Ma Y., Stopic S., Friedrich B. Hydrometallurgical Treatment of an Eudialyte Concentrate for Preparation of Rare Earth Carbonate. Johnson Matthey Technology Review. 2019. Vol. 63. N 1, p. 2-13. DOI: 10.1595/205651318X15270000571362
- Voßenkaul D., Birich A., Müller N. et al. Hydrometallurgical processing of Eudialyte Bearing Concentrates to Recover Rare Earth Elements Via Low-Temperature Dry Digestion to Prevent the Silica Gel Formation. Journal of Sustainable Metallurgy. 2016. Vol. 3, p. 79-89. DOI: 10.1007/s40831-016-0084-2
- Lokshin E.P. Sorption Conversion is a Perspective Method of Processing of Rare Earth Raw Materials. Trudy Kolskogo nauchnogo tsentra RAN. 2018. Vol. 9. N 2-1, p. 154-159 (in Russian). DOI: 10.25702/KSC.2307-5252.2018.9.1.154-159
- Balomenos E., Davris P., Deady E. et al.The EURARE project: development of a sustainable exploitation scheme for Europe’s rare earth ore deposits. Johnson Matthey Technology Review. 2017. Vol. 61, p. 142-153. DOI: 10.1595/205651317X695172
- Hoshino M., Sanematsu K., Watanabe Y. REE mineralogy and resources. Handbook on the Physics and Chemistry of Rare Earths. New York: Elsevier, 2016. Vol. 49, p. 129-291. DOI: 10.1016/bs.hpcre.2016.03.006
- Gedgagov E.I., Tarasov A.V., Giganov V.G., Lunkova M.A. Development of innovative sorption-extraction technology for obtaining high-purity (99.99 %) rare-earth metal compounds when processing ultimate concentrates (on the example of the light group). Tsvetnye Metally. 2017. N 8, p. 50-55 (in Russian). DOI: 10.17580/tsm.2017.08.07
- Rusakov I.Yu., Buynovskiy A.S., Sofronov V.L., Ageeva L.D. Decomposition of zirconium and hafnium fluorides using sublimation-sorption method. Izvestiya. Non-Ferrous Metallurgy. 2016. Vol. 3, p. 20-27 (in Russian). DOI: 10.17073/0021-3438-2016-3-20-27
- Dezhi Qi. Chapter 1. Extraction of Rare Earths from RE Concentrates. Hydrometallurgy of Rare Earths. Amsterdam: Elsevier, 2018, p. 1-185. DOI: 10.1016/B978-0-12-813920-2.00001-5
- Davris P., Stopic S., Balomenos E. et al. Leaching of rare earth elements from eudialyte concentrate by suppressing silica gel formation. Minerals Engineering. 2017. Vol. 108, p. 115-122. DOI: 10.1016/j.mineng.2016.12.011
- Bogatyreva E.V., Chub A.V., Ermilov A.G., Khokhlova O.V. The efficiency of alkaline-acid method of complex leaching of eudialyte concentrate. Part 1. Tsvetnye Metally. 2018. N 7, p. 57-61 (in Russian). DOI: 10.17580/tsm.2018.07.09
- Bogatyreva E.V., Chub A.V., Ermilov A.G., Khokhlova O.V. Efficiency of the alkaline-acid method of complex leaching of eudialyte concentrate. Part 2. Tsvetnye Metally. 2018. N 8, p. 69-74 (in Russian). DOI: 10.17580/tsm.2018.08.09
- Balinski A., Atanasova P., Wiche O. et al. Recovery of REEs, Zr (Hf), Mn and Nb by H2SO4 leaching of eudialyte concentrate. Hydrometallurgy. 2019. Vol. 186, p. 176-186. DOI: 10.1016/j.hydromet.2019.04.007
- Chanturia V.A., Chanturia E. L., Minenko V.G., Samusev A.L. Acid leaching process intensification for eudialyte concentrate based on energy effects. Obogashchenie rud. 2019. N 3, p. 29-36. DOI: 10.17580/or.2019.03.05
- 24. Chanturia V.А., Minenko V.G., Samusev А.L. et al. lnfluence exerted by ultrasound processing on efficiency of leaching, structural, chemical, and morphological properties of mineral components in eudialyte Concentrate. Journal of Mining Science. 2018. Vol. 54. Iss. 2, p. 285-29. DOI: 10.1134/S1062739118023641
- Ma Y., Stopic S., Gronen L., Friedrich B. Recovery of Zr, Hf, Nb from eudialyte residue by sulfuric acid dry digestion and water leaching with H2O2 as a promoter. Hydrometallurgy. 2018. Vol. 181, p. 206-214. DOI: 10.1016/j.hydromet.2018.10.002
- Saveleva I.L. The rare-earth metals industry of Russia: Present status, resource conditions of development. Geography and Natural Resources. 2011. Vol. 32. Iss. 1, p. 65-71. DOI: 10.1134/S1875372811010112
- Artamonov A.V., Smirnova D.N., Smirnov N.N., Ilyin A.P. Extraction of rare earth elements from solid waste of production of phosphoric acid followed by sorption on cation exchange resins. Izvestiya Vysshikh Uchebnykh Zavedenii. Khimiya Khimicheskaya Tekhnologiya. 2017. Vol. 60. N 10, p. 87-93 (in Russian). DOI: 10.6060/tcct.20176010.5571
- Turanov A.N., Karandashev V.K., Khvostikov V.A. Extraction of Rare-Earth Elements(III) with Mixtures of 1-Phenyl-3-Methyl-4-Benzoyl-5-Pyrazolone and Phosphoryl-Containing Podands. Russian Journal of General Chemistry. 2019. Vol. 89. N 9, p. 1830-1835. DOI: 10.1134/S1070363219090172
- Ma Y., Stopic S., Gronen L. et al. Neural network modeling for the extraction of rare earth elements from eudialyte concentrate by dry digestion and leaching. Metals – Open Access Metallurgy Journal. 2018. Vol. 8. Iss. 4. N 267. DOI: 10.3390/met8040267
- Chanturia V.А., Minenko V.G., Samusev А.L. et al. The mechanism of influence exerted by integrated energy impacts on intensified leaching of zirconium and rare earth elements from eudialyte concentrate. Journal of Mining Science. 2017. Vol. 53. Iss. 5, p. 890-896. DOI: 10.1134/S1062739117052921
- Chanturia V.A., Bunin I.Z., Ryazantseva M.V. et al. Modification of structural, chemical and process properties of rare metal minerals under treatment by high-voltage nanosecond pulses. Journal of Mining Science. 2018. Vol. 53. Iss. 4, p. 718-733. DOI: 10.1134/S1062739117042704
- Panayotova M., Panayotov V.An electrochemical method for decreasing the concentration of sulfate and molybdenum ions in industrial wastewater. Journal of Environmental Science and Health, Part A. Toxic/Hazardous Substances and Environmental Engineering. 2004. Vol. 39. 1, p. 173-83. DOI: 10.1081/ese-120027376
- Matveev V.A. Mayorov D.V., Solovev A.V. Integrated technology of sulfuric acid treatment for eudialyte concentrate. Tsvetnye Metally. 2018. N 1, p. 20-27 (in Russian). DOI: 10.17580/tsm.2018.01.02
- Vaccarezza V., Anderson C. Beneficiation and leaching study of norra kärr eudialyte mineral. Metal Technology. The Minerals, Metals & Materials Series. Cham: Springer, 2018, p. 340. DOI: 10.1007/978-3-319-72350-1_4
- Jha M.K., Kumari A., Panda R. et al. Review on hydrometallurgical recovery of rare earth metals. Hydrometallurgy. 2016. Vol. 165. Part 1, p. 2-26. DOI: 1016/j.hydromet.2016.01.003