Results of the study of kinetic parameters of spontaneous combustion of coal dust
- 1 — Ph.D. associate professor Saint Petersburg Mining University ▪ Orcid ▪ Elibrary ▪ Scopus ▪ ResearcherID
- 2 — Ph.D. associate professor Saint Petersburg University of State Fire Service of EMERCOM of Russia
- 3 — Ph.D. professor Saint Petersburg University of State Fire Service of EMERCOM of Russia
- 4 — Ph.D. associate professor Saint Petersburg University of State Fire Service of EMERCOM of Russia
Abstract
The article is devoted to the study of the problem of spontaneous combustion of energy grades of coal not only during storage, but also during transportation. As the main samples for the study, the energy grades of SS and Zh coals were selected. The main task of the scientific research was to study the rate of cooling and heating of coal depending on their thermophysical parameters and environmental parameters. To solve this problem, the authors used both the author's installations designed to study the thermophysical parameters of the spontaneous combustion process (the Ya.S.Kiselev method), and the NETZSCH STA 449 F3 Jupiter synchronous thermal analysis device, the NETZSCH Proteus Termal Analysis software package. On the basis of a complex study of the spontaneous combustion process, the authors of the article obtained the kinetic characteristics of the spontaneous heating process (activation energy and pre-exponential multiplier). Nomograms of the permissible size of coal density of different types and shapes of accumulation depending on the ambient temperature are presented, practical recommendations for the prevention (avoidance) of spontaneous combustion of coal fuel are given.
Introduction. Despite the development of nuclear and hydro-wind power engineering, the coal industry continues to provide a significant part of the world energy needs. Russia is one of the leaders in both coal production and export. Due to the fact that most coal basins are located at a significant distance from the consumer, the importance of its transportation methods increases. Currently, the most popular method of coal fuel transportation is international sea transportation.
The main advantage of coals is low cost, while they have a great disadvantage-increased fire and explosion hazard due to their high ability to spontaneous combustion. Therefore, it is possible to ensure safety on a ship engaged in bulk transportation only when taking into account weather conditions, the properties of the ship and the transported cargo [1, 2, 17].
The consequences of a fire at coal terminals due to spontaneous combustion of coal can be catastrophic, including for the environment. In the last decade, as a result of spontaneous combustion, large fires occurred at bulk coal terminals, bulk carriers and thermal power plants: on container ships MSC Katrina (2015) and Ludwigshafen Express (2016); bulk carrier MV Golden Ocean (2018); Khabarovsk CHPP-1 (2015), Severodvinsk CHPP-2 (2017) and Krasnoyarsk CHPP-1 (2019) [4, 9, 21].
This suggests that the problem of spontaneous combustion of coal in the process of storage and transportation is complex and relevant not only for our country. The main problem of fires caused by spontaneous combustion of coal is the lack of knowledge of its physical and chemical (thermophysical) and fire-explosive properties.
Formulation of the problem. Cargo properties are determined by its characteristics and loading during the sail (fire load per unit area). Transportation of dangerous cargo is a special type of transfer that requires an increased level of responsibility from the transport company. Therefore, the coals must be heat-treated and cooled before shipment. Coal transported in bulk is classified as dangerous cargo that is prone to spontaneous combustion [5, 15].
The preparing process for the coal transportation is associated with the large volume dust formation, which is an increased fire hazard. In the fine-dispersed state, combustible dust can remain suspended in the air for a long time and at the same time can form explosive mixtures with it. Dust that has settled on walls, ceilings, and equipment surfaces is a fire hazard [6, 7, 9]. The presence of combustible dust requires special measures to prevent its formation.
The fire and explosion risk assessment of solid combustible substances, in particular coal dust, is determined by a number of fire-technical indicators, of which the main one is lower explosive limit concentration (LELC) of the air suspension [3, 13, 14].
For most solid substances, the dust concentrations corresponding to the LELC are quite high and are usually possible only inside the technology equipment or directly near it [7, 9]. In the hold spaces, as a rule, dust concentrations are much lower. As for the upper explosive limit concentration (UELC), it is so high that its values are not reachable [8, 10, 18].
Coal mining and transportation can also release methane, which is a flammable gas. Since methane is lighter than air, it is concentrated in the upper parts of the cargo hold or in spaces where the requirements for the ventilation systems operation are not met. If there are openings in the enclosing structures, the gas penetrates into other spaces.
Some grades of coals are prone to spontaneous heating, which causes their spontaneous combustion in cargo areas. At a certain concentration of methane with oxygen and the presence of ignition sources (sparks, matches, cigarettes), an explosion can occur.
Studies show that for high-quality explosion protection from the resulting mixtures of coal dust with methane and oxygen, it is necessary to carry out a set of organizational and technical measures to assess the corresponding explosion hazards (LELC, mass, availability of an emergency ventilation system with the required air exchange rate) [5, 9, 21]. However, insufficient attention is paid to fire safety issues during coal transportation.
The literature sources analysis [11, 20, 23] shows the following shortcomings:
• indicators of dust explosion hazard in many countries are different, since coal available in sufficient quantities in this territory is chosen as the base sample (in the United States – it is Pittsburgh coal, in the Russian Federation – hard coal), they have different thermal properties and fire hazard characteristics;
• there are no qualitative analytical procedures for determining the degree of fire and explosion risk of coal dust (evaluation criteria, methods).
As a result, the problem of ensuring safe production conditions associated with using of coal (dust) is relevant. It is necessary to conduct further research to develop the theory of thermal processes during spontaneous heating of coal and its dust, which contribute to the occurrence of spontaneous combustion and explosion, using both high-tech equipment and various author's methods.
Methodology. According to statistical data, the most widely used fuel for CHPP is coals of the grade SS (low-caking coal) and Zh (fat coal), since they have a higher calorific value. However, to date, the characteristics of fire and explosion risk of hard coals of these brands have not been fully studied. Therefore, much attention has long been paid to this issue [10, 12, 16].
Academician N.N.Semenov developed the theory of thermal ignition, according to which heat sources are characterized by the Arrhenius equation, and heat removal – by the Newton formula. Later, there were works that more accurately described the process of spontaneous coal combustion. A number of researchers believe that the prediction of fires during the coal transportation can be carried out by the kinetic characteristics of spontaneous heating [2, 6]: the activation energy E) и the pre-exponential factor C.
For thermal analysis and determination of kinetic parameters, the authors applied the experimental research method based on the method of Yu.S. Kiselev [5, 6], and also used the methods of synchronous thermal analysis implemented using NETZSCH STA 449 F3 Jupiter and the NETZSCH Proteus Termal Analysis software package. Installations for determining kinetic parameters and synchronous thermal analysis are shown in Fig.1.
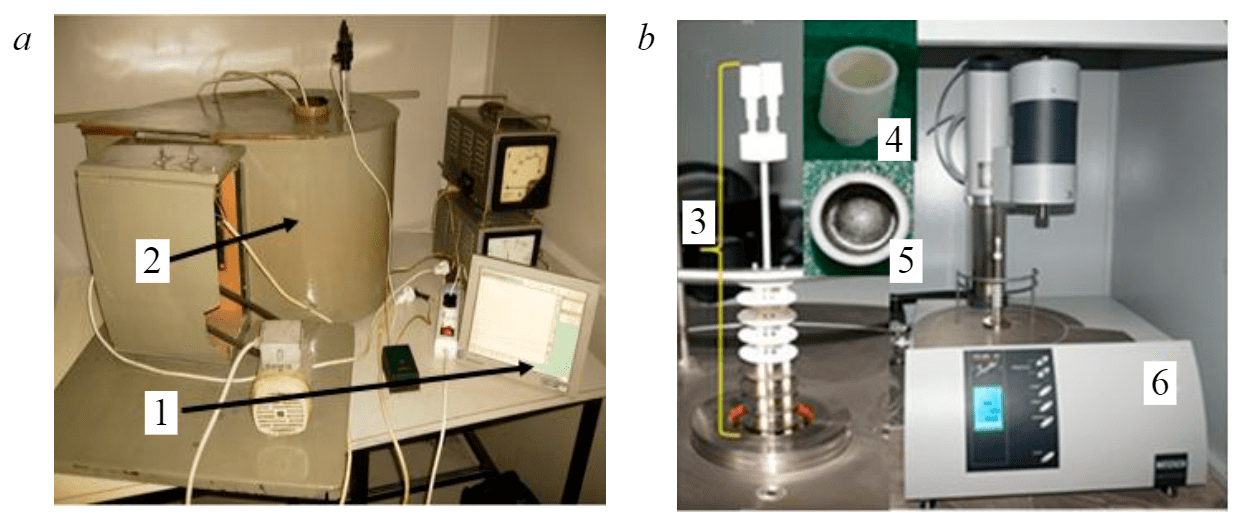
The installation for determining the kinetic parameters of spontaneous combustion (Fig.1, a) allows to record the rate of cooling and heating of the material. The advantage of this installation is that it allows to simultaneously analyze up to 15 samples (depending on the selected size of the sample basket). The sample mass, depending on the set tasks, varies widely – from 1 to 250 g.
For input control (determination of content of volatile agents, ash content, etc.) and studying the processes of thermal degradation of the studied coal samples, the STA 449 F3 Jupiter facility was used (Fig.1, b) [3, 12, 16].
Results. Experimental studies were carried out for coal dust obtained as a result of dispersion of SS grade coal of the Bachatsky coal deposit and Zh grade coal of the Pechora coal basin.
As a result of the input control by standard methods and the method of thermogravimetry, the samples had the technological characteristics shown in the table.
Technological characteristics of coal samples
Grade of coal | Weight fraction of | Content of volatile agents*, % | Specific heat capacity, kJ/(kg∙K) | Specific heat of combustion, MJ/kg | |
moisture Wr, % | ash Ad , % | ||||
Fat coal (Zh) | 10,1 | 8 | 34 | 1,21 | 35,38 |
Low-caking coal (SS) | 8 | 7 | 21,5 | 1,11 | 30,00 |
* Per fuel mass.
For the experiments, baskets (reaction vessels) made of thin steel mesh in the form of cylinders with a diameter equal to the height (d = h): 15; 30; 50 мм. were used: 15; 30; 50 mm. During the experiments, using chromel-copel thermocouples, the temperature was recorded at two points – in the thermostat and in the center of the reaction vessel.
The essence of the method was to determine the parameters of spontaneous combustion of coal by registering the cooling rate Vc and spontaneous heating rate Vsh of the tested samples [9, 21].
At the next stage, cooling thermograms were obtained for various sizes of samples, the most specific ones are shown in Fig.2. The curves were smoothed according to the results of the experiments using the least squares method. The number of measurements and processing of the results corres¬ponded to a confidence probability of 0.95.
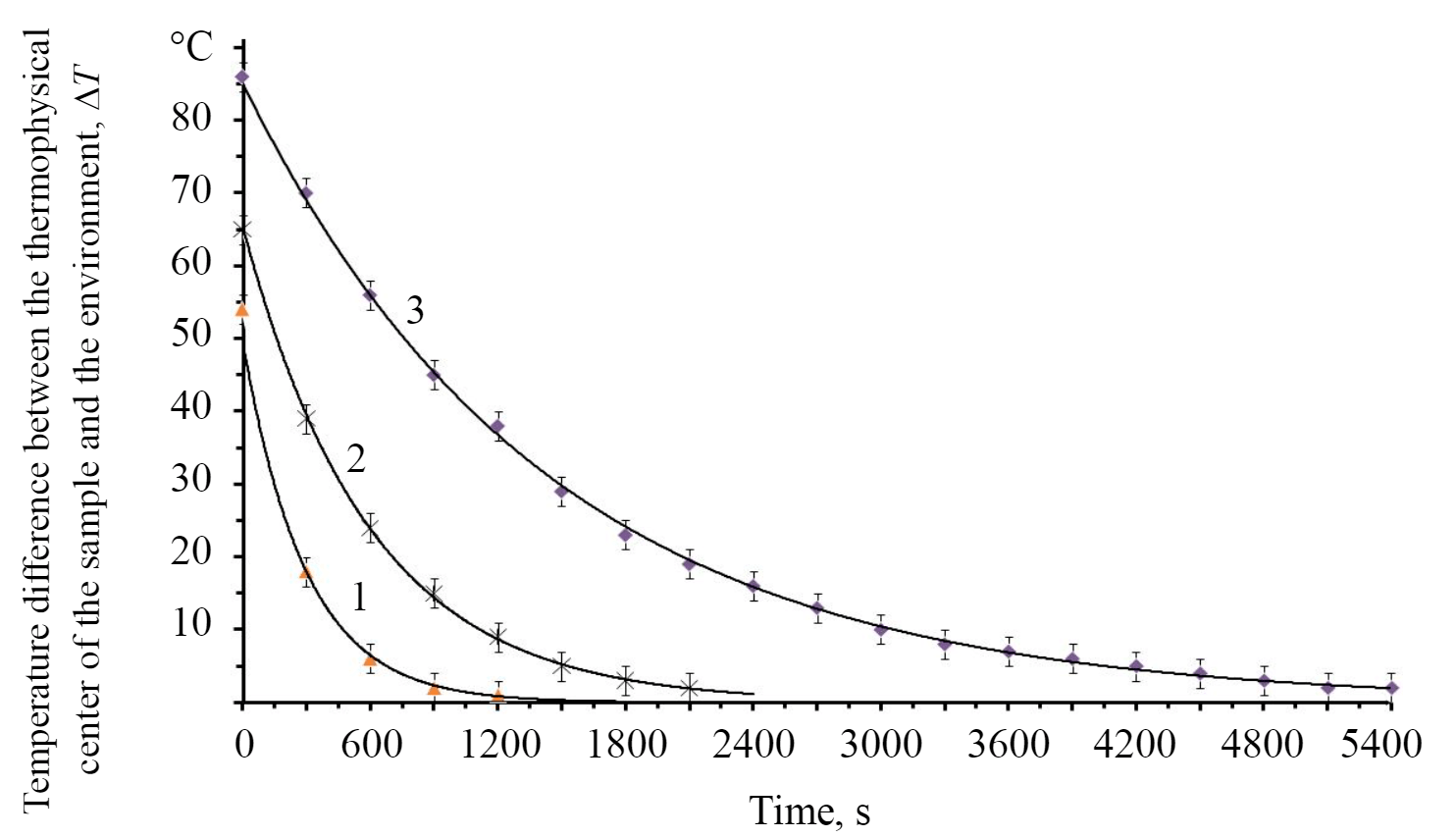
Cooling line in natural logarithmic coordinates (along the ΔТ, axis, for ease of presentation, clarity and further use in analytical expressions) are presented in Fig.3.
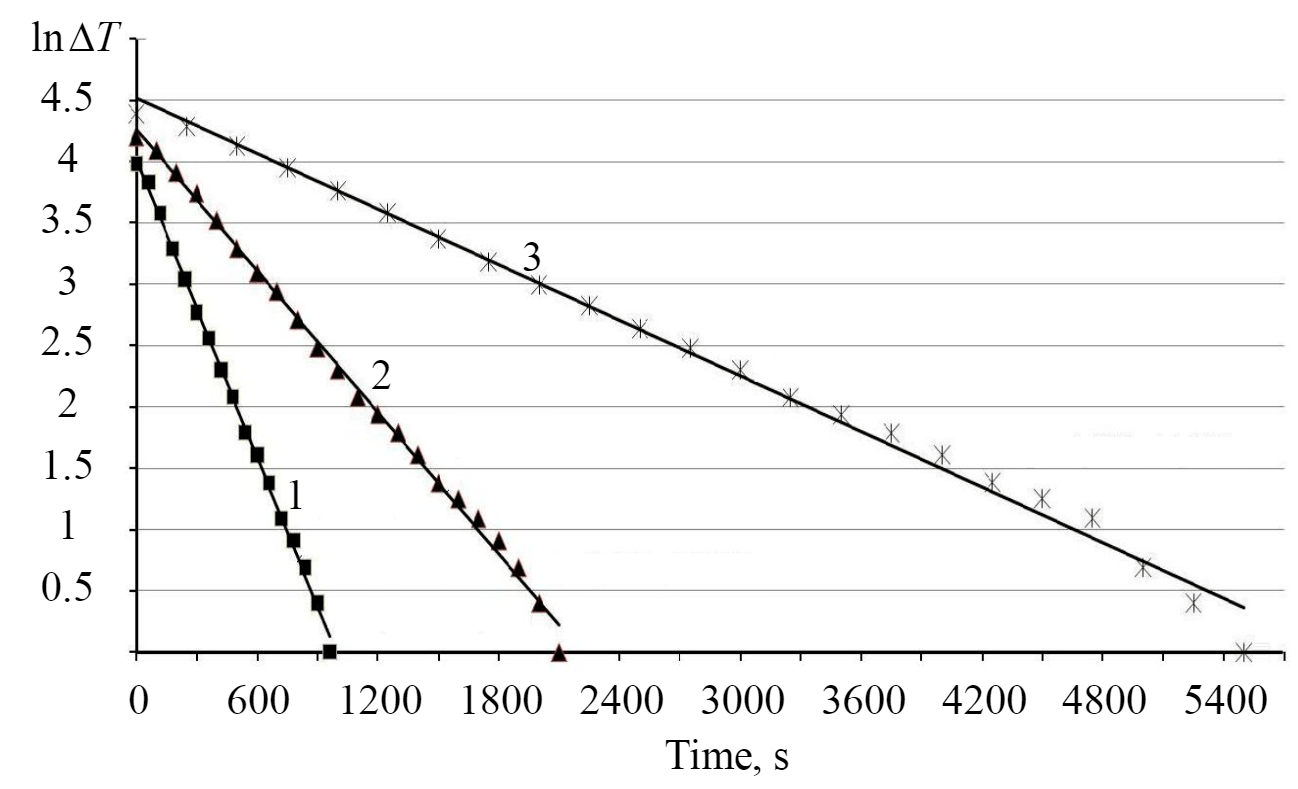
On the vertical axis, the natural logarithm of the temperature difference between the thermophysical center of the sample and the thermostation temperature ΔТ, was plotted, and on the horizontal axis, the time Т.
The results of determining the cooling rate m for hard coal grade Zh are as follows: К-15 = 4,15·10–3; К-30 = = 1,89·10–3; К-50 = 0,79·10–3.
According to experimental values using Arrhenius formulas \(V_{sh}=Ce^{\frac{-E}{RT}}\) (where R – molar gas constant 8.314 J/(mol∙K) and the Newton formula \(V_{c}=m\Delta T\) by approximation \(Ce^{\frac{-E}{RT}}=m\Delta T\) and taking the logarithm of Arrhenius equation
the values of the activation energy Е and the pre-exponential multiplier С were calculated.
If we point out: \(y=\ln V_{sh};\ a_0=\ln C;\ a_1=E/R;\ \chi=1/T,\) the logarithmic Arrhenius equation takes the form:
The solution of this equation makes it possible to determine the kinetic characteristics of spontaneous coals combustion (on the example of the Zh coal grade): E = 65,93 kJ/mol; С = 7,1 K/s. Similar calculations can be made for SS coal grade.
The calculated prediction of thermal spontaneous combustion was carried out for cylindrical, plane-parallel and cubic forms of cargo accumulation.
Using the method developed by Ya.S.Kiselev, a forecast calculation was performed for ambient temperatures in the range of 0-100 0C (with an interval of 10 0C), the shape and critical size of the accumulation according to the criterion of spontaneous coal combustion of the SS (Fig.4, a) and Zh (Fig.4, b) grades from the point of view of its storage and transportation [15].
The results obtained by the authors of the study of the explosion and fire-hazardous properties of coal (including spontaneous combustion) and, above all, the kinetic parameters are in good agreement with the results of other researchers described in [19, 22, 24].
Based on the obtained initial data, engineering and technical calculations, primarily the calculation and forecast of critical conditions of the spontaneous combustion process, clearly showed (Fig.4) that the best form for ensuring fire safety is cubic. With this method of storage, there is a longer induction period before spontaneous combustion and the possibility of storing coal mass in a significant temperature range.
Conclusion. As a result of the carried out experiments and the obtained calculation results, the following conclusions can be drawn.
Based on the obtained results, it is necessary to develop recommendations to reduce the risk of coal fires due to spontaneous combustion processes.
It is established that at an ambient temperature significantly different from the spontaneous combustion temperature (Tsh), the activation energy Е has a decisive effect on the spontaneous coal combustion. The lower the activation energy of coals, the higher the tendency to spontaneous combustion. At the same values of Е, the possibility of spontaneous combustion of coals will be higher if (Tsh) is less. When the ambient temperature approaches to (Tsh) the spontaneous combustion process is determined only by this temperature (Tсам), and not by the Е parameter.
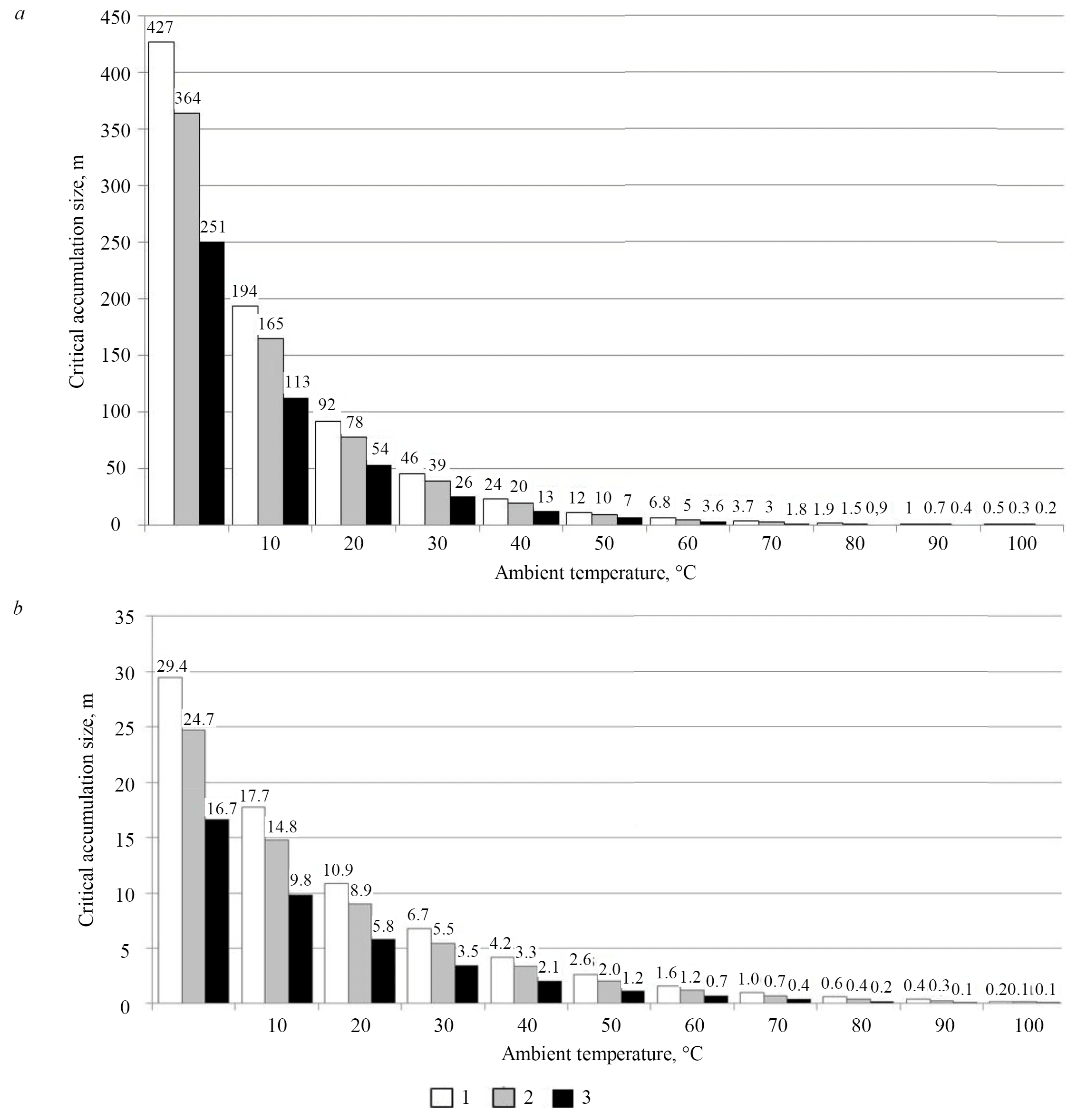
The kinetic parameters determined for the SS and Zh coal grades allow to quantitatively characterize the intensity of the processes of formation and consequent spontaneous combustion of flammable coal dust sediments and, as a result, to develop preventive measures aimed at preventing fires due to spontaneous combustion.
Based on the graphic material, it is concluded that the main characteristic that affects the indicators of coals distribution (warehousing, storage, transportation) is the activation energy of the spontaneous combustion process. The smaller it is, the more fire-prone coal is.
Coals that absorb more oxygen, i.e. having a porous structure, are more prone to spontaneous combustion (Zh grade). Coals of the SS grade are more resistant to spontaneous combustion due to their dense structure.
It is established that the most fire-prone form of coal storage is the cubic form. When storing coal in this way, a larger volume of coal is transported at the same ambient temperature.
Referenses