Morozkinskoye gold deposit (southern Yakutia): age and ore sources
- 1 — Postgraduate Student Russian Geological Research Institute named after A.P.Karpinsky ▪ Orcid
- 2 — Ph.D. Deputy Head of the Department Russian Geological Research Institute named after A.P.Karpinsky ▪ Orcid
- 3 — Ph.D. Head of the Department Russian Geological Research Institute named after A.P.Karpinsky ▪ Orcid ▪ Elibrary ▪ Scopus ▪ ResearcherID
- 4 — Leading Engineer Russian Geological Research Institute named after A.P.Karpinsky ▪ Orcid
- 5 — Ph.D., Dr.Sci. Head of the Department Russian Geological Research Institute named after A.P.Karpinsky ▪ Orcid
Abstract
The paper presents the results of the comprehensive isotope geochemical (Re-Os, Pb and δ 34 S) study of sulfide mineralization of the Morozkinskoye deposit. The ore zones of the deposit are localized in the syenite massif of Mount Rudnaya, which is located within the Central Aldan ore region (southern Yakutia). Gold mineralization is represented by vein-disseminated or vein type mineralization and is manifested in acidic low-temperature metasomatites – beresites (Qz-Ser-Ank-Py). For the first time we obtained an age estimate of the gold mineralization ~ 129 ± 3 Ma, which the synchronism of the hydrothermal ore process in the beresites, which formed the Morozkinskoye deposit, and magmatic crystallization of the syenites of Mount Rudnaya (~130 Ma). The osmium initial isotopic composition of the studied sulfides indicates a mixed mantle-crustal source of sulfide mineralization. New lead isotopic data of syenites indicate the predominance of mantle lead and an insignificant role of the lower – crust lead, while the isotopic composition of pyrite denotes the presence of the upper crustal material in the ore genesis. The sulfide δ 34 S values vary from –2.3 to +0.6 ‰ and indicate a predominantly magmatic source of sulfur in the ores.
Introduction
The Aldan Shield is the largest basement ledge of the Siberian Platform, within which in the Late Mesozoic potassium magmatism widely occurred and formed the high-K alkaline Aldan province [18]. One of the nine gold-bearing regions of this province is the Central Aldan [6, 7, 11], which also includes the intrusive massif of syenites of Mount Rudnaya with the recently discovered Morozkinskoye gold deposit with 17.3 tons of gold reserves. Mineralization is confined to the zones of beresitization (Qz-Ser-Ank-Py) and is represented by vein-disseminated and vein mineralization, located in steeply dipping submeridional shear zones within the intrusion. The history of the study, the results of isotope geochemical studies of syenites and, partly, the petrographic and geochemical features of the hydrothermal metasomatic formations of the deposit are given in previously published works [8, 10, 13]. Despite the fact that the object is well studied, some fundamental issues concerning the age of ore mineralization and the source of the substance remain unresolved. SHRIMP U-Pb zircon age of ~130 Ma indicates the time of magmatic crystallization of the syenites of Mount Rudnaya [13]. The formation of ore-bearing metasomatites (beresites) occurred during the final stage of crystallization as the intrusion cooled. At the same time, it cannot be excluded that ore mineralization in the beresites could be associated with later processes and superimposed on previously formed metasomatites.
To clarify the age of the hydrothermal processes and the ore source, the authors conducted complex isotope geochemical and geochronological (Re-Os, Pb and δ34 S) studies of ore-bearing metasomatites. The results of these studies allow to approach the question of the age of ore formation, the source of mineral matter and the evolution of the processes of ore formation and magmatism of the Mount Rudnaya.
Geological characteristics of the deposit
The ore bodies of the Morozkinskoye deposit are localized in the syenite massif of Mount Rudnaya, which is located within the Central Aldan ore region near the Lebediny village (Fig. 1). The geological structure of the territory of Mount Rudnaya is considered in detail in various articles [8, 10, 13].
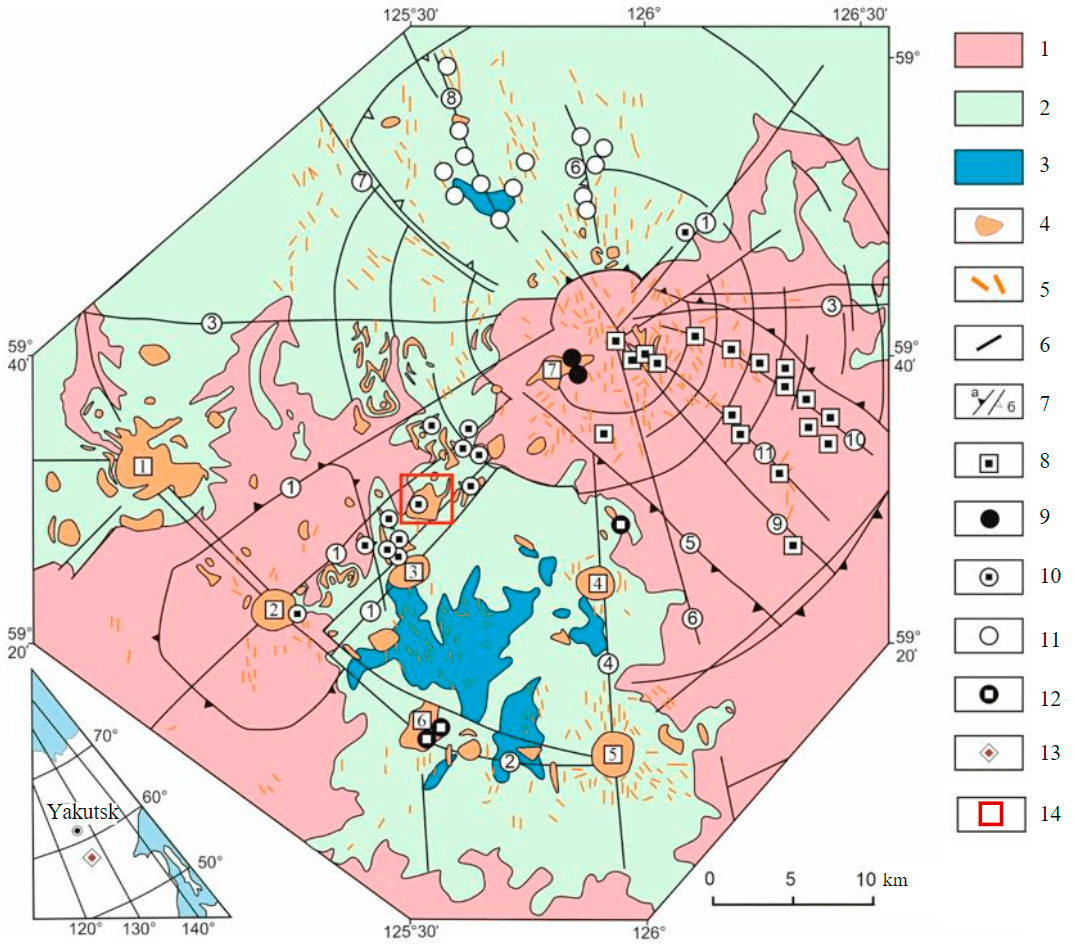
Fig.1. Geological and structural scheme of the Central Aldan ore district [9] 1 – the Early Precambrian crystalline basement; 2 – Vendian-Cambrian platform cover; 3 – terrigenous deposits of the Lower and Middle Jurassic; 4 – intrusions and alkaline and subalkaline dikes (5); 6 – faults; 7 – block borders: a – uplifts, b – troughs; 8-12 – commercial types of deposits: Elkonsky (Au-U) (8), Ryabinovy (Au-Cu) (9), Lebedinsky (Au) (10), Kuranakhsky (Au) (11), Samolazovsky (Au) (12); 13 – position of the Central Aldan ore district; 14 – study area. The largest Intrusive massifs (the numbers in the squares): 1 – Inaglinsky, 2 – Tommotsky, 3 – Yakokutsky, 4 – Dzhekondinsky, 5 – Yllymakhsky, 6 – Yukhtinsky, 7 – Ryabinovy. The largest linear faults (figures in circles): 1 – Tommotsky, 2 – Yukhtino-Purikansky, 3 – Severo-Aldansky, 4 – Dzhekondinsky, 5 – Yukhukhtinsky, 6 – Bayanai-Yllymakhsky, 7 – Kuranakhsky, 8 – Central Kuranakhsky (Bayanai-Kuranakhsky), 9 – Yukungrinsky, 10 – Yuzhny, 11 – Sokh-Solookhsky
In structural and tectonic terms, the massif coincides with the Tommotskaya magma- and ore-controlling fault zone, which acted as supply channels for magmatic melts in the Late Mesozoic [9]. The intrusion breaks through the rocks of the Fedorovskaya sequence (amphibole, hypersthene-amphibole, two-pyroxene gneisses and crystalline schists) and Early Proterozoic granitoids (Fig.2) and is partially underlain by thin layers of Vendian-Lower Cambrian dolomites [6, 15]. The laccolith intrusion has a maximum thickness of 180 m [10]. The massif is composed of Late Mesozoic and porphyritic syenites. The rocks of the massif are broken by later syenite porphyry and calc-alkaline lamprophyre dykes and sills, which are up to 1 km long and several meters thick.
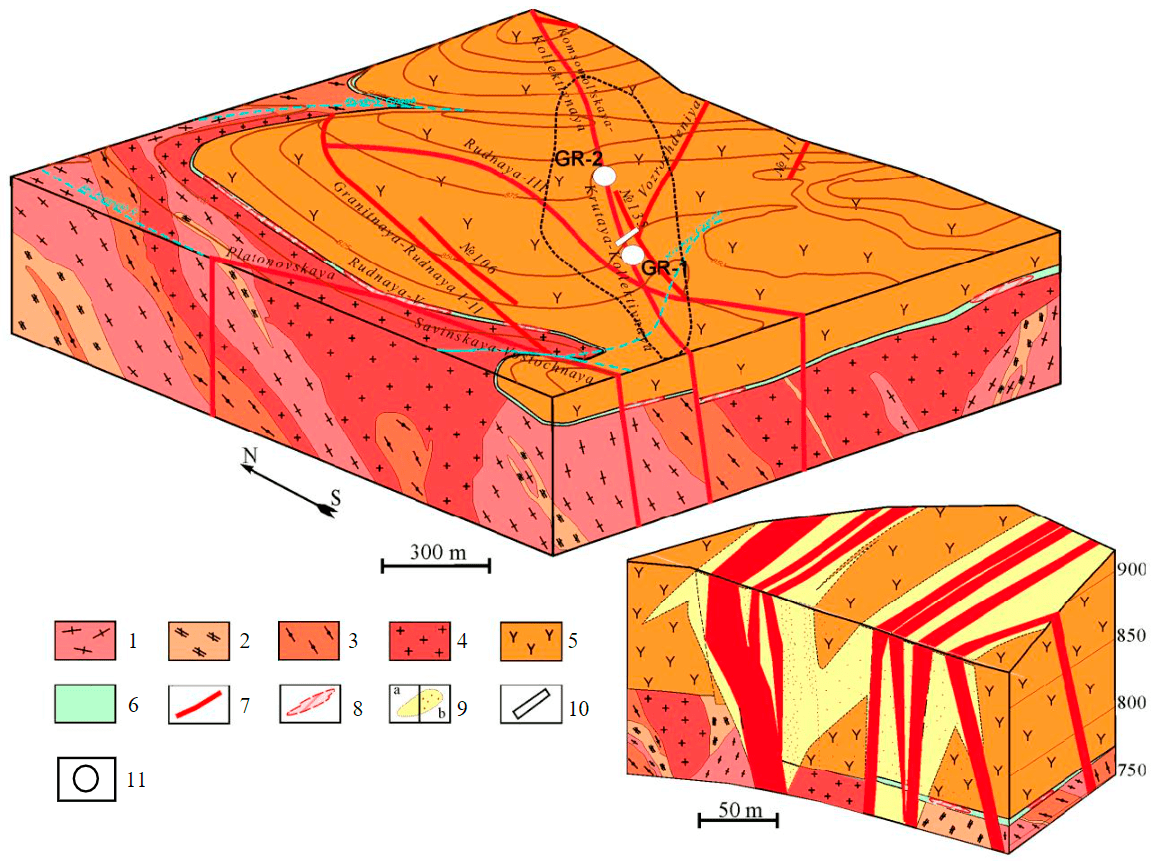
Fig.2. Block diagram of the geological structure of Mount Rudnaya [8, 10] 1-3 – rocks of the Fedorovsky strata: 1 – schist, 2 – plagiogneiss, 3 – gneiss; 4 – Paleoproterozoic granites; 5 – syenites; 6 – Vendian-Cambrian dolomites; 7 – gold mining steeply dipping veins and subhorizontal ribbon-like and sheet-like deposits; 8 – subhorizontal gold-bearing bodies of Lebedinsky (Au) type; 9 – ore-bearing hydrothermal metasomatic formations: a – zones of beresitization, b – zones of intensively beresitized and brecciated rocks; 10 – projection of the geological section on the surface; 11 – sampling sites
The intrusion of syenite melt led to the formation of contact- and hydrothermal-metasomatic associations. According to structural characteristics, as well as mineral parageneses, all metasomatites are divided into five groups: feldspatholites, propylites, skarnoids, gumbeites and beresites. The formation of metasomatites occurred along all stages of the massif formation: from the moment of the syenite melt intrusion (feldspatholites, skarnoids, propylites) to its crystallization (beresites and gumbeites) both in the host rocks and in the rocks of the intrusion itself. Commercial gold mineralization is confined to beresitization zones (Qz-Ser-Ank-Py) in areas of intensive metasomatic processing of rocks along steeply dipping fault structures within the intrusion [8]. The most extended and thick zones, containing at least 90 % of the gold resources in the deposit: Krutaya-Kollectivnaya, Vozrozhdeniye and Granitnaya-Rudnaya-I, II (Fig.2). Within the ore-bearing zones, sulfide (pyrite, chalcopyrite, and arsenopyrite) vein-disseminated and vein mineralization is developed. Gold is paragenetically associated with this mineralization.
According to published data [10], the reserves of the deposit are 17.3 tons of gold with an average content of 1.6 g/t. In terms of chemical composition, gold is a gold-silver alloy, the average fineness of which is 870 ‰. The hydrothermal metasomatic nature of the ore mineralization, the presence of shear zones and secondary enrichment under the oxidizing conditions of hypergenesis, and the vein-disseminated texture of metasomatites – indicate the multistage formation of the Morozkinskoye deposit, which the authors attributed to the gold-copper-porphyry type [8].
Methodology and research methods
Analytical studies were carried out at the Centre of Isotopic Research (CIR) of Russian Geological Research Institute (VSEGEI) (Saint Petersburg). Re-Os dating of ore-bearing beresites was carried out on pyrite monofractions from two representative samples GR-1 and GR-2. The samples were taken 300 m apart from the central part of the largest and most extended ore-bearing zone (Krutaya-Kollektivnaya) of the Morozkinskoye deposit, where gold mineralization is widespread. The samples are quartz-sericite-ankerite metasomatites with pyrite and chalcopyrite developed over brecciated syenite. Pyrite occurs in sulfide veins in association with chalcopyrite and in disseminated form in quartz-ankerite aggregate of the metasomatite matrix. The pyrite mineral fractions were sampled by hand picking under a binocular microscope. When studying pyrite, special attention was paid to the identification of inclusions. If there were any inclusions, sulfides were removed from the fraction. Five sulfide subsamples of 190 to 255 mg were taken from each sample. Minerals were dissolved in reverse aqua regia followed by separation of osmium and rhenium using bromine extraction, microdistillation, and liquid extraction with isoamyl alcohol [20]. Re and Os concentrations and the 187Re/188Os ratio were determined by isotopic dilution using a calibrated 185Re-190Os isotope indicator, which was added to a sulfide subsample prior to decomposition. The isotopic composition was measured in dynamic mode: osmium was measured in negatively charged ions on a Thermo Scientific Triton TIMS, and rhenium was measured from a 3 % HNO3 solution at low resolution mode using an electron multiplier detector of Thermo Scientific Neptune MC-ICP-MS.
The Pb isotopic composition was measured on a Triton mass spectrometer in the static multi-collector mode of ion current registration and single-tape sample input to the source. Each lead isotope analysis consisted of successive measurements of isotope ratios (206Pb/204Pb, 207Pb/204Pb and 208Pb/204Pb) of 50 blocks of 10 scans at a temperature of 1300 °C and an evaporate tape current of 2.2-2.3 A. The measurement session began with a 50 ng SRM-981 standard (206Pb/204Pb – 16.9374; 207Pb/204Pb – 15.4916; 208Pb/204Pb – 36.7219), the composition of which was used to further correction of the measured ratios of the samples for instrumental mass fractionation. The Pb blank value did not exceed 0.2 ng during the analyses and its composition was as follows: 206Pb/204Pb – 18.120; 207Pb/204Pb – 15.542; 208Pb/204Pb – 37.354. The blank to the sample lead ratio did not exceed 1/200000, therefore, no correction was made for the composition and content of blank lead for the performed analyzes.
The determination of the sulfur isotopic composition in sulfides was performed using the procedures described in the article [14]. A sample of sulfide (20-30 mg) was mixed with V2O5 and crushed quartz in the ratio of 1:10:10 and placed in a fused quartz reactor. Oxidation of sulfur was performed at 850 °С. The isotopic composition of sulfur was measured on a Thermo Finnigan Delta mass spectrometer in the double input mode. The correctness of measurements was controlled using standard samples with a known isotopic composition in the range of δ34S from –15.1 to +21.8 % relative to troilite from Canyon Diablo (CDT) (including international standards: NBS-123 – δ34S = +17.44; and NBS-127 – δ34S = +21.8). Reproducibility of the measured sulfur isotope composition, including sample preparation, is not worse than 2σ ± 0.1 % for δ34S. The values of δ34S are calculated relative to the CDT standard.
Results and discussion
Re-Os dating
The results of Re-Os isotope analysis of GR-1 and
GR-2 pyrite samples of ore-bearing beresites of the Morozkinskoye deposit are given in Table 1.
Table 1
Results of Re-Os isotope analysis of pyrite fractions from ore-bearing beresites of the Morozkinskoye deposit
Sample |
|
Subsample, mg |
Content, ppb |
187Re/188Os |
±2σ, % |
187Os/188Os |
±2σ, % |
T MA, Ma |
|
Re |
Os |
||||||||
GR-1 |
1 |
255.5 |
1.053 |
0.061 |
87.035 |
0.542 |
0.50550 |
0.004 |
261 |
2 |
216.3 |
1.691 |
0.053 |
163.67 |
0.155 |
0.67454 |
0.006 |
201 |
|
3 |
202.8 |
1.722 |
0.120 |
71.601 |
0.514 |
0.44923 |
0.007 |
270 |
|
4 |
199.2 |
0.938 |
0.182 |
25.453 |
0.294 |
0.37038 |
0.006 |
573 |
|
5 |
249.1 |
1.639 |
0.027 |
318.19 |
0.123 |
0.99408 |
0.014 |
163 |
|
GR-2 |
6 |
198.0 |
11.26 |
0.236 |
881.47 |
0.169 |
22.0269 |
0.247 |
1473 |
7 |
229.1 |
12.19 |
0.304 |
566.06 |
0.150 |
15.0660 |
0.083 |
1564 |
|
8 |
234.0 |
10.41 |
0.287 |
422.69 |
0.134 |
11.1138 |
0.038 |
1540 |
|
9 |
187.6 |
12.73 |
0.379 |
355.72 |
0.127 |
9.40346 |
0.069 |
1545 |
|
10 |
205.3 |
12.90 |
0.261 |
1078.8 |
0.075 |
27.3467 |
0.017 |
1496 |
The studied pyrite fractions of two samples are characterized by significant differences both in the content of rhenium and osmium, and in their ratio. If the rhenium content in GR-1pyrite varies from 0.938 to 1.722 ppb, and osmium from 0.027 to 0.182 ppb, then GR-2 pyrite is 2-10 times richer in these elements: the rhenium content varies from 10.4 to 12.9, and osmium – from 0.236 to 0.379 ppb. In terms of osmium content, the studied pyrite is comparable with pyrite from gold ore deposits associated with conglomerates and differs by an order of magnitude from pyrite from hydrothermal sulfide gold deposits (upwards) and gold deposits in komatiites (downwards) (Fig.3).
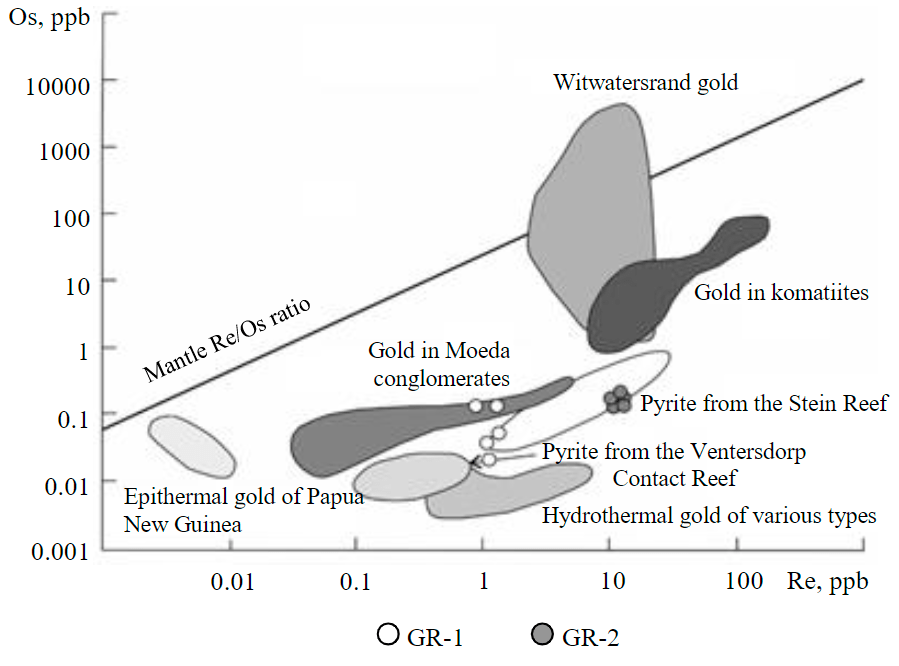
Fig.3. Diagram of rhenium versus osmium content in ore minerals of gold deposits [19, 25] in comparison with Morozkinskoye deposit pyrite composition
The osmium isotopic composition of the studied pyrite fractions also differs significantly for the two metasomatite samples. So, if in G-1 pyrite measured 187Os/188Os ratios ranges from 0.37 to 0.99, and differs both from crustal (>1,0 [28]) and mantle (≈ 0,1286 [24, 27]) values, for the GR-2 pyrite it changes from 9.4 to 27.3 and corresponds to typical crustal values. The high values of the isotopic 187Os/188Os ratio are the result of the 187Os radiogenic isotope accumulation due to the rhenium radioactive decay in situ in the sulfide matrix, as well as the initial osmium isotopic composition of the source, which formed sulfides of hydrothermal metasomatites (for example, crustal rocks). Accordingly, the mixing of substances from sources different in composition and osmium content (e.g., crust and mantle) during the formation of ore-bearing beresites, will also affect the value of the initial osmium isotope ratio in pyrite [31].
On the isochrones in 187Re/188Os – 187Os/188Os coordinates, the pyrite isotope compositions of GR-1 and GR-2 samples form linear trends (Fig. 4). Thus, for the GR-2 sample, three out of five analyses (Fig. 4, a) can be used to calculate a linear correlation, the slope of which corresponds to an age of 1468 ± 4 Ma at a MSWD value of 1.7 and an initial osmium isotope ratio (187Os/188Os)0 equal to 0.6320 ± 0.057. The calculated age significantly differs from the known ore mineralization and magmatism dating within the Central Aldan ore district and is not confirmed by direct geological observations of the structural and textural features of the ores. In terms of composition and rhenium and osmium content, the pyrite of GR-2 sample belongs to the group of so-called high-radiogenic with low content of non-radiogenic osmium (low-level-high-radiogenic, LLHR [31]) sulfides. The origin of such sulfides is still not clear, but, given the high value of the Re/Os ratio inherent to these sulfides, and using their rhenium-osmium isotopic systematics, it is often possible to obtain very accurate dating of mineralization (similar to the application of the rhenium-osmium system of molybdenites). But in this case, as a rule, the uncertainty of the initial isotopic composition of osmium at the time of the isotopic system formation is high [31].
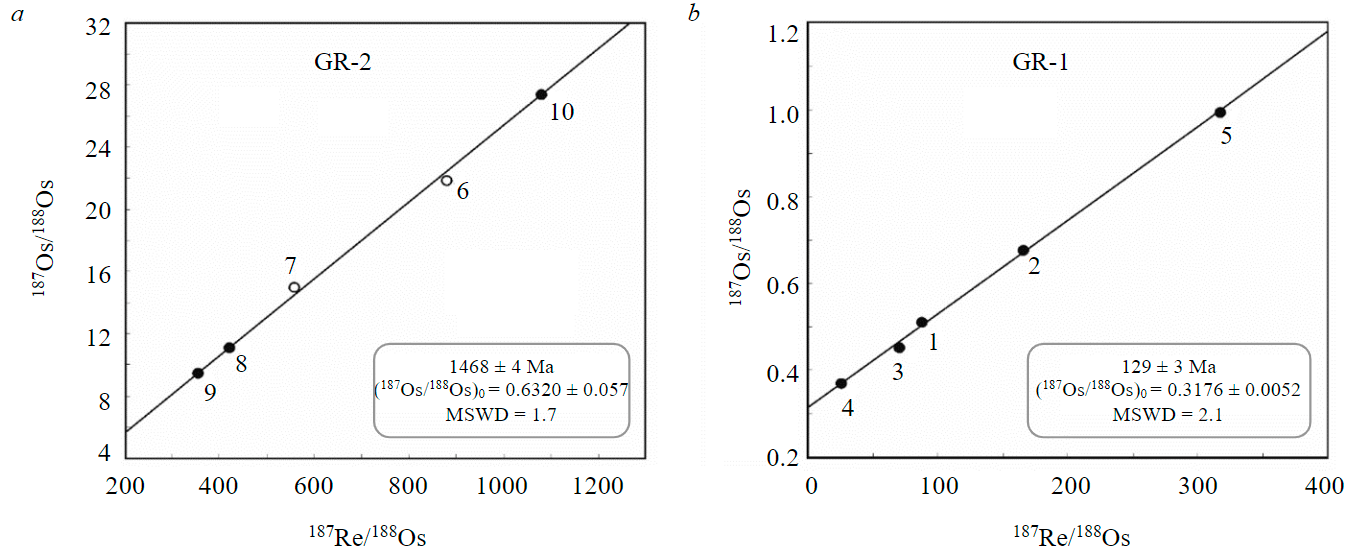
Fig.4. Re-Os isochron for pyrite fractions from the Morozkinskoye deposit: a – sample GR-2, the isochron is constructed using three analyses (filled points); b – sample GR-1
All this fully applies to the osmium isotope system in GR-2 pyrite sample: in which the content of 187Os varies within 0.17-0.21 ppb, and the content of non-radiogenic osmium is less than half of this value. At the same time, the uncertainty of the initial osmium isotope ratio according to the isochron is 10 %, but even with a change of 10-20 % of this value, the age estimation changes insignificantly within ±10 Ma.
If we assume that the composition of osmium in pyrite at the formation time corresponded to the mantle characteristics (187Os/188Os ≈ 0.1286), then the maximum age of the system increases to 1565 Ma. Moreover, if all five analyses of pyrite of this sample are used to calculate the linear trend, then the slope of the obtained errorchron will correspond to 1451 ± 96 Ma and an initial osmium isotope composition (187Os/188Os)0 of 0.8 ± 1.2 at MSWD of 33.
An additional argument in favor of the significance of the obtained linear trend on the isochron diagram is the value of the Re-Os model age of the studied fractions relative to the chondritic reservoir (TMA), varying from 1473 to 1564 Ma (see Table 1). Similar manifestations of magmatism with apatite ores with K-Ar age varying in the interval of 1400-1500 Ma (Seligdarskoye deposit) were previously recorded 20 km northeast of the object under study [26]. It is possible that the ore-forming fluid entered the discharge zone during the main stage of Mesozoic magmatism about 130 Ma ago and was formed both due to the residual material of the magmatic melt of syenites and due to the remobilization of material from the host rocks of the Vendian-Lower Cambrian platform cover or basement gneisses and crystalline schists. At the same time, the main source of osmium of sulfide mineralization was a crustal material [31]. In this case, the initial isotope composition of osmium (187Os/188Os)0 of pyrite fractions should have varied within the 25.00-8.63 range at the time of pyrite crystallization 130 Ma ago, or 15.554 ± 6.4 on average. Such a high value of the (187Os/188Os)0 isotope ratio: (1.2 for the upper crust, 0.8 for the lower crust [24]), could have formed in a source with a Re/Os ratio of 120-530 for 500-700 Ma. As a source of osmium could be sulfides of the host rocks of the platform cover, which are characterized by a fractionated character of the rhenium-osmium ratio up to 106 or more in the presence of molybdenite. In addition, the high variability of the initial osmium isotopic ratio for the studied pyrite fractions also agrees well with the sedimentary origin of the sulfides, when there is no prior homogenization of the isotopic composition of the rhenium-osmium system.
For the pyrite GR-1 sample, Re-Os isochron was built according to all five analyses, the MSWD value is 2.1, the initial osmium isotope ratio (187Os/188Os)0 is 0.3176 ± 0.0052, the slope of the isochron corresponds to the age of 129 ± 3 Ma (Fig.4, b). Despite the relatively high MSWD, this estimate is close to the age of crystallization of syenites from the Mount Rudnaya, which was obtained by the authors [13] based on the zircon U-Pb SHRIMP II dating. Based on the analysis of the rare-element composition, two even-aged, but contrasting in composition and appearance zircon types were distinguished in this work. It was also concluded that the magmatic crystallization of the Mount Rudnaya syenites and their fluid alteration were close in time. The obtained age estimation for sulfides is in good agreement with the age of the ore-bearing gumbeites of the Ryabinovoe deposit (129 ± 1 Ma) and the time of the main ore-producing stage of magmatism within the Central Aldan ore region [16]. Thus, the Re-Os isochron age (129 ± 3 Ma) of the pyrite of the GR-1 sample can be considered geologically significant, and the magmatic crystallization of the Mount Rudnaya syenites and the formation of ore mineralization in the beresites can be considered subsynchronous.
Isotopic composition of Pb
The lead isotope composition was studied in four samples of syenite and three single fractions of pyrite from the ore-bearing beresites of the Morozkinskoye deposit. The results of measurements of the lead isotope composition, the model age, and parameters of the lead source according to the Stacey-Kramer model [30] are given in Table 2, and the initial isotope characteristics calculated for the estimated time of intrusion crystallization of ~130 Ma are given in Table 3.
The measured values of syenites isotopic ratios vary in the following ranges: 206Pb/204Pb from 16.775 to 17.399; 207Pb/204Pb from 15.308 to 15.444; 208 Pb/204 Pb from 36.743 to 37.686. Lead from pyrite is characterized by significantly less variation in isotopic composition and a more radiogenic composition compared to syenites: 206Pb/204Pb from 17.369 to 17.521; 207Pb/204Pb from 15.450 to 15.537; 208Pb/204Pb from 37.680 to 38.178. In the 206Pb/204Pb-207Pb/204Pb coordinates (Fig.5) points of the lead isotope composition of the studied samples are below the line of evolution of the lead isotope composition of the Earth according to the two-stage Stacey – Kramers model [30] and to the left of the geochron, forming a distinct linear trend (except for the GR-1 pyrite sample), which may have both geochronological significance (if it was formed predominantly by the decay of uranium and in situ accumulation of radiogenic lead), and testify to the mixing of matter from at least two sources with different isotopic compositions during rock crystallization and sulfide formation. The calculated trends indicate a Late Archean age (about 2.6 Ga) of lead isotope system formation in syenites and pyrites. Fig.5 shows a reference isochron with an age of 2589 ± 110 Ma, calculated from four points with MSWD = 13. An alternative explanation may be the formation of the isotope system 130 Ma ago due to the mixing of crust lead with 206Pb/204Pb 18.505, 207Pb/204Pb 15.619 and lower crust-mantle lead with an age of 2400 Ma and a composition of 13.88 and 14.82, respectively (Fig.5). It is obvious that the share of lower crust-mantle lead in the mixture should be at least 20 % (up to 40-60 %), taking into account the predominant concentration of lead in upper crustal rocks (the average concentration is four times higher than in the mantle one [28]) and lead content in the studied samples (15-20 ppm). The uranium-lead second-stage ratio μ2 in the studied syenite samples vary in a relatively narrow range of 8.87-9.36, but the value of the ratio itself is significantly lower than 9.74 (the average value for the silicate Earth of the Stacey – Kramers model [30]), which may indicate relative depletion by uranium of the magmatic source of syenites. At the same time, the μ2 value for pyrite is significantly higher, reaching 9.70 in the GR-1 sample, which allows to estimate the model age of the uranium-lead system of this pyrite at 790-800 Ma (Fig.5) according to the two-stage Stacey – Kramers model of the lead isotope evolution. The values of ω2 and χ2 in syenites vary widely: 15.0-31.0 and 1.51-3.52, respectively, which reflects the increased mobility of uranium and thorium relative to lead in the source of melts and during rock crystallization due to the influence of the fluid component.
Table 2
Isotopic composition of lead (measured) in syenites and pyrite fractions of the Morozkinskoye deposit
Sample |
206Pb/204Pb ± 2σ |
207Pb/204Pb ± 2σ |
208Pb/204Pb ± 2σ |
T, Ma* |
μ* |
ω* |
χ* |
Syenites |
|||||||
1212 |
17.3987 ± 6 |
15.4185 ± 7 |
37.6861 ± 24 |
570 |
9.15 |
26.0 |
2.63 |
1213 |
17.1965 ± 3 |
15.4042 ± 3 |
37.1994 ± 11 |
700 |
9.15 |
15.0 |
1.51 |
1214 |
17.1744 ± 6 |
15.4443 ± 6 |
37.3495 ± 20 |
800 |
9.36 |
21.0 |
2.07 |
G-1 |
16.7748 ± 2 |
15.3084 ± 2 |
36.7432 ± 5 |
850 |
8.87 |
31.0 |
3.52 |
Sulfides (pyrite) |
|||||||
GR-1 |
17.4171 ± 4 |
15.5368 ± 5 |
38.1783 ± 17 |
790 |
9.70 |
– |
– |
GR-1/1 |
17.3688 ± 3 |
15.4496 ± 3 |
37.8907 ± 7 |
650 |
9.30 |
– |
– |
GR-2 |
17.5206 ± 3 |
15.4553 ± 3 |
37.6802 ± 6 |
550 |
9.28 |
– |
– |
* Model age and parameters μ (238U/204Pb), ω (232Th/204Pb), χ (232Th/238U) calculated by Isoplot using Version 3.70*a two-stage model [30].
Table 3
Initial isotopic characteristics of the studied samples from the Morozkinskoye deposit, calculated for the expected crystallization time ~130 Ma
Sample |
U, ppm |
Th, ppm |
Pb, ppm |
206Pb/204Pb |
207Pb/204Pb |
208Pb/204Pb |
μ* |
ω* |
χ* |
Syenites |
|||||||||
1212 |
3.01 |
7.93 |
14.4 |
17.1356 |
15.4057 |
37.4592 |
12.9 |
35.2 |
2.72 |
1213 |
1.60 |
2.42 |
19.0 |
17.0915 |
15.3991 |
37.1474 |
5.15 |
8.05 |
1.56 |
1214 |
1.28 |
2.65 |
18.2 |
17.0865 |
15.4400 |
37.2900 |
4.31 |
9.23 |
2.14 |
G-1 |
2.48 |
8.73 |
26.4 |
16.6593 |
15.3028 |
36.6101 |
5.67 |
20.6 |
3.64 |
Sulfides (pyrite) |
|||||||||
GR-1 |
– |
– |
– |
17.4171 |
15.5368 |
38.1783 |
– |
– |
– |
GR-1/1 |
– |
– |
– |
17.3688 |
15.4496 |
37.8907 |
– |
– |
– |
GR-2 |
– |
– |
– |
17.5206 |
15.4553 |
37.6802 |
– |
– |
– |
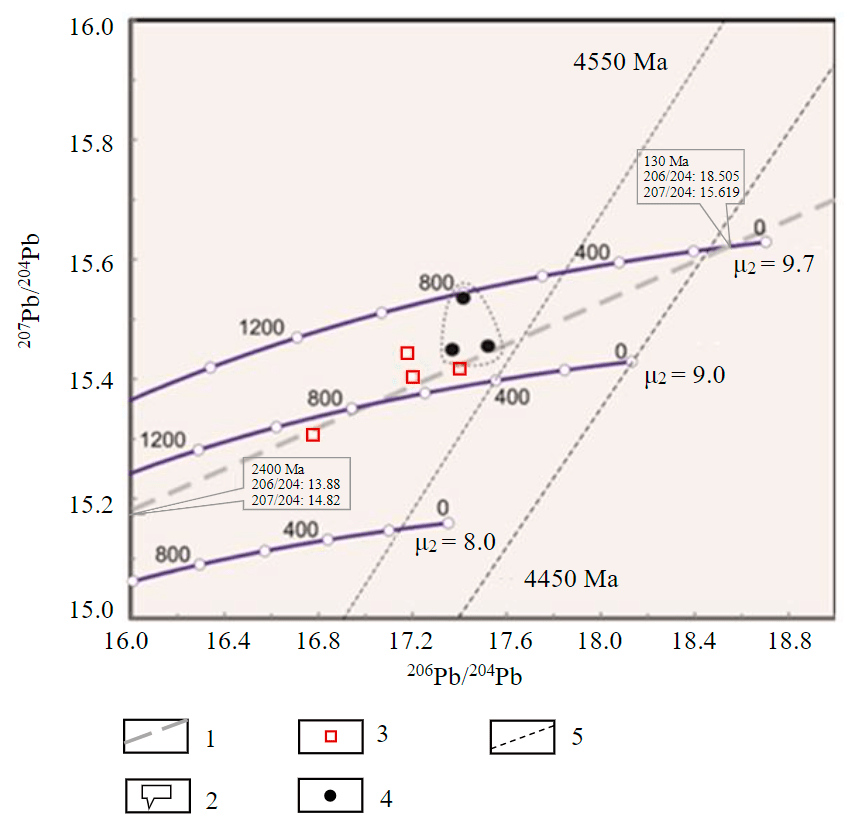
Fig.5. Measured 207Pb/204Pb and 206Pb/204Pb isotope ratios for studied syenites and sulfides (pyrites) of the Morozkinskoye field. The Pb isotope evolution curves were calculated for various ratios of uranium and lead (μ2) in the source according to the Stacey – Kramers model [30] 1 – reference isochron with an age of 2589 ± 110 Ma on four data-points (three syenite samples and one sulfide, MSWD – 13); 2 – Pb isotope composition and age of the end-members of the mixing during the formation of the observed trend; Pb isotope composition: 3 – syenites; 4 – sulfides; 5 – geochrons with ages of 4450 and 4550 Ma
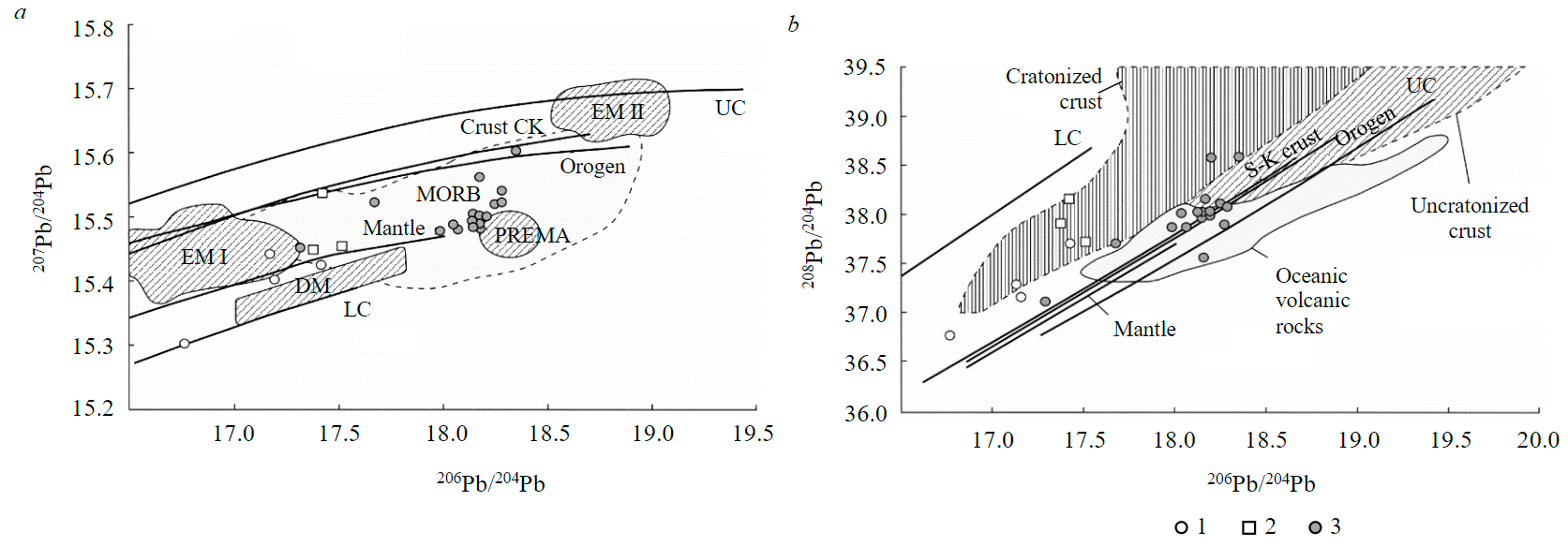
Fig.6. Diagrams of the initial isotope compositions of 207Pb/204Pb vs. 206Pb/204Pb (a) and 208Pb/204Pb vs. 206Pb/204Pb (b) for syenites and sulfides (pyrites) from the Morozkinskoye deposit. Compositions of mantle sources are shown according to [23, 32]: DM – depleted mantle; PREMA – predominant mantle; EM I – enriched mantle of type I; EM II –
enriched mantle of type II; MORB – basalts of mid-oceanic ridges [3]. Pb isotope evolution curves of the mantle, the Orogen type source, the lower (LC) and upper (UC) crust are shown according to the plumbotectonics model [23], and the S-K crust according to the Stacey – Kramers model [30]. Pb isotope composition: 1 – syenites; 2 – sulfides; 3 – igneous rocks of the Ket-Kap gold-ore region [3]
In the diagram in the 207Pb/204Pb-206Pb/204Pb coordinates (Fig.6, a) the points of initial lead isotope composition (corrected for the accumulation of radiogenic lead over 130 Ma since the formation) of syenite samples (samples 1212, 1213, and 1214) form a compact group near the evolution curve of the mantle and enriched mantle field of type EMI [32]. The point corresponding to the lead isotope composition of sample G-1 is located much lower and corresponds to the evolution curve of the lower crust. These results do not contradict the conclusions obtained in the study of Sr-Nd isotope systematics of the Central Aldan region [4, 33]. The initial lead isotopic composition of the two pyrite samples (GR-1/1 and GR-2) is close to that of syenites and is located on the mantle evolution curve in the field of isotopic compositions of MORB basalts [34]. The figurative point of the GR-1 pyrite fraction is shifted relative to the composition of other studied samples and is located directly at the Stacey – Kramers or Orogen lead isotope composition curve of the plumbotectonic model [23] and corresponds to the composition of lead with the age of 790-800 Ma.
In the diagram in 208Pb/204Pb-206Pb/204Pb coordinates (Fig.6, b), the points of Pb isotope compositions of syenites and pyrite (GR-2) form a linear trend between the evolution curves of the lower crust (LC) and the Stacey – Kramers crust (S-K). All points of isotopic compositions, except for a few samples (1213, G-1), are located in the field of cratonized continental crust [3]. The initial Pb isotope characteristics of the syenites of Mount Rudnaya are similar to those of igneous rocks of the Ket-Kap gold-ore region (located 400 km southeast of the Central Aldan region), for which the PREMA-type mantle source (predominant mantle [32]), was important [3]. A distinctive feature of the Ket-Kap magmatites is also the presence of the upper crustal source matter in the magmatic melts, while its role for the genesis of the syenite magmas of Mount Rudnaya is insignificant.
Thus, the study of the lead isotope composition of syenites and pyrite fractions of the Morozkinskoye deposit makes it possible to identify the source of the magmatic melts and their accompanying ore components. The linear trend shown in the Pb-Pb isotope diagrams (Fig.6) indicates a heterogeneous isotope composition of lead and a significant role of mantle and lower crust lead in the formation of igneous rocks. The lead isotope composition of pyrite indicates a similar to syenites mantle source, suggesting a dominant role of magmatic fluids in the formation of the mineralization. At the same time, the Pb isotopic composition of pyrite reveals also the presence of lead from an upper crustal (or Orogen-type) source during the genesis of the ore mineral.
Sulfur isotope composition
The isotope composition of sulfur was studied in sulfides of ore-bearing beresites of the Morozkinskoye deposit, 16 analyses were performed: 10 – pyrite (Py), 3 – chalcopyrite (Сcр), 3 – arsenopyrite (Ару) (Table 4). Despite the limited number of δ34S determinations, the obtained results allowed to characterize the conditions of sulfide mineralization formation at the studied deposit.
Table 4
Sulfur isotopic composition of sulfides from the Morozkinskoye deposit
Sample number |
Mineral |
δ34S, ‰ |
GR-1 |
Py |
0.2 |
GR-1/1 |
» |
0.3 |
GR-1/2 |
» |
–0.4 |
GR-1/3 |
» |
0.6 |
GR-1/4 |
» |
–0.3 |
GR-1/5 |
» |
–0.1 |
GR-2 |
» |
–1.9 |
GR-2/1 |
» |
–1.7 |
MT-152 |
» |
–2.3 |
GR-2/3 |
» |
–1.2 |
GR-1/7 |
Сcр |
0.3 |
GR-2/2 |
» |
–0.7 |
MT-152/2 |
» |
–0.9 |
GR-1/6 |
Apy |
0.5 |
GR-2/1 |
» |
–0.3 |
MT-152/1 |
» |
–0.4 |
The δ34S values of sulfides vary from –2.3 to +0.6 ‰, with δ34S variation interval for pyrite from –2.3 to +0.6 ‰, for chalcopyrite from –0.9 to +0.3 ‰, and for arsenopyrite from –0.4 to +0.5 ‰ (Fig.7, a). Obviously, the range of δ34S values for pyrite is wider, but this may be due to the large number of pyrite composition measurements. In general, variations in the sulfur isotope composition in the studied sulfides are insignificant. And this argues in favor of the homogeneous composition of the chamber of ore-bearing magmas and of the magmatic source of sulfur in the ores. However, the influence of the sedimentary component due to the mobilization of sulfur from the Vendian-Lower Cambrian dolomites during melt contamination cannot be completely excluded, but the sulfur isotope composition underlying dolomites remains unstudied.
The isotopic composition of sulfur from sulfide deposits of the Central Aldan ore district has previously been repeatedly studied [2, 5, 17]. A comparative analysis of different sites within the Central Aldan ore district showed significant variations in the isotopic composition of sulfur in sulfides (Fig. 7, b). The δ34S variations of sulfides from hydrothermal-metasomatic deposits are believed to be the result of various magmatic and post-magmatic processes: contamination of magma with matter from host rocks, migration of mobile components, changes in the redox potential of the mineral-forming fluid, exchange reactions during ore formation, etc. In addition, different magma areas with special isotope composition of sulfur, which form their own hydrothermal solutions, could take part in the same magmatic cycle [1, 22, 29]. Despite significant variations in the isotope composition of sulfur in the sulfide deposits of the Central Aldan ore district, it is obvious that within the study area sulfur is largely of magmatic origin (δ34S ± 5 %, Fig.7, b). However, the geological setting and the structural and textural features of the ores indicate that the formation of gold ore deposits occurred in near-surface conditions with the involvement of sedimentary sulfur in the process of mineral formation. Thus, the isotope composition of sulfur in sulfides from the ores of the Kuranakhskoye deposit (δ34S from +11 to +30 ‰) confirms the predominant borrowing of sulfur by ore-bearing solutions from terrigenous and carbonate rocks. According to the conditions of formation of ore mineralization (vein-disseminated mineralized beresites of syenite shear zones), the Morozkinskoye deposit occupies an intermediate position among the gold ore objects of the Central Aldan ore region, which determines both the composition of the sulfur involved in the process of mineral formation and the predominantly magmatic nature of its source.
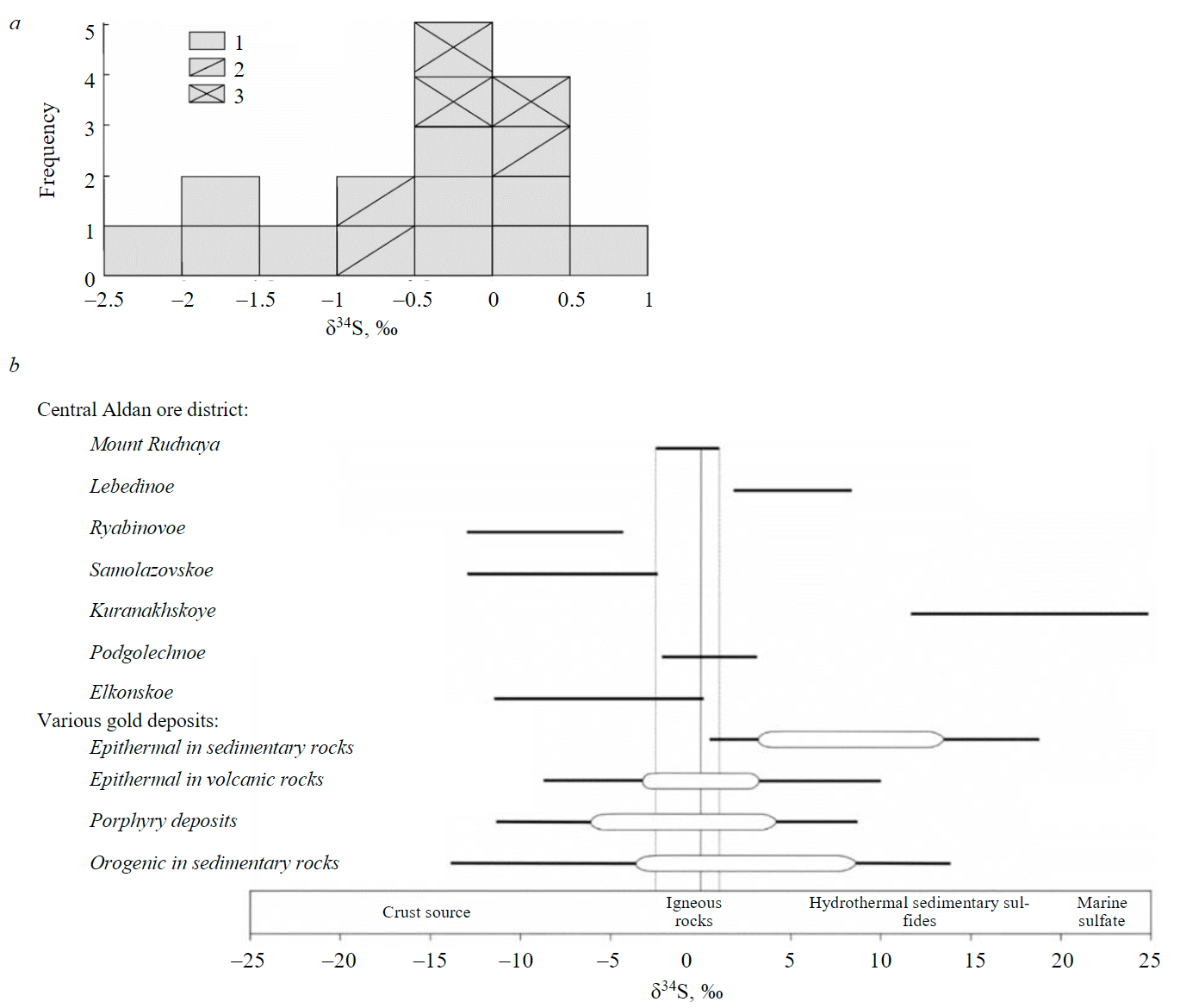
Fig.7. Sulfur isotope composition of sulfides of the Morozkinskoye (a) deposit and the Central Aldan ore district [2, 5, 17], as well as deposits of various gold genetic types [1, 21, 25] (b) 1 – pyrite, 2 – chalcopyrite, 3 – arsenopyrite
Conclusions
The results of this study allow to do the following conclusions and outline directions for further research.
Re-Os isotope dating of the sulfide mineralization of the Morozkinskoye gold deposit provided the first reliable age estimate of 129 ± 3 Ma of the gold mineralization. Thus, the hydrothermal ore process in the beresites of the Morozkinskoye deposit and the intrusion of the syenites magmas of Mount Rudnaya (℘130 Ma ago) occurred almost synchronously.
The initial isotopic composition of osmium of the studied sulfides indicates a mixed mantle-crust source of sulfide mineralization.
The lead isotope composition of syenites indicates the presence of mantle lead, and the mantle itself should be ancient, Paleoproterozoic or even Neoarchean, while the role of lower crust lead is insignificant. The lead isotope composition of pyrite indicates a close mantle source, but with the participation of upper crust matter in the genesis of the ore mineral.
The isotope composition of sulfur in sulfides (from –2.3 to +0.6 ‰) is clearly magmatic.
The entirety of the obtained isotope and geochemical characteristics of sulfide minerals of ore metasomatites indicates a close composition of the source of syenite melts of the Mount Rudnaya massif and ore-bearing fluids. However, the predominant concentration of gold in the oxidized zones of mineralized metasomatites indicates the importance of secondary enrichment processes. Further study of sulfide mineralization, including characterization of the copper and iron isotope composition sensitive to redox conditions, is necessary to assess the role of these secondary processes.
References
- Anikina E.Y., Gamyanin G.N., Bortnikov N.S. Sulfur Isotopic Composition of Sulfides at the Mangazeya Silver Deposit, Eastern Sakha-Yakutia, Russia. Geology of Ore Deposits. 2010. Vol. 52. N 6, p. 479-495. DOI: 10.1134/S1075701510060048
- Boytsoy V.P., Pilipenko G.N., Dorozhklna L.A. Formation Model of Complex Gold-Uranium Deposits of Central Aldan Mining Region. Proceedings of Higher Educational Establishments. Geology and Exploration. 2006. N 2, p. 23-31. URL: https://www.elibrary.ru/item.asp?id=9218021
- Polin V.F., Dril S.I., Khanchuk A.I. et al. Lead isotope variations in polyformational magmatites of the Ketkapsko-Yunskoe magmatic province of the Aldan Shield as evidence of mantle-crustal interaction. Doklady Akademii nauk. 2016. Vol. 468. N 5, p. 566-571. DOI: 10.7868/S0869565216170229
- Vasyukova E.A., Ponomarchuk A.V., Doroshkevich A.G. Petrological and Geochemical Characteristics and Age of the Rocks of the Yllymakh Massif (Aldan Shield, southern Yakutia). Russian Geology and Geophysics. 2020. Vol. 61. N 4, p. 396-411. DOI: 10.15372/RGG2019147
- Dobrovolskaya M.G., Razin M.V., Prokofev V.Y. Lebediny Gold Deposit, Central Aldan: Mineral Parageneses, Stages, And Formation Conditions. Geology of Ore Deposits. 2016. Vol. 58. N 4, p. 308-326. DOI: 10.1134/S1075701516040024
- Vetluzhskikh V.G., Kazanskii V.I., Kochetkov A.Ya., Yanovskii V.M. Gold deposits of Central Aldan. Geologiya rudnykh mestorozhdenii. 2002. Vol. 44. N 6, p. 467-499 (in Russian).
- Kazansky V.I. The Unique Central Aldan Gold-Uranium Ore District (Russia). Geology of Ore Deposits. 2004. Vol. 46. N 3, p. 167-181. URL: https://www.elibrary.ru/item.asp?id=17359219
- Molchanov A.V., Terekhov A.V., Shatov V.V. et al. Lebedinsky gold ore node (features of geological structure, metasomatites and mineralization). Regionalnaya geologiya i metallogeniya. 2013. N 55, p. 99-110 (in Russian). URL: https://www.vsegei.ru/ru/public/reggeology_met/content/2013/55/55_10.php
- Maximov Ye.P., Uyutov V.I., Nikitin V.M. The Central Aldan gold-uranium ore magmatogenic system (Aldan-Stanovoy shield, Russia). Tikhookeanskaya Geologiya. 2010. Vol. 29. N 2, p. 3-26 (in Russian). URL: http://itig.as.khb.ru/POG/2010/2010_t29_n2.pdf
- Petrov O.V., Molchanov A.V., Terekhov A.V., Shatov V.V. Kozkinskoe gold deposit (geological structure and short story of the exploration). Regional Geology and Metallogeny. 2018. N 75, p. 112-116. URL: https://vsegei.ru/ru/public/reggeology_met/content/2018/75/index.php
- Parfenov L.M., Kuzmin M.I. Tectonics, geodynamics and metallogeny of the Republic of Sakha (Yakutia). Moscow: Nauka/Interperiodika, 2001, p. 571 (in Russian).
- Velikoslavinsky S.D., Kotov A.B., Salnikova E.B. et al. Protoliths of the Metamorphic Rocks of the Fedorov Complex, Aldan Shield: Character, Age, and Geodynamic Environments of Origin. Petrology. 2006. Vol. 14. N 1, p. 21-38. DOI: 10.1134/S0869591106010036 (in Russian).
- Guzev V.E., Terekhov A.V., Skublov S.G., Leontev V.I., Molchanov A.V. The first data on the U-Pb age and composition of zircon from ore-bearing syenites of Mount Rudnaya (South Yakutia). Tikhookeanskaya geologiya. 2021. Vol. 40. N 6, p. 85-99.
- Palyanova G.A., Sobolev E.S., Reutskii V.N., Bortnikov N.S. Pyritized bivalves from the Upper Triassic of the Sentachan orogenic gold-antimony deposit (Eastern Yakutia): mineral composition and sulfur isotopic composition. Geologiya rudnykh mestorozhdenii. 2016. Vol. 58. N 6, p. 513-521. DOI: 10.7868/S0016777016060071 (in Russian).
- Velikoslavinskii S.D., Kotov A.B., Salnikova E.B. et al. Early Precambrian Granite-Gneiss Complexes in the Central Aldan Shield. Petrology. 2011. Vol. 19. N 4, p. 382-398. DOI: 10.1134/S0869591111040060
- Shatova N.V., Molchanov A.V., Terekhov A.V. et al. Ryabinovoe copper-gold-porphyry deposit (southern Yakutia): geology, noble gases isotope systematics and isotopic (U-Pb, Rb-Sr, Re-Os) dating of wallrock alteration and ore-forming processes. Regional Geology and Metallogeny. 2019. N 77, p. 75-97 (in Russian). URL: https://vsegei.ru/ru/public/reggeology_met/content/2019/77/index.php
- Borisenko I.D., Borovikov A.A., Borisenko A.S., Gaskov I.V. Physical and chemical conditions for the formation of ores of the Samolazovskoe gold deposit (Central Aldan). Geologiya i geofizika. 2017. Vol. 58. N 12, p. 1915-1927. DOI: 10.15372/GiG20171205
- Khomich V.G., Boriskina N.G. Essence of the Late Mesozoic ore-magmatic systems of Aldan Shield. Lithosphere. 2016. N 2, p. 70-90 (in Russian). URL: https://www.lithosphere.ru/jour/article/view/17
- Kirk J., Ruiz J., Chesley J. et al. A major Archean, gold- and crust-forming event in the Kaapvaal Craton, South Africa. Science. 2002. Vol. 297. Iss. 5588, p. 1856-1858. DOI: 10.1126/science.1075242
- Birck J.L., Barman M.R., Campas F. Re-Os isotopic measurements at the femtomole level in natural samples. Geostandards Newsletter. 1997. Vol. 20. N 1, p. 19-27. DOI: 10.1111/j.1751-908X.1997.tb00528.x
- Chang Z., Large R.R., Maslennikov V. Sulfur isotopes in sediment-hosted orogenic gold deposits: Evidence for an early timing and a seawater sulfur source. Geology. 2008. Vol. 36. Iss. 12, p. 971-974. DOI: 10.1130/G25001A.1
- Cooke D.R., Hollings P., Wilkinson J.J., Tosdal R.M. Geochemistry of porphyry deposits. Treatise on Geochemistry. 2nd edition. Elsevier, 2014. Vol. 13, p. 357-281. DOI: 10.1016/B978-0-08-095975-7.01116-5
- Halla J. Pb isotopes – a multi-function tool for assessing tectonothermal events and crust-mantle recycling at late Archaean convergent margins. Lithos. 2018. Vol. 320-321, p. 207-221. DOI: 10.1016/j.lithos.2018.08.031
- Harvey J., Warren J.M., Shirey S.B. Mantle sulfides and their role in Re-Os and Pb isotope geochronology. Reviews in Mineralogy & Geochemistry. 2017. Vol. 81. Iss. 1, p. 579-649. DOI: 10.2138/rmg.2016.81.10
- Hattori K.H., Keith J.D. Contribution of mafic melt to porphyry copper mineralization: evidence from Mount Pinatudo, Philippines and Bingham Canyon, Utah, USA. Mineralium Deposita. 2001. Vol. 36. Iss. 8, p. 799-806. DOI: 10.1007/s001260100209
- Prokopyev I.R., Doroshkevich A.G., Ponomarchuk A.V., Sergeev S.A. Mineralogy, age and genesis of apatite-dolomite ores at the Seligdar apatite deposit (Central Aldan, Russia). Ore Geology Reviews. 2017. Vol. 81. Part 1, p. 296-308. DOI: 10.1016/j.oregeorev.2016.10.012
- Reisberg L. Osmium isotope contraints on formation and refertilization of the non-cratonic continental mantle lithosphere. Chemical Geology. 2021. Vol. 574. N 120245. DOI: 10.1016/j.chemgeo.2021.120245
- Rudnick R., Gao S. Composition of the continental crust. Treatise on Geochemistry. 2nd edition. Elsevier, 2014. Vol. 4. p. 1-51. DOI: 10.1016/B978-0-08-095975-7.00301-6
- Shanks W.C.P. III. Stable isotope geochemistry of mineral deposits. Treatise on Geochemistry. 2nd edition. Elsevier, 2014. Vol. 13, p. 59-85. DOI: 10.1016/B978-0-08-095975-7.01103-7
- Stacey J.S., Kramers I.D. Approximation of terrestrial lead isotope evolution by a two-stage model. Earth and Planetary Science Letters. 1975. Vol. 26. Iss. 2, p. 207-221. DOI: 10.1016/0012-821X(75)90088-6
- Stein H.J. Dating and tracing the history of ore formation. Treatise on Geochemistry. 2nd edition. Elsevier, 2014. Vol. 13, p. 87-118. DOI: 10.1016/B978-0-08-095975-7.01104-9
- Stracke A. Earth’s heterogeneous mantle: a product of convection-driven interaction between crust and mantle. Chemical Geology. 2012. Vol. 330-331, p. 274-299. DOI: 10.1016/j.chemgeo.2012.08.007
- Davis G.R., Stolz A.J., Mahotkin I.L. et al. Trace element and Sr-Pb-Nd-Hf isotope evidence for ancient, fluid-dominated enrichment of the source of Aldan Shield lamproites. Journal of Petrology. 2006. Vol. 47. Iss. 6, p. 1119-1146. DOI: 16.1093/petrology/egl1005
- White W.M., Klein E.M. Composition of the oceanic crust. Treatise on Geochemistry. 2nd edition. Elsevier, 2014. Vol. 4, p. 457-498. DOI: 10.1016/B978-0-08-095975-7.00315-6