Indicator assessment of the reliability of mine ventilation and degassing systems functioning
Abstract
The gas emission control in the mines is operated by ventilation and degassing systems that ensure the aerological safety of the mines or minimize the aerological risks. The ventilation system of the mine and its individual sites includes a significant number of technical devices and equipment, and the air tubes are mainly mining workings, the condition of which determines the quality of the ventilation network (its capacity) and depends on a number of mining factors. Similarly, one of the most important elements of the degassing system, which includes its own chain of technological equipment, are wells, and in some cases, mining workings. Thus, mine ventilation and degassing systems cannot be attributed to purely technical systems, since they include mining elements characterized by high variability of the determining parameters. To assess their reliability, it is necessary to use various combined methods that include additional characteristics in relation to the mining component. At the same time, the reliability of technical devices that ensure the functioning of mine ventilation and degassing systems largely determines the efficiency (stability and reliability) of these systems and, consequently, affects the level of aerological risks. The described approach to assessing the reliability of ventilation and degassing systems of coal mines when analyzing aerological risks is based on the developed system of risk indicators for the methane factor and will allow determining the risk dynamics in automatic mode based on monitoring the parameters of the ventilation and degassing system state.
Introduction
The mining industry is characterized by the most dangerous working conditions, especially during underground mining, which significantly affects the technical and economic indicators of mining companies [26]. Ensuring safe and healthy working conditions is one of the most important guarantees of the state, fixed by the main federal laws [2]. Occupational safety management [2] provides for a system of organizational and technical measures, including control and monitoring of compliance with established norms and rules [20]. At gassy high-efficiency coal mines methane release is the main factor limiting the load on the stoping and tunneling faces, as well as representing a serious danger [8]. The transition to a risk-oriented approach in control and monitoring activities requires the development of a risk indicators system that can be used to assess the work safety at any enterprise. To reduce the explosion hazard in coal mines, a complex of protective technologies is used, which is called gas emission control in coal mines. This complex performs a targeted redistribution of methane-air mixture flows in the mining network or reduces their gas content by engineering methods. To assess the effectiveness of this process in terms of its contribution to reducing production risk, it is necessary to have a system of indicators (risk indicators) that reflect the degree of change in the level of danger in relation to the reference – the minimum possible or technically achievable.
The article is devoted to the substantiation of such an indicators system that would allow determining the risk dynamics by the methane factor in an automatic mode based on monitoring the parameters of the ventilation and degassing system state.
Methodology. The transition to a risk-oriented approach in the implementation of state monitoring and control activities implies the need to identify key values protected by the state, as well as key risks and their sources, opportunities to influence these sources in order to reduce risks. According to the selected key risks, it is planned to develop enlarged groups of mandatory requirements and ways to monitor their compliance. Aerological risks in underground coal mining are among the key [13].According to the annual reports of Rostechnadzor (2019 and 2020), the accident rate of coal mines by aerological factors remains quite high. In addition, if we consider the causes of major accidents at gas mines, their catastrophic development is always due to the insufficient state of ventilation, degassing and dust suppression systems. However, the systems that ensure environmental safety are quite complex and include many heterogeneous elements, which complicates the organization of determining factors control. The most important role for hazardous production is played by the state system of monitoring and control [2], while the effectiveness of occupational safety management is significantly influenced by the strategy of production control [28, 32]: with a high degree of rigidity, efficiency increases, but only in the short term; with a freer approach, the general safety culture gradually increases [29]. Therefore, the accepted course of changing the state monitoring strategy [11] seems progressive, but at the first stage of implementing a risk-oriented approach, an increase in production risks for coal mines (primarily aerological) is possible due to the transition to the control of enlarged safety parameters (indicators) [9].
The generally accepted definitions of production and acceptable risks as probabilistic characteristics have not found wide application in mining [9] due to the high stochasticity of mining technical factors of production risks and their constant variability [13]. Similarly, the methods of evaluating mine ventilation systems based on the reliability theory proposed by domestic and foreign scientists [25, 31] remained unclaimed. Determining probabilistic estimates requires additional labor, even the creation of an appropriate service that will only deal with statistics and its analysis. It seems more expedient to use as risk indicators those parameters that are actually monitored at enterprises. This idea is considered on the example of technical systems – the most predictable element of the technological scheme. It is proposed to assess the risks associated with the operation of equipment by indicators that determine the reliability of mining equipment.
In domestic practice, technologies for ensuring the explosion safety of coal mines by the methane release factor are called gas release control [12]. The equipment of providing aerological safety systems (ventilation, air conditioning, degassing, monitoring) is their most important element, since its uninterrupted operation and reliability [5] are the key to maintaining the required parameters of the mine atmosphere.
The purpose of gas emission control is to comply with safety requirements for the concentrations of harmful gases in mine workings [17], primarily in excavation and preparatory sites, where the most intense release of gases and dust (including explosive) occurs. Gas emission control is provided by various methods: aerodynamic (ventilation), gas-dynamic (degassing) and combined, as well as methods of their implementation (see figure).
Aerodynamic methods dilute dangerous gases or redistribute their flows in the mining system of the site due to multi-entry development for separate dilution of the gas balance components of the site and change the direction of the leakage flow through the developed space [19]. Mine ventilation systems do not reduce the overall gas content of mine workings, their function is to dilute and/or redistribute gas flows through the workings. Aerodynamic methods are implemented by means of a mine ventilation system, the main elements of which are: the mine ventilation network (MVN), draught sources, ventilation structures and air distribution regulators. Thus, this system is not purely technical, since MVN includes mining workings and developed spaces, which in this case are air tubes, the condition of which depends on the geomechanical reactions of the rock mass to mining operations. The influence of the stressed state of the rock mass on the aerodynamic resistance of the ventilation network does not depend on the design characteristics of the workings with the same certainty as in mechanical systems [24]. Therefore, the mine ventilation system is referred to as mining engineering systems.
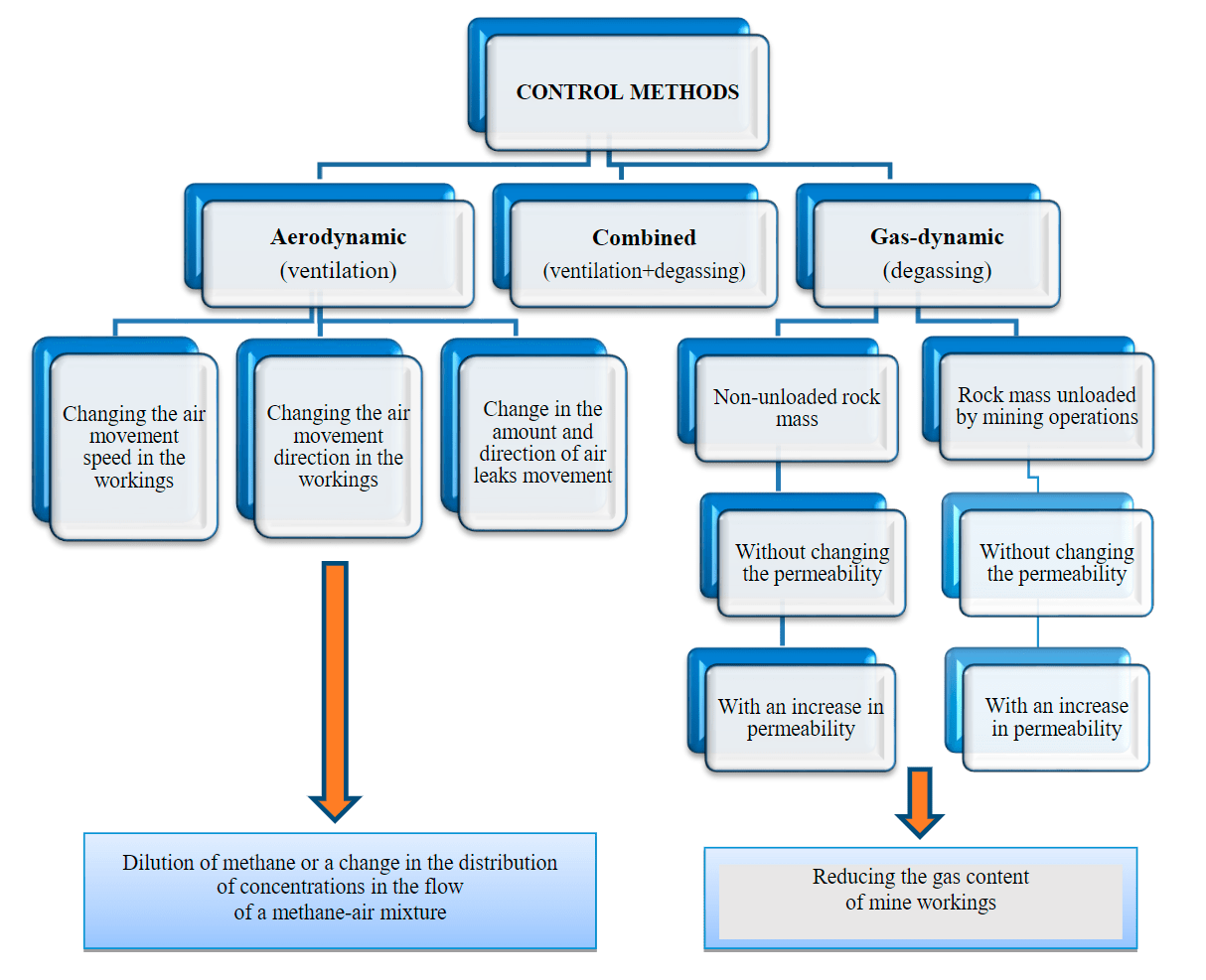
Classification of gas emission control methods and methods of their implementation
Gas-dynamic methods reduce the gas content of mine workings due to the purposeful and controlled impact on the rock mass by engineering-physical and/or physical-chemical methods. At the same time, the impact on the rock mass can be made both in advance – before the start of mining operations (i.e. on a non-unloaded rock mass), and during operation (on a rock mass unloaded by mining operations) [6]. In the latter case, the permeability of the rock mass and the gas output of the sub-worked coal seams significantly increas under the influence of mineral extraction, additional impact may not be made. However, in recent years, technologies have been developing to improve the efficiency of gas-dynamic methods, including on unloaded formations from underground workings. Gas-dynamic methods are implemented by degassing systems that provide forced extraction from the rock mass and isolated removal of methane, avoiding the atmosphere of mine workings; or blocking methane in the pores and micropores of carbonaceous matter, preventing its release into the workings during tunneling or stoping excavation [30].
The degassing system (DGS) includes: wells or mine workings, a system of degassing pipelines, vacuum pumps, start-up and control equipment and devices. This system also cannot be attributed to a purely technical one, since along with technical means and devices it includes mining elements (workings and wells, developed spaces). The network of degassing pipelines also interfaces with MVN and, like MVN, changes in time and space as the stoping and tunneling faces move.
At gassy mines, high extraction productivity can be ensured only by combined methods of gas emission control, i.e. a combination of ventilation and degassing methods [7]. To improve the management efficiency, the risks assessment of methane emission control system failure should be carried out in the mode of continuous automated control of equipment operating modes [27].
The efficiency of the combined gas emission control system during operation determines the level of risk [14] by the methane factor. The indicators characterizing the efficiency of this system are the non-exceeding the permissible concentrations of methane in the mine atmosphere according to the safety rules, the achieved degassing coefficient of each source, as well as the reliability and stability of the functioning of each element of this system [25]. The first two indicators are determined in a continuous mode by air-gas control systems. There is no such unambiguity regarding the reliability indicators for the gas emission control system.
The terms and definitions used in the theory of reliability are regulated by GOST 27.002-2015 “Reliability in engineering. Basic concepts. Terms and definitions”. Reliability is the property of an object to preserve in time the ability to perform the required functions in the specified modes and conditions of use, maintenance, repairs, storage and transportation. When assessing its reliability, an object is understood as a set of elements that are structurally interconnected so that the breakdown (failure) of one of the elements of the system can lead to the failure of several related elements or the system as a whole.
Reliability is a complex property of an object that includes a number of the following simple properties: trouble-free, maintainability, recoverability, durability, persistence, readiness. These properties are evaluated statistically based on the probabilistic approach, except for the latter. Readiness – the ability to maintain a state in which an object can perform the required functions in the specified modes and conditions of use. It is a derivative of the properties of reliability, maintainability and recoverability and is determined by complex reliability indicators:
- availability/unavailability coefficients (the probability that the object will/will not be in a working state at a given time);
- operational readiness (the probability of working capacity at the moment and trouble-free operation for a given time);
- the coefficient of technical use (the ratio of the total time spent in working condition to the total operating time, including downtime for maintenance and repair for the same period);
- the efficiency rate (the ratio of the efficiency indicator specified in the technical documentation for the object to the nominal value of this indicator, calculated on the condition that the failures of the object do not occur during the considered period of operation).
Quantitative and qualitative requirements for the mass-produced technical objects reliability are established in the regulatory and technical documentation, for re-created technical systems and objects – in the engineering and design documentation. To determine the reliability indicators of technical systems, various methods are used (GOST 27.002-2015 “Reliability in engineering. Basic concepts. Terms and definitions”):
- calculated, involving calculations based on reference data on the reliability of components and components of the object; on statistical data on the reliability of analog objects, used materials and other information known from literature data or operational experience;
- calculation and experimental, combining the calculation method and experimental determination of reliability indicators of all or some of the components of the object;
- experimental, when reliability indicators are determined based on statistical processing of data obtained during testing or operation of the object as a whole.
The last group of methods refers to complex objects and technical systems with a large number of diverse factors. Just this group includes mine ventilation and degassing systems. A distinctive characteristic of these systems is that they constantly transform in time and space as the mining front moves forward. The complexity of their assessment by the methods of reliability theory lies in the significant variability of operating conditions, which does not allow extending statistically obtained quantitative estimates of properties that define reliability as the probability of trouble-free operation, obtained in different periods, even to the same object. At the same time, reliability indicators for technical elements – machines, devices, start – up equipment-can be determined by the described methods, since these elements are subject to predicted wear, which can be estimated both by the calculation method (according to reference information) and experimentally. Such calculations are carried out during the development of the energy-mechanical part of the mine project or at the stage of planning the mining operations development, if it is planned to modernize the equipment.
To assess the reliability of mine ventilation and degassing systems, it is necessary to use various combined methods that include additional characteristics in relation to the mining component.
Discussion. A complex reliability indicator of the ventilation-degassing system can be represented as a dimensionless indicator corresponding to its stable and trouble-free operation with the parameters of the air supply and the pumped methane-air mixture set in the project and regulated as the face moves It will be the sum of indicators reflecting the features of operation and maintenance of MVN and DGS, taken with their own weight coefficients:
where НMVN, НDGS are reliability indicators of ventilation and degassing systems, respectively; KMVN, KDGS are coefficients showing the weight value of performing a full range of work at the current moment in terms of the quality of ventilation and degassing systems, respectively, unit fraction.
The reliability indicators НMVN and НDGS are assumed equal to 1 at the time when the coal mine operations start. This is the maximum possible value that corresponds to the reliability of the equipment defined by the project. The values of these indicators, if everything is done in accordance with the project, remain constant for the entire period of the coal mine operation. In case of deviation from the design solutions, their change should correspond to the degree of increase in production risks. Based on the technical condition of MVN and DGS, the values of the coefficients KMVN and KDGS can vary from 0 to 1 in accordance with the actual state of affairs, depending on the completeness of the mandatory maintenance of the equipment. The rating scale and its details are set by the enterprise.
The life support of any coal mine directly depends on the correct design, installation, operation and maintenance of the ventilation system, the most important elements of which are the main fans (MF) [12, 21, 26].
The reliability of any machine is determined not only by its design features, but also by its technical condition as an object as a whole, and individual elements (nodes). Long-term and trouble-free operation of the MF depends on the correct choice of the type and brand of the fan, as well as its correct installation, operation and timely maintenance [23]. When creating new machines, as well as when choosing them during the design of mines, the reliability indicators of their operation are determined by calculation methods. However, it is impractical to make such time-consuming calculations during operation. It is much more convenient and faster to determine the risks of machine failures through indicator parameters.
A complex reliability indicator of the MF can be represented as a dimensionless indicator corresponding to its stable and trouble-free operation with the air supply parameters specified in the project and regulated as the production capacity of the mine changes. It will be the sum of indicators reflecting the features of operation and maintenance of MF, taken with their own weighting factors:
where Нpi, Нi, Нiae, Нr, Нm are reliability indicators of preparation for installation and installation of MF, installation and adjustment of auxiliary equipment, run-in of MF, maintenance and repair, respectively; Kpi, Ki, Kiae, Kr, Kmare coefficients showing the weight value of performing a full range of works at the current moment for preparing for the installation of MF, for the installation of MF, installation and adjustment of auxiliary equipment, run-in of MF, timely and complete maintenance or repair, respectively, unit fraction.
The reliability indicators Нpi, Нi, Нiae, Нr, Нm should be taken equal to 1 at the beginning of MF operation (these are the best indicators in accordance with the project). The values of these indicators, if everything is done in accordance with the technology, remain constant for the entire period of MF operation. The values of the coefficients Kpi, Ki, Kiae, Kr, Kmdepending on the technical condition of MF, can vary from 0 to 1, reflecting the current quality of service – what proportion of the planned work is fully completed. In this case, the maximum value of НMF will be 5. If some type of work is not completed fully or with violations, the value of this indicator decreases. The permissible reduction is set by the enterprise in accordance with the technical requirements for each type of equipment.
The maintenance and repair reliability indicator includes a number of parameters, the values of which must be constantly monitored, since they show the current technical condition of the MF. Depending on the schedule of maintenance or repair, the results and deadlines of the following types of work should be monitored: monthly, daily and two-week maintenance; monthly repair maintenance; quarterly and semi-annual maintenance; annual and two-year audit and adjustment of automation systems.
When assessing the risk based on reliability indicators, the deviation of the complex indicator from the maximum possible (assumed to be equal to 1) on the basis of the given structure of the complex indicator, it is easy to determine which component measures are needed, and immediately take the necessary control actions.
To ensure high efficiency and reliability of the MF operation, the following types of work must be performed qualitatively, in accordance with the safety requirements and technical operation rules [1]: preparation for installation; installation of the fan; checking the completeness, safety and depreservation of the equipment; installation of frames on the foundation; filling the frames with concrete mortar; installation and installation of rotor shafts; installation of rotor shafts mounted on a common frame or on a foundation without a frame; revision of the bearing units of the rotor shafts [4]; centering of the shafts; installation and adjustment of the fan rotor bearing lubrication systems; installation and adjustment of the guide devices of centrifugal fans; installation and adjustment of the guide and straightening devices of axial fans.
Maintenance and repair of fan installations is carried out according to a continuous method, i.e. the elements of the equipment are inspected and repaired not simultaneously, but alternately in accordance with the established procedure. The structure of the maintenance cycle of fan installations has the form: (M1) – (M2) – (M4), where M1, M2, M4 are monthly, daily and two-week maintenance, respectively. The structure of the repair cycle is as follows: MM – RS1 – M1 – M2 – RS1 – M1 – (M2 – – ASA) – RS1 – M1 – M2 – RS1 – M1 – AST – RS1 – M1 – M2 – RS1 – M1 – (M2 – ASA) – RS1 – M1 – – M2 – RS1 – M1 – AST – RS1 – M1 – M2 – RS1 – M1 – (M2 – ASA) – RS1 – M1 – M2 – RS1 – M1 – +… – MM, where RS1 is monthly repair service; M1 and M2 are quarterly and semi-annual maintenance, respectively; ASA и AST are annual and two-year audit and adjustment of automation systems, respectively; MM is major maintenance.
In order to detect and eliminate failures in a timely way, maintenance and repair personnel perform maintenance of the working and standby fan installations [22]. The current repair carried out during operation consists in replacing and repairing individual parts of the fan unit and adjusting them, which guarantees its operability. Repair services and scheduled maintenance are performed for an installation that has been forcibly placed in reserve.
Thus, the reliability of the MF operation during use is determined by the quality of maintenance for each type of operation (the reliability indicator should include parameters that characterize the correctness of the installation and mounting of the MF), as well as the timely scheduled maintenance, revision, adjustment and scheduled repairs. The quality of maintenance and repairs during operation depends on the qualification and discipline of the operating personnel and engineering control. Thus, the indicators will also reflect organizational risks.
Similarly, it is possible to determine reliability indicators for booster fans, air distribution regulators, and the state of the ventilation network.
The degassing system reliability [16] also significantly affects the level of aerological risk of the coal mine operation and ensures the stability of the operation of mine methane consumers [3]. The reliability and efficiency of coal mine degassing systems depends on the correct choice of the method and scheme of degassing, high-quality design, installation, operation and maintenance of equipment [10, 15, 18].
The reliability of the mining component (the method and scheme of degassing, well parameters and operating modes of vacuum pumps) can be determined by the efficiency of methane extraction – achieving the required degassing coefficient. This is one of the main indicators of system reliability.
For technical means, standard reliability indicators (GOST 27.002-2015 “Reliability in engineering. Basic concepts. Terms and definitions”) at the design stage can be determined by computational methods of reliability theory. At the stage of operation, it is impractical to perform time-consuming calculations with uncertain parameters of the constants included in them for operational control of the reliability of the system functioning. It is more convenient to use indicators of aerological risk.
The DGS reliability indicator is a dimensionless indicator corresponding to the stable and trouble-free operation of the system with the supply of a methane-air mixture to the mine surface with the parameters specified in the project and/or adjusted as the production capacity of the mine changes. The long-term and trouble-free operation of the degassing system depends on the correct choice of equipment, as well as its correct installation, operation and timely maintenance.
The reliability indicator can be represented as the sum of indicators reflecting the features of operation and maintenance of DGS:
where Нdw, Нvps, Нp, Нm are reliability indicators of degassing wells packoff and operation of vacuum pumping stations, underground degassing pipelines packoff, maintenance and repair of DGS equipment, respectively; Kdw, Kvps, Kp, Kmare coefficients showing the weight value of performing a full range of work at the current moment for degassing wells packoff, operating vacuum pumping stations, underground vacuum degassing gas pipelines packoff, timely and complete maintenance or repair of DGS equipment, respectively, unit fraction.
The reliability indicators of Нdw, Нvps, Нp, Нm are assumed to be equal to 1 at the time of the DGS operation start. The values of these indicators, if everything is done in accordance with the project, remain constant for the entire period of the DGS operation. The values of the coefficients of Kdw, Kvps, Kp, Kmdepending on the technical condition of the DGS, can vary from 0 to 1.
The degassing system of a coal mine is a complex multicomponent technical system, the reliability of which depends on many factors, therefore, standard reliability indicators must be determined by computational and experimental or only experimental methods. For operational management of aerological risks associated with DGS, it is advisable to use reliability indicators, which include parameters that characterize the quality of well construction, the correctness of installation and operation of DGS, as well as timely scheduled maintenance, audit, adjustment and scheduled repairs.
Constant monitoring of the DGS reliability indicator, which includes a number of indicators of DGS elements, will allow timely detection of deviations in their operation, as well as in the timing and quality of the equipment maintenance and repair schedule, which includes the following types of work: daily and two-week maintenance; monthly maintenance and repair maintenance; quarterly and semi-annual maintenance; annual and two-year audit and adjustment of equipment.
Many mining enterprises have automated monitoring of safety parameters, including those characterizing the state of the mine atmosphere. In the near future, such a system should become mandatory for all companies, as an information base for multifunctional security systems. The data used to calculate equipment reliability indicators should be stored in a common information database. Algorithms for their determination should be included in a single system of intelligent data processing to support the technical decisions made. This will allow automating the operational control of the maintenance of the equipment of ventilation and degassing systems and improving its quality, as well as identifying unfavorable trends in reducing the efficiency of systems associated with the use of worn-out equipment. The storage of operational information will allow us to more accurately determine the causes of accidents, including catastrophic ones (explosions, fires) and identify those responsible for not taking measures to reduce risks during post-accident investigations.
Conclusion
Based on the research results, the structure of indicators is proposed, which allows ensuring the following at the stage of mine operation:
- systematic identification of unfavorable trends in the reliability of equipment of mine ventilation and degassing systems as a potential hazard;
- identification of factors that cause the risk of accidents and weak links in the system related to the operation of equipment;
- selection of measures to reduce the risk of ventilation and degassing systems equipment failures;
- formation of a database for the rational organization of preventive maintenance, repair and control of systems equipment that ensure aerological safety;
- development of a database that facilitates the post-accident investigation of accidents and provides targeted development of measures to prevent similar accidents.
This approach to the analysis and management of the mining equipment reliability can be re-commended for use in the current planning of mine production processes. To implement it, it is necessary to expand the range of tasks solved by multifunctional security systems, which will provide not only the formation of databases on changes in the parameters of the state of technology, ventilation and degassing equipment, but also their analytical processing, including determining the dynamics of deviations of the controlled parameters from the design values and presenting information in a form convenient for making decisions.
References
- Alymenko N.I., Alymenko D.N. Fan installations for various mining and technical conditions. Gornoe ekho. 2008. N 3-4, p. 34-41 (in Russian).
- Artemev V.B., Galkin V.A., Kravchuk I.L. Industrial safety (organizational aspect). Moscow: Gornaya kniga, 2016, p. 144 (in Russian).
- Bezpflyug V.A. Experience in the utilization of coal mine methane in Germany and the possibility of its utilization in Russia. Ugol. 2006. N 8, p. 66-68 (in Russian).
- Gerike B.L., Mokrushev A.A. Recognition of rolling bearing defects in mining machine reducers. Naukoemkie tekhnologii razrabotki i ispolzovaniya mineralnykh resursov. 2017. N 3, p. 192-197 (in Russian).
- Gerike P.B., Gerike B.L. Formation of a single diagnostic criterion for assessing the technical condition of mining equipment. Gornoe oborudovanie i elektromekhanika. 2021. N 2 (154), p. 17-22. DOI: 10.26730/1816-4528-2021-2-17-22 (in Russian).
- 6. Slastunov S.V., Ponizov A.V., Sadov A.P., Khautiev A.M.-V. Hydraulic separation of coal seams for their effective degassing preparation through underground wells. Gornyi informatsionno-analiticheskii byulleten. 2020. N 6-1, p. 15-25. DOI: 10.25018/0236-1493-2020-61-0-15-25 (in Russian).
- Grazhdankin A.I. Hazard analysis and accident risk assessment in the justification of industrial safety. Bezopasnost truda v promyshlennosti. 2013. N 6, p. 90-92 (in Russian).
- Grazhdankin A.I., Pecherkin A.S., Sidorov V.I. Will quantitative risk assessment replace compliance with industrial safety requirements? Bezopasnost truda v promyshlennosti. 2012. N 10, p. 43-45 (in Russian).
- Grazhdankin A.I., Lisanov M.V. Modernization of industrial safety requirements. Risk-based approach. Bezopasnost truda v promyshlennosti. 2013. N 1, p. 77-79 (in Russian).
- Ermakov A.Yu., Kachurin N.M., Senkus Val.V. A systematic approach to ensuring ventilation and safety of coal mines based on the air-gas-dynamic factor. Gornyi informatsionno-analiticheskii byulleten. 2018. N 7, p. 212-218. DOI: 10.25018/0236-1493-2018-7-0-212-218 (in Russian).
- Zaburdyaev V.S. Technological schemes for the preparation, processing and control of gas release, which reduce the risks of explosions of methane-dust-air mixtures in coal mines. Gornyi informatsionno-analiticheskii byulleten. 2015. N S1, p. 373-381 (in Russian).
- Kaledina N.O., Meshcheryakov D.A. Aerological safety of coal mines. Gornyi informatsionno-analiticheskii byulleten. 2011. N 1, p. 227-237 (in Russian).
- Kaledina N.O., Malashkina V.A. Reserves for increasing the efficiency of underground degassing of coal seams in order to improve the working conditions of miners. Gornyi zhurnal. 2017. N 6, p. 86-89. DOI: 10.17580/gzh.2017.06.17 (in Russian).
- Kaledina N.O. Risk-based approach in ensuring industrial safety of mining enterprises. Gornyi informatsionno-analiticheskii byulleten. 2020. N 6-1, p. 5-14. DOI: 10.25018/0236-1493-2020-61-0-5-14 (in Russian).
- Kurta I.V. The management of gas emission at the excavation sites of coal mines is a key factor in creating safe working conditions. Journal of Mining Institute. 2012. Vol. 195, p. 108-110 (in Russian).
- Malashkina V.A. Investigation of the possibilities of improving the efficiency of underground degassing of coal mines. Gornyi informatsionno-analiticheskii byulleten. 2019. N 9, p. 131-137. DOI: 10.25018/0236-1493-2019-09-0-131-137 (in Russian).
- Malashkina V.A. Monitoring the effectiveness of the coal mine degassing system is the basis for the safe work of miners. Gornyi informatsionno-analiticheskii byulleten. 2020. N 6-1, p. 38-45. DOI: 10.25018/0236-1493-2020-61-0-38-45 (in Russian).
- Malashkina V.A. Directions for improving the efficiency of using coal mine degassing systems. Gornyi informatsionno-analiticheskii byulleten. 2019. N 6, p. 206-214. DOI: 10.25018/0236-1493-2019-06-0-206-214 (in Russian).
- Matveev V.V. Development, research and implementation of control and automation systems for methane removal complexes at coal mines. Izvestiya vysshikh uchebnykh zavedenii. Gornyi zhurnal. 2010. N 2, p. 71-77 (in Russian).
- Artemev V.B., Galkin V.A., Makarov A.M. et al. Mechanism for preventing the implementation of a dangerous production situation. Ugol. 2016. N 5, p. 73-77. DOI: 10.18796/0041-5790-2016-5-73-77 (in Russian).
- Patrushev M.A., Samoilenko E.Ya., Us V.N. Improving the coal mines ventilation: from the experience of developing and implementing effective ventilation schemes. Donetsk: Donbas, 1976, p. 126 (in Russian).
- Sigoshin A.V. Analysis of the main failures of the main fans with an expired standard service life. Izvestiya Uralskogo gosudarstvennogo gornogo universiteta. 2003. Iss. 16, p. 18-22 (in Russian).
- Sigoshin A.V. Problems of assessing the technical condition of the main fans. Izvestiya Uralskogo gosudarstvennogo gornogo universiteta. 2003. Iss. 17, p. 343-346 (in Russian).
- Ushakov V.K. Analysis of costs for the creation and operation of mine ventilation systems in order to improve labor safety. Gornyi informatsionno-analiticheskii byulleten. 2018. N 6, p. 214-221. DOI: 10.25018/0236-1493-2018-6-0-214-221 (in Russian).
- Ushakov V.K. Dynamic hierarchical segmentation of mine ventilation systems when modeling ways to improve the aero-logical labor safety. Gornyi informatsionno-analiticheskii byulleten. 2019. N 12, p. 76-85. DOI: 10.25018/0236-1493-2019-12-0-76-85 (in Russian).
- Chmykhalova S.V. A systematic approach to risk assessment that helps to prevent losses and improve the mining safety. Gornyi informatsionno-analiticheskii byulleten. 2020. N 6-1, p. 146-153. DOI: 10.25018/0236-1493-2020-61-0-146-153 (in Russian).
- Shershukova K.P., Telyuk A.S. Risk assessment of hazardous production facilities for the automatic safety systems design. Gornyi informatsionno-analiticheskii byulleten. 2012. N S6, p. 82-89 (in Russian).
- Gi Heung Choi. Effectiveness of direct safety regulations on manufacturers and users of industrial machines: its implications on industrial safety policies in Republic of Korea. Safety and Health at Work. 2017. Vol. 8. Iss. 1, p. 59-66. DOI: 10.1016/j.shaw.2016.09.005
- Nikulin A., Nikulina А.Y. Assessment of occupational health and safety effectiveness at a mining company. Ecology, Environment and Conservation. 2017. Vol. 23. N 1, p. 351-355.
- Krings Т., Gerilowski К., Buchwitz М. et al. Quantification of methane emission rates from coal mine ventilation shafts using airborne remote sensing data. Atmospheric Measurement Techniques. 2013. Vol. 6. Iss. 1, p. 151-166. DOI: 10.5194/amt-6-151-2013
- Luo Wengui, Xie Xianping, Xiao Houzao et al. Reliability calculation of mine ventilation network. Procedia Engineering. 2014. Vol. 84, p. 752-757. DOI: 10.1016/j.proeng.2014.10.492
- Cheng-lin Miao, Meng-meng Duan, Xin-xiu Sun, Xin-yu Wu. Safety management efficiency of China's coal enterprises and its influencing factors – Based on the DEA-Tobit two-stage model. Process Safety Environmental Protection. 2020. Vol. 140. p. 79-85. DOI: 10.1016/j.psep.2020.04.020