Concept of technology for determining the permeability and porosity properties of terrigenous reservoirs on a digital rock sample model
- 1 — Senior Lecturer Northern Arctic Federal University ▪ Orcid ▪ Elibrary ▪ Scopus ▪ ResearcherID
- 2 — Ph.D., Dr.Sci. Professor Northern Arctic Federal University ▪ Orcid ▪ Elibrary ▪ Scopus ▪ ResearcherID
Abstract
The aim of the article is to form the concept of technology for determining the permeability and porosity properties of terrigenous reservoirs using mathematical modeling methods on a digital rock sample model. Digital rock sample modeling is used to assess geological oil reserves. The article presents the concept of digital rock sample modeling technology, which allows carrying out qualitative investigations to determine the permeability and porosity characteristics of the formation, including modeling the pore space and filtration processes. The essence of the concept is that the simulation model of the microstructure for the digital model is formed on the basis of a large number of parameters obtained during lithological and petrographic investigations of thin sections, a study of the sludge and geophysical investigations of wells. The acquired model can be used as a basis for subsequent modeling of filtration processes. Conductivity of single channels of the formed model can be calculated using molecular dynamics methods, models of Boltzmann's lattice equations, and other mathematical models and methods. Based on the results of the study carried out, the application of stochastic packing methods for modeling the structure of the pore space in the digital rock sample model of terrigenous reservoirs is substantiated. In connection with the development of computer and nanotechnologies and their use in the oil and gas industry, solutions that allow obtaining adequate results of digital rock sample models are of high importance and relevance for the production sector. It is especially important to use digital rock sample models in the study of reservoir rocks of shelf fields in the western part of the Russian Arctic, oil shales, rocks represented by loose weakly cemented reservoirs, and others, which are complex for physical experiments.
Introduction. Investigations to determine the permeability and porosity properties are an integral part of the structured laboratory study of the rock sample material of the oil reservoir. Permeability and porosity properties are determined by a system of lithological parameters affecting the size, ability to contain and transmit fluids, their configuration, and location in the rock, i.e., have a pore space, and characterize the industrial value of the rock as a reservoir [3].
Experts in laboratory investigations of rock sample material do not always have a sufficient amount of rock samples from wells to conduct research; in some cases, it is not possible to acquire rock sample material. An example is the case when a rock sample from the investigated wells is represented by loose, weakly cemented and other rocks, which are difficult to research. Sometimes the rock sample from investigated wells is absent [1]. With the help of a digital rock sample model, it becomes possible to effectively refine and supplement the calculated parameters of the permeability and porosity properties of reservoir rocks obtained in the process of a laboratory study of a rock sample, reducing the likelihood of errors in the results.
At present, digital rock sample modeling technology is a popular and developing trend in the assessment of geological oil reserves [4, 5, 7, 9]. In Russia, digital rock sample modeling technology is at an early stage of development, the first laboratory for digital rock sample modeling was opened in 2016 at the Skolkovo technopark.
Analysis of the current state of the problem. Researchers have taken various approaches to assessing the permeability and porosity properties of reservoir rocks using digital rock sample models. 3D-tomography of rock samples and petrographic thin sections was used in work [6], for modeling pores and particles of rock samples. In [8], to obtain data on the microstructure of the reservoir, 2048-voxel tomograms and X-ray diffraction patterns of the terrigenous reservoir were used.
Modeling of filtration processes occurring in the formation was also carried out in various works using a number of mathematical models and calculation methods. The most common are pore network models, Boltzmann’s lattice equations model, smoothed particles method, diffuse boundary models, models based on solutions of Navier – Stokes equations, finite volume methods [10].
Due to the lack of clear advantages for these methods in comparison, there is currently no single idea about which of the models and methods are most suitable for modeling filtration processes on a digital rock sample model. Nevertheless, the finite volume methods in [12,15],and the Boltzmann’s lattice equations in [13,14], are most often used to estimate the properties of filtration flows on a pore scale. However, the numerical approaches used in these methods reduce the accuracy of determining the permeability, which was shown in the works [10,11].
Based on the mentioned above, it was determined that the formation of an effective concept for the creation and functioning of the technology for determining the permeability and porosity properties of terrigenous reservoirs using mathematical modeling methods on a digital rock sample model is a complex but very urgent research and applied problem.
Concept of digital rock sample modeling technology. The authors have developed the concept of digital rock sample modeling technology, which makes it possible to determine the permeability and porosity characteristics of the reservoir by mathematical modeling methods with a sufficient degree of accuracy. The essence of the concept is that the simulation model of the microstructure of the digital model works on the basis of a large number of parameters obtained during the lithological and petrographic study of thin sections and other investigations.
Obviously, the digital rock sample model is most valuable in the absence of rock sample material. In this case, samples of cuttings from the reservoir under study, as well as the results of geophysical investigations of wells, can be used as a material for conducting research.
Modeling the pore space of the model. In the process of creating a digital rock sample model, the generation of its dense stochastic packing is used to model the structure. At the same time, based on the distribution of the rock grains’ sizes, it is possible to apply the Monte Carlo method and other mathematical models and methods designed to study random processes. Grain sizes and other parameters of created models can be used according to the results of lithological and petrographic investigations of thin sections and data from the granulometric analysis of rocks using laser diffraction or other methods.
Latent-semantic analysis of lithological-petrographic thin sections and other methods of clarifying the geometric structure of the simulated rock grains, as well as their correspondence to the created model can be applied in the future. This will allow an increase in the degree of similarity of the created model to the physical samples of the studied reservoirs.
Pore space modeling should be carried out in several stages. At the initial stage of creating the structure of the pore space, primitives of the grain shapes (particles) are simulated. Taking into account the particle size distribution obtained from the results of determining the granulometric composition of the rock by laser diffraction, lithological and petrographic study of thin sections, or other methods, an image is set for each layer of the created model. Figure 1 shows an example of lithologic-and-petrographic thin sections used to define images for model layers. Results of lithologic and petrographic analysis of thin sections is below:
Thin section | Thin section porosity, % | Grains size, mm | Sorting | Brief lithological description |
1 | 10 | 0,22-0,40 | Good | Small and medium-grained oligomictic quartz sandstone with combined cement, pigmented with bitumen, porous. The texture is spotted due to uneven pigmentation of bituminous organic substances (BOS). Psammitic structure, fine- and medium-grained |
2 | До 15 | 0,20-0,45 | Good | Small and medium-grained oligomictic quartz sandstone with combined cement, pigmented with bitumen, porous. The texture is spotted, due to the pigmentation of BOS, porous. Psammitic structure, fine- and medium-grained |
3 | 10 | 0,15-0,35 | Good | Small and medium-grained oligomictic quartz sandstone with contact type cementation (compaction), pore-type carbonate cement, rare quartz regeneration cement, motted oil-saturated. The texture is spotted due to the uneven distribution of carbonate material and BOS |
4 | 10-12 | 0,20-0,40 | Medium | Fine and medium-grained oligomictic quartz sandstone with combined cement, unevenly oil-saturated. The texture is spotted. Psammitic structure, fine- and medium-grained |
5 | 10 | 0,25-0,40 | Medium | Small and medium-grained oligomictic quartz sandstone with combined cement, pigmented with bitumen, porous. The texture is spotted, due to the pigmentation of BOS. Psammitic structure, fine- and medium-grained |
6 | 10-12 | 0,22-0,35 | Medium | Small and medium-grained oligomictic quartz sandstone with cementation of contact type, areas with pore type carbonate cement, pigmented with bitumen. The texture is spotted. Psammitic structure, fine- and medium-grained |
7 | 10-15 | 0,15-0,32 | Medium | Medium and fine-grained oligomictic quartz sandstone contact-type cementation, regenerative quartz cement, with local pore-type carbonate cement, spottily oil-saturated. The texture is spotted due to uneven oil saturation. Psammitic structure, fine- and medium-grained |
8 | 10 | 0,25-0,35 | Good | Medium-grained oligomictic quartz sandstone with contact-type cementation, sporadically with pore-type carbonate cement and quartz regeneration cement. The texture is spotted. Psammitic medium-grained structure |
9 | 10 | 0,25-0,40 | Good | Medium-grained oligomictic quartz sandstone with contact type cementation, regenerative quartz cement, sporadically with chlorite-hydromical cement of the film-porous type. The texture is spotted. Psammitic medium-grained structure |
10 | 10-12 | 0,22-0,40 | Good | Medium-grained oligomictic quartz sandstone with combined cement, pigmented with bitumen, porous. The texture is spotted, due to the pigmentation of BOS. Psammitic medium-grained structure |

An important stage in the implementation of a digital rock sample model is taking into account the clay content of the modeled particles during its creation. For this, at the next stage of modeling the pore space, it is necessary to calibrate the created models by porosity, taking into account the clay content. The content of clay minerals, as well as their composition and morphology, significantly affect the permeability of terrigenous reservoirs. In addition, clay content affects the oil and gas saturation of sandy-clay reservoirs [2].
At the final stage, parameters of the physical model, calibration algorithm, and presentation of the results are indicated.
Modeling clay content in a digital rock sample model can be implemented by partially filling the microstructure with various types of cement, the permeability of which is determined through numerical experiments on molecular dynamics, and modeling the flows in microchannels, as well as using other models and methods. In this case, the desired coefficient of clay content for the simulated reservoir can be determined during geophysical investigations of wells using the self-potential method or gamma-ray logging.
In the future, the obtained structure can be used to estimate the coefficients of open and total porosity. At the same time, the coefficient of total porosity can be taken as the ratio of the total volume of voxels free from modeled grains of the rock (particles) to the volume of all voxels in the model space, and the coefficient of open porosity, in its turn, can be estimated as the ratio of the volume of voxels composing an open communicated space free of modeled grains of the rock (particles) to the volume of all voxels in the model space.
Modeling of filtration processes. The generated pore space model can be used as a basis for subsequent modeling of filtration processes. At this stage, the conductivity of single channels in the created model can be calculated using the methods of molecular dynamics, models of Boltzmann’s lattice equations, and other mathematical models and methods.
The solution to the problem of determining the filtration properties of a digital rock sample model should begin with determining the absolute gas permeability of the model. When it is determined on physical rock samples, inert gas with respect to the medium is filtered, which significantly reduces the effect of chemical interactions of the medium being filtered and the clay content of the rocks. Absolute gas permeability of the created digital rock sample model can be estimated using methods of electrodynamic analogy, finite volumes, and other mathematical models and methods.
Estimation of the phase permeability of the digital model, as well as the permeability of the model for oil, kerosene, water, hydrocarbon gases, and other liquids and gases that are not inert, requires the introduction of correction factors that take into account the chemical interaction of the filtered liquids and gases with the rock. The issue of obtaining these coefficients is complex and integrated, since rocks, even relatively similar in their material composition, can have completely different physical and chemical effects on the value of permeability.
When simulating work processes in the created model, various analytical dependencies can also be used, obtained on the basis of the analysis of the results of geophysical investigations of wells. In [1], it was concluded that the results of geophysical investigations of wells to determine the permeability and porosity properties of terrigenous reservoirs in digital rock sample modeling are of significant interest since they can be effectively used in calibrating a digital rock sample model for porosity, clay content, etc. The authors established the dependence of the oil permeability determined on the rock sample material and on the porosity determined from the results of geophysical investigations (GI) for five wells of fields with terrigenous reservoirs (Fig.2).
In addition to this, other indicators determined during the geophysical investigations of wells are of interest for digital rock sample modeling. In [10],it is indicated that the values of the electrical resistance of rocks determined during the electrometry of wells can be used to determine the permeability of a digital rock sample model by the method of electrodynamic analogy as the electrical resistance of a circuit when replacing a network of micro-channels in a porous medium with a circuit of connected electrical resistors.
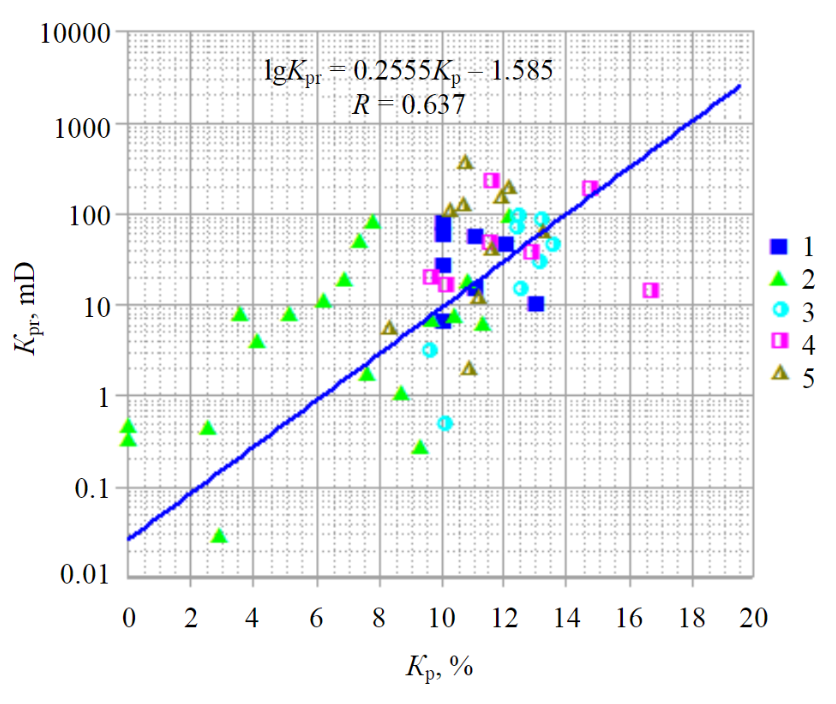
Discussion. The creation, improvement and successful functioning of the technology for determining the permeability and porosity properties of terrigenous reservoirs using methods of mathematical modeling on a digital rock sample model lead to the need for multiscale modeling and finding solutions to a number of problems. The implementation of this technology can also vary.
Due to the high complexity of the numerical processes that take place when conducting research on digital modeling of the pore space and filtration processes occurring in it, it is important to organize the sequential operation of various software packages so that the result of the work of one package is the input data for the work of the next one. At the same time, today there is no clear systematization of the input and output data formats for the operation of such models, which often requires researchers to perform operations for converting data formats using additional software.
Automation of the construction of work streams, when using middleware that enables sequential execution of tasks and the transfer of data from one software package to another, in some cases would significantly reduce the time of computational experiments in digital rock sample modeling.
Conclusions
1. The proposed concept for creating a technology of determining the permeability and porosity properties of terrigenous reservoirs using mathematical modeling methods on a digital rock sample model is based on the use of a large amount of experimental data that can be obtained without using rock sample material. Such data can include the results of geophysical investigations of wells, as well as the results of determining the granulometric composition of rocks, lithologic and petrographic study of thin sections, and other investigations that are obtained when studying the sludge.
2. Determination technology allows adequate assessment of the permeability and porosity properties of terrigenous reservoirs. However, an actual assessment of the efficiency and accuracy of determining the permeability and porosity properties on a digital rock sample model created using certain mathematical models and methods can only be confirmed by comparing the experimental data obtained on physical samples with the results obtained in the course of mathematical modeling.
References