Black shales – an unconventional source of noble metals and rhenium
- 1 — Ph.D., Dr.Sci. Professor Saint Petersburg State University ▪ Orcid
- 2 — Ph.D. Leading Expert All-Russian Geological Research Institute of A.P.Karpinsky ▪ Orcid
- 3 — Ph.D. Leading Engineer All-Russian Geological Research Institute of A.P.Karpinsky ▪ Orcid
- 4 — Ph.D. Head of Central Laboratory All-Russian Geological Research Institute of A.P.Karpinsky ▪ Orcid
- 5 — Ph.D. Associate Professor The Herzen State Pedagogical University of Russia ▪ Orcid
Abstract
The content of noble metals and rhenium in the Lower Paleozoic black shales of the eastern Baltic paleobasin in Russia was estimated. Shales are enriched in platinoids (PGM to 0.12 g/t) and rhenium (to 1.54 g/t). The main accessory elements of noble metals and Re are U, V, Mo, Cu, and Ni. Black shales consist of organic (9-26 rel.% ), clay (40-60 rel.% ), and silt-sandy (25-50 rel.% ) components and a nodule complex (2-5 rel.% ) (carbonate, sulfide, phosphate and silicate nodules). Noble metals occur sporadically in the silt-sandy admixture as native forms and intermetallics: Au nat , Au-Ag, Au-Cu, Au-(Cu)-Hg, Au-Hg, Ag-Cu, Pt-Fe. Micromineral phases of noble metals were found in diagenetic sulfides: Au nat , chalcopyrite with Au admixture, pyrite with platinoid admixture. Clay fraction is 10-fold enriched in noble metals as compared to shale – to 0.28 g/t Au, 0.55 g/t Pt, 1.05 g/t Pd, and 1.56 g/t Re. Organic matter sorbs noble metals to a limited extent but accumulates rhenium. Pore space of black shales contains a colloidal salt component (submicron fraction) which is represented by particles smaller than 1,000 nm. The share of submicron fraction in black shales is 0.1-6 wt.%. The submicron fraction contains on average: PGM – 14 g/t, Au – 0.85 g/t, and Re – 4.62 g/t. The geochemical resource of noble metals (Au, Pt, Pd), Re and the accessory elements (U, V, Mo, Cu, and Ni) for black shales as a whole and their submicron fraction was estimated. Black shales are recommended as an integrated source of raw materials.
Introduction
A keen interest in noble metals (NM) and rhenium is associated with the expansion of the scope of use as well as their status as strategic raw materials. In Russia, Au, platinoids and Re are included in the list of the main types of strategic mineral raw materials along with oil, natural gas, uranium, diamonds, tungsten and other elements. Rhenium deserves special attention, the combination of its chemical and physical properties determines a significant interest to its use in engineering industries that require wear, heat and acid resistance. Blades for gas turbine engines, rocket and aircraft nozzles are manufactured from rhenium-containing alloys. A minor 1.5-2.5 % addition of Re to high-quality alloys of refractory metals W, Ta, Mo significantly improves the heat resistance and strength of alloys. The main raw material sources of rhenium are copper-molybdenum-porphyry deposits, stratiform (copper sandstones and slates), carbonaceous bituminous shales of different ages and epigenetic uranium deposits associated with zones of formation oxidation. There are publications on the occurrence of rhenium in oils and heavy bitumens, in modern volcanic fumaroles. Main suppliers of primary rhenium to the world market are Chile, Kazakhstan, the USA, Peru, Germany, Poland, Canada, Mexico, China, and Uzbekistan. A distinctive feature of Re consumption in Russia is its almost complete dependence on the imported raw materials.
Due to depletion of rich NM ores and a limited number of explorable rhenium deposits the exploration and use of the new unconventional sources – formations with low metal content in ore – becomes relevant. The emphasis is placed on advanced processing of ore and integrated metal extraction. An important research objective is to identify the forms of element occurrence and their distribution within the formation.
A persistent horizon of the Early Paleozoic carbonaceous rocks is known in the sedimentary cover section in the northwestern East European Platform within the Fennoscandian Shield framing. The horizon of carbonaceous shale within the Baltic paleobasin extends from the Southern Ladoga area in Leningrad Region across northern Estonia and can be traced to Southern Sweden. A specific feature of rocks is their enrichment in carbonaceous matter and a significant amount of uranium, vanadium and chalcophile elements [1-3]. Increased contents of NM in them were mentioned in a number of articles [4-6]. The objective of the study is to estimate the distribution of NM and rhenium in black shales (BS) of the Baltic paleobasin, determine the occurrence forms of these elements in rock and nodule complex for identifying the possibility of their use as an integrated source of ore raw materials.
Materials and methods
Factual material is represented by samples from exposures along the Baltic-Ladoga Glint (over 80 samples) and from borehole core obtained in the course of implementation of the VSEGEI project 2012-2014 “Prospecting for rhenium in Dictyonema shales and phosphorites of the Baltic Basin in the Kaibolovo-Gostilitskaya area with an estimation of the predicted rhenium resources in categories P2-P1” (more than 700 samples). During field observations, photo documentation, field documentation, sampling and geochemical sampling were carried out. Rock radioactivity averages 120 Bq/m3 (to 250 Bq/m3).
In the course of office processing of samples modern laboratory methods of studying and processing the obtained data were applied. Macroscopic and petrographic investigations were accomplished under a binocular microscope and in thin sections under the optical microscope Leica microsystems DL MP for the reference sample (640 samples). Scanning electron microscopy and micro-X-ray spectral analysis were performed at the Resource Centre of St. Petersburg State University “Geomodel” on a scanning electron microscope TESCANVEGALMU with X-ray fluorescence microanalysis systems Quanta 200 3D and Hitachi (over 1,000 analyses). The samples were divided into silt-sandy and clay components (30 samples). Grain size analysis was performed, and their mineral composition was studied using X-ray phase analysis (Rigaku, Co-cathode) and IR spectroscopy (BRUKER VERTEX 70). The chemical composition of samples was determined at the Central Laboratory of VSEGEI: Corg (infrared spectrometry method, SC-144DR device, LECOCorporation); Ccarb (coulometric method, AN-7529 carbon analyser); C-H-N (10 samples); X-ray spectral silicate analysis (ARL-9800) (640 samples). Noble metals, rhenium and impurity elements were determined by ICP-MS methods on Agilent-7700 and Elan-drc-e devices, by atomic absorption (Analyst-600) (640 samples) and ICP-MS method with laser sampling (laser ablation) (50 samples).
The submicron fraction (SMF) was isolated by aqueous extraction using Sartoris filters with a pore size of 1 μm (86 samples). The SMF is interpreted as a part of the sample where chemical elements are in ionic, molecular and colloidal form with a particle size of 1-1,000 nm, which is extracted with an aqueous solution under specially selected conditions. The SMF was analysed by inductively coupled plasma mass spectrometry (ICP MS) on Agilent-7700 (86 samples). Nanoparticle size determination was performed on a Nanosight nanosizer (30 samples). For X-ray spectral silicate analysis, the samples were pre-crushed and ground to a grain size of 74 μm. After fusion, the tablets were analysed on Agilent-7700 spectrometer. Divalent iron was determined chemically. The ICP-MS method was used to analyse the distribution of microelements. The methodology and analysis algorithm developed for black shale rocks and coals were used [7]. During the analysis, the samples were pre-crushed and ground to a grain size of 74 μm. In order to decompose the samples and dissolve the salts, special purity nitric, hydrochloric and hydrofluoric acids additionally purified by distillation as well as deionized water were used. The analysis of the prepared solutions was carried out on Agilent 7700 and ELAN-6100 DRC. Atomic absorption spectrometry allows the quantitative analysis of the NM (Au, Pt, Pd) content, which was carried out using complete acid digestion with additional melting of the residue. The prepared solutions were analysed on Aanalyst-600 and -800 device. Isolation of the submicron fraction and its ICP-MS analysis were performed at the VSEGEI Central Laboratory. The fundamentals of the method are described in a number of publications and patents [8, 9]. Particle size of the CMF in BS was determined using a universal laser express analyser of particle size distribution (laser granulometer) HORIBA LA-950. The results of all types of analysis were entered into the database and processed using modern software (Microsoft Office, Excel (Microsoft), Corel Draw, Statistica (Statsoft), BioLayout Express3D (Free Software Foundation) etc.).
Discussion of results
Black shale formations of the world
Black shale formations are known in some complexes of different age in Russia and different regions of the world [10-12]. Such formations are known in Poland [13], Scandinavia [14, 15], Spain [16], Canada [17], and China [18]. Black shale sedimentogenesis was most active at the Precambrian/Paleozoic boundary [19-21]. Younger formations are also encountered [22]. The formations containing organic matter were re-tested to estimate the accumulation of NM and rhenium in them [[23-25]. There are numerous publications on metal content of BS, and, in particular, the presence of NM [26-28] and rhenium [29] in them.
Discoveries of large and unique deposits of scarce types of mineral resources in Russia and abroad associated with BS fundamentally changed the attitude to this type of natural formations. It was ascertained that giant deposits of gold, platinoids, uranium and rare metals are genetically and/or paragenetically associated with BS [30, 31]. Large formations are known in Russia, Finland, Sweden, Norway, Uzbekistan, the USA, Canada, Australia, the Czech Republic, China, and Brazil (Table 1). In general, the geochemical spectrum of chemical elements in black shale rocks is extensive and comprises about 20 chemical elements. Most occurrences contain U, V, Mo, Ni, Cu, Zn, Pb, Se, As, Cd, Ag and a number of other elements. To optimize the search for such formations, weathering processes of black shale rocks and mobility of the main and associated elements were studied [32-34].
Table 1
NM and rhenium contents in black shale formations of the world, g/t
Au |
Pt |
Pd |
Re |
Publication |
0.7 |
0.5 |
0.76 |
– |
Chen Nansheng, R.M.Coveney, 1988 (China) |
183 |
0.82 |
1.25 |
– |
[18] (China) |
– |
0.003 |
0.009 |
– |
[19] (China) |
0.10 |
0.52 |
0.26 |
18.5 |
[17] (Canada) |
0.21 |
0.19 |
0.27 |
– |
[26] (Muruntau, Uzbekistan) |
1.5 |
– |
0.1 |
– |
A.Parviainen, K.Loukola-Ruskeeniemi, 2019 (Finland) |
183 |
200 |
65 |
2200 |
[13] (Poland) |
5 |
1.3 |
– |
– |
[26] (Australia) |
– |
– |
– |
1500 |
[26] (Germany) |
– |
– |
– |
21.4 |
S.M.Basitova et al., 1972 (Kirgizia) |
Published evidence indicates that the form of NM manifestation in black shale complexes is complex and diverse, and it is not always possible to determine it. According to the data in article [26], NM minerals found in BS form a complex which was divided into several classes: native metals; metallic solid solutions and intermetallic compounds (natural alloys); sulfides; arsenides, and sulfoarsenides; selenides; tellurides; bismuthides; antimonides. In addition, many new minerals were found in ores of this type including their compounds with biophilic elements – selenium, phosphorus, and arsenic.
Due to the fact that the occurrence form of NM and the spectrum of their accessory elements define their technological properties, the determination of mineral and chemical composition of black shale rocks is among the priority challenges. In recent decades, close attention was paid to rhenium in carbonaceous shales, which contain its elevated concentrations. Rhenium quite rarely occurs as independent mineral forms, therefore, as an isomorphic impurity it is present in the composition of carrier minerals: molybdenite, bornite, chalcopyrite, jordisite, and pyrite.
Thus, the review of the published evidence points to BS enrichment in noble metals and rhenium. The association of metals with BS can be of genetic nature or only spatial (paragenetic). Black shale rocks, along with traditional ores of magmatic origin, can be considered as a potential source of noble metal stratiform mineralization. Black shales are a new raw material source of platinum group metals, gold and rhenium. Therefore, it is important to understand their distribution and occurrence forms to draw up the basis for forecasting, prospecting of this type of formations and drawing-up the processing flowsheets.
Brief information on geological structure of the region
Structurally, the Baltic paleobasin is in the marginal northwestern part of the Russian Plate, in the zone of its structural junction with the southern Fennoscandian Shield. The Early Ordovician paleobasin of carbonaceous shales is conditionally divided into two parts: the western part in Southern Sweden and the eastern part in the Leningrad Region of Russia (Fig.1). The shales received the name of Dictyonema carbonaceous shales due to occurrence of the remains of dendroid graptolites of Dictyonema genus. In accordance with modern terminology, graptolites of this genus have a new name – Rhabdinopora, while the name of these shales remained unchanged.
Geologically, the occurrence area of Dictyonema shales is confined to the Baltic, Ladoga and Prionezhskaya monoclines of the Russian Plate complicated by the extensive Baltic-Mezen tectonic zone traced from the Mezen River Basin in the northeast to the Gulf of Finland in the southwest. They belong to the Lower Ordovician Koporskaya Formation. The most complete sections are confined to the Baltic-Ladoga Glint band, where numerous natural exposures are known along the Syas’, Volkhov, Sablinka, Tosna, Popovka, Izhora, Koporka, Lamoshka and other rivers. Average thickness of the Koporskaya Formation is 2.5-3 m and reaches 8 m.
According to the lithological composition, Dictyonema shales are divided into the lower unit – an alternation unit – and the upper unit – Dictyonema shales proper.
The lower unit is composed of alternating dark brown, almost black mudstones, grey, sometimes brown siltstones and fine-grained sandstones with rare detritus of obolid shells. The thickness of siltstone and sandstone interlayers ranges from 0.5 to 2 cm, the thickness of shales varies between 1-3 mm and the first tens of centimetres. There are interlayers of white spiculites 0.5-2 cm thick. In rocks making up the alternation unit (mainly in its upper part), radial-rayed anthraconite nodules are recorded, carbonate and sulphide (pyrite) cement is found. This unit has a limited occurrence and is developed mainly in the western Leningrad Region. An increase in the unit thickness from 0.0 to 2.6 m from east to west is recorded.
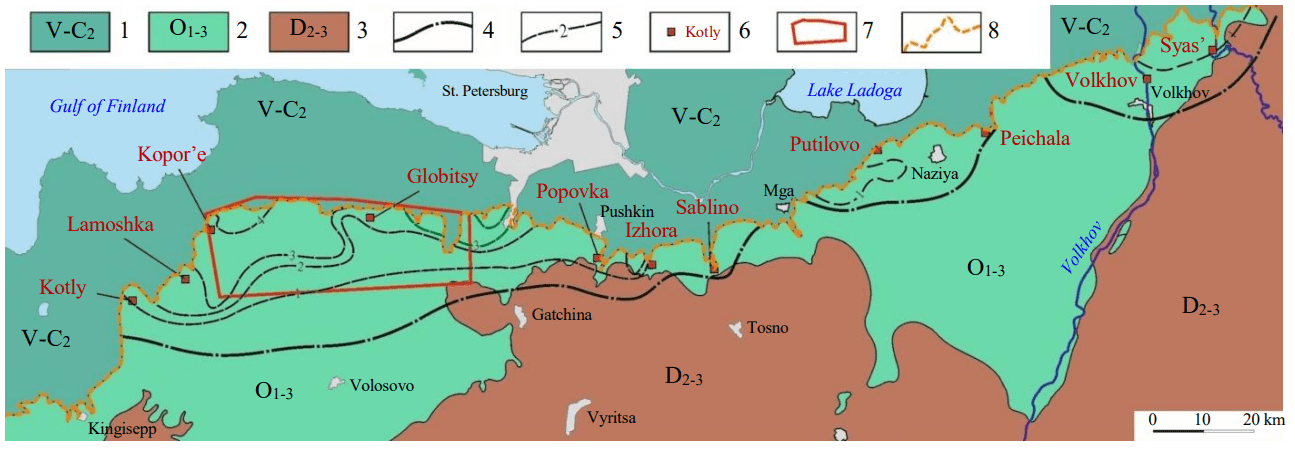
Fig. 1. Occurrence of Dictyonema shale exposures in the Baltic-Ladoga Glint band Sampled NM and rhenium
1 – Vendian-Cambrian deposits; 2 – Ordovician deposits; 3 – Middle-Upper Devonian deposits; 4 – boundary of Dictyonema shale formation wedging out; 5 – isopachs of Dictyonema shale formation, m; 6 – sampled exposures and their names; 7 – boundaries of the Kaibolovo-Gostilitskaya prospecting area, where detailed studies of Dictyonema shale for rhenium were carried out; 8 – Baltic-Ladoga Glint
The upper unit is more widespread than the underlying alternation unit is. It is composed of microhorizontally layered mudstones from pitch-black to greyish black. Sometimes, mudstones have a dark brown tint. Dictyonema shales contain thin interbeds of siltstones and an admixture of silty material, often with an admixture of sponge spicules and their fragments 0.5 to 5 mm thick, to the contacts of which sulfide dissemination is confined. Across the unit section, interbeds enriched in phenocrysts and sulfide pockets are recorded. Thin siltstone interbeds appear in the lower part of the unit. Thickness of the upper unit ranges from 0.5 to 4.0 m and decreases from west to east and from north to south.
According to paleogeographic data, Dictyonema shales formed in the conditions of an epicontinental shallow-water marine basin, the depositional area of which was a wide bay. The composition of sediments and their thickness were affected by the distance from the coastline, the strength of currents, seafloor topography and other factors. A northward increase in the thickness of shales indicates that the axial part of the sedimentary basin, which has a sublatitudinal position, was north of the modern occurrence boundary of shales in the central Gulf of Finland. The northern coast of the basin was land, which at that time was in the southern Fennoscandian Shield and was the main source of detrital material. The Ordovician sedimentary basin had a sublatitudinal orientation and in the west communicated with a deeper basin in the territory now occupied by northern areas of the Baltic Sea. During formation of shales, mass burial of planktonic organisms occurred. This contributed to appearance of a markedly reducing environment and hydrogen sulfide contamination of silt waters in paleobasin, which, in turn, served as a geochemical barrier to the accumulation of elevated concentrations of metals.
Dictyomena shales in Russia are uranium-bearing across their occurrence area. Uranium content ranges from 0.001 to 0.1 % reaching 0.176 % [31]. Contents of other valuable components, %: Mo 0.02-0.049, V 0.09-0.11, Ni 0.010-0.018. The presence of rhenium in oil shales was known since the early 1970s. Active study of shales in the Leningrad Region began in 2012, when the task was set to investigate their rhenium potential.
Structural and textural features of shales
Black shales of the Baltic paleobasin are a dark brown rock, almost black when wet. To identify the structural features of the rock, layer-by-layer sampling of shales from bottom up the borehole and exposure section was accomplished, and their grain size analysis was made. The size of shale particles decreased regularly from bottom up the section. The amount of clay fraction increased from the base to the roof of the formation ranging from 4.5 to 71.7 %. The share of silt-and-sandy fraction varies from 23.8 to 95.5 %. In general, BS are composed of silt-sandy, clay and organic components and a nodule complex.
The silt-sandy component is represented mainly by quartz and potassium feldspar grains of medium roundness, slightly corroded, of a rounded shape. In addition to quartz and feldspar, the following allothigenic minerals were found: biotite, muscovite, Ca-Mg-amphibole, almandine, pyrope, zircon, rutile, rutile-Nb, ilmenite, magnetite, titanomagnetite, corundum, scheelite, Fe and Mn oxides, yellow lead, antimony anhydrite, apatite, xenotime, monazite, and graphite. Research by the FEGI FEB RAS experts revealed native metals and intermetallics: Au, Pd, Os-Ir, Au-Ag, Au-Cu, Au-(Cu)-Hg, Au-Hg, Ag-Cu, and Pt-Fe.
The elutriated clay part of BS was investigated by X-ray phase analysis and IR spectroscopy. It contains, rel.%: hydromica 42-65, kaolinite 13-33, montmorillonite 10-28, and chlorite 1-8. Jarosite, goethite, gypsum, muscovite, hydrotalcite, and alunogen were recorded as impurities.
The organic component of BS is represented by graptolite remains (zoochitinite) as elongated fragments of the skeleton (thecae) clearly distinguished by their colour (from dark brown to black) and clear outlines against the lighter-coloured background of the matrix. Graptolite thecae are found throughout the layer section; they are elongated along the bedding. Oval bodies – pseudovitrinite fragments of unclear origin – are less common. Petrographic studies revealed the inclusions of gelified humus, coal detritus, and cutinite. The amount of organic carbon was studied using infrared spectroscopy. According to this method, the average content of organic matter is about 9.5 % varying in the range of 8-13 %.
Authigenic minerals and nodules are an important component of BS. Nodules are represented by the following mineral types (in order of decreasing prevalence): carbonate, sulphide, silicate, and phosphate. The last two types are rare.
Carbonate nodules are represented by anthraconite and calcite formations of a spherical shape to 10 cm in diameter and a radial-ray internal structure. Detailed studies of carbonate nodule sections revealed their heterogeneous internal structure. Detailed electron probe study revealed the presence of microinclusions of sulfide phases inside carbonate nodules (Fig.2). Sphalerite ranks second in abundance. The size of its segregations reaches 100 μm. Along with iron and zinc sulfides, intermetallics Ni-Cu and Ni-Cu-Zn were found. Native bismuth was recorded. Chalcopyrite and pentlandite are less common. Chalcopyrite contained increased amounts of Co and carbon, and native gold was also found in chalcopyrite.
Sulfide nodules occur mainly as micronodules, less frequently as isolated interlayers. In samples, they are present as flattened rounded segregations (to 4 cm in diameter) and interlayers 0.3-0.7 cm thick. The results of scanning electron microscopy showed that they occurred as individual pyrite crystals and framboidal pyrite. The leading minerals of sulfide nodules are pyrite and, presumably, marcasite. In addition, mackinawite, greigite and iron sulfides of non-stoichiometric composition were diagnosed. Among individual grains of pyrite and marcasite contain an admixture of platinum – to 2.5 wt.%. Admixtures of Ni, Co, Zn, Cu, and As were found in pyrite. Other sulfides, sphalerite (with admixture of Cd, Fe), chalcopyrite with admixture of gold (8 wt.%), galena (with admixture of Zn), bismuthinite and pentlandite (with admixture of Co) are recorded.
Native elements are represented by a number of minerals: gold, iron, aluminium, bismuth and copper. Oxide (Ni, Cu, Zn)O, intermetallics Ni-Cu-Zn, Zn-Cu were identified. Gold is commonly present in chalcopyrite micronodules. Phosphate nodules are rare in BS. Apatite is the most typical mineral, fluorapatite and chlorapatite are less common. Silicate nodules were also found in BS. They are predominantly spherical, with an oval habitus, and their sizes range from 50 to 200 µm. Areas enriched in carbonaceous matter are distinguished in the matrix.
Petrographic studies and scanning electron microscopy results allowed establishing the formation sequence of the nodule complex. The earliest formations are sulphide phases, native metal and intermetallic segregations. They are found inside phosphate and silicate nodules, which, in turn, are enclosed in carbonate nodules. Shales contain minerals of the salt group. The following was found in BS: gypsum-anhydrite, anglesite, Ba-bearing anglesite, massicot and plattnerite, barite, cornelite, destinezite-diadochite, and graphite. Sylvine and halite occur among the chlorides.
Thus, the authigenic complex of minerals is represented by individual crystals, aggregates and nodules. A wide range of minerals was found in nodules: carbonates, sulfides, native metals and intermetallics, oxides, silicates, phosphates, and salts.
Macroelement composition of shales
Data on the content of petrogenic oxides in BS were compared with the sialite standard in article [1] which showed the similarity of compositions (Table 2). High values of SiO2 were recorded in shales, which is accounted for by a significant proportion of silt-sandy impurity in rock. In addition, shallow-water accumulation conditions are characterized by elevated values of Fe2O3.
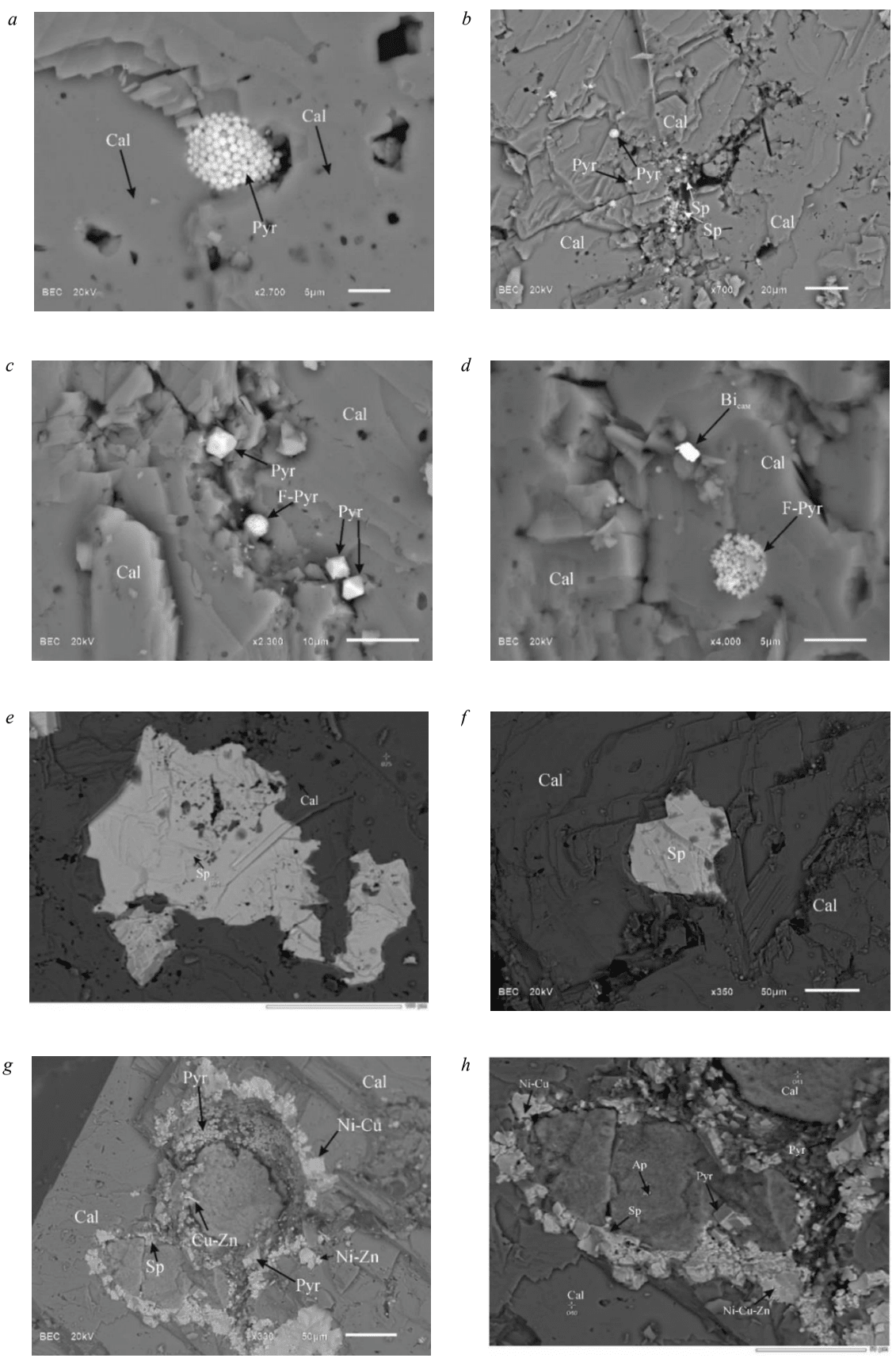
Fig.2. Electron microscopic images of carbonate nodules, BSE images:
a – framboidal pyrite; b – pyrite and sphalerite; c – pyrite crystals and framboidal pyrite; d – framboidal pyrite and Binat; e, f – sphalerite (light); g, h – pyrite, sphalerite, intermetallics Ni, Cu, Zn
Table 2
Average contents of petrogenic oxides in BS, wt.%
Petrogenic oxides |
Average (n = 560) |
Standard deviation σ |
Carbonaceous sialite (Ya.E.Yudovich et al., 1988) |
SiO2 |
58.3 |
8.57 |
54.02 |
TiO2 |
0.55 |
0.11 |
0.87 |
Al2O3 |
8.41 |
2.11 |
9.11 |
Fe2O3 |
5.89 |
1.88 |
2.68 |
MnO |
0.03 |
0.02 |
0.01 |
MgO |
0.99 |
0.33 |
1.19 |
CaO |
2.30 |
0.92 |
0.56 |
Na2O |
0.11 |
0.02 |
0.17 |
K2O |
5.03 |
1.21 |
4.31 |
P2O5 |
0.42 |
0.26 |
0.11 |
POI |
18.05 |
7.78 |
26.97 |
Noble metals, rhenium and accessory elements
The contents of NM and rhenium are presented in Table 3. The methodology and analysis algorithm developed for black shale rocks and coals were used in the analysis of rocks [7]. In general, platinum content in BS is low, often at the detection limit (0.001 g/t), but sometimes reaches the values of 0.051 g/t. Palladium content varies from 0.001 to 0.076 g/t, gold is negligible and averages 0.003 g/t.
Table 3
Average contents of NM and rhenium, g/t
Chemical element |
Average (n = 100) |
Standard deviation σ |
Minimum commercial concentrations |
Shales(E.V.Sklyarov et al., 2001) |
Au |
0.003 |
0.003 |
0.1 |
0.0033 |
Pt |
0.009 |
0.008 |
0.03-0.08 |
0.001 |
Pd |
0.01 |
0.009 |
0.03-0.08 |
0.001 |
Re |
0.133 |
0.093 |
0.008-0.045 |
0.001 |
Special emphasis was placed on the analysis of BS for rhenium. Average Re content in refe-rence sections along the glint range from 0.01 to 1.54 g/t. The highest values were recorded in sections of the Popovka (1.54 g/t), the Volkhov rivers (0.32 g/t) and Globitsy settlement (0.29 g/t). In the section of the Dictyonema shale sequence, the highest contents are confined to the upper part of the section. In the area of the greatest shale thicknesses in the western Leningrad Region, rhenium content is 0.14 g/t (Kopor’e section).
Rhenium in BS has a polymodal distribution, therefore, the sample set was divided into four blocks by its content, g/t: less than 0.005 (22 samples); 0.005-0.05 (134 samples); 0.05-0.27 (494 samples); 0.27-1.54 (24 samples). The obtained data revealed the distribution character of rhenium contents, most of which are above the clarke values.
The contents of accessory elements in BS are presented in Table 4.
Table 4
Average contents of accessory elements in BS, g/t
Chemical element |
Average (n = 560) |
Standard deviation σ |
Clarke [1] |
Clarke of concentration Cc |
Commercial clarke Ccom* |
Commercial clarke of concentration Cccom |
U |
168.7 |
96.9 |
8.5 |
15.1 |
100 |
1.3 |
V |
773.3 |
359.7 |
205 |
3.4 |
224 |
3.1 |
Mo |
159.5 |
103 |
20 |
9.3 |
100 |
1.9 |
Cu |
89.8 |
21.5 |
70 |
1.3 |
100 |
0.9 |
Co |
12.3 |
4.6 |
19 |
0.5 |
14 |
0.7 |
Ni |
132.4 |
58.5 |
70 |
1.4 |
67 |
1.5 |
Zn |
33.2 |
10.7 |
130 |
0.3 |
500 |
0.1 |
Sb |
6.5 |
3.1 |
5 |
1.2 |
30 |
0.2 |
Ga |
12.1 |
3.2 |
16 |
1.0 |
19 |
0.8 |
Ge |
1.6 |
0.5 |
2.4 |
0.6 |
5 |
0.3 |
Sc |
8.2 |
2.1 |
12 |
0.8 |
5 |
2.0 |
Cr |
50 |
11.9 |
96 |
0.7 |
81 |
0.8 |
Rb |
82.2 |
21.1 |
74 |
1.5 |
71 |
1.6 |
Sr |
64.5 |
13.3 |
190 |
0.4 |
775 |
0.1 |
Ba |
346.2 |
76 |
500 |
1.0 |
590 |
0.8 |
Li |
15.7 |
4.7 |
31 |
0.7 |
n.d. |
– |
Y |
42.7 |
14.4 |
26 |
1.1 |
n.d. |
– |
Zr |
159.7 |
47.6 |
120 |
1.2 |
670 |
0.2 |
Nb |
11 |
2.1 |
11 |
1.2 |
70 |
0.2 |
Cs |
3.5 |
1.1 |
4.7 |
1.5 |
3 |
2.8 |
Ta |
0.9 |
0.2 |
0.7 |
1.6 |
1 |
1.7 |
* Standardized for minimum commercial values of chemical elements at which the mineral is fit for economic development.
Accessory elements of NM and rhenium in shales are Mo, Ni, Zn, Pb, U, Se, V, Tl, Ba, and in shales from the alternation unit – U, Se, Sb, V, Ni, Cu. Increased rhenium contents are characteristic of samples with Corg content 7-8 %. According to the results of clarke analysis, the excess of clarke values for U, V and Mo was recorded. Clarke of concentration (Cc) for uranium is 8-16, molybdenum – 4-11, vanadium – 3-5.
Geochemical data on BS composition were processed using correlation analysis. The results are shown in Fig.3. In BS, Pt, Pd, and Au elements have very weak bonds with each other; this group is isolated from other elements. Rhenium correlates with organic matter, V, Mo, Ga. Silver shows strong correlation links with Sc and V as well as with U, Mo, and chalcophile elements.
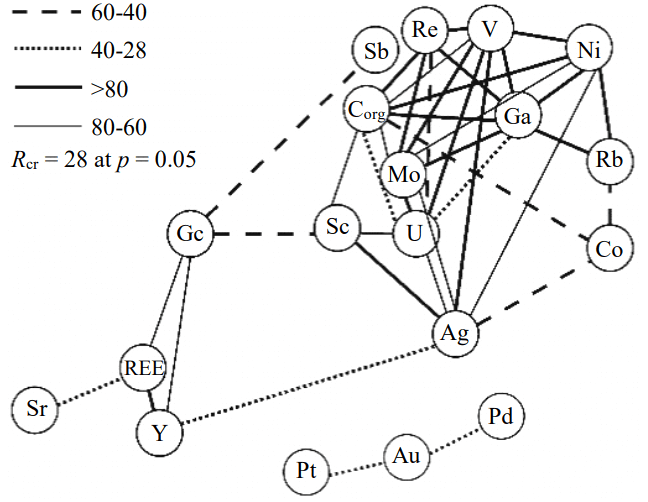
Fig.3. Scheme of branching connections of NM, rhenium and accesory elements in BS
Numbers indicate the intervals of values of the correlation coefficient; rcr is critical value of the correlation coefficient; р = 0.05 (95 % probability)
Forms of NM and rhenium occurrence
As is known, chemical elements are found in nature in mineral and non-mineral forms. The latter include simple and complex ions, colloidal particles, sorbed and suspended particles [8, 9]. In addition, they are divided into mobile and firmly bound ones based on extractability from rocks by simple leaching without destruction of mineral lattice. It is well known that the mineralogical and chemical composition of rocks determines their technological properties and schemes for extracting useful components [35-37]. Published data indicate that NM in BS are found in various forms, which is important when drawing-up the extraction scheme for each specific formation. Mineral form is most favourable [38, 39]. A reliable method for their extraction from sulfides was developed. At the same time, it is known that the proportion of an element in a non-mineral form in nature is higher than its proportion in mineral form [40, 41].
Mineral forms of elements
The search of NM mineral phases in BS of the Baltic paleobasin received great attention in our studies [42]. For the first time, the proportion of colloidal salt component of rock and the accumulation of NM and rhenium in this fraction were estimated. The silt-sandy admixture contains native metals and intermetallics: Aunat, Pd, Os-Ir, Au-Ag, Au-Cu, Au-(Cu)-Hg, Au-Hg, Ag-Cu, and Pt-Fe. Along with them, native Fe, Al, Cu, Fe-Ni, Ni-Fe, Cu-Zn as well as tellurides and bismuth oxides are found in samples. Mineral phases of NM – Aunat, chalcopyrite with an admixture of Au – were found in the nodule complex. They are in association with pyrite with admixtures of Ni, Co, Zn, Cu, As, sphalerite with admixtures of Cd, Fe and pentlandite. Thus, a conclusion can be drawn about the presence of mineral and micromineral forms of NM occurrence in BS. This share is extremely small, occurs sporadically and is 1-5 rel.%.
Non-mineral forms of elements
The most important forms of presence of elements in carbonaceous shales can be the sorbed (on organic matter and clay minerals) and colloidal-salt forms.
Association of NM with organic matter of shale
BS contains 8-13 rel.% Corg. The correlation analysis revealed strong correlation links of organic matter with rhenium. Microprobe analysis was conducted over the sample area for estimating the content of dispersed phases of NM. The contents of these elements appeared to be below the detection limit. Thus, organic matter of BS in the Baltic paleobasin practically does not accumulate NM, but is enriched in rhenium, uranium, vanadium, and molybdenum.
Association of NM with clay part of shale
Samples with high values of NM, rhenium and other accessory elements were taken from the general geochemical sample. Clay component (Cl) was isolated from shale samples, and ICP-MS analysis of the shale and Cl samples was made separately (Table 5).
Table 5
Average contents of chemical elements in a BS sample and its clay component, g/t
Sample |
Pd |
Pt |
Au |
Re |
U |
V |
Mo |
|||||||
BS |
Cl |
BS |
Cl |
BS |
Cl |
BS |
Cl |
BS |
Cl |
BS |
Cl |
BS |
Cl |
|
1 |
0.06 |
0.45 |
0.012 |
0.11 |
0.067 |
0.093 |
0.15 |
1.23 |
64 |
119 |
279 |
1,760 |
83 |
782 |
2 |
0.05 |
0.36 |
0.011 |
0.089 |
0.034 |
0.089 |
0.22 |
1.14 |
172 |
310 |
1,483 |
2,360 |
176 |
217 |
3 |
0.07 |
0.77 |
0.078 |
0.55 |
0.078 |
0.13 |
0.29 |
1.56 |
152 |
204 |
860 |
653 |
114 |
218 |
4 |
0.29 |
1.05 |
0.049 |
0.083 |
0.066 |
0.28 |
0.17 |
1.22 |
123 |
278 |
287 |
1,087 |
122 |
302 |
Clay component is enriched in NM, rhenium and other accessory elements. This is accounted for by a higher sorption capacity of clay minerals. Clay fraction enrichment in platinum and palladium is approximately twofold versus that of the shale sample. Rhenium content in the clay part is 3-7-fold versus that in the sample as a whole.
Colloidal-water-soluble forms of NM in BS
Chemical elements in black shale rocks can be dispersed and represented by ultra-small particles – less than 1,000 nm. Important results shedding light on the occurrence forms of NM and Re in Dictyonema shales were obtained during extraction of the SMF from shales. The first experiments were carried out on a BS hand specimen from the Leningrad Region with rhenium content 1.75 g/t. Yield of the SMF from the shale sample was 1.94 %. In the course of further research, the maximum rhenium content in the SMF was obtained – 22.6 g/t. At the above rhenium content in the sample, its extraction into the colloidal salt fraction was 25.3 %. Water extraction of a part of rhenium from BS can be accounted for by the fact that this element is present in rock mainly in a dispersed state without forming its own mineral forms. Localization of rhenium nanoparticles in cracks and pore space of rock is most probable. In this case, if there is the maximum access of water to the localization site, a colloidal solution of rhenium particles forms (in combination with salts and nanoparticles of other elements). Impurity elements accompanying rhenium in the SMF behave similarly.
Proportion of the SMF in different samples is not the same and varies from 0.1 to 6 wt.%. A total of 71 samples of this fraction were isolated and analysed. According to the measurement results, the particle size of this fraction varies in the range of 300-500 nm. The particle size distribution is symmetrical, the average particle size is 400 nm, but the solution also contains particles of both larger (to 1 μm) and smaller (less than 100 nm) sizes.
Table 6
Average contents of NM and rhenium in SMF BS, g/t
Element |
SMF (n = 71) |
BS |
Accumulation coefficient (SMF/BS) |
Au |
0.85 |
0.003 |
283 |
Pt |
0.60 |
0.009 |
67 |
Pd |
4.51 |
0.015 |
301 |
Re |
4.62 |
0.133 |
35 |
Table 7
Geochemical potential of NM, rhenium and accessory elements in BS and their SMF
Element |
BS |
CMF |
Au, t |
370 |
15.6 |
PGM, t |
9,166 |
280 |
Re, t |
577.5 |
85.5 |
U, |
564.8 |
5.3 |
V, thousand t |
2,856 |
52.7 |
Mo, thousand t |
380 |
5.7 |
Cu, thousand t |
680 |
10.3 |
Ni, thousand t |
342 |
4.7 |
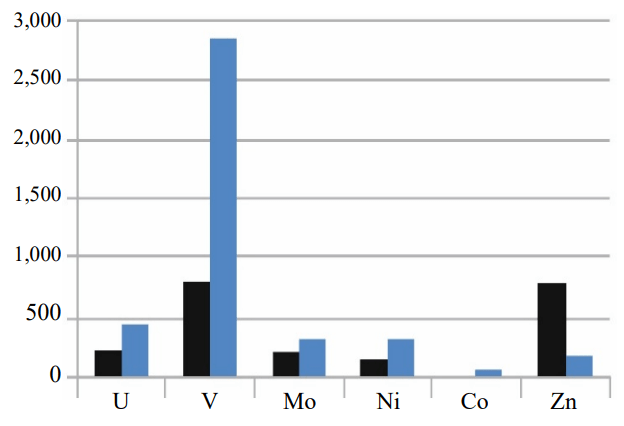
Fig.4. Average content of accessory elements in BS (black columns) and their SMF (blue columns), g/t
The contents of elements in bulk samples and their SMF were compared, and the accumulation coefficient was calculated (AC = content in the SMF/content in shale). Al2O3, Fe2O3, K2O accumulate in the SMF. Other petrogenic oxides do not pass into solution. For NM and rhenium, the accumulation coefficient turned out to be quite high (Table 6).
A comparison of the contents of accessory elements of NM in black shales and their SMF was accomplished. The results are shown in Fig.4. The highest values of accumulation coefficients are characteristic of vanadium, uranium and chalcophile elements (Mo, Ni, Co). Mineral forms of segregation are characteristic of zinc.
Thus, four forms of NM and rhenium are present in the composition of BS. The first form occurs as an admixture in the composition of sulfide mineralization (pyrite, marcasite, chalcopyrite, galena and other sulfides) present as a fine admixture, nodules or thin sulfide interlayers in shales. The content of sulfides in shales is at the level of 8-10 %. The second form is in clay substance of shales, which is determined by a high sorption capacity of clay material. The third form is characteristic of rhenium and is associated with organic matter of shales. The fourth form is in the composition of easily mobile – ionic, molecular and colloidal – forms.
Estimation of BS as an integratedsource of ore raw materials
In Russia, methods for integrated processing of Dictyonema shales were developed in different years taking into account the specific features of the contents of uranium, molybdenum, vanadium and other useful components, their distribution in shale and a relatively high content of organic matter. In Estonia (Sillamäe), a mining and metallurgical plant was built which started processing uranium from Dictyonema shales. About 40 t of uranium were obtained in three years. In Sweden, a uranium production plant with a capacity of 120 t operated at the Ranstad deposit from 1965 to 1969.
Knowledge of the distribution volumes of BS in the Baltic paleobasin, the contents of NM and rhenium in them allows calculating the geochemical potential of rocks (Table 7). When calculating the predicted resource, the following formula was adopted as the initial one
where q is specific ore content, t/km3; W – the volume of ore-bearing formation, km3.
The specific ore content is determined from the formula
where d is density of ore-enclosing rock, d = 2.403 t/m3; Cav is average content of the element.
Volume of ore-enclosing formation is calculated from the formula
where S is the size of prospecting area, km2; h – thickness of ore-enclosing formation, m.
Thus, the formula for geochemical potential has the form
Knowledge of the forms of NM occurrence in black shale will allow estimating the technological prospects of developing this type of formation. Data on the content of accessory elements will allow implementing a comprehensive approach to estimating this type of raw material.
Conclusions
Elevated contents of NM in BS of the Baltic paleobasin are distributed unevenly along the strike of the horizon – shales are enriched in platinoids and rhenium. The contents of BM and the accessory elements vary irregularly along the section.
Black shales are composed of organic, clay, silt-sandy components and a nodule complex. Noble metals are sporadically found in the silt-sandy admixture as native forms and intermetallics. Micromineral phases of NM were identified in diagenetic sulfides. Clay fraction enrichment in NM is 10-fold as compared to that of shale. Organic matter sorbs NM to a limited extent but accumulates rhenium.
Pore space of black shales contains a colloidal salt component which is represented by particles smaller than 1,000 nm.
The geochemical potential of BS in the eastern Baltic paleobasin is estimated. The geochemical resource of NM, Re and accessory elements for BS as a whole and their submicron fraction is calculated. Black shales are recommended as an integrated source of raw materials.
References
- Ketris M.P., Yudovich Ya.E. Estimations of Clarkes for Carbonaceous biolithes: World averages for trace element contents in black shales and coals. International Journal of Coal Geology. 2009. Vol. 78. Iss. 2, p. 135-148. DOI: 10.1016/j.coal.2009.01.002
- Vyalov V.I., Mironov Y.B., Nezhensky I.A. On the metal content of dictyonema shale in the Baltic basin. Mineral Resources of Russia. Economics & Management. N 5, p. 19-23 (in Russian).
- Karpuzov A.F., Karpunin A.M., Sobolev N.N., Mozoleva I.N. Mineral resources potential of black shale formations of Russian cratonic assemblages. Mineral Resources of Russia. Economics & Management. N 2, p. 12-18 (in Russian).
- Dodin D.A., Chernyshov N.M., Yatskevich B.A. Platinum-metal deposits of Russia. Saint Petersburg: Nauka, 2000, p. 754 (in Russian).
- Chernyshov N.M. Regional and local features and criteria for forecasting and prospecting gold-platinoid mineralization of a black-shale type of the precambrian basement of Kursk-Voronezh region. Proceedings of Voronezh State University. Series: Geology. N 1, p. 79-93 (in Russian).
- Shpirt M.Ya., Punanova S.A., Strizhakova Yu.A. Trace elements in black and oil shales. Solid Fuel Chemistry. Vol. 41. N 2, p. 119-127. DOI: 10.3103/S0361521907020115
- Oleinikova G.A., Shishlov V.A., Fadin Ya.Yu. Elemental Analysis of Coals and Black Shale Rocks by Laser Ablation Mass Spectrometry. Solid Fuel Chemistry. Vol. 49. N 4, p. 254-260. DOI: 10.3103/S0361521915040084
- Oleinikova G.A., Panova E.G., Shishlov V.A., Rusanova L.I. Patent N 2370764 RF. Nanotechnological method of determining presence and quantitative content of rare and dispersed chemical elements in rocks, ore and products from their processing. Publ. 20.10.2009. Bul. N 29 (in Russian).
- Oleinikova G.A., Panova E.G. Geochemistry of Nanoparticles in the Rocks, Ores and Waste. Journal of Earth Science and Engineering. 2011. Vol. 1. N 3. p. 207-219. DOI: 10.17265/2159-581X/2011.03.009
- Berdnikov N.V., Pugachevsky M.A., Komarova V.S. Platinum in carbonaceous schists: morphology, composition and Ores and Metals. 2014. N 6, p. 18-25 (in Russian).
- Baturin G.N., Ilin A.V. Comparative geochemistry of shell phosphorites and Dictyonema shales of the Baltic region. Geokhimiya. 2013. N 1, p. 27-37 (in Russian). DOI: 10.7868/S0016752513010020
- Khanchuk A.I., Plyusnina L.P., Molchanov V.P., Medvedev E.I. Noble metals in carbon-rich metamorphic rocks of the Khanka terrane, Primorie. Russian Journal of Pacific Geology. Vol. 1. N 1, p. 61-70. DOI: 10.1134/S1819714007010071
- Kucha H. Palladium Minerals in the Zechstein Copper Deposits in Poland. Chemie der Erde. 1984. Vol. 43. P. 27-43.
- Bjørlykke A., Olerud S., Sandstad J.S. Metallogeny of Finnmark, North Norway. Norges Geologiske Undersøkelse Bulletin. 1985. Vol. 403, p. 183-196.
- Loukola-Ruskeeniemi K., Lahtinen H. Multiphase evolution in the black-shale-hosted Ni-Cu-Zn-Co deposit at Talvivaara, Finland. Ore Geology Reviews. 2013. Vol. 52, p. 85-99. DOI: 1016/j.oregeorev.2012.10.006
- Canet C., Alfonso P., Melgarejo J.C., Belyatsky B.V. Geochemical evidences of sedimentary-exhalative origin of the shale-hosted PGE-Ag-Au-Zn-Cu occurrences of the Prades Mountains (Catalonia, Spain): trace-element abundances and Sm-Nd isotopes. Journal of Geochemical Exploration. 2004. Vol. 82. Iss. 1-3, p. 17-33. DOI: 10.1016/j.gexplo.2004.01.002
- Hulbert L.J., Carne R.C., Gregoire C.D., Pactunc D. Sedimentary nickel, zinc, and platinum-group-element mineralization in Devonian black shales at the Nick Property, Yukon, Canada; a new deposit type. Exploration and Mining Geology. 1992. Vol. 1. N 1, p. 39-62.
- Shao-Yong Jiang, Jing-Hong Yang, Hong-Fei Ling et al. Extreme enrichment of polymetallic Ni-Mo-PGE-Au in Lower Cambrian black shales of South China: An Os isotope and PGE geochemical investigation. Palaeogeography, Palaeoclimatology, Palaeoecology. 2007. Vol. 254. Iss. 1-2, p. 217-228. DOI: 10.1016/j.palaeo.2007.03.024
- Tao Han, Xiaoqing Zhu, Kun Li et al. Metal sources for the polymetallic Ni–Mo–PGE mineralization in the black shales of the Lower Cambrian Niutitang Formation, South China. Ore Geology Reviews. 2015. Vol. 67, p. 158-169. DOI:1016/j.oregeorev.2014.11.020
- Jianping Wang, Jiajun Liu, Runmin Peng et al. Gold mineralization in Proterozoic black shales: Example from the Haoyaoerhudong gold deposit, northern margin of the North China Craton. Ore Geology Reviews. 2014. Vol. 63, p. 150-159. DOI:1016/j.oregeorev.2014.05.001
- Lingang Xu, Lehmann B., Jingwen Mao. Seawater contribution to polymetallic Ni-Mo-PGE-Au mineralization in Early Cambrian black shales of South China: Evidence from Mo isotope, PGE, trace element, and REE geochemistry. Ore Geology Reviews. 2013. Vol. 52, p. 66-84. DOI:1016/j.oregeorev.2012.06.003
- Lee C.-T.A., Wasserburg G.J., Kyte F.T. Platinum-group elements (PGE) and rhenium in marine sediments across the Cretaceous–Tertiary boundary: constraints on Re-PGE transport in the marine environment. Geochimica et Cosmochimica Acta. 2003. Vol. 67. Iss. 4, p. 655-670. DOI: 10.1016/S0016-7037(02)01135-3
- Arbuzov S.I., Rikhvanov L.P., Maslov S.G. et al. Anomalous gold concentrations in brown coals and peat of the southeastern West Siberian Plate. Izvestiya Tomskogo politekhnicheskogo universiteta. 2004. Vol. 307. N 7, p. 25-30 (in Russian).
- Khanchuk A.I., Rasskazov I.Yu., Aleksandrova T.N., Komarova V.S. Natural and technological typomorphic associations of trace elements in carbonaceous rocks of the Kimkan noble metal occurrence, Far East. Russian Journal of Pacific Geology. Vol. 6. N 5, p. 339-348. DOI: 10.1134/S181971401205003X
- Chernyshov N.M., Chernyshova M.N. Localisation regularities, composition and forecasting and exploration criteria of black-shale gold-platinum ores in Voronezh crystal massif. 2008. N 6, p. 39-53 (in Russian).
- Gurskaya L.I. Platinum metal mineralization of black shale type and criteria for its prediction. Saint Petersburg: VSEGEI, 2000, p. 207 (in Russian).
- Lazarenkov V.G., Petrov S.V., Talovina I.V. Platinum metal deposits. Saint Petersburg: Nedra, 2002, p. 298 (in Russian).
- Khanchuk A.I., Molchanov V.P., Medkov M.A. et al. Processing routes of graphite-bearing rocks in Primorye. Khimicheskaya tekhnologiya. 2009. Vol. 10. N 1, p. 33-36 (in Russian).
- Engalychev S.Yu. Rhenium content in sedimentary cover of the East European Platform. Saint Petersburg: VSEGEI, 2019, p. 288 (in Russian).
- Aleksandrova T.N. Some typemorphic associations of microelements in black shale breeds of the Bureinsky file. Proceedings of the Southwest State University. Series: Engineering and Technology. N 2, p. 95-104 (in Russian).
- Mikhailov V.A., Chernov V.Ya., Kushnerenko V.K. Dictyonema shales of the Baltic Basin – a promising formation for economic development for uranium and other minerals. Materialy po geologii mestorozhdenii urana, redkikh i redkozemelnykh metallov. 2006. N 149, p. 92-98 (in Russian).
- Falk H., Lavergren U., Bergbäck B. Metal mobility in alum shale from Öland, Sweden. Journal of Geochemical Exploration. 2006. Vol. 90. Iss. 3, p. 157-165. DOI: 10.1016/j.gexplo.2005.10.001
- Georgiev S., Stein H.J., Hannah J.L. et al. Chemical signals for oxidative weathering predict Re-Os isochroneity in black shales, East Greenland. Chemical Geology. 2012. Vol. 324-325, p. 108-121. DOI: 10.1016/j.chemgeo.2012.01.003
- Jaffe L.A., Peucker-Ehrenbrink B., Petsch S.T. Mobility of rhenium, platinum group elements and organic carbon during black shale weathering. Earth and Planetary Science Letters. 2002. Vol. 198. Iss. 3-4, p. 339-353. DOI: 10.1016/S0012-821X(02)00526-5
- Aleksandrova T.N., Prokhorova E.O. Modification of properties of rock-forming minerals during flotation. Mining Informational and Analytical Bulletin. N 12, p. 123-138 (in Russian). DOI: 10.25018/0236_1493_2023_12_0_123
- Aleksandrova T., Nikolaeva N., Kuznetsov V. Thermodynamic and Experimental Substantiation of the Possibility of Formation and Extraction of Organometallic Compounds as Indicators of Deep Naphthogenesis. Energies. 2023. Vol. 16. Iss. 9. N 3862. DOI: 10.3390/en16093862
- Chanturia V.A., Nikolaev A.I., Aleksandrova T.N. Innovative Environmentally Safe Processes for the Extraction of Rare and Rare-Earth Elements from Complex Ores of Perplexed Material Composition. Geology of Ore Deposits. Vol. 65. N 5, p. 425-437. DOI: 10.1134/S1075701523050045
- Aleksandrova T., Nikolaeva N., Afanasova A. et al. Extraction of Low-Dimensional Structures of Noble and Rare Metals from Carbonaceous Ores Using Low-Temperature and Energy Impacts at Succeeding Stages of Raw Material Transformation. Minerals. 2023. Vol. 13. Iss. 1. N 84. DOI: 10.3390/min13010084
- Helz G.R., Dolor M.K. What regulates rhenium deposition in euxinic basins? Chemical Geology. 2012. Vol. 304-305, 131-141. DOI: 10.1016/j.chemgeo.2012.02.011
- Ultra- and microdispersed state of mineral matter. Exec. Ed. N.P.Yushkin, A.M.Askhabov, V.I.Rakin. Saint Petersburg: Nauka, 2005, p. 581 (in Russian).
- Konev R.I. Nanomineralogy of Gold Epithermal Deposits in the Chatcal-Kurama region (Uzbekistan). Saint Petersburg: DELTA, 2006, p. 218 (in Russian).
- Fadin Ya.Yu. Noble metals in black shales of the Baltic paleobasin: dis. ... kand. geol.-mineral. nauk. Saint Petersburg: VSEGEI, 2018, p. 20 (in Russian).