Development of a hydrocarbon completion system for wells with low bottomhole temperatures for conditions of oil and gas fields in Eastern Siberia
- 1 — Ph.D., Dr.Sci. Head of Department Saint Petersburg Mining University ▪ Orcid ▪ Elibrary ▪ Scopus ▪ ResearcherID
- 2 — Postgraduate Student Saint Petersburg Mining University ▪ Orcid ▪ Elibrary ▪ Scopus
Abstract
The paper presents the results of investigations on the influence of low bottomhole temperatures in the intervals of productive formations on the technological properties of solutions used for drilling and completion of wells in order to determine the possibility of increasing gas recovery coefficient at the field of the “Sila Sibiri” gas pipeline. The analysis of technological measures determining the quality of the productive horizon drilling-in was carried out. It was found out that the dispersion of bridging agent in the composition of the hydrocarbon-based drilling mud selected from the existing methods does not have significant influence on the change in the depth of filtrate penetration into the formation in conditions of low bottomhole temperatures. The main reason for the decrease in the near-bottomhole zone permeability was found out – the increase in plastic viscosity of the dispersion medium of the hydrocarbon-based drilling mud under the influence of low bottomhole temperatures. A destructor solution for efficient wellbore cleaning from hydrocarbon-based solution components in conditions of low bottomhole temperatures was developed. The paper presents the results of laboratory investigations of hydrocarbon-based drilling mud and the developed destructor solution, as well as its pilot field tests. The mechanism of interaction between the destructor solution and the filter cake of the hydrocarbon-based drilling mud ensuring the reduction of the skin factor in the conditions of the geological and hydrodynamic structure of Botuobinsky, Khamakinsky and Talakhsky horizons of the Chayandinskoye oil and gas condensate field has been scientifically substantiated.
Introduction
Promising resource base for the development of Russian fuel and energy industry are the fields of Eastern Siberia and the Arctic shelf, where more than 60 % of the Earth strategic oil and gas resources are concentrated. Rather large oil and gas fields have been discovered here: in Irkutsk region – Kovyktinskoye gas condensate field, Verkhnechonskoye and Dulisminskoye oil and gas condensate fields; in Krasnoyarsk region – Yurubcheno-Tohomskoye oil field and Sobinskoye gas field; in Republic of Sakha (Yakutia) – Talakanskoye oil field, Srednebotuobinskoye oil and gas field, Srednevilyuiskoye, Srednetyungskoye and Chayandinskoye oil and gas condensate fields. The gas and gas condensate fields of Eastern Siberia, unlike the fields of the Senomanian system in Western Siberia, are complex objects with abnormally low thermobaric conditions.
Often, during the operation of wells at the East Siberian fields, the problems related to insufficiently high gas recovery coefficient arise [1, 2]. This indicator is caused by hydrodynamic influence of drilling mud (DM) during initial drilling-in of the object, as well as by selection of technology and types of development fluids.
For a more detailed study of this issue, let us consider technical and technological solutions used in developing the Chayandinskoye oil and gas condensate field (ChOGCF). The field is located in the Lenskiy district of Yakutia and is the base for the formation of the Yakutsk gas production center and the resource base for the “Sila Sibiri” gas pipeline [3]. Geology of the Chayandinskoye OGCF has specific features, the geological section of the operation area is characterized by the Lower Proterozoic formations of the crystalline foundation and Vendian, Cambrian, Jurassic and Quaternary deposits of sedimentary cover [4]. The main role in the structure of the sedimentary cover is played by terrigenous-carbonate Vendian deposits and halogen-carbonate Cambrian formations.
The productive formations confined to the Botuobinsky, Khamakinsky and Talakhsky horizons are characterized by complex structure of natural reservoirs, the formations of which are represented by different permeability and porosity properties (PPP) [5]. According to petrophysical investigations the range of reservoir properties for the Botuobinsky horizon is extensive and is characterized by, porosity from 26 % (medium-grained sandstones) to a few percent (clay siltstones) and high permeability in the medium-grained sandstones up to 6000·10–15 m2, for siltstones – not higher than 10·10–15 m2. The Khamakinsky horizon is characterized by porosity of 23 to 1 percent and permeability of 4500-1.1·10–15 m2. Talakhsky horizon has porosity of 22-23 to a few percent and permeability of 2000·10–15 to 0.5·10–15 m2. According to analysis of PPP distribution in the sediments drilled-in by the most productive wells, the Botuobinsky horizon has the best filtration characteristics [6-8]. The share of high-permeable reservoirs decreases from the Botuobinsky to the Khamakinsky, then to the Talakhsky horizon, and the content of low-permeable reservoirs increases. The geological structure of ChOGCF hydrocarbon deposits is complicated, formation-type, lithologically and tectonically screened, characterized by abnormally low formation pressure (12.0-13.5 MPa, which is 15-30 % lower than the hydrostatic pressure) and formation temperature (8-12 °С) [9, 10].
Serious complication in development of fields in Eastern Siberia, ChOGCF in particular, is well drilling in salt deposits, as drilling in these intervals is associated with significant difficulties – formation of caverns and ledges, wellbore collapses [4, 11, 12]. Application of widespread water-based saline solutions does not exclude cavern formation caused by temperature gradient “bottomhole – wellhead”. Hydrocarbon-based solutions (HBS) are used as flushing fluids for primary drilling-in of gas-condensate and gas intervals. Hydrocarbon-based flushing fluids are widely used in Russian and foreign practice for drilling and completion of wells, which ensures successful well drilling in complicated geological conditions [9, 13, 14]. This is proved by works of many Russian and foreign scientists [15]. The reason for selecting HBS is that the hydrocarbon phase is neutral with respect to the penetrated rocks, including salts and clays. It is especially difficult to drill-in formations by long horizontal wells, because natural reservoir properties of rocks decrease with depth [16-18]. Application of water-based drilling fluids in these conditions leads to deterioration of permeability in the near-bottomhole zone (NBHZ) and necessity for a large volume of works on inflow stimulation [19-21].
Methodology of the research
At present, invert-emulsion drilling mud based on vegetable oil is used as a basic drilling fluid for drilling wells at the ChOGCF, the main part of which consists of a mixture of fatty acids and their ethyl esters [22-24]. The absence of refined oil products in the composition of the drilling mud allows reducing considerably the fire hazard of the system and all its components; the flashpoint in the closed crucible of the organophilic base exceeds 150 °С. The field data show that the mud has a number of advantages over its modern counterparts: it has high lubricating and inhibiting ability; does not have water in the filtrate; helps achieving maximum productivity at drilling-in hydrophobic reservoirs; has minimal negative environmental impact due to its composition based on vegetable oils.
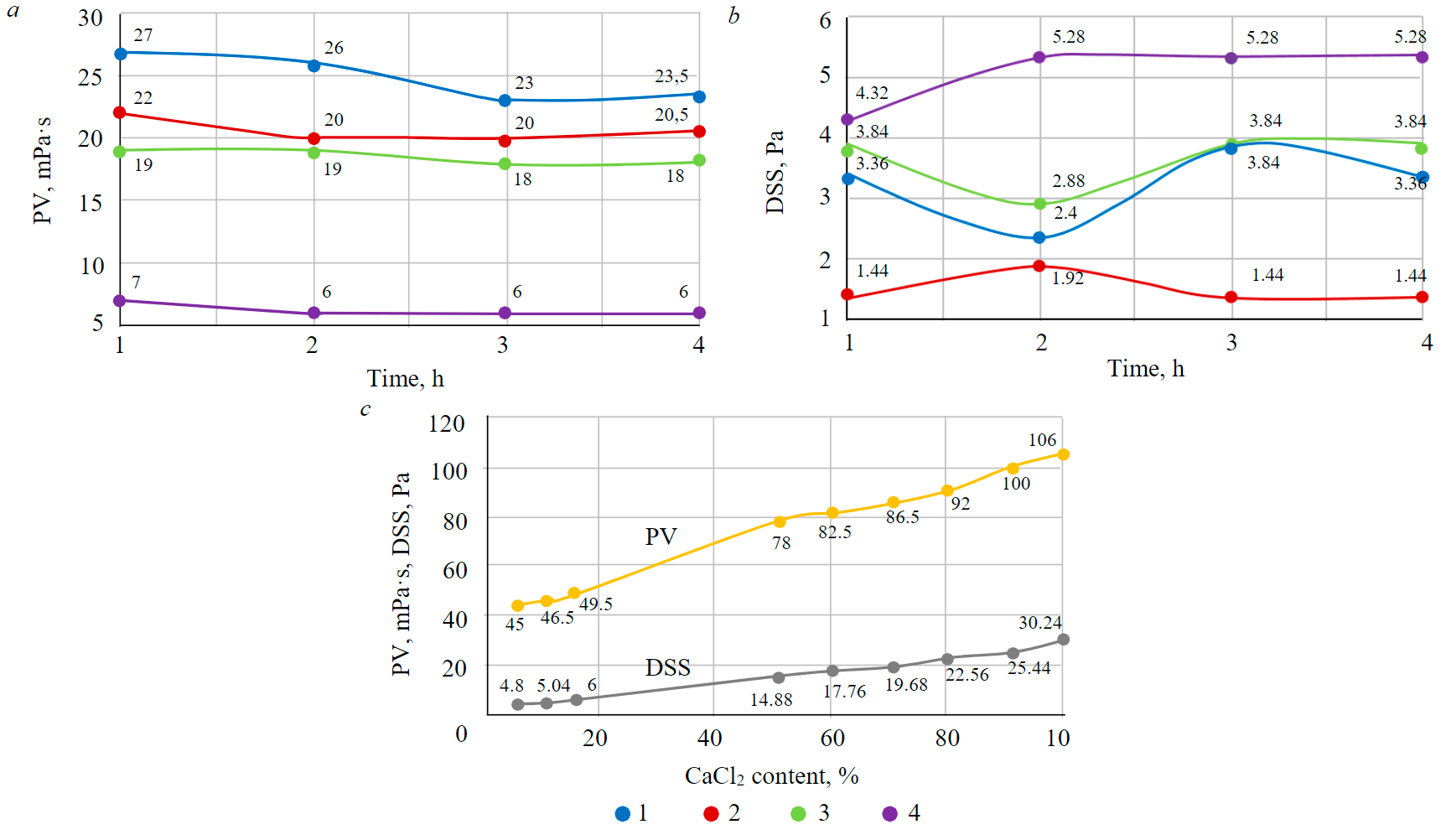
Fig.1. HBS plastic viscosity (a) and dynamic shear stress (b) dependencies on time at different salt contents (1 – 5 %; 2 – 10 %; 3 – 15 %; 4 – 50 %) and on CaCl2 content (c)
In order to evaluate the efficiency of muds based on fatty acids and their natural esters and well completion system, a number of investigations are carried out to determine the effect of temperature on changes in viscosity and physical and mechanical properties. All experimental investigations of HBS were carried out on the laboratory equipment of the Well Drilling Department and Scientific Centre “Arktika” of Saint Petersburg Mining University. For investigation of physical and mechanical properties of the considered drilling mud the equipment of FANN and OFITE manufacturers is used, taking into account thermobaric conditions according to the approved methods of experimental research, as well as in accordance with GOST, ISO, API standards.
The geological section of the considered field is represented by salt deposits, that is why the first stage of the research was the investigation on compatibility of HBS and mineralized water (composition: CaCl2 – 50 %; MgCl2 – 25 %; NaCl – 25 %), as well as HBS and calcium chloride solution (ρ = 1050 kg/m3) in the ratio 1:0.5. This concentration is chosen according to formation water salinity of the ChOGCF.
The graphs in Fig.1, a, b show that the plastic viscosity (PV) decreases slightly for the first three hours. Then there is a stabilisation of the parameters. Meanwhile, dynamic shear stress (DSS) decreases for the first two hours at concentrations of 5 and 15 %, increases at concentrations of 10 and 50 %, and then it reaches initial values measured one hour after the beginning of investigation. The dependencies in Fig.1, c show, that with increasing salt concentration PV and DSS of drilling mud grows linearly. With the rise of salt concentration from 0 to 50 %, there is an increase of HBS filtration from 3.2 to 62 cm3/30 min. The results of the experiments determine the efficiency of the selected drilling mud and its resistance to salt aggression in the conditions of low bottomhole temperatures.
The analysis of field and laboratory data revealed that the applied HBS contains 10 µm solid phase represented by micromarble as a weighting agent, which, taking into account complex thermodynamic conditions and PPP heterogeneity of Botuobinsky, Khamakinsky, Talakhsky productive horizons during drilling-in leads to uneven depth of filtrate penetration into reservoir zone and formation of a bridged, impermeable screen in productive formations and, consequently, an increase in skin factor. In order to form a filtration cake, the drilling mud should contain solid particles of a certain size, which will facilitate shallow penetration, closing pores and creating a filter cake [25, 26]. In this regard, based on the petrophysical properties of the productive horizons, it is feasible to divide the productive intervals into two objects of investigation – highly permeable Botuobinsky and medium-permeable Khamakinsky and Talakhsky productive horizons for quality selection of fractional size for weighting agent of the drilling mud and further optimal control of its technological properties.
Based on field geophysical well investigations (GWI), it was established that after treatment of productive formation with process fluid systems (destructors) based on diesel fuel, polyatomic spirits or stable gas condensate, a part of drilling mud filter cake on the well walls is not subject to complete destruction. Prolonged static interaction of hydrocarbon systems with productive formation fluid leads to additional increase of viscosity and, consequently, to difficulty of their mechanical and chemical removal from pore space of the reservoir. It should be noted that the presence of swelling packers behind the filter part of the liner does not allow complete replacement and cleaning of the near-wellbore zone from the HBS residue during well operation.
To reduce the skin factor in productive intervals, the development of a completion system
(a destructor solution) that effectively removes organic hydrocarbons from the productive formation and increases well flow rates is required. Because the investigated drilling mud is composed of nonpolar compounds, the complete destruction of the filter cake requires the development of a completion system which is a mixture of nonpolar solvents.
The second stage of the investigation includes the development of the destructor solution and filtration experiments on interaction of hydrocarbon-based drilling mud with it. The developed “WC-1” destructor solution consists of a composition of two non-polar solvents – white spirit and sulfate turpentine in concentrations 75 and 25 % by mass. The mechanism for chemical destruction of filter cake by the well completion system is presented by the following formula:
White spirit promptly dissolves organic compounds, vegetable fats, rubbers, oils and epoxy esters, effectively degreases metal surfaces, evaporates quickly, has low toxicity, does not form deposits and is stable as a chemical compound [27-29]. Sulfate turpentine, in its turn, not only increases the efficiency of dissolving oils with white spirit, but also increases permeability to the volumetric phase of oil and dissolution product removal. To prove the efficiency of destructor solution “WC-1” its physical, mechanical and chemical properties were studied, as well as the degree of near-bottomhole zone cleaning from the organic constituent of hydrocarbon-based drilling mud (Table 1).
Table 1
Effect of thermal aging on technological indicators of completion fluids
Parameter |
Value before thermal aging |
Value after thermal aging at 20 °С in 24 h |
Measuring temperature, °С |
8 |
20 |
Density after sieving at the mesh 1-1.5 mm, kg/m3 |
1100 |
1100 |
Specific viscosity, s |
15 |
16 |
Filtration, cm3/30 min |
50 cm3/50 s |
50 cm3/50 s |
Filter cake, mm |
Absent |
Absent |
рН, units |
– |
– |
PV, mPa×s |
2.5 |
2.5 |
DSS, Pa |
0.48 |
0 |
SSS 1/10, dPa |
0 |
0 |
Jet losses (VSL), cm3/30 min |
0 |
0 |
Table 1 shows that the plastic viscosity after 24 h and an increase in temperature from 8 to 20 °С remained unchanged at 2.5 mPa×s. At the same time, the dynamic shear stress decreased from 0.48 to 0 Pa. The obtained data indicate that the developed destructor solution in its structure is a non-Newtonian fluid, and under the effect of increasing temperature high-molecular weight chemical compounds in its medium are destructed.
The investigation included testing for solubility of rock (sodium) salt in the medium of the completion fluid. Based on the results of the experiments (comparison of the solution density before and after the salt introduction, comparison of the salt mass before introduction into the solution and that collected on the surface of the mesh when the solution was sieved) the inhibiting ability of the system to prevent dissolution of the sodium salt was determined. The results are shown in Table 2.
Table 2
Results of investigation on the effect of NaCl on the completion fluid
Parameter |
Initial solution properties |
Value after salination |
Density after sieving at the mesh 1-1.5 mm, kg/m3 |
|
|
Specific viscosity, s |
15 |
16 |
Filtration (10 µm disc), cm3/30 min |
50 cm3/50 s |
50 cm3/50 s |
Filter cake, mm |
Absent |
Absent |
рН, units |
– |
– |
PV, mPa×s |
2 |
1.2 |
DSS, Pa |
0 |
0.432 |
SSS 1/10, dPa |
1/1 |
1/1 |
Mass of salt residue, g |
100 |
91 |
The investigation showed that the plastic viscosity of the completion system after salination decreased from 2 to 1.2 mPa×s, the specific viscosity increased from 15 to 16 s and the dynamic shear stress increased from 0 to 0.432 Pa, satisfying the requirements of its application in a saline formation.
To determine the coefficients of permeability change for natural rock samples by kerosene after their interaction with drilling mud and “WC-1” destructor solution, filtration investigations were conducted in accordance with geological and physical features of Botuobinsky horizon development at ChOGCF in thermobaric conditions, similar to formation conditions [7, 30, 31]. The result of laboratory investigations was determination of initial permeability recovery coefficient of rock samples by kerosene after modeling of primary drilling-in process by formation damage evaluation system FDES-645 (Coretest Systems Corporation) (Fig.2). Then the final permeability of rock sample after treatment with destructor solution was determined. Formation conditions of laboratory filtration experiments were as follows: repression during simulation of primary drilling-in 3 MPa, filtration rate (flow rate) 5 cm3/min, formation temperature 8 °С, formation pressure 12 MPa, kerosene viscosity 1.014 mPa∙s.
The results of filtration investigations on the impact of HBS and the developed destructor solution on filtration properties of reservoir rocks of Botuobinsky horizon at ChOGCF: rock sample permeability by kerosene before DM injection 69.01·10–3 μm2; coefficient of relative permeability change after DM injection with constant pressure/destructor –71.4/–32.0 %; maximum pressure gradient for the filtration beginning of the kerosene after DM injection with constant pressure/destructor 109.42/25.60 atm/m
The permeability recovery coefficient after the DM application was –71.4. The application of the developed destructor solution shows positive dynamics at injection and curing for 4-5 h, the permeability recovery coefficient according to the experiment was 32.0 % (after DM –71.4 %)
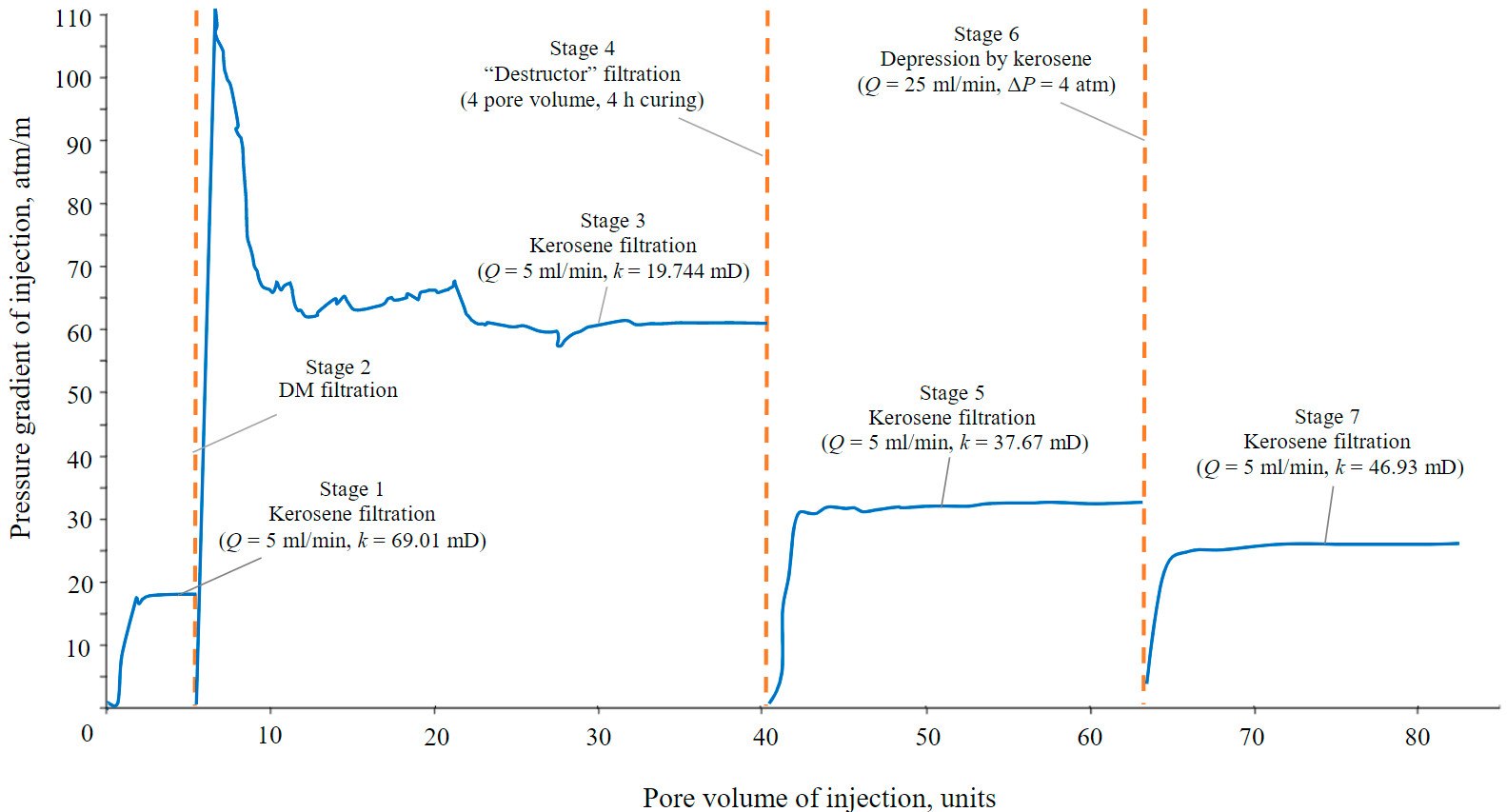
Fig.2. Dependence of pressure gradient of kerosene injection on pore pumping volume in the simulation of the primary drilling-in process using drilling mud and “WС-1” destructor solution
To determine the depth of penetration for HBS filtrate into the gas reservoir after the rock sample PPP recovery investigation and according to Vickers method of solids diameter selection, experiments were conducted (Table 3). Based on these experiments, different sizes (dispersion) of bridging agent solid particles were selected. Fig.3 shows an example of rock samples tomography of Botuobinsky, Khamakinsky and Talakhsky horizons on SkyScan 1173 tomograph for 25 µm fraction. The results obtained showed that the size of the solid phase in the composition of the investigated HBS does not cause the significant change in the penetration zone of the filtrate. The studied solution is an inverse emulsion consisting largely of fatty acids and their natural esters tending to solidify with decreasing temperature. Its physical and mechanical properties were studied. After measuring the plastic viscosity of the drilling mud, it was revealed that the complex thermodynamic conditions (from 8 to 12 °С in the zone of productive formation) of the given geological object lead to a sharp increase in the viscosity of the drilling mud (Fig.4)
Table 3
Results of determining the depth of filtrate penetration into the rock sample depending on the dispersion of bridging agent
Horizon |
Petrophysical properties |
Solid phase dispersion, μm |
||||||
Permeability |
Porosity, % |
5 |
10 |
25 |
50 |
100 |
150 |
|
Depth of filtrate penetration, mm |
||||||||
Botuobinsky |
3-7 D |
25-26 |
< 5 |
< 5 |
< 10 |
< 15 |
< 15 |
< 15 |
Khamakinsky and Talakhsky |
20-150 mD |
22-23 |
< 3 |
< 3 |
< 3 |
< 3 |
< 3 |
< 3 |
Figure 4 shows that the plastic viscosity at 7 °C reaches 16 mPa·s. Decrease in temperature by 1 °С leads to increase in viscosity by 3.81 mPa·s and increase in hydrodynamic pressure at borehole bottom.
The depth of filtrate penetration depends on filtration rate, time of formation stimulation, and one of the key factors is viscosity of drilling mud filtrate. The next stage of the research was to determine filtration zone depending on plastic viscosity of drilling mud with temperature change (ΔT = 7-50 °С). The calculations were carried out according to the formula:
where Rw – well radius, m; Vf – filtration rate of the DM liquid phase, m/s; Т – repression time during initial drilling-in of the formation, s; m – reservoir porosity, units; η – DM plastic viscosity, mPa·s.
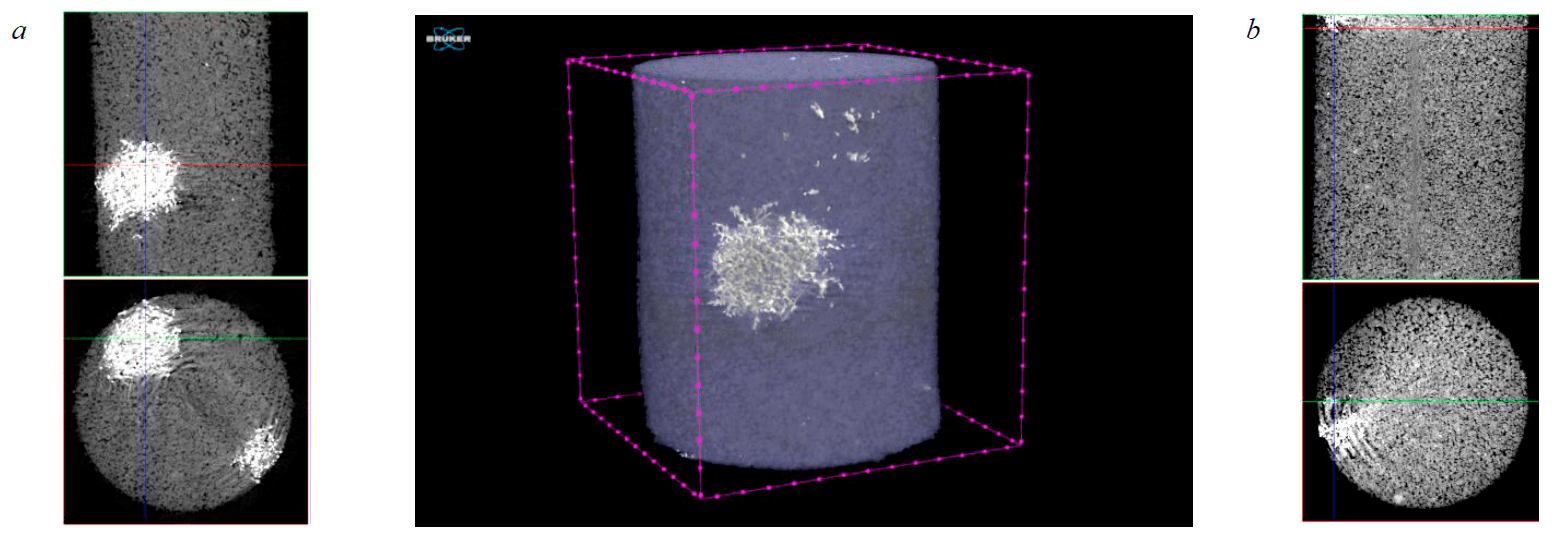
Fig.3. Results of tomography for rock samples of Botoubinsky (a) and Khamakinsky with Talakhsky (b) productive horizons after exposure by HBS
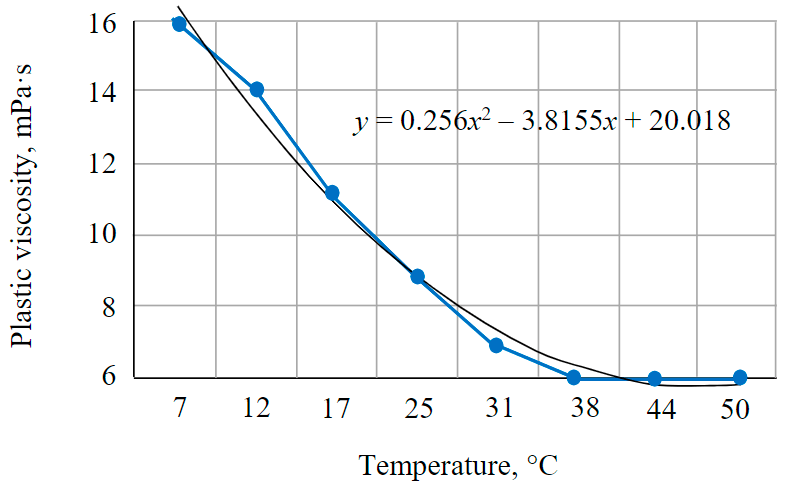
Fig.4. Variation of the plastic viscosity of a drilling mud based on fatty acids and their natural esters depending on temperature
The results of the plastic viscosity investigations (Table 4) indicate that the penetration zone of the filtrate in the pore reservoirs at low bottomhole temperatures is determined by the viscosity properties of the dispersion medium.
Table 4
Results of a plastic viscosity investigation depending on temperature variation
Temperature, °С |
Plastic viscosity, mPa·s |
Depth of filtrate penetration, mm |
7 |
16 |
76 |
12 |
14 |
75 |
17 |
11 |
75 |
25 |
9 |
74 |
31 |
7 |
74 |
38 |
6 |
74 |
44 |
6 |
74 |
50 |
6 |
74 |
The pilot tests of “WС-1” at ChOGCF showed that the technology of well cleaning with application of developed hydrocarbon completion system is carried out in the following way. Tripping is carried out by coiled tubing through the tubing string to the borehole bottom (Fig.5) [10, 32]. Then the destructor is injected by the volume, equal to the length of the tubing and annular space between coiled tubing and internal cavity of slotted filter [33]. A device with concentrically arranged nozzles “jet blaster” is attached at the bottom of the assembly. The device produces an additional hydro-wave effect [34-36]. The coiled tube is lifted and flushed with the “WC-1” destructor solution.
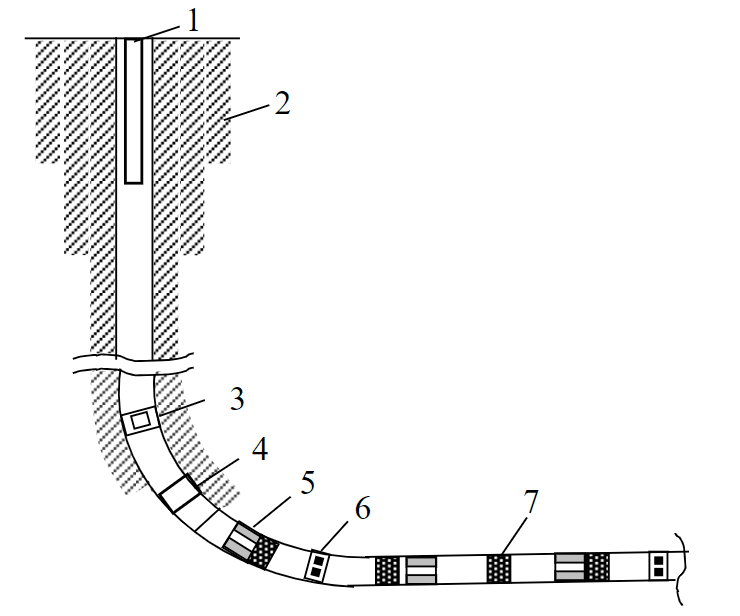
Fig.5. Well configuration with a horizontal ending 1 – tubing; 2 – well design; 3 – drillable and retrievable plug packer with check valve; 4 – uncemented liner mount; 5 – oil-swelling packer; 6 – multistage cementing coupling; 7 – slotted filter
Analysis of the results in Table 5 and Fig. 6 shows a high efficiency for treatment of filter and tubing inside surface with “WС-1” hydrocarbon completion system using “jet blaster” rotating nozzle. Wellhead pressures increase and skin factor decrease from +4.9 to +2.55 are observed. Decrease in hydraulic resistance to gas flow is revealed. Thus, hydraulic parameters of the well lift are changed, flowing part of the lift and filter part of the bottomhole formation zone are cleaned from contamination of the organic component of the drilling mud. The results of field geophysical investigations of wells show the increase of working intervals by 20 m.
Table 5
Results of gas-dynamic investigations of the well
Diaphragm, mm |
Pressure, ata |
Wellhead t, оС |
ΔР,kg/cm2 |
Q, thousand m3/day |
|
tubing |
DIKT |
||||
After drilling before treatment by “WC-1” |
|||||
9 |
90.3 |
90 |
–7 |
7.1 |
129.7 |
10 |
85.1 |
84.9 |
–7 |
9.9 |
148.5 |
11 |
82.6 |
82.3 |
–8 |
11.5 |
172.5 |
14 |
71.5 |
70.8 |
–10 |
16.3 |
231.7 |
Рst |
99.8 |
||||
Skin factor |
+4.9 |
||||
After treatment by “WC-1” “jet blaster” |
|||||
9 |
91.89 |
91.69 |
–0.8 |
5.47 |
122.7 |
10 |
89.74 |
89.30 |
5.5 |
7.12 |
145.0 |
11 |
86.66 |
85.87 |
0.5 |
9.01 |
172.1 |
14 |
78.03 |
75.94 |
–3.4 |
14.72 |
244.7 |
Рst |
99.64 |
||||
Skin factor |
+2.55 |
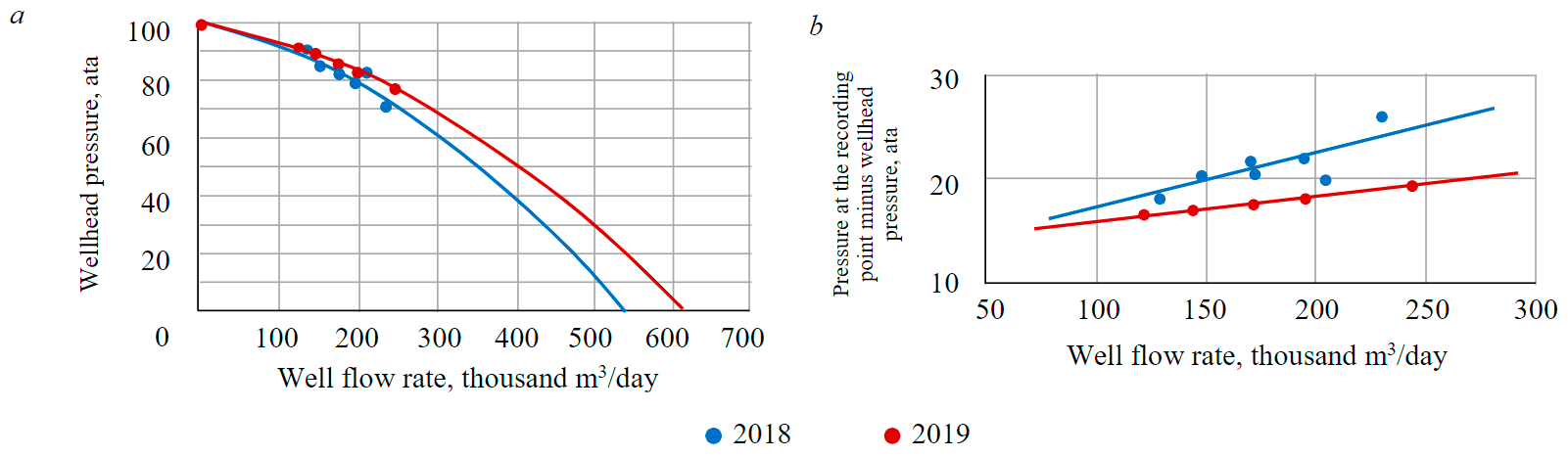
Fig.6. Well productivity changes depending on wellhead pressures before (a) and after (b) treatment by the “WC-1” destructor solution
Conclusion
Feasibility of using HBS for drilling wells at ChOGCF was theoretically substantiated and experimentally proved. The laboratory tests of drilling mud based on fatty acids and their natural esters determined its efficiency in the intervals of low bottomhole temperatures from 8 to 12 °С.
Theoretical and experimental data about substantial difference of permeability and porosity of reservoir rocks in productive horizons allowed scientifically proving and confirming the necessity of their division into two objects of the primary drilling-in during well drilling – highly permeable Botu-obinsky horizon (permeability 3-7 D, porosity from 26 % to a few %) and medium-permeable Khamakinsky and Talakhsky horizons (permeability 20-150 mD, porosity 22-23 %) in order to investigate the influence of solid phase dispersion on the filtrate penetration depth. It is established that the increase of filtrate penetration depth into the pores of the gas formation reservoir is caused by the increase of plastic viscosity of the hydrocarbon drilling mud dispersion medium based on fatty acids under the influence of anomalous low bottomhole temperatures. The absence of influence of the fractional composition of bridging agent selected from the existing methods in the composition of reverse emulsions based on vegetable oil and a mixture of fatty acids and their natural esters on the changes in the filtration zone was established.
Hydrocarbon completion system was developed, the component composition of which includes a combination of two non-polar solvents based on light hydrocarbons – white spirit and sulphate turpentine in concentrations of 75 and 25 %. The system allows cleaning the well from the organic content of HBS, represented mainly by a mixture of fatty acids and their natural esters.
The mechanism for dissolution of filter cake of hydrocarbon-based drilling mud represented by a solidified emulsion of fatty acids by a destructor solution is scientifically substantiated. As a result of this interaction, the “fatty acid-fatty acid” bonds in the solid state are destroyed, and stronger bonds are formed in soluble solvates “fatty acid-alkane” in the case of white spirit, or “fatty acid-terpene” in the case of turpentine. This results in a decrease in the skin factor from +5 to +2 in the Botuobinsky, Khamakinsky and Talakhsky horizons of the ChOGCF.
Field trials of the developed completion system, conducted at two wells of the ChOGCF, showed high efficiency.
References
- Bergenov S.U., Chernov O.S., Zipi M.G. Methodology for Assessing the Expected Starting Flow Rates of Horizontal Wells in Gas And Gas Condensate Fields. Bulletin of the Tomsk Polytechnic University. Geo Assets Engineering. 2020. Vol. 331. N 3, p. 207-212 (in Russian). DOI: 10.18799/24131830/2020/3/2563
- Burova I.A. Paleogeographic Reconstruction of the Vendian-Cambrian Carbonate Deposits of the Western Slope of the Nort-hern Part of the Nepa-Botuoba Anteclise. Neftegasovaya geologiya. Teoriya i practika. 2014. Vol. 9. N 4, p. 9 (in Russian). DOI: 10.17353/2070-5379/42_2014
- Litvinenko V. The Role of Hydrocarbons in the Global Energy Agenda: The Focus on Liquefied Natural Gas. Resources. 2020. Vol. 9. Iss. 5. N 59. DOI: 10.3390/resources9050059
- Vorobev V.S., Klinovaya Ya.S. Causes of salination in terrigenous rocks within the Verkhnechonskoye field (Eastern Siberia). Gazovaya promyshlennost. 2017. N 4, p. 36-42 (in Russian).
- Davydov A.V., Pogretckii A.V., Smirnov O.A. et al. Chayandinskoye Field is the Project of New Technologies Implementation in East Siberia. Perm Journal of Petroleum And Mining Engineering. 2017. N 2, p. 113-128 (in Russian). DOI: 10.15593/2224-9923/2017.2.2
- Burakova S.V., Izyumchenko D.V., Minakov I.I. et al. Problems of developing thin oil rims of gas-condensate deposits in Eastern Siberia (the example of the Botuobinsky deposit in the Chayandinskoye OGCF). Vesti gazovoi nauki: Problemy resursnogo obespecheniya gazodobyvayushchikh raionov Rossii do 2030 goda. 2013. N 5 (16), p. 124-133 (in Russian).
- Ryzhov A.E., Krikunov A.I., Ryzhova L.A., Kanunnikova N.Yu. Refinement of the geological model of the Chayandinskoye oil and gas condensate field. Vesti gazovoi nauki: Aktualnye voprosy issledovanii plastovykh sistem mestorozhdenii uglevodorodov. 2011. N 1 (6), p. 132-145 (in Russian).
- Ryzhov A.E. Types and properties of terrigenous Vendian reservoirs of the Chayandinskoye field. Vesti gazovoi nauki: Aktualnye voprosy issledovaniya plastovykh sistem mestorozhdenii uglevodorodov. 2013. N 1 (12), p. 145-160 (in Russian).
- Kryuchkov V.E., Medvedev A.G. Lithological, facies and geodynamic conditions for the formation of Vendian deposits in the Chayandinskoye field. Vesti gazovoi nauki: Problemy resursnogo obespecheniya gazodobyvayushchikh raionov Rossii do 2030 g. 2012. N 1 (9), p. 202-207 (in Russian).
- Litvinenko V.S., Dvoinikov M.V. Methodology for determining the parameters of drilling mode for directional straight sections of well using screw downhole motors. Journal of Mining Institute. 2020. Vol. 241, p. 105-112. DOI: 10.31897/PMI.2020.1.105
- Nifantov V.I., Melnikova E.V., Melnikov S.A. Peculiarities of oil and gas well development under various rock and geological conditions. Moscow: Gazprom VNIIGAZ, 2012, p. 38 (in Russian).
- 12. Liu T., Leusheva E.L., Morenov V.A. et al. Influence of polymer reagents in the drilling fluids on the efficiency of deviated and horizontal wells drilling. Energies. 2020. Vol. 13. N 18, p. 1-16. DOI: 10.3390/en13184704
- Kryuchkov V.E., Penzin A.A. Prospects for increasing proven hydrocarbon reserves at the Chayandinskoye oil and gas condensate field. Vesti gazovoi nauki: Problemy resursnogo obespecheniya gazodobyvayushchikh raionov Rossii. 2016. N 1 (25),
p. 34-39 (in Russian). - Fadeev D., Ivanov S. Features of the walking mechanism of a floating platform autonomous modular complex for the extraction and processing of peat raw materials. Scientific and Practical Studies of Raw Material Issues: Proceedings of the Russian-German Raw Materials Dialogue. London: Taylor & Francis Group, 2020, p. 239-243. DOI: 10.1201/9781003017226
- Litvinenko V.S., Bowbrick I., Naumov I.A., Zaitseva Z. Global guidelines and requirements for professional competencies of natural resource extraction engineers: Implications for ESG principles and sustainable development goals. Journal of Cleaner Production. 2022. N 338, p. 1-9. DOI: 10.1016/j.jclepro.2022.130530
- Koval M.E. Impact of Oil-Based Mud Preparation Methods on the Main Properties of the Drilling Mud. Neft. Gaz. Novatsii. 2020. N 3, p. 30-36 (in Russian).
- Koteleva N., Buslaev G., Valnev V., Kunshin A. Augmented Reality System and Maintenance of Oil Pumps. International Journal of Engineering. 2020. Vol. 33. Iss. 8, p. 1620-1628. DOI: 10.5829/ije.2020.33.08b.20
- Mardashov D.V., Islamov Sh.R., Nefedov Yu.V. Specifics of well killing technology during well service operation in complicated conditions. Periodico Tche Quimica. 2020. Vol. 17. N 34, p. 782-792. DOI: 10.52571/PTQ.v17.n34.2020.793_P34_pgs_769_781.pdf
- Leusheva E., Morenov V., Tabatabaee Moradi S. Effect of Carbonate Additives on Dynamic Filtration Index of Drilling Mud. International Journal of Engineering. 2020. Vol. 33. N 5, p. 934-939. DOI: 10.5829/IJE.2020.33.05B.26
- Behrens S. Oil-coated bubbles in particle suspensions, capillary foams, and related opportunities in colloidal multiphase systems. Current Opinion in Colloid & Interface Science. 2020. Vol. 50. N 101384. DOI: 10.1016/j.cocis.2020.08.009
- Dmitriev M., Ponomarenko A., Klachkov A. Thermophysical Validation of the Technology of Asphalt-Resin-Paraffin Deposit Removal from Well Casings in Continuous High-Viscosity Oil Production Condition. Chemical and Petroleum Engineering. 2019. Vol. 55. Iss. 7, p 627-631. DOI: 10.1007/s10556-019-00671-0
- Ishbaev G., Dilmiev M., Khristenko А., Mileyko А. The Use of Fractional Calcium Carbonate in the Invert-Emulsion Drilling Fluid to Reduce Formation Damage. Burenie i neft. 2012. N 3, p. 40-43 (in Russian).
- Islamov K.M. Drilling Fluids Influence on Change of Reservoir Properties. Construction of Oil and Gas Wells on Land and Sea. 2015. N 6, p. 18-20 (in Russian).
- Krylov V.I., Kretsul V.V., Medentsev S.V. Contemporary Technological Fluids for Well Completion and Work-Over. Part 1. Construction of Oil and Gas Wells on Land and Sea. 2015. N 1, p. 36-44 (in Russian).
- Artamonov V.Yu., Ganiev S.R., Kuznetsov Yu.S. Modern Ideas about Formation of Colmatage Layer and Mud Cake. Construction of Oil and Gas Wells on Land and Sea. 2015. N 5, p. 29-32 (in Russian).
- Akhmetova V.M. New Effective Chemical Destructors Eliminating Reservoir Polymeric Contamination (Blockade) During Well Primary Uncovery and Overhaul. Construction of Oil and Gas Wells on Land and Sea. 2011. N 6, p. 35-37 (in Russian).
- Jha P.K., Mahto V., Saxena V.K. Study the rheological and filtration properties of oil-in-water emulsion for its application in oil and gas well drilling. Journal of Petroleum Engineering and Technology. 2013. Vol. 3. N 3, p. 25-30. DOI: 10.37591/jopet.v3i3.2220
- Kambulov E.Yu., Myazin T.O.Issue of drilling fluids services in the context of import substitution. Oil Industry. 2017. N 9, p. 76-81 (in Russian). DOI: 10.24887/0028-2448-2017-9-76-81
- Savenok O., Arutyunyan A., Petrushin E. Improving well construction efficiency in the context of drilling a wellbore and a pay zone: Primary and secondary drilling. Journal of Mechanical Engineering Research & Developments (JMERD). 2018. Vol. 41 (2), p. 28-30. DOI: 10.26480/jmerd.02.2018.28.3
- Sidorkin D.I., Kupavykh K.S. Justification on choosing screw pumping units as energy efficient artificial lift technology. Energetika. Proceedings of CIS Higher Education Institutions and Power Engineering Associations. 2021. Vol. 64. N 2, p. 143-151. DOI: 10.21122/1029-7448-2021-64-2-143-151
- Tashbulatov R.R., Karimov R.M., Valeev A.R., Mastobaev B.N. Modeling rheological properties of thixotropic oils at direct measurements on a rotary viscometer for evaluating the start-up modes of the trunk oil pipeline. Oil Industry. 2020. N 4, p. 80-84 (in Russian). DOI: 10.24887/0028-2448-2020-4-80-84
- Nikolayev N.I., Shipulin A.V., Kupavykh K.S. Results of research and efficiency of applying integrated solution for chemical treatment of bottomhole formation zone. Oil and Gas Territory. 2015. N 4, p. 79-83 (in Russian).
- Nigamatov Sh.A., Ismagilova L.R., Boschenko A.N. The Prediction of Salted Zones of Botuobinskiy Formation Sandstone on the Example of Chayandinskoye Field (East Siberia). PRONEFT. Professionals about Oil. 2019. N 3 (13), p. 35-40 (in Russian). DOI: 10.24887/2587-7399-2019-3-35-40
- Polyakov E.E., Churikova I.V., Pylev E.A. et al. Issues on the Permeability Coefficient Determination by Geophysical Well Logging for the Composite Reservoirs of Vendian Period in the Chayandinskoe Oil and Gas Condensate Field at the Development Drilling Stage. Oil and Gas Territory. 2018. N 10, p. 30-41 (in Russian).
- Fetisov V., Mohammadi A.H., Pshenin V. et al. Improving the economic efficiency of vapor recovery units at hydrocarbon loading terminals. Oil and Gas Science and Technology. 2021. Vol. 76. N 38. DOI: 10.2516/ogst/2021022
- Okesanjo O., Meredith J., Behrens S. Structure-Property Relationship in Capillary Foams. Langmuir: the ACS journal of surfaces and colloids. 2021. Vol. 37. Iss. 35, p. 1050-10520. DOI: 10.1021/acs.langmuir.1c01479