Platinum group elements as geochemical indicators in the study of oil polygenesis
- 1 — Ph.D., Dr.Sci. Head of the Department Empress Catherine II Saint Petersburg Mining University ▪ Orcid ▪ Elibrary ▪ Scopus ▪ ResearcherID
- 2 — Ph.D. Associate Professor Empress Catherine II Saint Petersburg Mining University ▪ Orcid
- 3 — Postgraduate Student Empress Catherine II Saint Petersburg Mining University ▪ Orcid
Abstract
This study examines elements of the platinum group (PGE), primarily platinum and palladium, as geochemical indicators in the investigation of oil polygenesis. It has been found that, like other trace elements such as nickel, vanadium, and cobalt, platinum group elements and gold can occur in oil fields at both background levels and in elevated or even anomalously high concentrations. The objective of this research is to analyze PGE and trace elements as geochemical markers to identify the geological factors, including endogenous processes, responsible for these unusually high concentrations in oil. A comprehensive review of the literature on this subject was conducted, along with new data on the presence of precious metals in oils from Russia and globally. The study explores the geological mechanisms behind elevated PGE concentrations in oils, utilizing atomic absorption spectroscopy with atomization in the HGA-500 graphite furnace to measure PGE content. Previously, the tellurium co-deposition method (ISO 10478:1994) was used to isolate noble metals from associated elements. Possible geological origins of abnormally high concentrations of platinum metals in oils have been identified. These include endogenous factors such as the spatial proximity of oil fields to ultrabasic rock massifs, the effects of contact-metasomatic processes, and influences from mantle dynamics. Moreover, data concerning mantle elements can serve as indicators of the depth origins of certain hydrocarbon fluids, thus contributing to the study of oil polygenesis.
Funding
The study was conducted as part of the state-funded project assigned by the Ministry of Science and Higher Education of the Russian Federation (FSRW-2023-0002. Fundamental Interdisciplinary Research of Earth's Interior and Processes for the Integrated Development of Geo-Resources).
Introduction
In 1996, Professor V.G.Lazarenkov of Saint Petersburg Mining University was among the first in global literature to obtain data on the content of platinum group metals and gold in samples of Middle Eastern oil from the Dzhbissa and Omar fields in the Deir ez-Zor region, located in eastern Syria [1]. According to the data, the oil ash from the studied fields contained 4.1 g/t of platinum, 5.9 g/t of palladium, and 1.6 g/t of gold, while the raw oil contained 40 mg/t of platinum, 50 mg/t of palladium, and 14 mg/t of gold. The concentrations of platinum group elements (PGE) in the ash of the studied oil samples exceeded the industrial-grade levels typically found in platinum metal ores [2].
Russian researchers [3] using geoelectrochemical methods have discovered a previously unknown phenomenon in the Earth's crust. Near oil deposits, they identified the formation of directional, inversely superimposed “jet” dispersion halos of mobile chemical elements, including heavy metals. Studies using partial extraction of metals to analyze electrically mobile forms, along with diffusion extraction for weakly fixed and sorbed forms of chemical elements, demonstrated that the accumulation of PGE and gold in oils is greater than that of other heavy metals [4]. In addition to precious metals, researchers have identified approximately 50 micro-components of interest in oils. For instance, an estimated 1 billion tons of heavy oils contain around 100 t of lead, gallium, rubidium, mercury, thallium, selenium, and arsenic, along with 1,000 t of nickel, iodine, bromine, and potassium, over 10,000 t of vanadium, several tons of silver, and hundreds of kilograms of gold. Moreover, new data on the presence of rhenium in oils has emerged [5].
Information on the content and distribution of PGE in oils, both domestically and internationally, remains limited. High concentrations of PGE have been detected in oil samples from Syria, the Orenburg region [6], and certain North Sea fields [7], highlighting the urgent need for research on platinum metals in Russian oil fields. This includes determining average PGE content, understanding their concentration levels, and identifying the nature and geochemical distribution patterns in oils. Such research is critical for several practical reasons: forecasting PGE resources for potential future extraction, investigating the causes behind oil fields with elevated PGE concentrations, and exploring the use of mantle-derived elements as indicators of the depth of origin for certain hydrocarbon fluids. As the global energy industry continues to grow [8], the demand for metals essential to renewable energy infrastructure [9], including platinum group metals, increases – further underscoring the importance of studying PGE in oils.
The primary aim of this study is to investigate PGE as geochemical indicators to identify the geological factors, including endogenous processes, responsible for the formation of abnormally high concentrations of these elements in oils. Additionally, the study seeks to analyze the polygenesis of oils. To achieve this, an extensive analytical review of the literature was conducted, and new proprietary data on the PGE and trace element content in oils from Russia and around the world were gathered. The research also explores possible geological causes behind the unusually high concentrations of PGE and gold in oils.
Materials and methods
The concentrations of PGE and trace impurities were analyzed in oils from various Russian fields, including the Kaliningradskoye (Kaliningrad region), Surgutskoye (Western Siberia), Romashkinskoye (Republic of Tatarstan), Yurubchenskoye oil and gas condensate (Eastern Siberia), and Yaregskoye (Komi Republic) fields. Additionally, two previously studied fields from Syria, Dzhbissa and Omar, were examined. Preliminary research on oil fields in Syria’s Deir ez-Zor region [1] revealed that PGE content is more pronounced in heavy oils compared to light ones. Therefore, we selected samples of high-viscosity oils from these fields for further analytical study.
Laboratory studies on PGE were conducted at the regional analytical center “Mechanobr Engineering Analyt” (Saint Petersburg). The authors based their analysis on well-established methods used for geological materials with low PGE concentrations, adapting a sample preparation technique described in detail in [10]. Samples weighing no less than 40 g were analyzed. After sample preparation, a large mass of ash residue remained. Oil ashing was performed using three different methods with the addition of oxidizers. The dry residue (ash) was dissolved in acids. To concentrate the samples, fire assay concentration with lead was used according to the method of incomplete cupellation. To separate the noble metals from associated elements, the well-known co-precipitation method with tellurium was employed (ISO 10478:1994). For final determination, atomic absorption spectrometry with atomization in an HGA-500 graphite furnace was used. Measurements were carried out on a Perkin-Elmer model 603 atomic absorption spectrophotometer. Electron microscopy studies of basaltic and pyroxene dolerite samples were conducted at the Freiberg University of Mining and Technology (Germany), using a JEOL JSM 6400 scanning electron microscope with a Noran Explorer energy-dispersive system. Imaging conditions included an accelerating voltage of 15 kV and a pulse resolution of 0.89 kHz.
Discussion
The authors have obtained new data on the content of PGE in oils from the Kaliningradskoye and Surgutskoye oil fields, as well as the Romashkinskoye, Yurubchenskoye, and Yaregskoye fields in Russia. Additional samples were taken from oil fields in Syria (Dzhbissa, Omar) and Poland (Gorzhitsa-2, Gorzhitsa-7, Mozov-1, Babimost-1, Kargova-6, Chartov-6, Tsichri-6, Buk-1). As supplementary data, results from external researchers studying oil fields in Western Siberia [11], Orenburg region [6], Poland [12], the United Kingdom [7], Canada [13], and China [14] were utilized. All studied oil fields in Russia are categorized according to their respective oil and gas provinces (Fig.1). Average concentrations of PGE and trace elements in oils from fields in Russia and worldwide are summarized in the accompanying Table.
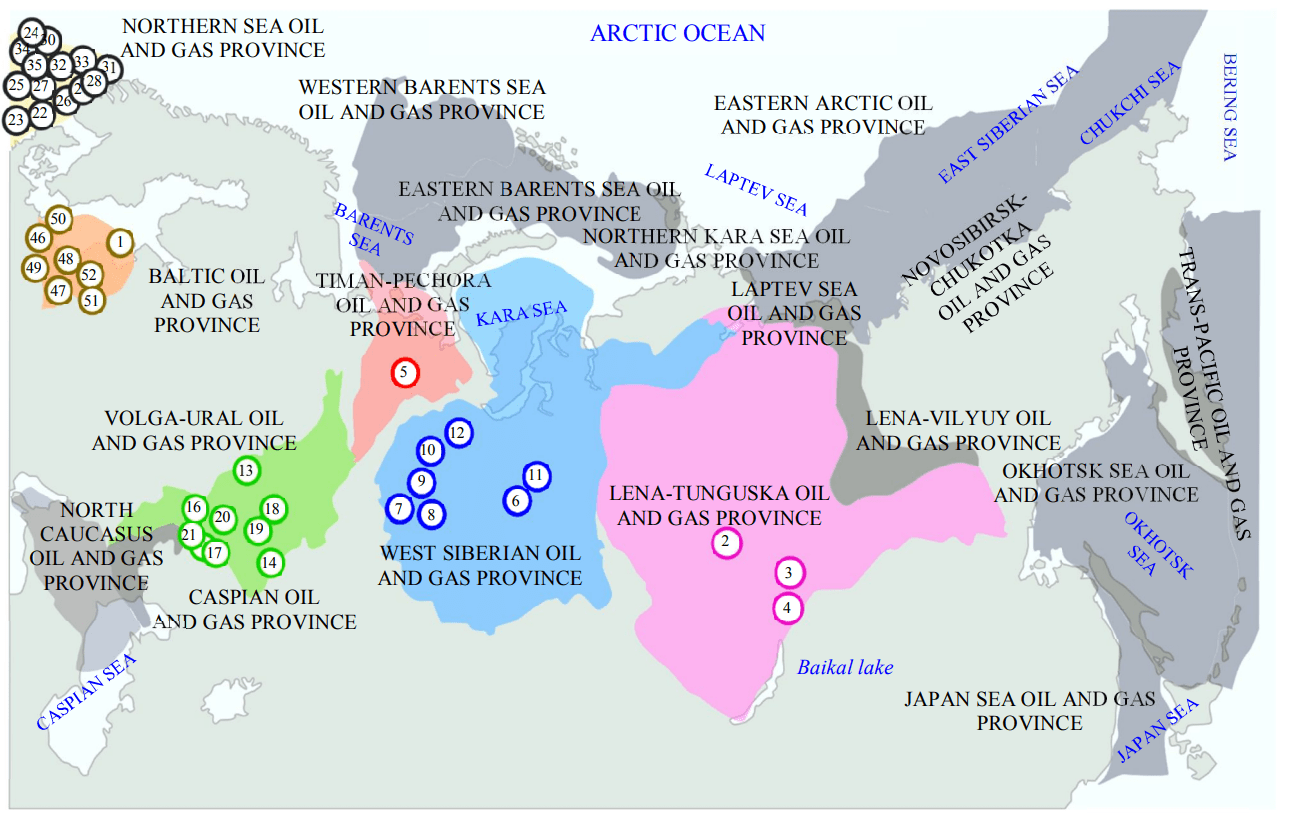
Fig.1. Oil and gas provinces and their associated oil fields. Scale: 1:15,000,000.
The numbers of the fields correspond to the numbers in the Table
From the perspective of metallogenic zoning of oil and gas provinces [15], the Kaliningradskoye and Yurubchenskoye oil fields fall within areas characterized by zinc-type oils, while the Surgutskoye oil fields correspond to nickel-type oils. The Romashkinskoye and Yaregskoye fields are classified as vanadium-type oils with elevated sulfur content. Thus, the oils from the fields we analyzed encompass all types of metallogenic districts within the oil and gas provinces of Russia.
Analysis of the data presented in the Table allows for the following preliminary conclusions:
- Crude oil from Siberia (samples N 2-4, 6-12), Western Canada (samples N 36-42), and China (sample N 43) is characterized by low platinum content, ranging from 0.05 to 5.0 mg/t. In contrast, palladium content in the oils from these fields is found to be higher, ranging from 1.3 to 16.4 mg/t.
- Oil from the Volga-Ural (samples N 13-21) and Mesopotamian oil and gas provinces (samples N 44-46) is enriched with platinum group elements, exhibiting platinum concentrations from 0.4 to 102 mg/t and palladium concentrations from 3 to 70 mg/t.
- Anomalously high platinum concentrations of 319.8 mg/t are observed in the Dauntless field (sample N 22) in the North Sea. In contrast, the oil from most fields in this province (samples N 23-24, 26-28, and 30-35) exhibits low platinum concentrations, ranging from 0.59 to 4.5 mg/t, and very low palladium levels compared to oils from other provinces under consideration [7].
Average concentrations of PGE and other trace elements in oils from fields in Russia and worldwide, mg/t
Pro-vince |
|
Field, site, well |
Pt |
Pd |
Ru |
Rh |
Ir |
Os |
∑PGE |
Au |
Ag |
Ni |
V |
Co |
Pb |
Re |
Baltic (Russia) |
1. |
Kaliningradskoye |
4 |
1 |
– |
– |
– |
– |
5 |
1.2 |
– |
– |
– |
– |
– |
– |
Leno-Tunguska |
2. |
Yurubchenskoye |
0.21 |
2.0 |
– |
– |
– |
– |
– |
3 |
– |
– |
– |
– |
– |
– |
3. |
Northern-Danilovskoye (well 6567) |
0.13 |
6.9 |
5.5 |
0.3 |
0.03 |
0.04 |
12.86 |
0.2 |
53.7 |
1570 |
12110 |
13 |
41.9 |
0.02 |
|
4. |
Danilovskoye (well 2459) |
0.13 |
3.8 |
5.9 |
0.3 |
0.002 |
0.06 |
10.13 |
0.12 |
42.9 |
4290 |
6890 |
17 |
41.4 |
1.2 |
|
|
Average |
0.16 |
4.23 |
5.7 |
0.3 |
0.02 |
0.05 |
11.5 |
1.11 |
48.3 |
2930 |
9500 |
15 |
41.65 |
0.61 |
|
Timan-Pechora |
5. |
Yaregskoye |
0.58 |
7.6 |
5.4 |
– |
– |
– |
13.58 |
0.35 |
– |
47000 |
80000 |
– |
– |
– |
West Siberian |
6. |
Surgutskoye |
0.73 |
7.0 |
5.0 |
– |
– |
– |
12.73 |
0.65 |
– |
– |
– |
– |
– |
– |
7. |
Dorozhnoye (well 1746) |
0.05 |
1.3 |
5.9 |
0.29 |
0.006 |
0.04 |
7.5 |
0.11 |
79.1 |
1690 |
8530 |
15 |
30.2 |
0.03 |
|
8. |
Ust-Teterevskoye (well 1856) |
0.12 |
1.6 |
5.6 |
0.3 |
0.009 |
0.05 |
7.63 |
0.13 |
44.9 |
1580 |
6490 |
15 |
45.8 |
0.02 |
|
9. |
Ubinskoye (well 1236) |
0.33 |
2.2 |
5.1 |
0.7 |
0.006 |
0.02 |
8.34 |
0.19 |
147.5 |
1640 |
6800 |
15 |
304.6 |
0.008 |
|
10. |
Lovinskoye (well 9556) |
0.17 |
1.3 |
5.4 |
0.4 |
0.004 |
0.04 |
7.27 |
0.12 |
14.1 |
1710 |
6100 |
13 |
64.2 |
0.28 |
|
11. |
Eastern-Pridorozhnoye (well 402/2) |
0.94 |
10.6 |
3.3 |
0.3 |
0.37 |
0.06 |
15.51 |
0.39 |
4.2 |
10110 |
10450 |
12 |
140.1 |
5.64 |
|
12. |
Kustovoye (well 1182/26) |
1.19 |
16.4 |
5.8 |
0.2 |
0.27 |
0.05 |
23.86 |
0.02 |
3 |
4580 |
11630 |
0.7 |
103.6 |
2.72 |
|
|
Average |
0.5 |
5.8 |
5.2 |
0.4 |
0.1 |
0.04 |
11.8 |
0.2 |
48.8 |
3551 |
8333 |
11.8 |
114.8 |
1.45 |
|
Volga Ural |
13. |
Romashkinskoye |
5.2 |
7 |
4.1 |
– |
– |
– |
>16.3 |
2.7 |
– |
– |
– |
– |
– |
– |
14. |
Donetsko-Syrtovskoye |
0.4 |
– |
– |
– |
– |
– |
– |
0.4 |
– |
– |
– |
– |
– |
– |
|
15. |
Rostashinskoye |
17 |
3.3 |
– |
– |
– |
– |
>20.3 |
2.6 |
0.1 |
4.6 |
– |
3.3 |
– |
– |
|
16. |
Tananykskoye |
102 |
9 |
– |
– |
– |
– |
>111 |
29 |
68 |
80 |
– |
51 |
– |
– |
|
17. |
Garshinskoye |
56 |
27 |
– |
– |
– |
– |
>83 |
140 |
– |
8.6 |
– |
36 |
– |
– |
|
18. |
Pokrovskoye |
24 |
14 |
– |
– |
– |
– |
>38 |
25 |
– |
12 |
– |
5 |
– |
– |
|
19. |
Pronkinskoye |
3 |
3 |
– |
– |
– |
– |
>6 |
8 |
– |
6.9 |
– |
1 |
– |
– |
|
20. |
Dolgovskoye |
71 |
10 |
– |
– |
– |
– |
>81 |
14 |
– |
19.4 |
– |
4 |
– |
– |
|
21. |
Sakharovskoye |
2 |
3 |
– |
– |
– |
– |
>5 |
2 |
0.1 |
4.6 |
– |
3 |
– |
– |
|
|
Average |
31.2 |
9.5 |
– |
– |
– |
– |
45.1 |
24.9 |
22.7 |
19.4 |
– |
14.8 |
– |
– |
|
Northern Sea |
22. |
Dauntless |
319.8 |
0.14 |
– |
– |
0.31 |
– |
>320.25 |
– |
– |
– |
– |
– |
– |
1.52 |
23. |
Ekofisk |
1.33 |
0.13 |
– |
– |
0.02 |
– |
>1.77 |
– |
– |
– |
– |
– |
– |
0.66 |
|
24. |
West Shetland |
1.38 |
0.03 |
– |
– |
0.01 |
– |
>1.42 |
– |
– |
– |
– |
– |
– |
2.07 |
|
25. |
Abbot |
15.72 |
– |
– |
– |
0.01 |
– |
>15.73 |
– |
– |
– |
– |
– |
– |
1.38 |
|
26. |
Scott |
1.45 |
– |
– |
– |
– |
– |
>1.45 |
– |
– |
– |
– |
– |
– |
1.06 |
|
27. |
Marmion |
0.59 |
– |
– |
– |
– |
– |
>0.59 |
– |
– |
– |
– |
– |
– |
0.94 |
|
28. |
Hannay |
1.05 |
– |
– |
– |
– |
– |
>1.05 |
– |
– |
– |
– |
– |
– |
1.08 |
|
29. |
Telford |
8.35 |
0.28 |
– |
– |
0.31 |
– |
>8.94 |
– |
– |
– |
– |
– |
– |
1.06 |
|
30. |
Sheilhalion |
0.97 |
0.13 |
– |
– |
0.02 |
– |
>1.12 |
– |
– |
– |
– |
– |
– |
0.91 |
|
31. |
Hudson |
1.38 |
0.07 |
– |
– |
– |
– |
>1.45 |
– |
– |
– |
– |
– |
– |
2.4 |
|
32. |
Mobil |
1.1 |
0.06 |
– |
– |
– |
– |
>1.16 |
– |
– |
– |
– |
– |
– |
– |
|
33. |
Kler |
4.5 |
9.5 |
– |
– |
– |
1.17 |
>15.17 |
– |
– |
– |
– |
– |
– |
190.8 |
|
34. |
Miller |
3.5 |
7.6 |
– |
– |
– |
1.16 |
>12.26 |
– |
– |
– |
– |
– |
– |
80.6 |
|
35. |
Foinaven |
3.2 |
8.3 |
– |
– |
– |
0.06 |
>11.56 |
– |
– |
– |
– |
– |
– |
1.19 |
|
|
Average |
26.02 |
2.62 |
– |
– |
0.11 |
0.80 |
28.13 |
– |
– |
– |
– |
– |
– |
22.0 |
|
Western Canada |
36. |
Athabasca |
1.46 |
1.02 |
– |
– |
– |
0.09 |
>2.57 |
– |
– |
– |
– |
– |
– |
16.77 |
37. |
Cold Lake |
1.95 |
2.49 |
– |
– |
– |
0.21 |
>4.65 |
– |
– |
– |
– |
– |
– |
36.58 |
|
38. |
Grosmont |
2.00 |
3.04 |
– |
– |
– |
0.15 |
>5.19 |
– |
– |
– |
– |
– |
– |
26.13 |
|
39. |
Lloydminster |
4.53 |
3 |
– |
– |
– |
0.14 |
>7.67 |
– |
– |
– |
– |
– |
– |
23.47 |
|
40. |
Peace River |
2.45 |
2.8 |
– |
– |
– |
0.06 |
>5.31 |
– |
– |
– |
– |
– |
– |
12.47 |
|
41. |
Provost |
0.64 |
3.84 |
– |
– |
– |
0.048 |
>4.53 |
– |
– |
– |
– |
– |
– |
3.19 |
|
42. |
Vabasca |
1.84 |
2.65 |
– |
– |
– |
0.18 |
>4.67 |
– |
– |
– |
– |
– |
– |
31.55 |
|
|
Average |
2.12 |
2.69 |
– |
– |
– |
0.13 |
>4.94 |
– |
– |
– |
– |
– |
– |
21.45 |
|
Bohaiwan |
43. |
Shengli-Changsheng |
1.29 |
2.65 |
0.09 |
0.07 |
0.03 |
– |
4.14 |
– |
– |
– |
– |
– |
– |
– |
Mesopotamian |
44. |
Dzhbissa-2 |
90 |
70 |
<1 |
<0.7 |
– |
– |
160 |
12 |
– |
20 |
94 |
– |
– |
– |
45. |
Omar-111 |
<10 |
50 |
<1 |
<0.7 |
– |
– |
50 |
<10 |
– |
0.3 |
3.2 |
– |
– |
– |
|
46. |
Omar-137 |
30 |
30 |
<1 |
<0.7 |
– |
– |
60 |
30 |
– |
0.4 |
2.0 |
– |
– |
– |
|
|
Average |
43.3 |
50 |
<1 |
<0.7 |
– |
– |
90 |
17.3 |
– |
6.9 |
33.1 |
– |
– |
– |
|
Baltic (Poland) |
47. |
Gorzhitsa-2 |
5 |
5 |
– |
– |
– |
– |
10 |
5 |
9 |
240 |
340 |
11 |
5 |
– |
48. |
Gorzhitsa-7 |
5 |
5 |
– |
– |
– |
– |
10 |
5 |
13 |
230 |
190 |
10 |
5 |
– |
|
49. |
Mozov-1 |
5 |
5 |
– |
– |
– |
– |
10 |
490 |
5 |
290 |
490 |
34 |
5 |
– |
|
50. |
Babimost-1 |
5 |
5 |
– |
– |
– |
– |
10 |
320 |
10 |
500 |
15 |
51 |
5 |
– |
|
51. |
Kargova-6 |
5 |
5 |
– |
– |
– |
– |
10 |
40 |
5 |
120 |
12 |
4 |
5 |
– |
|
52. |
Chartov-6 |
5 |
5 |
– |
– |
– |
– |
10 |
170 |
6 |
210 |
91 |
11 |
5 |
– |
|
53. |
Tsichri-6 |
5 |
5 |
– |
– |
– |
– |
10 |
5 |
2 |
60 |
3 |
3 |
5 |
– |
|
54. |
Buk-1 |
5 |
5 |
– |
– |
– |
– |
10 |
67 |
3 |
110 |
4 |
5 |
5 |
– |
|
|
Average |
5 |
5 |
– |
– |
– |
– |
10 |
137.8 |
6.6 |
220 |
143.1 |
16.1 |
5 |
– |
Note: Data points 1, 2, 5, 6, 13, and 44-54 are based on the authors' materials; data points 3, 4, and 7-12 are from [11]; data points 14-21 are from [6]; data points 22-32 are from [7]; data points 33-42 are from [13]; and data point 43 is from [14].
- The concentrations of platinum and palladium in the oils from most of the oil and gas provinces examined in this study are found to be higher than the crustal abundances of these elements in the continental crust and sedimentary rocks as a whole, where they correspond to 0.4 mg/t for Pd and 0.4 mg/t for Pt [16].
- In the oils of all the examined fields in Western Siberia, the concentrations of palladium exceed those of platinum by at least an order of magnitude. In contrast, oils from the Volga-Ural and Northern Sea oil and gas provinces generally exhibit the opposite relationship. The oils from Western Siberia are characterized by very high concentrations of nickel, ranging from 1580 to 10,110 mg/t, and vanadium, ranging from 6100 to 11,630 mg/t.
- Limited data are available for the rare platinum group elements, specifically ruthenium and rhodium, whose concentrations are apparently significantly lower than those of palladium and platinum (Pd + Pt > Ru + Rh) in the oils from Syrian fields (samples N 44-46). In contrast, the concentrations of these elements in the oils from Western Siberia are found to be comparable. No discernible patterns in the distribution of osmium and iridium have been observed.
- Elevated concentrations of rhenium are noted in the oils from the North Sea (sample N 33, up to 190.8 mg/t) and in the oil sands of Western Canada (sample N 37, up to 36.58 mg/t) [13].
- The gold concentrations in the table can be categorized into two groups based on their levels: 0.02-14 mg/t and 25-490 mg/t. The values in the first group are evidently close to the background concentrations, noting that the average gold content in the continental crust and sedimentary rocks is 2.5 mg/t [16]. The values in the second group are considered anomalous. This range includes gold concentrations in oils from certain fields in Orenburg (25-140 mg/t) and Syria (up to 30 mg/t). Particularly high gold concentrations are found in the Polish fields of Mozov (sample N 49, 490 mg/t) and Babimost (sample N 50, 320 mg/t). These last two fields exhibit very high concentration values compared to the average gold content in the continental crust.
- The oils from the fields in Western Siberia and Poland exhibit elevated concentrations of silver. In Western Siberia, the range of silver concentrations spans two orders of magnitude (3-147.5 mg/t), while the oils from Polish fields show a relatively uniform distribution of this metal (2-13 mg/t). The silver content in the oils from most fields in Western Siberia exceeds its average crustal abundance of 70 mg/t in the continental crust and sedimentary rocks as a whole [16].
Thus, the background concentrations of noble metals in the oils are one or two orders of magnitude higher compared to the average abundances in the continental crust and sedimentary rocks found in most of the fields examined in this article. In this regard, the oils do not differ from other carbon-rich sedimentary rocks (such as black shales and other high-carbon formations), which also exhibit elevated concentrations of platinum group metals and gold. Alongside the background concentrations, certain oil fields contain high and even anomalously high levels of platinum metals and gold, similar to manganese, nickel, and other trace elements.
Overall, the analysis of platinum group metals and gold concentrations from various oil fields reveals a distinct geochemical specificity among the reservoirs. Notably, Syrian and Orenburg fields are enriched in platinum group elements, Polish fields exhibit higher gold content, and Western Siberian fields show elevated silver concentrations. Data from the Table indicates that oils from the Orenburg region exhibit high levels of platinum group elements alongside significant concentrations of gold and silver (N 13-21). In Western Siberia, a clear correlation can be observed between the concentrations of platinum group elements, cobalt, nickel, and vanadium. This relationship is also evident in incomplete data from fields in the Orenburg region and Poland (N 47-54). For vanadium, a distinct correlation with sulfur is established [5]. Similar to other chemical elements (such as nickel, vanadium, cobalt, etc.), platinum group elements and gold can be found in elevated and even anomalously high quantities in specific reservoirs. This trend is notably observed in the fields of the Deir ez-Zor region (Syria), as well as in the Polish fields of Mozov and Babimost, the Tananykskoye field (Orenburg region), and the Dauntless field (United Kingdom). To adopt a predictive approach for identifying similar oil reservoirs, it is essential to determine the potential geological causes behind the formation of anomalously high concentrations of platinum metals and gold in oils:
- The sedimentation process, which formed the rocks of future oil reservoirs, significantly influences the assimilation of heavy metals by oils from host rocks or mineralized waters during their migration and filtration. Notably, elements such as gold, rhenium, lanthanum, scandium, cadmium, and vanadium are effectively extracted by oil from both acidic and neutral environments [5, 17, 18]. It is plausible to suggest that the spatiotemporal proximity of the formation of Polish and Kaliningrad oils, along with black shales, contributes to the elevated concentrations of platinum metals and gold in these oils when compared to other hydrocarbons [19].
- Hypergenic oxidation or the sulfidization of oil can lead to secondary enrichment with microelements, particularly vanadium. This is especially relevant given that high-sulfur oils often also exhibit high vanadium content [5].
- Epigenetic changes in oils occur as oil reservoirs are subjected to increased temperature and pressure, as well as the aging of the oil. This process results in a depletion of impurity elements associated with the volatile components that are removed, while simultaneously enriching the remaining phase with accumulating elements. Consequently, oils found at greater depths often exhibit higher concentrations of vanadium and nickel.
- The spatial proximity of oil fields to ultramafic rock formations enriched with platinum group elements is significant [20].
- The influence of contact metasomatic processes, which develop during the intrusion of igneous bodies into sedimentary oil and gas complexes, significantly impacts these formations through intense thermal and chemical effects [21, 22]. In the contact zones of intrusions, organic matter decomposes under high temperatures; however, in areas further from the contact, the thermal energy from the intrusions can promote the maturation of hydrocarbons [23].
- The influence of the mantle factor could play a crucial role in the transport of Pt and Au in the form of organometallic compounds along tectonically weakened zones [24, 25]. This process can lead to the precipitation of metals in their native forms when the reduced fluid is oxidized to H2O and CO₂.
Let us examine in greater detail the endogenous factors (points 4-6) that contribute to the formation of abnormally high concentrations of platinum metals and gold in oils, as these factors are crucial for understanding the potential for abiogenic synthesis of specific hydrocarbons.
The influence of spatial proximity to oil fields associated with ultramafic rocks has been thoroughly examined in the works of G.A.Ponomareva and co-authors [20]. In their study of the regional patterns of platinum distribution in oils from the Orenburg region, they divided the western part into two platinum-bearing oil zones: the western zone (Buzuluk Basin and the southern slope of the Tatar Arch) and the eastern zone (Eastern Orenburg Arch, Sol-Iletsk Arch, and the northern edge of the Caspian Depression). According to [20], the boundary between these zones represents a sharp gradient in platinum concentrations, which are more than three times higher in the western region compared to the eastern part. In the western zone, elevated concentrations of palladium, gold, and silver have been established. The distribution of platinum and palladium in the oils is characterized by an ultramafic nature, with average concentrations of Pt at 18 mg/t, Pd at 8 mg/t, and a Pt/Pd ratio of 2.2. The isoconcentration patterns illustrated in the accompanying diagrams correspond to the boundaries of structural-tectonic elements and oil and gas geological districts, often forming steps along these boundaries. Typically, elevated concentrations of noble metals spatially coincide with the outcrops of ultramafic rocks, including peridotite, pyroxenite, and gabbro-norite formations in the crystalline basement, the existence of which has been substantiated by deep drilling revealing the foundation [20].
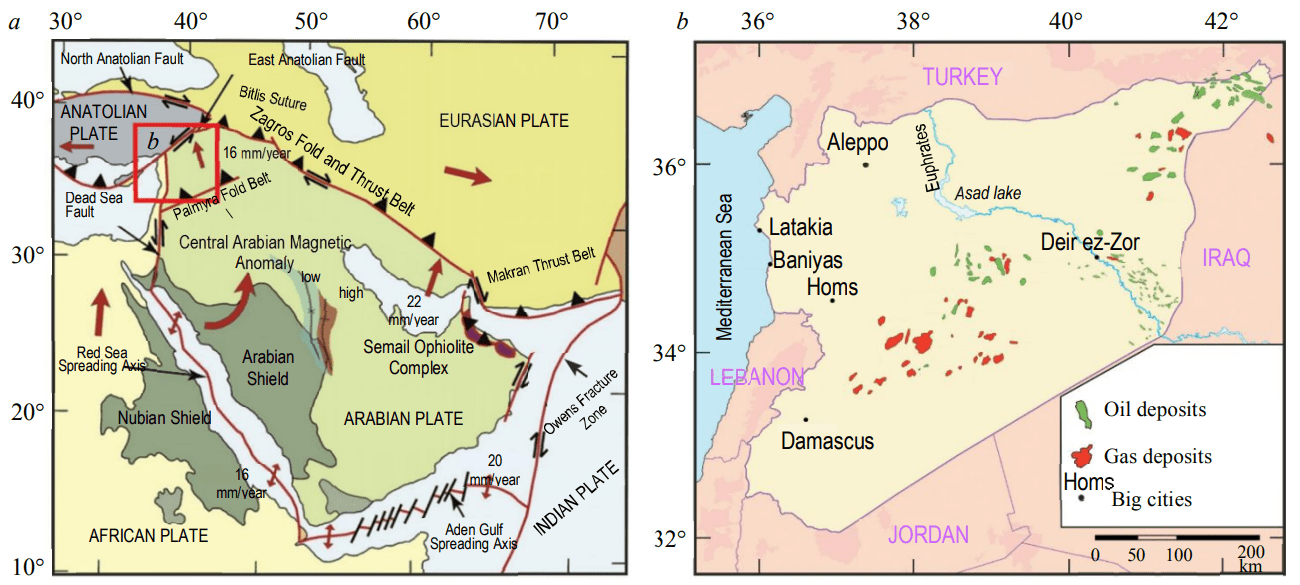
Fig.2. Tectonic map of the Arabian Plate (a); oil fields in the Deir ez-Zor region, Syria [27] (b)
The study [26] demonstrates that gold and platinum are transported as organo-elemental compounds along faults and precipitate in their native forms (C, Au, and Pt) during the oxidation of reduced fluids to H2O and CO2. This migration of Au and Pt is reflected in the hydration and carbonation processes of ultrabasic rocks. These processes can be attributed to the presence of a mixture of heavy hydrocarbons and inorganic compounds (CO2, H2O, N2, etc.) in the upper mantle. As these compounds ascend through deep faults, they facilitate the carbonation and serpentinization of fresh harzburgites. The same authors have shown that an increase in the degree of serpentinization corresponds to a rise in the amounts of carbon and hydrocarbon compounds. A noteworthy example of this phenomenon is the presence of carbonized zones developed within serpentinized ophiolites in the Eastern Sayan, which are rich in a variety of organic compounds, noble metals, and ore minerals, including graphite, diamond, moissanite, native platinum, palladium, gold, osmium, iron, silver, lead, magnetite, chromspinel, ilmenite, rutile, zircon, and others. The isotopic composition of carbonized ultrabasic rocks is similar to that of diamonds, which may serve as significant evidence for the endogenous genesis of hydrocarbons [28].
The impact of contact-metasomatic processes on the formation of abnormally high concentrations of platinum metals and gold in oils can be examined through the example of the Syrian fields in the Deir ez-Zor region, which are representative oil fields of the Middle East (Fig.2). Tectonically, Syria is situated in the northwest part of the Arabian Plate, characterized by numerous ancient and modern tectonic dislocations resulting from the complex interactions among the Arabian, African, and Eurasian plates, as well as the fold-thrust belts of the Zagros and Palmyra regions [29]. A significant feature of the geological structure in the Deir ez-Zor area is the association of the formation of Syrian oil fields with the trap and alkaline magmatic processes that have occurred and continue to occur within the context of Late Mesozoic and Cenozoic riftogenic structures of the Euphrates graben. The spatial relationship between hydrocarbon-bearing and alkaline provinces has been known since the time of R.Daly, yet the reasons for this relationship have not been previously analyzed. It appears that hydrocarbon-bearing and alkaline provinces are products of processes occurring in riftogenic zones and simultaneously act as markers of these zones. An example of such province associations is the West African zone of periocceanic rifts [30] and the West African alkaline province [1]. The traps and alkaline basalts of the Deir ez-Zor region of the Euphrates Rift exemplify this seemingly unusual paragenesis.
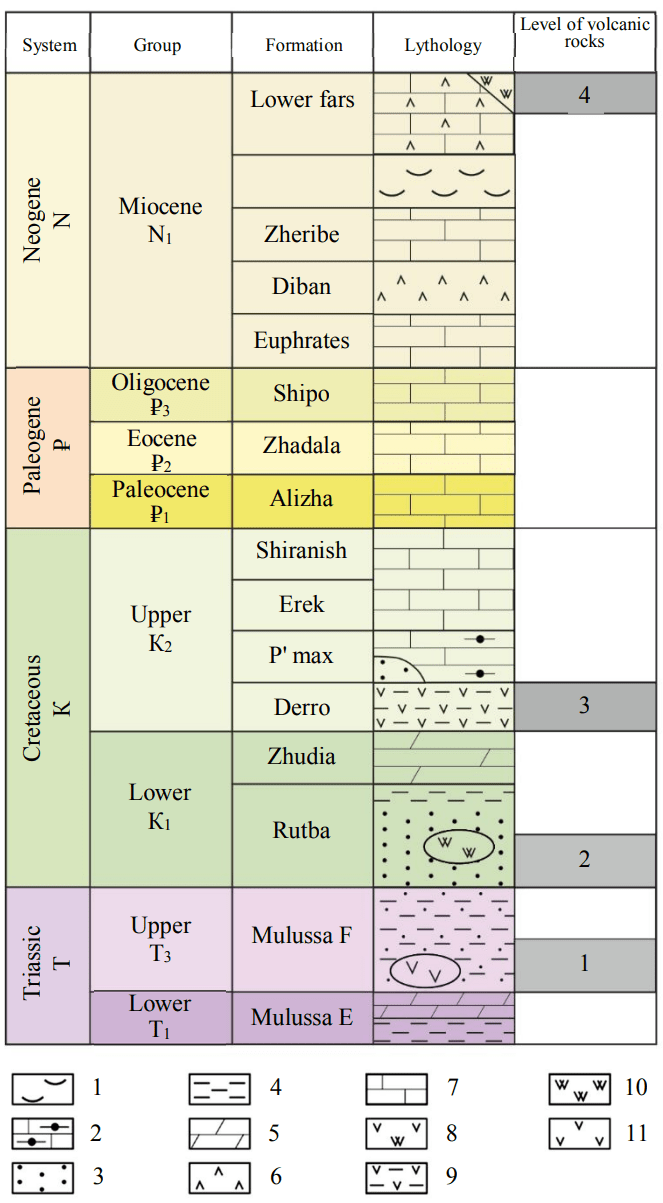
Fig.3. Levels of volcanic rock occurrence in the stratigraphic section of the oil fields in the Deir ez-Zor area, Syria [1]
1 – salt; 2 – limestone with silica inclusions; 3 – sand, sandstone; 4 – clay; 5 – dolomite; 6 – anhydrite; 7 – limestone; 8 – basalt; 9 – weathered basalt; 10 – alcaline basaltoids; 11 – basalt of the trapp formation
Let us consider the relationship between the magmatic process and oil formation using the example of the deposits in the Deir ez-Zor region. The surface basaltoids of Syria have been well studied and described in several works, particularly by E.V.Sharkov and colleagues (1994). Data on the effusive rocks from the deeper horizons of the substantial sedimentary cover, which hosts the country’s oil fields, are provided in [27], based on results from oil drilling to depths of 4,300 m in the Deir ez-Zor region. This area, including the Omar, Izba, and Tayem deposits, is located in eastern Syria within the Euphrates graben. It is composed of a sedimentary sequence, 7-8 km thick, which lies on the Precambrian granite-gneiss basement of the Arabian Plate (Fig.2). The age of the strata ranges from the Early Paleozoic at the base to the Quaternary near the surface. The stratigraphic division of the sedimentary rocks and basaltoids in the deeper horizons of the Deir ez-Zor region is presented in Fig.3. Within the general stratigraphic section of the sedimentary sequence, igneous rocks – predominantly effusive in nature – are distinctly observed at four stratigraphic levels (from bottom to top):
- The normal basalts and dolerite sills are located within the rocks of the Mulussa F Formation, which dates back to the Late Triassic.
- Moderately alkaline basaltoids of Early Cretaceous age (mugearites, teschenites, trachydolerites), located at the base of the Rutba Formation.
- Weathered to red clays basic effusive rocks and their tuffs of the Late Cretaceous Derro Formation.
- Surface basaltoids (basalts, melanocratic basalts, alkaline basalts, nepheline basalts) of Late Cenozoic age, forming small fields in the vicinity of the Deir ez-Zor deposit.
These formations are analogous to the rocks of the Late Cenozoic Miocene-Holocene volcanic region (20.9-0.3 million years), as described by E.V.Sharkov for Western Syria. The thicknesses of effusive rock intervals in wells usually range from several tens of meters, varying from 14 to 83 m, corresponding to one to three overlapping lava flows and sheets. The thickness of dolerite sills ranges from 14 to 95 m.
The subvolcanic rocks of the Deir ez-Zor region can be classified into two magmatic associations: the Upper Triassic basaltic (trappean) and the Meso-Cenozoic alkaline-basaltic. The petrological and geochemical evolution of the platform basaltic magmatism in the Euphrates Rift region demonstrates a transition from normal basalts in the Triassic to alkaline basaltoids in the Quaternary period. This geochemical progression is marked by an increase in rare alkali elements, primarily rubidium, radioactive elements such as uranium and thorium, and other elements including zirconium and strontium [1].
Based on several indirect indicators, the age of the oils in the Deir ez-Zor fields can be considered Cretaceous. Consequently, in terms of the oil formation process, some of the basaltoids in this region are pre-oil, while others are post-oil. The pre-oil basaltoids likely include the Lower Cretaceous alkaline basaltoids of the Rutba Formation and the Upper Cretaceous weathered effusive rocks of the Derro Formation. The post-oil group comprises Paleogene dolerite sills and Late Cenozoic surface alkaline basaltoids. The first group of basaltoids likely played a passive role as seals in relation to the oil-bearing horizons of Deir ez-Zor. The second group, particularly the dolerite sills, likely exerted active thermal influence on the oil reservoirs and the surrounding rocks.
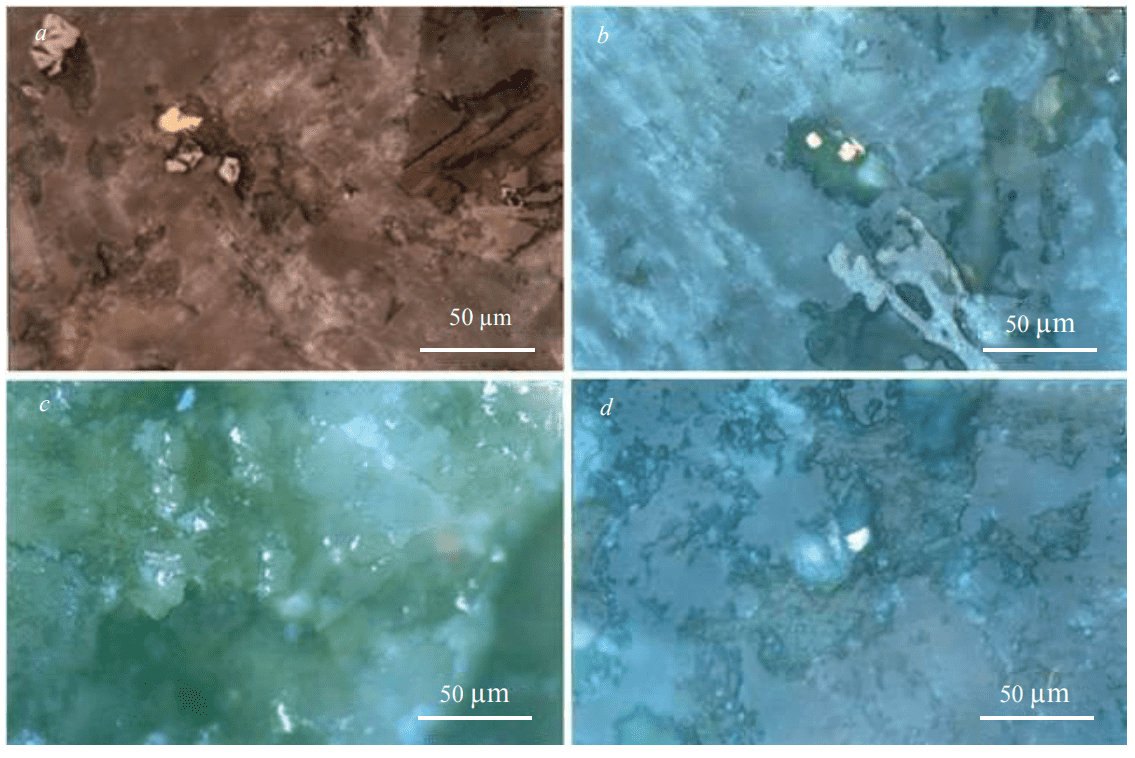
Fig.4. Grains of platinum metals and gold in the basaltoids of deep horizons of Syrian oil fields:
a – Mujerite: grains of gold (20 µm) and titanomagnetite (gray) within feldspar, partially replaced by sphene; a brown biotite plate and pearly carbonate are observed (Omar, 3679 m, ×500, reflected light, parallel polars); b – Pyroxene Dolerite: two grains of gold (0.008-0.010 mm) within pelitized plagioclase (Omar, 4085.95 m, ×500, reflected light, parallel polars); c – Pyroxene Dolerite: a cluster of platinum grains within chlorite and plagioclase (Omar, 4085.95 m, ×500, reflected light, parallel polars); d – Pyroxene Dolerite: a grain of platinum within chlorite on plagioclase (Omar, 4085.95 m, ×500, reflected light, parallel polars)
The analysis of the contact relationships between the intervals of dolerite sills and oil horizons at the Omar field revealed that in most wells, the dolerites were in direct contact with these horizons. Furthermore, the examination of the rocks in the exocontact zone under an optical microscope showed that they are often represented not by typical sedimentary or effusive rocks but by zeolite-chlorite-albite-carbonate metasomatites, i.e., rocks that have undergone the process of metasomatism (Fig.4).
Microscopic studies of sedimentary rocks from the Izba and Omar fields have revealed widespread development of this process, including in the basaltoids and dolerites. It can be concluded that extensive metasomatic alteration of the reservoir sedimentary rocks, as well as the underlying and overlying formations, is associated with contact-metasomatic processes. The most plausible cause of this metasomatism, in our view, is the contact influence of basaltoids on the enclosing rocks, including the earlier dolerite sills. The hot dolerite sills and basaltic dikes, which served as conduits during the formation of late Cenozoic effusive basaltoid fields, likely exerted a strong thermal effect on the oil. Direct measurements indicate that the temperature of basaltic melts ranges from 1450 to 1500 °C [31]. The petrostatic pressure in the oil reservoir, with a roof thickness of 1.0-4.3 km, varied from 0.25 to 1.2 kbar. Based on the stability field of the zeolite-chlorite-albite paragenesis, the thermodynamic conditions of the contact-metasomatic process corresponded to the conditions of the propylitic facies. During the formation of propylites, the thermodynamic conditions align with temperature ranges of 150-300 °C and pressures of 0.3-2.0 kbar. It is evident that similar thermodynamic parameters characterized the metasomatic alteration of the sedimentary and effusive strata of the oil reservoir in the Deir ez-Zor region. Assuming that the oil layers are underlain by water and that carbonate rocks play a significant role in the stratigraphy of the Deir ez-Zor region, it follows that the metasomatic process occurred under high partial pressures of Н2О and CO2.
Considering the relatively high percentage of basaltoids 8.8-19 % within the stratigraphy of the oil reservoir in Deir ez-Zor, along with the elevated temperatures of basaltic magma and its high heat capacity at pressures around 0.5-2.0 kbar, it can be concluded that the dolerite sills exerted an influence on the oil similar to that of the cracking process, leading to the separation of heavy and light components. This assumption is supported by the well-established fact that the oil from the Deir ez-Zor region is lighter compared to that from neighboring northeastern deposits such as Remelan and Suedia, which are located in the Sinjar Trough outside the Euphrates Rift. It is likely that, under conditions of excess pressure, lighter fractions migrated into zones of lower pressure, primarily toward the upper regions of the sedimentary basin. Certain sections of the oil reservoir that underwent thermal influence from dolerite sills may exhibit a different oil composition compared to those that were not subjected to this process. However, it should be noted that the current study did not include an analysis of the physicochemical characteristics of the oil.
The study of ore minerals in polished sections of basalts and pyroxene dolerites revealed the presence of small grains of highly reflective minerals – platinum group elements and gold (Fig.5, a). To investigate the composition of these grains, samples were prepared from basaltoids at a depth of 4085.95 m and from pyroxene dolerites at a depth of 3679 m, and the preparations were examined using a scanning electron microscope. However, due to the small size of the grains, it is not possible to determine their quantitative composition; instead, the qualitative composition is presented in Fig.5. In the dolerite from the Izba deposit, grains of platinum group elements were noted within chlorite and plagioclase. In the porphyritic pyroxene dolerite from the Omar deposit, individual grains of platinum group elements and gold, as well as a concentration of such grains, were found in chlorite developed along plagioclase. Gold grains with a rim of sphene and platinum group elements were identified in the mugearite from the Omar deposit.
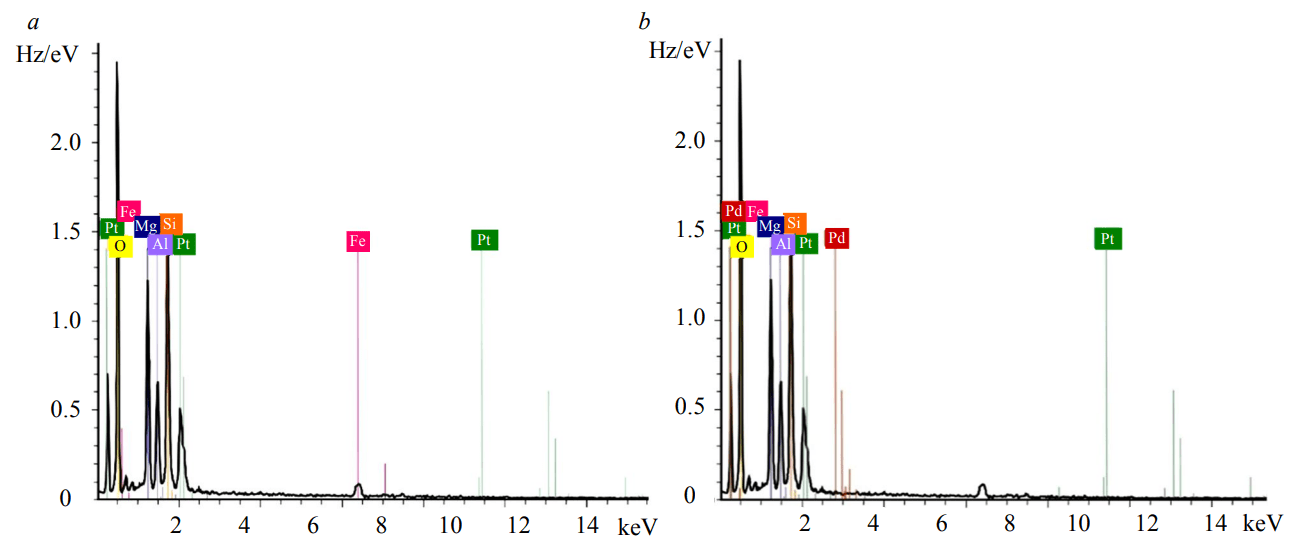
Fig.5. Qualitative composition of PGM grains with a significant platinum content based on scanning electron microscopy in basaltoid (Omar, 4085.95 m) (a); platinum-palladium composition based on scanning electron microscopy in pyroxene dolerite (Omar, 3679 m) (b)
According to the research by V.G.Lazarenkov and I.V.Talovina (2001), the content of PGE in the basaltoids of Syrian oil fields can reach several grams per ton. In contrast, typical PGE concentrations in basalts and dolerites range from several milligrams per ton [32], which is three to four orders of magnitude lower. An exception to this trend is the dolerite sills of Norilsk, which contain PGE concentrations similar to those found in Syrian oil fields [33-35].
Overall, our observations of platinum group minerals and gold indicate that microscopically visible, individualized particles of these minerals are found within secondary chlorite, which develops from plagioclase during the autometasomatic propylitization process at temperatures ranging from 150 to 300 °C and pressures of 0.3 to 2.0 kbar. This suggests that the redistribution of platinum group minerals and gold, as well as their transport into the oil reservoir zone of the Deir ez-Zor region deposits, likely occurred as a result of metasomatism.
A similar geological setting can also be observed in other oil fields, including those in Russia. This scenario may occur when metasomatic injections (commonly traps) penetrate oil reservoirs and exert contact effects on the oil, enriching it with metals that they release into the fluid phase – elements such as iron group metals, platinum group minerals, and others. As search indicators for the influence of magmatic factors on the metal content of oils, two main aspects should be considered: first, the younger age of basaltic injections in relation to oil reservoirs, and second, the geochemical characteristics of these injections, particularly their fluid phase. Further analysis of this issue, involving sampling and analysis of oil in contact with dolerites, undoubtedly represents significant practical interest not only for Syrian oil fields [36, 37] but also for Russian deposits. Another possible reason for the elevated concentrations of noble metals in the oils of the Deir ez-Zor region can be attributed to the mantle factor. The fundamental possibility of abiogenic hydrocarbon generation and the significance of this mechanism in the inorganic genesis of oils have been analyzed in numerous studies [38]. It is noteworthy that the mere presence of elevated platinum group metal concentrations in the oils of the Deir ez-Zor region can be interpreted as indirect evidence supporting the abiogenic hypothesis of hydrocarbon formation, as these metals are typically characteristic of deep Earth zones, potentially even the liquid outer core [39]. Their involvement in fluid metasomatic flows from the Earth's interior is undoubtedly illustrated by the mechanism of formation of the renowned Bushveld platinum pipes of Driekop, Onverwacht, and Moihuk. Another example is the enrichment of platinum group metal in gas streams accompanying volcanic eruptions. For instance, during the 1976 eruption of the Kamchatka volcano Tolbachik, approximately 70 t of iridium were released into the atmosphere [40]. In Hawaii, during the eruption of Kilauea volcano, significant iridium emissions were observed [41]. Academician N.A.Shilo, while studying various gold-bearing deposits, such as Witwatersrand in South Africa and Muruntau in Uzbekistan, noted the coexistence of gold and hydrocarbons at depths of 4005-4300 m within granitoid masses. For example, in the Witwatersrand gold mines, up to 500 million m3 of hydrocarbon gases are extracted annually [42].
The formation of oils in the Deir ez-Zor region occurred during the development of the Euphrates Rift, characterized by volcanic activity and rift-induced destruction of the Earth's crust, which unfolded over an extended and multi-stage process. A complex series of basaltic and alkaline basaltic events accompanied this destruction, with the presence of mantle inclusions of spinel lherzolites and websterites in the late Cenozoic basaltoids of Syria serving as evidence of its deep roots [43].
Alkaline magmatism particularly that of the Cenozoic basaltoids, was likely accompanied by a flow of mantle fluids that utilized the system of disjunctive faults of the Euphrates Rift for their penetration. Consequently, from the perspective of the inorganic hypothesis regarding the formation of oil in the Deir ez-Zor region, a significant or, at the very least, a substantial amount of inorganic hydrocarbons could have been introduced simultaneously with the flow of heat associated with basaltic, alkaline basaltic, and alkaline magmatism of the late Cenozoic age. The propylitic metasomatism that affected all the rocks of the oil reservoir in the Deir ez-Zor region serves as tangible evidence for the feasibility of such heat transfer. Given that platinum group elements and gold are present in a mineralogically individualized form within the chlorite of the propylites, it is plausible that the inferred mantle fluid could have been enriched with platinum group elements and gold.
Overall, analyzing the specific geological context of the formation of the oil reservoir in the Deir ez-Zor region leads to the conclusion that the development of its enhanced platinum content occurred under the influence of deep fluids enriched with PGE and gold. The close temporal relationship between alkaline basaltic magmatism and the process of hydrocarbon formation suggests a possible additional contribution of an inorganic hydrocarbon component to the oil resources of the Deir ez-Zor region. This component likely accompanied the intense alkaline heat transfer during the riftogenic process. The presence of mantle inclusions of spinel lherzolites and websterites in the slag cones of the late Cenozoic basaltoids in Syria indicates the deep mantle roots of this process [43].
The relatively high concentration of PGE in the oils of Syrian deposits raises the important question of conducting a broad analysis of their distribution in Russian oil fields. Of particular interest are those products from which these elements can be profitably extracted alongside vanadium and nickel.
Conclusion
The conducted studies indicate that crude oil from the majority of investigated deposits in Russia (including most fields in Western Siberia, the Kaliningrad region, the Romashkinskoye and Yubrichenskoye fields) typically exhibits low concentrations of palladium (5-7 mg/t) and platinum (0.05-5 mg/t), which are likely close to the background levels of these elements in crude oils. These concentrations are, in fact, an order of magnitude higher in certain samples compared to the average contents of such elements in the continental crust and sedimentary rocks as a whole. Notably, the concentrations of Pt, Pd, and Au are elevated in most fields in Orenburg and in all examined Syrian deposits. In this respect, these oils do not differ from other carbon-rich sedimentary rocks, such as black shales, which are also characterized by increased levels of platinum group metals and gold. Alongside the background concentrations observed in specific oil fields, the platinum group metals and gold exhibit high and even anomalously high levels, similar to manganese, nickel, and other trace elements, indicating a comparable distribution pattern to that found in other sedimentary rocks. The analysis of the concentrations of platinum group metals and gold from various oil fields also revealed a distinct geochemical specificity of the deposits. Specifically, Syrian and Orenburg fields are enriched with platinum group elements, Polish fields with gold, and Western Siberian fields with silver.
In order to adopt a predictive approach for identifying similar oil fields, it is essential to determine the potential geological reasons for the formation of anomalously high concentrations of platinum group metals and gold in crude oils. In our view, the following factors may contribute to these elevated concentrations:
- the influence of the sedimentation process characteristics, during which the rocks of the future oil reservoir were formed, leading to the subsequent assimilation of heavy metals from the enclosing rocks or mineralized waters during the migration and filtration of the oils; hypergenic and epigenetic alterations of the oils;
- the factor of spatial proximity between oil fields and ultramafic massifs, which could have been the primary source of platinum metals, both during the formation of high-carbon oil source rocks and through the hydrogeneous redeposition of platinum metals by groundwater;
- the magmatic factor and the contact-metasomatic influence of magmatic intrusions, which could have enriched oil with metals released into the fluid phase – elements of the iron group, platinum metals, and others;
- the influence of mantle fluids, which carried platinum group elements from Earth's outer liquid core along with hydrogen. The enrichment of Earth's core with these elements, as well as with nickel and other elements of the iron group, is well known. Identifying and establishing correlations between platinum group metals and nickel, vanadium, and iron group elements holds genetic and exploratory significance. This factor could have operated independently from, or in close interaction with, the magmatic factor.
In general, our analysis of the geological reasons for the formation of high and anomalously high concentrations of platinum group metals in various oil fields can be viewed as a set of positive exploration indicators. These indicators can be utilized in the search for and identification of oil deposits with elevated concentrations of platinum group metals.
Using this approach, it makes sense to analyze the concentrations of platinum group metals in the oils of those fields located in relative proximity to ultra-basic massifs. None of the fields whose oils we analyzed in the table belongs to such oil fields. However, such fields are known in Russia. Considering that oils in provinces containing enriched platinum group metal ultra-basic massifs (layered, zonal, ophiolitic) are likely to be enriched with platinum group metals, we propose to analyze the oil fields of the Republic of Bashkortostan and the Yamal Peninsula, adjacent to the Ural Province of ultra-basic rocks. Moreover, the metallogenic zoning map of oils in the Russian Federation indicates that the oil fields adjacent to the Urals exhibit vanadium geochemical specialization. In this regard, the oil fields of the Nuralinsky shaft (A.S.Egorov, 2018) – specifically, the Novy Port, Bovanenkovskoye, and Kruzenshternskoye fields – are of particular interest. All of these fields are located on the northern-northwestern fault of the Nuralinsky shaft, and ophiolitic massifs of the Polar Ural are presumed to lie beneath them. Another potential object for studying the platinum metal content of petroleum products in this region is the Yamburg field.
From a genetic perspective, determining the content of platinum metals in the oils of Venezuela and the Gulf of Mexico is of particular interest. In these areas, oil accumulations are found in chalk deposits that directly overlay the serpentinites of ophiolitic massifs. Geochemically, Venezuelan oils are renowned for containing record amounts of vanadium, a typomorphic siderophile of ultrabasic rocks. The fame of Gulf of Mexico oils is also linked to the assumption that this region represents an area of contemporary hydrocarbon generation.
References
- Lazarenkov V.G., Ganai A.Kh.M., Kolosova L.P. et al. Distribution of platinum group elements and gold in the oils of the Jbissa and Omar fields (Syria). Doklady Earth Sciences. 1998. Vol. 360. N 1, p. 93-95 (in Russian).
- Aleksandrova T.N., O’Connor C. Processing of platinum group metal ores in Russia and South Africa: current state and prospects. Journal of Mining Institute. 2020. Vol. 244, p. 462-473. DOI: 10.31897/PMI.2020.4.9
- Putikov O.F., Veshev S.A., Voroshilov N.A. et al. Jet halos of dispersion of base metals over oil-gas deposits and their application in evaluation of oil-gas pools parameters. Doklady Earth Sciences. 2000. Vol. 370. N 5, p. 668-671 (in Russian).
- Putikov O., Kholmyanski M., Ivanov G., Senchina N. Application of geoelectrochemical method for exploration of petroleum fields on the Arctic shelf. Geochemistry. 2020. Vol. 80. Iss. 3. N 125498. DOI: 10.1016/j.geoch.2019.02.001
- Nadirov N.K., Kotova A.V., Kamyanov V.F. et al. New Oils and Their Use. Metals in Oils. Alma-Ata: Nauka, 1984, p. 448 (in Russian).
- Ponomareva G.A., Pankratyev P.V. The distribution of precious metals in the oil of the western Orenburg Region. Vestnik of the Orenburg State University. 2011. N 5 (124), p. 125-131 (in Russian).
- Woodland S.J., Ottley C.J., Pearson D.G., Swarbrick R.E. Microwave digestion of oils for platinum group and rare earth elements by ICP-MS. Plasma Source Mass Spectrometry: the New Millennium. Cambridge: Royal Society of Chemistry, 2001, p. 17-24. DOI: 10.1039/9781847551696-00017
- Litvinenko V. Foreword: Sixty-year Russian history of Antarctic sub-glacial lake exploration and Arctic natural resource development. Geochemistry. 2020. Vol. 80. Iss. 3. N 125652. DOI: 10.1016/j.chemer.2020.125652
- Litvinenko V.S., Leitchenkov G.L., Vasiliev N.I. Anticipated sub-bottom geology of Lake Vostok and technological approaches considered for sampling. Geochemistry. 2020. Vol. 80. N 3. DOI: 10.1016/j.chemer.2019.125556
- Gupta J.G.S. Determination of trace and ultra-trace amounts of noble metals in geological and related materials by graphite-furnace atomic-absorption spectrometry after separation by ion-exchange or co-precipitation with tellurium. Talanta. 1989. Vol. 36. Iss. 6, p. 651-656. DOI: 10.1016/0039-9140(89)80257-7
- Fedorov Yu.N., Ivanov K.S., Erokhin Yu.V., Ronkin Yu.L. Inorganic geochemistry of the oil of west Siberia: First ICP-MS data. Doklady Earth Sciences. 2007. Vol. 414. № 4, p. 634-637. DOI: 10.1134/S1028334X07040319
- Yakutseni S.P., Kudriavtseva E.I. Metals in oils and condensates of Poland. Oil and Gas Geology. 1998. N 3, p. 44-46 (in Russian).
- Finlay A.J., Selby D., Osborne M.J. Petroleum source rock identification of United Kingdom Atlantic Margin oil fields and the Western Canadian Oil Sands using Platinum, Palladium, Osmium and Rhenium: Implications for global petroleum systems. Earth and Planetary Science Letters. 2012. Vol. 313-314, p. 95-104. DOI: 10.1016/j.epsl.2011.11.00
- Xiugen Fu, Jian Wang, Yuhong Zeng et al. Geochemistry of platinum group elements in marine oil shale from the Changshe Mountain area (China): Implications for modes of occurrence and origins. International Journal of Coal Geology. 2011. Vol. 86. Iss. 2-3, p. 169-176. DOI: 10.1016/j.coal.2011.01.002
- Natural Bitumens of the USSR (Patterns of Formation and Distribution). Leningrad: Nedra, 1981, p. 195 (in Russian).
- Wedepohl K.H. The composition of the continental crust. Geochimica et Cosmochimica Acta. 1995. Vol. 59. Iss. 7, p. 1217-1233. DOI: 10.1016/0016-7037(95)00038-2
- Cheremisina O., Litvinova T., Sergeev V. et al. Application of the Organic Waste-Based Sorbent for the Purification of Aqueous Solutions. Water. 2021. Vol. 13. Iss. 21. N 3101. DOI: 10.3390/w13213101
- Kudinova A.A., Poltoratckaya M.E., Gabdulkhakov R.R. et al. Parameters influence establishment of the petroleum coke genesis on the structure and properties of a highly porous carbon material obtained by activation of KOH. Journal of Porous Materials. 2022. Vol. 29. Iss. 5, p. 1599-1616. DOI: 10.1007/s10934-022-01287-1
- Gurskaya L.I. Platinum Metal Ore Formation of the Black Shale Type and Criteria for Its Forecasting. Saint Petersburg: VSEGEI, 2000, p. 207 (in Russian).
- Ponomareva G.A., Nikiforov I.A. Regularities of directional distribution of plate and palladium at oil-gas fields of Orenburg region. News of the Higher Institutions. Mining Journal. 2015. N 7, p. 28-34 (in Russian).
- Trofimuk A.A., Molchanov V.I., Paraev V.V. Peculiarities of geodynamic settings of formation of gigantic petroleum deposits. Russian Geology and Geophysics. 1998. Vol. 39. N 5, p. 673-682 (in Russian).
- Skublov S.G., Makeyev A.B., Krasotkina A.O. et al. Isotopic and Geochemical Features of Zircon from the Pizhemskoye Titanium Deposit (Middle Timan) as a Reflection of Hydrothermal Processes. Geochemistry International. 2022. Vol. 60. N 9, p. 809-829. DOI: 10.1134/S0016702922090063
- Novikov D.A., Gordeeva А.О., Chernykh A.V. et al. The Influence of Trap Magmatism on the Geochemical Composition of Brines of Petroliferous Deposits in the Western Areas of the Kureika Syneclise (Siberian Platform). Russian Geology and Geophysics. 2021. Vol. 62. N 6, p. 701-719. DOI: 10.2113/RGG20194079
- Egorov A.S., Bolshakova N.V., Kalinin D.F., Ageev A.S. Deep structure, tectonics and geodynamics of the Sea of Okhotsk region and structures of its folded frame. Journal of Mining Institute. 2022. Vol. 257, p. 703-719. DOI: 10.31897/PMI.2022.63
- Egorov A.S., Prischepa O.M., Nefedov Y.V. et al. Deep Structure, Tectonics and Petroleum Potential of the Western Sector of the Russian Arctic. Journal of Marine Science and Engineering. 2021. Vol. 9. Iss. 3. N 258. DOI: 10.3390/jmse9030258
- Zubkov V.S. Hypotheses of heavy hydrcarbons and bitumen genesis in different-age ophiolites. Lithosphere. 2009. N 1, p. 70-80 (in Russian).
- Ganai A.Kh.M. Petrophysical Studies in the Exploration of Oil and Gas Fields in Eastern Syria. Geofizika. 1995. Vol. 2, p. 47-51 (in Russian).
- Vasilev E.A., Klepikov I.V., Kozlov A.V., Antonov A.V. The Nature of the Elongated Form of Diamond Crystals From Urals Placers. Journal of Mining Institute. 2019. Vol. 239, p. 492-496. DOI: 10.31897/PMI.2019.5.492
- Stern R.J., Johnson P. Continental lithosphere of the Arabian Plate: A geologic, petrologic, and geophysical synthesis. Earth-Science Reviews. 2010. Vol. 101. Iss. 1-2, p. 29-67. DOI: 10.1016/j.earscirev.2010.01.002
- Khain V.E. Regional Geotectonics: North and South America, Antarctica, and Africa. Мoscow: Nedra, 1971, p. 548 (in Russian).
- Xingfen Chen, Yunsheng Zhang, Hui D. et al. Study of melting properties of basalt based on their mineral components. Composites Part B: Engineering. 2017. Vol. 116, p. 53-60. DOI: 10.1016/j.compositesb.2017.02.014
- Borodin L.S. Geochemistry of Major Series of Volcanic Rocks. Мoscow: Nedra, 1981, p. 195 (in Russian).
- Krivolutskaya N., Makvandi S., Gongalsky B. et al. Chemical Characteristics of Ore-Bearing Intrusions and the Origin of PGE–Cu–Ni Mineralization in the Norilsk Area. Minerals. 2021. Vol. 11. Iss. 8. N 819. DOI: 10.3390/min11080819
- Krivolutskaya N.A. Siberian Traps and Pt-Cu-Ni Deposits in the Norilsk Area. Cham: Springer, 2016, p. 364.
- Canhimbue L.S., Talovina I.V. Platinum-metal mineralization of the Norilsk district: history and prospects of research. News of the Ural State Mining University. 2022. Iss. 4 (68), p. 56-63 (in Russian). DOI: 10.21440/2307-2091-2022-4-56-63
- Diсko Mouhamadou B.B., Schekoldin R.A., Dmitrieva T.V., Shapiro A.I. Geological conditions and model of bitumoids formation in the Fresco area of the western sector of the Côte d’Ivoire basin. Neftegazovaya geologiya. Teoriya i praktika. 2022. Vol. 17. N 3, p. 19 (in Russian). DOI: 10.17353/2070-5379/30_2022
- Dicko Mouhamadou B.B., Schekoldin R.A., Dmitrieva T.V. Lithofacial features and formation conditions of the Cenozoic section of the Gran-Lau region – petroleum basin of Cоte-d’Ivoire. Neftegazovaya geologiya. Teoriya i praktika. 2019. Vol. 14. N 2, p. 18 (in Russian). DOI: 10.17353/2070-5379/16_2019
- Litvinenko V.S., Kozlov A.V.The Ural-African Transcontinental Oil and Gas Belt. Journal of Petroleum & Environmental Biotechnology. 2014. Vol. 5. Iss. 2. N 174. DOI: 10.4172/2157-7463.1000174
- Dobretsov N.L. Mantle plumes and their role in the formation of anorogenic granitoids. Russian Geology and Geophysics. 2003. Vol. 44. N 12, p. 1243-1261 (in Russian).
- Krikun N.S., Babenko I.A., Talovina I.V., Duryagina A.M. Geological Structure and Perspectives of Oil and Gas Bearing in Neogene Sediments of the Southern Part of the Kuril Island Arc System. Russian Journal of Earth Sciences. 2024. Vol. 24. N 2. N ES2001 (in Russian). DOI: 10.2205/2024ES000905
- Hinkley T.K., Lamothe P.J., Wilson S.A. et al. Metal emissions from Kilauea, and a suggested revision of the estimated worldwide metal output by quiescent degassing of volcanoes. Earth and Planetary Science Letters. 1999. Vol. 170. Iss. 3, p. 315-325. DOI: 10.1016/S0012-821X(99)00103-X
- Shilo N.A. Ore genesis physics of Witwatersrand (SAR). Bulletin of Perm University. Geology. 2008. Iss. 10 (26), p. 62-73 (in Russian).
- Barrier E., Machhour L., Blaizot M. Chapter 11: Petroleum Systems of Syria. Petroleum Systems of the Tethyan Region: AAPG Memoir 106. Tulsa: American Association of Petroleum Geologists, 2014, p. 335-378. DOI: 10.1036/13431862M1063612