Results of aeromagnetic survey using unmanned aerial system at the Bunger Hills and Highjump Archipelago, Wilkes Land, East Antarctica
- 1 — Chief Geophysicist Radar mms JSC, Unmanned Aerial and Marine System Division ▪ Orcid
- 2 — Senior Geophysicist Radar mms JSC, Unmanned Aerial and Marine System Division ▪ Orcid
- 3 — Ph.D., Dr.Sci. Deputy General Director VNIIOkeangeologia ▪ Orcid
- 4 — Senior Researcher VNIIOkeangeologia ▪ Orcid
- 5 — Director Radar mms JSC, Unmanned Aerial and Marine System Division ▪ Orcid
- 6 — Researcher VNIIOkeangeologia ▪ Orcid
Abstract
The paper focuses on the technique and results of an aeromagnetic survey conducted using a fixed-wing unmanned aerial system (UAS) in East Antarctica at the Bunger Hills and Highjump Archipelago (Wilkes Land) during the 69th Russian Antarctic Expedition. The above survey was carried out at a 250-meter distance between flight lines (scale 1:25,000) over the area of 600 km 2 to increase the geological knowledge of the area. The magnetic anomaly map obtained after data processing is more detailed than any of known published geological maps of the area. The size of anomalies detected varies from dozens of meters up to large, kilometer-scale structures traced within the entire area under survey. The data analysis shows that the surveyed region is characterized by morphological heterogeneity and amplitude variability of anomalous magnetic field. Along with relatively calm zones one can observe strong gradient ones. Even the fluent analysis of aeromagnetic survey results proves their high information content. The UAS-based survey results demonstrate that the technique implemented is an important tool of applied geophysics and can effectively solve tasks of geological mapping in harsh weather conditions of Antarctica. It can adequately replace conventional aeromagnetic surveys that are now done using manned aircraft.
Introduction
The southern continent, Antarctica, is the least studied region in the world. Despite a number of regular geological and geophysical surveys have been conducted there almost for 70 years, its vast territory, harsh weather conditions and poor density of research stations cause strong obstacles for such explorations.
The aerogeophysical research including aeromagnetic surveys is accomplished in Antarctica from the middle of the 20th century [1, 2]. The magnetic field measurements play a very important role in studying this area and adjacent territories of the World Ocean because it allows us to expand understanding of the geological structure of the region and its place in the global tectonic processes. The anomalies distribution of the Earth’s magnetic field (Ta) ensures obtaining some unique information on tectonomagmatic processes that take place in the Earth’s crust during long periods. It also allows one to detect spatial boundaries of geological formations, sedimentary basins, intrusions, Earth’s crust blocks, tectonic disturbances, etc. In the conditions of Antarctica mostly covered with ice the magnetometry data play a key role in the analysis of Earth’s crust structure and study of its geotectonic history as well as in establishing correlations on poorly studied territories characterized by the lack of geological information [3]. Until recently, such explorations were mainly based on a manned aircraft although some unmanned aerial system (UAS)-based survey attempts were made as well [4]. However, unmanned aerial systems are widely used to research Antarctica in aerial photogrammetry, atmospheric studies etc. [5-7].
Various UAS-based survey techniques are being successfully implemented into geophysical prospecting in the multiple fields and gradually complement or substitute manned aircraft- and ground-based surveys [8-10]. During last two decades of the above techniques being developed, the most notable achievements have been made in the aeromagnetometry [11-13].
A number of geological tasks solved by the middle-scale aeromagnetic surveys frequently do not require precise ground surface draping. Under such circumstances it makes possible to conduct a survey either along some smooth flight line that follows the terrain in some known range of clearances (general draping), or even at fixed altitude. Such approach allows using a fixed-wing UAS [14]. The unmanned aerial vehicles (UAV) equipped with internal combustion engines are characterized by significant time of autonomous flight (up to 12 h and even more) and larger operating range compared to an electric rotary-wing UAS. It can exceed 100 km and make it possible to accomplish 1000-kilometers survey for one flight.
The aeromagnetic survey using the UAS was conducted in the region of the Bunger Hills and Highjump Archipelago (Wilkes Land, East Antarctica) during the work of the 69th Russian Antarctic Expedition (69 RAE) in the season of 2023 to 2024. The survey of 1:25,000 scale was carried out by Radar mms JSC over the area of 600 km2 according to the requirements under the contract with VNIIOkeangeologia as a part of the task given by the Government Authority, the Federal Agency for Mineral Resources (Rosnedra). Since the area under survey is not provided with instrument runways, the geophysical survey using manned aircraft is difficult and inefficient.
The landscape of the area under survey includes both big hills up to 165 m above sea level and valleys partly filled with fresh and salt lakes. Almost 50 % of the area under survey is covered with glaciers and sea gulfs. In such conditions the ground magnetic survey is connected with obvious difficulties, whereas aerial measurements are quite favorable.
It should be noted that before the project was approved, we had some doubts regarding the possibility of such kind of UAS-based survey in severe climate conditions in Antarctica. The area under survey is located close to the Knox Coast and its weather conditions are unstable and characterized by strong gusty wind as well as sudden changes in temperature and atmospheric pressure. Moreover, the boundary between South Ocean, from the one hand, and an ice shield of almost several hundred meters thick, from the other hand, could provoke unpredictable disturbances in the near-surface atmosphere. These features increase crush risks, especially taking into account UAS-based survey peculiarities: low flight altitude, light enough UAV weight and limited power-to-weight ratio. Despite the above natural factors, we made a decision to conduct this survey. Considering the significant dimensions of the area under survey, our choice fell on the fixed-wing UAV as a platform for magnetometry equipment. The results of this aeromagnetic survey using a fixed-wing UAS are represented in the paper.
Survey tasks and general data on the area under survey
The Bunger Hills (Fig.1) is a coastal range in Antarctica situated in the western part of Wilkes Land (Mawson sea, Knox coast) at a distance of 46 km to South from Mill Island and 370 km to East from the Russia’s Station Mirny. During the 1st Soviet Antarctic Expedition, the scientific station Oasis was founded on this territory as the base for the researchers who explored the Bunger Hills and surroundings [15-17]. At the present time, there are three seasonal camps here: Oasis (Russia), Dobrowolski (Poland) and Edgeworth David (Australia).

Fig.1. Overview of the survey area
1 – ice domes; 2 – icecap slopes; 3 – outlet glaciers; 4 – ice shelf; 5 – terrain; 6 – water reservoirs; 7 – survey area
The Bunger Hills continue to be explored until now because it is one of the key regions in East Antarctica that attracts researchers and scientists from different countries [18-20]. From the geology point of view, this area is a part of the Mesoproterozoic orogen and is mostly composed of metamorphosed Archaean and Proterozoic rocks of different origin. The metamorphic strata are crumpled to big folds complemented by minor ones located at flanks.
The area under survey is characterized by relatively poor geophysical knowledge. The exploration of the Earth’s magnetic field of Antarctica started in 1956-1957 near the Mirny station as a part of the 1st Integrated Antarctic Expedition [16, 17]. The aeromagnetic survey was conducted at the vast area including the Bunger Hills at a distance of 1-2 km between survey lines. For a long period, these data poor enough in quality were the only source of geophysical data on the vast region of East Antarctica including Wilkes Land [21].
The next stage of the Denman glacier and Obruchev archipelago explorations is connected with international projects ICECAP-IceBridge and EAGLE* (East Antarctic Grounding Line Experiment) in 2009-2011 and 2015-2016 correspondingly [22-24]. They provided new geophysical data on potential fields and subglacial terrain of vast regions of East Antarctica unexplored before [25, 26]. These studies were also accomplished in the region of the Bunger Hills and Highjump Archipelago, but they insignificantly affected their territory.
Since the 62nd RAE, PMGRE JSC has been conducting ground magnetic surveys in the central part of the Bunger Hills under the contracts with VNIIOkeangelogia to support 1:50,000 scale geological mapping. The ground surveys are performed over mineralization zones, intrusions, and other specific objects for the purpose of explorations along both separate lines and regular observation network at a 250-300 m distance between lines. By 2024, the total line length of ground magnetic survey is about 500 km.
The main objective of the work described was the efficiency proving middle-scale magnetic surveys for geological mapping of Antarctic oases partly covered with moraines, glaciers and lakes. Аt the stage of the UAS-based survey design, we set both the spatial boundary of the area and the survey parameters. The dataset that includes magnetic anomalies maps and digital databases was tasked to prepare basing on the survey results to use it later along with data of previous exploration, for the development of improved geological structure model of the Bunger Hills and Highjump Archipelago.
UAS-based Magnetometry System
The magnetometry system used in the survey described was installed on board the fixed-wing UAS Diam-20, an unmanned aircraft, capable of carrying up to 5 kg, its max take-off weight is 29 kg. This UAV has a canard configuration with its engine and propeller located in the rear part of the fuselage. At the same time, the sensor element included in the cesium-vapor magnetometer DM (Table 1) is mounted on the outrigger in front of the UAS at a maximum distance from the main magnetic parts and electromagnetic noise sources – the engine and the power electronics. Despite such a layout, the UAV anyway produces some magnetic disturbances that have to be compensated to avoid artefacts in the data.
Table 1
Specifications of UAS magnetometers
Specifications |
Model |
|
DM |
MTF-C |
|
Manufacturer |
Radar mms JSC (St.Petersburg) |
Radar mms JSC (St.Petersburg) |
Type |
Optical-pumped Cs |
Fluxgate |
Measured value of Earth’s magnetic field |
Total field |
Three components – х, у, z |
Measurement range, nT |
From 20,000 to 100,000 |
From –100,000 to 100,000 |
Gradient tolerance, nT/m |
20,000 |
> 50,000 |
Operating zone, deg |
±35 |
360 |
Resolution, nT |
0.002 |
1 |
Sensitivity, pT/ |
0.2 |
100 |
Noises |
0.003 nT in band; 0.1-1 Hz |
≤ 1nT; 0.1-1 Hz |
Heading error, nT |
≤0,5 |
– |
Absolute accuracy, nT |
≤1 |
No more ±100 |
Power consumption, W |
12 |
1 |
Operating frequency, Hz |
1000 |
1000 |
Operating temperature, °С |
From –40 to +65 |
From –40 to +65 |
Weight, kg |
1.5 |
0.5 |
The magnetic deviation compensation techniques developed for manned platforms [27-29] can be equally applied for unmanned aerial systems. In our scenario, we used 3-axis fluxgate magnetometer MTF-C (Table 1) as a deviation compensator. It was placed in the cargo compartment inside the fuselage. The configuration scheme and dimensions of the UAS-based magnetometry system used for the survey are represented in Fig.2. The specifications of the unmanned UAV are listed in Table 2. The two identical systems were used during the survey at the Bunger Hills.
It should be noted that the magnetometry system was sufficiently debugged and proved its effectiveness during several field campaigns of 2019-2023 held in northern regions of Russia, including mountain areas. In this period, almost 11,000 km of the survey lines were covered using these UAS systems.
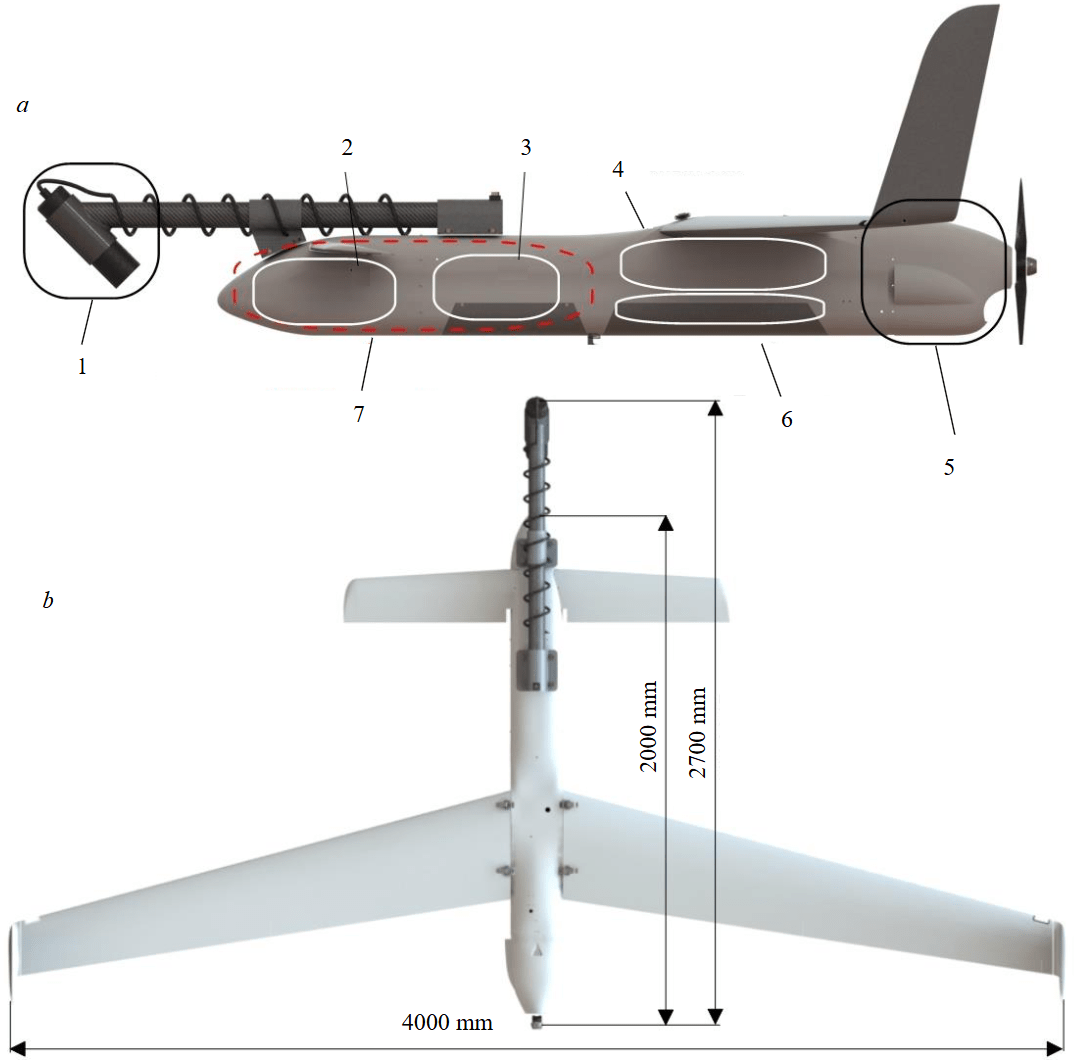
Fig.2. Configuration (a) and dimension (b) scheme of the fixed-wing UAS-based magnetometry system
1 – cesium vapor sensor; 2 – 3-axis fluxgate sensor; 3 – logger; 4 – fuel tank; 5 – engine and electronics; 6 – parachute; 7 – cargo compartment
Table 2
Diam-20 UAS Specifications (DIAM-AERO OJSC, Saint Petersburg, Russia)
Specifications |
Parameter |
Max take-off weight, kg |
To 29 |
Wing span, mm |
4,000 |
Length, mm |
2,000 |
Max payload, kg |
To 5 |
Max speed, m/s (km/h) |
45 (160) |
Motor type |
Internal-combustion engine |
Cruise speed, m/s (km/h) |
22-24 (80-90) |
Travel time, h |
14 |
Flight range, km |
1,000 |
Max flight altitude, m |
4,000 |
Take-off mode |
From catapult |
Landing mode |
On parachute |
Operating wind speed, m/s (for take-off) |
≤15 |
Operating temperature, °C |
From –30 to +40 |
The data flows from both cesium and fluxgate sensors as well as from GNSS receiver (OEM 7700 by NovAtel Inc.) are transferred in binary format to a logger at a frequency of 1000 Hz. The logger has Wi-Fi and Ethernet interfaces which provide the on-line control of the system before take-off and data storage after landing. The data from magnetometers are also transferred via radio to the ground control station at a decreased rate (2 Hz) enabling the operators to estimate data quality and system performance during the flight.
Survey and preliminary data processing techniques
The survey team including one geophysicist and one UAS pilot as well as all necessary equip-ment reached East Antarctica at the end of December in 2023 on board the Russian research vessel Akademik Fedorov. From 29 December, 2023 to 2 January, 2024 the personnel were preparing for aeromagnetic survey, testing the UAS, equipping the take-off area and installing the magnetic base station. Since the UAS uses mechanical catapult for take-off, there is no need to prepare a runway. The selected take-off area is a flat enough location approximately 50 ´ 50 m in size with the catapult installed in the middle. The area used was surrounded by rocky hills about 30 m in height at a distance of 200-300 m from the take-off point. The catapult azimuth was set before each flight according to the actual wind direction.
Diurnals recording. The MVS-DM type magnetic base station (Radar mms JSC) was equipped with cesium sensor identical to one installed on board the UAS (see Table 1). After the reconnaissance operations, it was installed at a distance of 300 m from the ground control center at the coast of lake Figurnoye where the magnetic gradient was low. The base station includes the GNSS module identical to one the UAS is equipped with. The navigation corrections were applied to the UAV data in post-processing kinematic (PPK) mode. The base station location (–66.2753°S; 100.7444°E; WGS 84) did not change during the entire survey.
The average daily amplitude of diurnals was about 200 nT that, of course, is a significant value, although in some days it was even higher. At the same time, the magnetic activity index (Kp-index) never exceeded 4 units during the survey period, that corresponds to weak disturbance level.
Survey technique. The UAS-based survey was carried out from 3 to 11 January, 2024 with 3-day skip caused by bad weather conditions. The flights were done along 112 regular survey lines (RL) at a 250-meter interline distance. The line length varied from 20,000 to 25,000 m, the line azimuth was 90° and 270°.
For leveling purposes, the measurements were also performed across RL down to 31 tie lines (TL) with 2,000-29,000 m. The distance between them was 1,250 m, the azimuth was 0° and 180°. Moreover, three diagonal special lines (SL) were made across all RL to estimate the error of the final magnetic anomaly map. Their length varied from 20,000 to 47,000 m. The aeromagnetic survey design developed for the Bunger Hills and Highjump Archipelago is represented in Fig.3, a.
This survey included ten 4-hour flight missions (Fig.3, b). Along with lines they included all necessary maneuvers of the UAS and its routes from take-off site to waypoints and back. The flight missions were downloaded to the UAV during pre-flight procedures. As being launched, the UAS flew completely in autonomous mode and there was no need for constant connection with the ground control center. The UAV specifications allow conducting longer flights but we decided to make missions short enough to decrease take-off weight due to the fuel amount and increase the UAS mobility.

Fig.4. Draping example of the fixed-wing UAS (survey line-54 flight dated 8 January, 2024)
1 – elevation; 2 – real path; 3 – ideal path

Fig.5. Path of the compensation flight (a) and UAS magnetic deviation diagram (b); flight dated 11 January, 2024
The survey was conducted with general draping at a 130-meter clearance. The draping parameters were planned taking into account technical requirements of the survey, terrain slopes and UAV specifications with air speed of 24 m/s and vertical speed of 1.5 m/s. The terrain draping was based on the digital elevation model (DEM) of the Bunger Hills and Highjump Archipelago that was developed by VNIIOkeangeologia using ESA SPOT 5 HRS satellite 5-meter grid. The draping example is represented in Fig.4.
The common survey was carried out for 3,343 km including all types of measurement lines (RL, TL, SL, control lines etc.) at the area of 601 km2. The regular and tie lines common length was 2,948 km. The flights were accomplished completely in autonomous mode being monitored in safety reasons from the ground control center via both radio and GNSS-tracker.
During the period of 3 to 11 January, 2024, 13 flights were conducted at the area of the Bunger Hills with the total flight time of 48 h 11 min.
Deviation compensation. As has been mentioned above, the UAS-based magnetometry system includes deviation compensation unit based on a 3-axis fluxgate instrument. To obtain compensation factors, we carried out special deviation flights for each system used in the survey. The flight line includes two orthogonal 4-kilometer passes with three radial maneuvers: two – at the ends and one – in the middle (Fig.5, a) where the UAS aircraft changes its direction. The directions of straight lines coincide with TL and RL ones, coursed 0° and 90° correspondingly. The flight was made both in direct and opposite directions. The flight altitude was 1,500 m to minimize the influence of the local anomalies of Earth’s magnetic field.
The data obtained by both unmanned aircrafts in the deviation flights were used for calculation of compensation factors arrays, which consider the disturbances caused by permanent, vortex and induction magnetic interferences of the UAS [28, 30]. The course deviation of both UAS did not exceed 3.5 nT (Fig.5, b), and uncompensated field was less than 0.2 nT. The last value is comparable with heading error of cesium sensor itself.
Preliminary data processing. After each flight, the data file containing records of all blocks of magnetometry system, was copied to a personal computer equipped with specialized PostFlyProcessor (PFP) software developed by Radar mms JSC. This software allows the operator to estimate the quality of collected data such as interruptions in records, sensor noises and stability, flight mission errors etc. It also provides the compensation of magnetic deviation. Further data processing using PFP software provides the division of the entire record at separate survey lines according to the flight plan and synchronize survey data with the magnetic base station ones.
After this stage was over, the processed survey data were saved in the ASCII text file and could be imported by various advanced processing software (such as Oasis Montaj). The data were finally processed using the standard approach and included diurnal and normal field (IGRF) corrections, as well as statistical levelling using tie lines.
The reference level for diurnals correction was set as an average intensity of Earth’s magnetic field measured at the base station during the survey period from 3 to 11 January, 2024 (61,658 nT). After the processing was completed, the 1:25,000 magnetic anomalies map was constructed. This map is used now for the improvement of the Bunger Hills geological structure model.
Data quality estimation
The first quality control stage represents the estimation of the UAS flight path and altitude deviations from the ideal ones stated by the survey requirements. The analysis of the UAS navigation data showed the RMS of altitude deviation was 7.3 m (6 %) with the average terrain clearance of 131.5 m.
The technical survey requirements allowed ±25 m flight path deviation, i.e. 10 % from interline distance (250 m). The conducted quality control estimation showed no critical deviations from the ideal path. The average deviation of both UAS used in the survey was about ±6 m. The maximum deviation did not exceed ±20 m when the flights were performed in windy days.
Fourth difference. The own noises of the magnetometry system were estimated using fourth differences filter* [13, 14]. The analysis showed that a number of its values exceeding ±1.6 nT limit is less than 3 % from total count, whereas the survey requirements allow 10 %. The standard deviation of forth difference within ±1.6 nT was 0.56 nT, that is within the normal range.
It should be noted that the proved noise level of DM cesium sensor is 0.003 nT in the band of 0.1-1 Hz. According to the experience of previous field campaigns after it was installed on the UAS, the fourth difference RMS was 0.015 nT. For safety reasons, at the stage of survey preparation, some servomotors of both UAVs have been changed to more powerful ones, to increase the reliability of systems as flying in severe conditions of Antarctica. The magnetic noises of these new UAS components increased the fourth difference value to 0.5 nT. Based on the experience obtained at the Bunger Hills, we will improve the UAS construction to go back to the standard noise parameters of the system (0.015 nT).
Control line. According to the survey requirements, both at the beginning and at the end of each flight, the UAS carried out the flight on control route in opposite directions along the same line. This procedure was mainly aimed at the estimation of magnetometer zero-level daily offset but also it can be used for the quality estimation of the applied base station corrections. This control route located a few kilometers north of the take-off point is marked orange (Fig.3, a). The control route of 3,600 m long was carried out 22 times during the survey campaign (11 times in each direction). The anomalous field recorded during check flights is shown in Fig.6, a. The quality control of data recorded at direct and opposite one-day flights was performed at measurement points located within 10-meter range one from another – is approximately 1200 points per line. The standard deviation d was calculated by Bessel formula:
In general, there was a slight difference between one-day check flights. The exception was the flight conducted on 3 January, 2024, it was carried out in a strong wind conditions when wind speed reached 28 m/s, so the RMS value obtained in the control line was 9.93 nT. The average RMS of all control lines data was 5.32 nT, while for the ones covered only on the low wind days it was 4.8 nT.
If, to calculate the survey error, we take only a kilometer-long section of the control route in a zone with a relatively low (100 nT/km) horizontal gradient of the anomalous magnetic field (Fig.6, a), then the standard deviation for this sample will be 4.03 nT.
Survey line repetition. In accordance with the methodology of the work, repeated measurements were carried out on eight survey lines, 25 km each. In total, the flight length was 160 km that corresponds to 5.75 % of total RL distance.
The standard and repeated flights were conducted on different days (3 and 7 January, 2024) using two different unmanned aerial magnetometry systems with the same flight mission. The data example along one of the lines (RL-4) is shown in Fig.6, b. Despite the magnetic anomaly field has high gradients, the standard deviation of these flights is 4.87 nT.
Statistical levelling and map error estimation. The final error estimation of the magnetic anomaly map obtained was conducted using data at intersections of RL and SL. Both survey lines and diagonal ones have been statistically levelled before using same tie lines. These two data sets were leveled separately one from another using the same technique.
Initially, it was planned to select low-gradient (50 nT/km) zones where RL and SL intersections need to be chosen for map error estimation. However, taking into account that such zones are almost absent (only 10 % of intersections satisfy this condition), we decided to increase the appropriate gradient limit to 40 nT per 100 m which corresponds to 1 nT per record fiducial at 10 Hz frequency at a survey speed of 24 m/s.

Fig.6. The Ta values recorded on the control line for entire period of field work (a) and results of repeated measurements on one of the survey lines (RL = 54) (b)
Using such approach, 106 intersections between regular and diagonal lines were selected, what corresponds to 40 % from their total number (255 intersections). The obtained standard deviation, i.e. magnetic anomaly map error, calculated by formula is 7.3 nT. From the one hand, such a value is higher than an expected limit, 5 nT, which is standard for this type of surveys, from the other hand, it should be pointed that the obtained error is calculated for 40 % of intersections in zones where horizontal magnetic anomaly field gradients reach hundreds of nT, and calm field zones within 50 nT/1000 m gradient range are almost not present. Thus, the standard map error estimation using diagonal lines can not be an appropriate procedure for this survey. Nevertheless, the standard deviation value obtained is adequate for the purpose of geophysical mapping especially taking into account differentiated high amplitude magnetic anomalies of the Bunger Hills.
In our opinion, the main source of increased levelling error is high values of both horizontal and vertical gradients of the Earth’s magnetic field that produce disturbances even with minimal (first meters) intersection altitude difference between survey and diagonal lines.
Survey results and discussion
The magnetic anomaly map has been constructed based on the results of survey carried out at the Bunger Hills and Highjump Archipelago. Its analysis shows the DT has a behavior typical for strong metamorphized complexes of old orogens, such as morphological heterogeneity and amplitude variance. Alongside with relatively calm zones, the survey area contains differentiated ones. The magnetic field anomalies detected, and, correspondingly, the spatial distribution of sources of magnetic activity are, in general, more detailed than any of published geological maps [15, 31, 32]. The scale of contrast anomalies detected varies from local ones with the size of some dozen meters to regional structures traced through the entire area under survey (Fig.7, a).
The complete geological interpretation of the aeromagnetic survey results described is not a point of discussion in this paper but even a cursory analysis of magnetic anomaly map shows high informative content compared to available archive data on geological structure of the Bunger Hills and Highjump Archipelago (Fig.7, b).
An illustrative example is the appearance in the magnetic field of large intrusions (batholiths) of gabbro and monzogabbro composition in the Paz Cove Bay area of the Bunger Hills (Fig.7). Its marginal part is mapped by a high-amplitude anomaly in the southern half and can be traced as a relative maximum in the northern one, hidden under the glacier, forming an oval structure. The anomalous effect is associated with near-contact changes in the intrusion itself and in the host rocks. These changes occurred during the introduction of igneous rocks. The bulk of the batholith is mostly weakly magnetic and is characterized by local magnetic anomalies, including oval-shaped ones.
The previous geological mapping of the south-eastern part of the Bunger Hills revealed two rock units. One of them is represented by tonalitic-trondiemite-granodiorite gneisses of the Archaean block, whereas the second unit is formed by undivided layers of amphibolite-biotite pyroxene gneisses and crystal schists of Mesoproterozoic orogen (Fig.7, b), At the same time, there is no difference in the magnetic field of these two units (Fig.7, a). It means that the geological map has to be updated. Most likely, the entire zone is occupied by aged craton of Archaeanrocks which, probably, were partly metamorphized in Mesoproterozoic, as suggested by recent studies [33, 34].
Also noteworthy is the contrasting magnetic field in the northwestern part of the area under survey (Fig.7, a), where the map shows the development of an undifferentiated stratum of garnet-sillimanite-cordierite and garnet-biotite gneisses (Fig.7, b). These anomalies are the obvious evidence of geological structure heterogeneity of the above zone that could be caused by possible availability of other types of rocks that produce positive magnetic anomaly of NE-SW strike.
Due to the high accuracy of aeromagnetic observations, it is possible to trace even small dolerite dikes of Mesoproterozoic age. This is best seen in that survey lines where linearly correlated anomalies are observed, the amplitude of which reaches 100 nT at the height of the survey, but the amplitude of the most thick (up to 20-30 m) and extended dike, located in the central part of the Banger Hills, is weaker. It appears on the map as a slight but visible linear trend (Fig.7, a).
Conclusions
The comprehensive middle-scale UAS-based aeromagnetic survey was carried out in 2023-2024 for the first time ever in Antarctica. The fixed-wing unmanned aerial magnetometry system allowed conducting the survey along 2940 km over the area of 601 km2 in a very short period of time.

Fig.7. 1: 25,000 scale magnetic anomaly map of the Bunger Hills and Highjump Archipelago obtained by UAS-based magnetometry survey during the 69th RAE (a) and simplified 1: 200,000 scale geological map of the region (b), by [35] with corrected age of magmatic and metamorphic rocks according to [32]
Based on the survey results the magnetic anomaly map of 1:25,000 scale has been constructed. The map clearly demonstrates both morphological and amplitude variety of the detected anomalies, where not only their directions but also shapes of separate objects vary greatly. The length of detected anomalies can reach dozens of kilometers and their thickness varies from dozen meters to several kilometers. A full set of amplitude, morphological and structure characteristics of magnetic anomaly field clearly reveals complicated geological structure of the studied area, where Neoarchean rocks verge on both Mesoproterozoic ones and the plenty of intrusions of different composition from felsic to mafic [15, 36, 37].
The survey results provided an increase in the geological and geophysical study of the area under survey, especially those parts of it that are hidden under glaciers or water. It has been confirmed that unmanned aeromagnetic survey is an important tool for applied geophysics, which makes it possible to effectively solve the problems of geological mapping on a scale of 1:25,000 and smaller in severe Antarctic conditions. It can effectively replace similar operations traditionally performed using manned aircraft, especially at stations that are not equipped with aircraft bases. Additional advantages of unmanned aerial survey are its relative cost-effectiveness compared to manned aircraft, and the safety of an external crew performing remote UAS control.
The experience of the operations described in the paper will make it possible to improve both the unmanned magnetometry systems themselves and the methodology for conducting surveys in Antarctica and other regions.
References
- Frémand A.C., Bodart J.A., Jordan T.A. et al. British Antarctic Survey’s aerogeophysical data: releasing 25 years of airborne gravity, magnetic, and radar datasets over Antarctica. Earth System Science Data. 2022. Vol. 14. Iss. 7, p. 3379-3410. DOI: 10.5194/essd-14-3379-2022
- Ferraccioli F., Armadillo E., Jordan T. et al. Aeromagnetic exploration over the East Antarctic Ice Sheet: A new view of the Wilkes Subglacial Basin. Tectonophysics. 2009. Vol. 478. Iss. 1-2, p. 62-77. DOI: 10.1016/j.tecto.2009.03.013
- Golynskii D.A., Mandrikov V.S., Egorov M.S. Results of ground magnetic survey of 64 RAE. Rossiiskie polyarnye issledovaniya. 2019. N 3, p. 22-25 (in Russian).
- Funaki M., Higashino S.-I., Sakanaka S. et al. Small unmanned aerial vehicles for aeromagnetic surveys and their flights in the South Shetland Islands, Antarctica. Polar Science. 2014. Vol. 8. Iss. 4, p. 342-356. DOI: 10.1016/j.polar.2014.07.001
- Gaffey C., Bhardwaj A. Applications of Unmanned Aerial Vehicles in Cryosphere: Latest Advances and Prospects. Remote Sensing. 2020. Vol. 12. Iss. 6. N 948. DOI: 10.3390/rs12060948
- Grigoreva S.D., Kuznetsova M.R., Kinyabaeva E.R. The experience of application of unmanned aerial vehicles in research-to-practice investigations of 63-66 RAE in Antarctica. Rossiiskie polyarnye issledovaniya. 2021. N 1, p. 31-33 (in Russian).
- Pina P., Vieira G. UAVs for Science in Antarctica. Remote Sensing. 2022. Vol. 14. Iss. 7. N 1610. DOI: 10.3390/rs14071610
- Watts A.C., Ambrosia V.G., Hinkley E.A. Unmanned Aircraft Systems in Remote Sensing and Scientific Research: Classification and Considerations of Use. Remote Sensing. 2012. Vol. 4. Iss. 6, p. 1671-1692. DOI: 10.3390/rs4061671
- Huang Yao, Rongjun Qin, Xiaoyu Chen. Unmanned Aerial Vehicle for Remote Sensing Applications – A Review. Remote Sensing. 2019. Vol. 11. Iss. 12. N 1443. DOI: 10.3390/rs11121443
- Cunningham M., Samson C., Wood A., Cook I. Aeromagnetic Surveying with a Rotary-Wing Unmanned Aircraft System: A Case Study from a Zinc Deposit in Nash Creek, New Brunswick, Canada. Pure and Applied Geophysics. 2018. Vol. 175. Iss. 9, p. 3145-3158. DOI: 10.1007/s00024-017-1736-2
- Yaoxin Zheng, Shiyan Li, Kang Xing, Xiaojuan Zhang. Unmanned Aerial Vehicles for Magnetic Surveys: A Review on Platform Selection and Interference Suppression. Drones. 2021. Vol. 5. Iss. 3. N 93. DOI: 10.3390/drones5030093
- Walter C., Braun A., Fotopoulos G.High-resolution unmanned aerial vehicle aeromagnetic surveys for mineral exploration targets. Geophysical Prospecting. 2020. Vol. 68. Iss. 1, p. 334-349. DOI: 10.1111/1365-2478.12914
- Døssing A., Lima Simoes da Silva E., Martelet G. et al. A High-Speed, Light-Weight Scalar Magnetometer Bird for km Scale UAV Magnetic Surveying: On Sensor Choice, Bird Design, and Quality of Output Data. Remote Sensing. 2021. Vol. 13. Iss. 4. N 649. DOI: 10.3390/rs13040649
- Astafev P.F., Konoplin A.D. Estimation of self noise of geomagnetic and geoelectric field sensors using multichannel measurement method. Izmeritelnaya Tekhnika. N 9, p. 60-66 (in Russian). DOI: 10.32446/0368-1025it.2020-9-60-66
- Ravich M.G., Klimov L.V., Solovev D.S. Precambrian of East Antarctica. Moscow: Nedra, 1965, p. 470 (in Russian).
- Glebovskii Yu.S. Key results of small-scale aeromagnetic survey fulfilled south to Shakleton ice shelf. Byulleten Sovetskoi antarkticheskoi ehkspeditsii, 1959. N 12, p. 37-40 (in Russian).
- Glebovskii Yu.S. Geological structure of the region of Mirny observatory. Byulleten Sovetskoi antarkticheskoi ehkspeditsii, 1959. N 8, p. 21-23 (in Russian).
- Berg S., Melles M., Gore D.B. et al. Postglacial evolution of marine and lacustrine water bodies in Bunger Hills. Antarctic Science. 2020. Vol. 32. Special Issue 2, p. 107-129. DOI: 10.1017/S0954102019000476
- Gore D.B., Leishman M.R. Salt, sediments and weathering environments in Bunger Hills. Antarctic Science. 2020. Vol. 32. Special Issue 2, p. 138-152. DOI: 10.1017/S0954102020000073
- Abdrakhmanov I.A., Gulbin Y.L., Skublov S.G., Galankina O.L. Mineralogical Constraints on the Pressure-Temperature Evolution of Granulites in the Bunger Hills, East Antarctica. Minerals. 2024. Vol. 14. Iss. 5. N 488. DOI: 10.3390/min14050488
- Golynsky A.V., Golynsky D.A., Kiselev A.V., Masolov V.N. Russian geomagnetic investigations in Antarctica. Problems of Geography. Vol. 150, p. 175-197 (in Russian).
- Aitken A.R.A., Betts P.G., Young D.A. et al. The Australo-Antarctic Columbia to Gondwana transitio. Gondwana Research. 2016. Vol. 29. Iss. 1, p. 136-152. DOI: 10.1016/j.gr.2014.10.019
- Aitken A.R.A., Roberts J.L., van Ommen T.D. et al. Repeated large-scale retreat and advance of Totten Glacier indicated by inland bed erosion. Nature. 2016. Vol. 533. N 7603, p. 385-389. DOI: 10.1038/nature17447
- Aitken A.R.A., Young D.A., Ferraccioli F. et al. The subglacial geology of Wilkes Land, East Antarctica. Geophysical Research Letters. 2014. Vol. 41. Iss. 7, p. 2390-2400. DOI: 10.1002/2014GL059405
- Wei Wei, Donald D. Blankenship, Jamin S. Greenbaum et al. Getz Ice Shelf melt enhanced by freshwater discharge from beneath the West Antarctic Ice Sheet. The Cryosphere. 2020. Vol. 14. Iss. 4, p. 1399-1408. DOI: 10.5194/tc-14-1399-2020
- Liu-Schiaffini M., Ng G., Grima C., Young D. Ice Thickness From Deep Learning and Conditional Random Fields: Application to Ice-Penetrating Radar Data With Radiometric Validation. IEEE Transactions on Geoscience and Remote Sensing. 2022. Vol. 60. N 5119014. DOI: 10.1109/TGRS.2022.3214147
- Tuck L.E., Samson C., Laliberté J., Cunningham M. Magnetic interference mapping of four types of unmanned aircraft systems intended for aeromagnetic surveying. Geoscientific Instrumentation, Methods and Data Systems. 2021. Vol. 10. Iss. 1, 101-112. DOI: 10.5194/gi-10-101-2021
- Karshakov E.V., Tkhorenko M.Yu., Pavlov B.V. Aeromagnetic Gradiometry and Its Application to Navigation. Automation and Remote Control. Vol. 79. N 5, p. 897-910. DOI 10.1134/S0005117918050107
- Groom R.W., Ruizhong Jia, Bob Lo. Magnetic Compensation of Magnetic Noises Related to Aircraft’s Maneuvers in Airborne Survey. 17th EEGS Symposium on the Application of Geophysics to Engineering and Environmental Problems, 22-26 February 2004, Colorado Springs, CO, USA. European Association of Geoscientists & Engineers, 2004. N cp-186-00008. DOI: 10.3997/2214-4609-pdb.186.AIR02
- Semevskii R.B., Averkiev V.V., Yarotskii V.A. Special magnetometry. St.Petersburg: Nauka, 2002, p. 227 (in Russian).
- Abdrakhmanov I.A. Physicochemical simulation of the conditions for the mafic granulite formation (Bunger Hills, East Antarctica). Vestnik of Geosciences. N 2 (314), p. 14-18 (in Russian). DOI: 10.19110/geov.2021.2.3
- Tucker N.M., Hand M., Clark C. The Bunger Hills: 60 years of geological and geophysical research. Antarctic Science. 2020. Vol. 32. Special Issue 2, p. 85-106. DOI: 10.1017/S0954102019000403
- Leitchenkov G.L., Grikurov G.E. The Tectonic Structure of the Antarctic. Geotectonics. 2023. Vol. 57. Suppl. 1, S28-S33. DOI: 10.1134/S0016852123070087
- Cox S.C., Lyttle B.S., Elkind S. et al. A continent-wide detailed geological map dataset of Antarctica. Scientific Data. 2023. Vol. 10. N 250. DOI: 10.1038/s41597-023-02152-9
- Ravich M.G., Solovev D.S. Geological map of the Bunger Hills of 1: 100 000 scale. Atlas Antarktiki. 1966. Vol. 1. Moscow: Glavnoe upravlenie geodezii i kartografii; Leningrad: Gidrometeorologicheskoe izd-vo, p. 166-167 (in Russian).
- Sheraton J.W., Tingey R.J., Oliver R.L., Black L.P. Geology of the Bunger Hills-Denman Glacier region, East Antarctica. Canberra: Australian Government Publishing Service, 1995. Bulletin 244, p. 124.
- Tucker N.M., Payne J.L., Clark C. et al. Proterozoic reworking of Archean (Yilgarn) basement in the Bunger Hills, East Antarctica. Precambrian Research. 2017. Vol. 298, p. 16-38. DOI: 10.1016/j.precamres.2017.05.013
* Roberts J.L., Blankenship D.D., Greenbaum J.S. et al. EAGLE/ICECAP II – geophysical observations (surface and bed elevations, ice thickness, gravity disturbance and magnetic anomalies. Australian Antarctic Data Centre, 2023. Ver. 2. DOI: 10.26179/5xcc-4836
**Aslamov Yu.V., Babayants P.S., Glinsky N.A. et al. Methodology recommendations for the multi-method aero geophysical surveying. St.Petersburg: Kartograficheskaya fabrika VSEGEI, 2021, p. 50 (in Russian).