Rationale for a possibility of using humic preparations production waste for wastewater purification from metals (Cd2+, Zn2+, Mg2+, Cu2+) aimed at developing efficient measures on environmental rehabilitation
Abstract
Results of studying optimal conditions and parameters for afterpurification of underspoil waters from metal ions using humic acids production waste are presented with a view to develop the efficient measures on environmental rehabilitation of ecosystems disturbed by the development of copper pyrite deposits. The influence of contact time and waste dosage on the purification process was analysed, changes in the pH of wastewater and its impact on the growth and development of plants were studied. The key factors were identified allowing to achieve the efficiency of the purification process – the optimal contact time in the range from 120 to 180 min and waste dosage of 10 g/l. The study showed that the use of waste resulted in a neutral pH value of 7.03 compared to the initial pH value of 5.95. It was ascertained that the use of iron-magnesium production waste in combination with waste from humic preparations production made it possible to achieve the MPC of commercial fishing importance (with the exception of magnesium). Wastewater after the afterpurification process with high magnesium concentrations did not have a stimulating effect on the growth and development of Lepidium sativum L. plants. From biotesting results it can also be stated that there is no negative impact on the growth and development of Lepidium sativum L. The results obtained indicate a potentiality of using afterpurified wastewater for watering plants in the process of initiating the environmental rehabilitation of the disturbed ecosystems.
Fauding The article was prepared under the state assignment N 075-00412-22 PR. Theme 2 (2022-2024). Development of geoinformation technologies for assessing the protection degree of mining areas and forecasting the development of negative processes in mineral resources management (FUWE-2022-0002), N 1021062010532-7-1.5.1.
Introduction
The development of solid mineral deposits in the Ural region, the occurrence of abandoned or sealed-off mining facilities as well as objects of accumulated environmental damage predetermine a complicated environmental situation in the region; therefore, progressing degradation of the natural environment is still a major challenge at present, despite the fact that the environmental factor is gradually becoming one of the fundamental issues in decision making [1, 2].
According to the governmental report on the state and protection of environment in the Sverdlovsk Region, mining industry was the main source of waste generation in 2022. The share of mining waste in generation, disposal and availability at the end of the year was 90.1; 87.4 and 93.2 % of all generated, recycled and accumulated production and consumption waste in the Sverdlovsk Region. Generation of waste from this group increased by 8.147 million tons (5.24 %) compared to 2021 and amounted to 163.6 million tons, mainly due to the growing overburden volume. It should be noted that the share of recycled waste also increased. The amount of recycled mining waste compared to 2021 increased by 2.01 million tons (3.1 %) and reached 67.5 million tons (41.2 % of the generation of this group of waste) due to the use of hard rock overburden for own needs.
Despite a positive dynamics, the presence of large-tonnage waste from the mineral raw materials complex, of both operating and sealed-off production facilities, is one of the reasons for the negative processes occurring in the environment [3, 4]. In 2022, in the Sverdlovsk Region, water of water bodies at 26 % of observation stations was classified as “polluted”, at 57 % as “dirty”, at 13 % as “very dirty”, and at 4 % of stations as “extremely dirty”. The main ingredients from the list of extremely high surface water pollution levels at the stations of the national observation network in the Sverdlovsk Region for 2022 are zinc, copper, and manganese. As regards groundwater quality, cases of exceeding the MPC for cadmium and lead can be recorded.
The relevance of solving the environmental problems of disturbed territories is obvious in connection with accumulation of a huge amount of waste from mining and metallurgical industries stored in sludge and tailings dumps, dumps of overburden rocks, slags and substandard raw materials [5-7]. Therefore, identification of the pattern of mining facilities impact on the deposit environment in respect of studying the processes that determine the accumulation and migration of pollutants, the use of reclamation techniques for soil detoxification, the development of measures on environmental rehabilitation of water bodies with the help of plants and sorption materials produced from natural and technogenic raw materials is an intensely developing field of research [8-10]. A great number of publications illustrate the broad range of materials used for removing metals from wastewater solution including red mud [11-13], ash [14-16], steelmaking slags [17], biochar [18-20], vermiculite [21], and different waste of plant origin [22-24]. Among them, the industrial waste is attracting more and more attention, since it is expected that the use of waste for environmental purposes will be low-cost [25].
The earlier experiments [8] confirmed the possibility of using iron-magnesium production waste to neutralize industrial wastewater from metal ions (Cd2+, Zn2+, Co2+, Cu2+). The dependence of purification efficiency on the dose of waste introduced, contact time, initial concentration of metals and acid-alkaline balance was experimentally determined. Optimal conditions are the 120-180 min contact time, dosage of 4-10 g/l and an initial concentration of metal ions not exceeding 500 mg/l.
The purpose of the work is to assess the possibility of using waste generated in production of humic preparations as a material for afterpurification of wastewater to the MPC level of commercial fishing importance from metal ions (Cu2+, Zn2+, Cd2+, Mg2+) in concentrations obtained as a result of experimental research on redistribution of heavy metals in the “wastewater – waste” system using iron-magnesium production waste [8]. In addition, the task was set to assess the impact of already purified water on the growth and development of agricultural biological plant resources.
Analysis of the initial concentration of metals in solution and pH of solutions was accomplished. The optimal contact time and dosage of materials were determined. An assessment was made of the effect of water following afterpurification on the growth of Lepidium sativum L. watercress plants.
Materials and methods
Waste used in this study was obtained from the production of humic preparations (HPW). As the source raw material for the production of a humic preparation
(potassium humate), the following ratio of components by weight of dry matter is used, %: high-moor peat – 40-60; sapropel (TU 0135-001-94744244-2007) – 25-38; diatomite (TU 5761-001-59266087-2005) – 14-20; KOH – the rest. The main inorganic compounds of peat are nitrogen to 1.5 %, phosphorus, potassium, calcium (in total) to 0.6 % (N:P:K). The content of humic substances in peat is 7.4-7.9 %. Diatomite is by more than 80 % composed of silica.

Fig.1. Humic acids production waste: a – before treatment; b – after treatment
HPW is a pasty substance of dark grey colour which forms as a result of solid phase separation from the target product by centrifugation (Fig.1).
The waste was dried at a temperature of 95 °C and crushed to a fraction of 1 mm. A drying cabinet (ShS-80-01-SPU, OOO PriborUfa, Russia) was used for drying the samples, and laboratory sieves and a porcelain mortar and pestle were used for grinding. Bulk density of the HPW is 400-450 kg/m3, humidity – 3-5 %, pH – 8.0-8.5.
Wastewater is underspoil water from the exhausted copper pyrite deposit Kaban-1 within the boundaries of the Sverdlovsk Region, 190 km from Yekaterinburg. Wastewater was investigated after interaction with iron-magnesium production waste applying the procedure [8], pH = 5.95. Elemental composition, mg/l: Cd2+ – 0.01 (MPC – 0.005); Zn2+ – 0.2 (MPC – 0.01); Mg2+ – 849.60 (MPC – 40); Cu2+ – 0.1 (MPC – 0.001) [8]. The MPC was adopted by Order of the Ministry of Agriculture of Russia dated December 13, 2016 N 552 (as amended on 10.03.2020) “On approval of water quality standards for water bodies of commercial fishing importance including the standards for the maximum permissible concentrations of harmful substances in waters of water bodies of commercial fishing importance”.
Elemental analysis and sample preparation
To ash the samples, a MARS 5 Digestion Microwave System by CEM Corporation (USA) was used in accordance with methodology EPA 3052. The process started with addition of 0.5 g sample to which 9 ml of HNO3 (GOST 11125-84, 70 %) and 3 ml of HF (GOST 10484-78, 45 %) were added. Then, the mixture was stirred, after which it was left for 15 min, and the vessels were closed. Special vessels were used recommended by the manufacturer – EasyPrep. The time required to heat to 180 °C was 6 min, the time to maintain the temperature was 10 min, and the power was 1,800 W. After treatment with dilution to 50 ml, a transparent sample without colour and foreign particles was obtained. 1 M HNO3 was used to determine the mobile forms of metal.
Air-acetylene flame atomic absorption spectroscopy (AAS) (Varian AA 240 FS by Varian Australia Pty Ltd. Australia) was applied to determine metal concentrations in wastewater, mineralized samples and leachates. Specific wavelengths were used for different elements: Cd – 228.8; Zn – 213.9; Mg – 202.6; Cu – 324.7 nm. The detection limits of elements in solution were: Cd – 1.5; Zn – 1.6; Cu – 1.2; Mg – 0.3 µg/l.
Experiments
Redistribution of metals in the “wastewater – HPW” system
The effect of HPW dosage on the degree of metal ions removal from solution was investigated. In the experiments, samples of 0.1; 0.2; 0.5; 1.0; 1.5 and 2.0 g were weighed and placed in conical falcon tubes. Wastewater in a volume of 50 ml was poured to the samples. Then, the samples were mixed (at a frequency
of 99 rpm) for 120 min using a rotary mixer ELMI RM-1L manufactured by ELMI LTD (Latvia). Afterwards, the resulting solutions underwent filtration using “Blue ribbon” filters.
Determination of the optimal contact time for removing metal ions from solution
Wastewater in a volume of 50 ml was poured to 0.2 g weighed quantities. Samples were mixed at different time intervals from 5 to 180 min. The resulting solutions underwent filtration using “Blue ribbon” filters.
To assess the influence of the initial concentration of metal ions Cu2+, Zn2+, Cd2+, Mg2+ on wastewater purification process, solutions with different concentrations were prepared: 5, 10, 50, 100, 300, 500 and 1,000 mg/l. The solutions were prepared using test-grade “high purity” reagents and the state standard samples (with concentrations from 1 to 10 g/l). The experiment was conducted with contact time of 120 min, a 0.2 g weighed quantity was applied, the solution volume was 50 ml, at room temperature. In the experiment, the static exchange capacity of waste (SEC) and the degree of pollutant recovery from the solution (E) were determined, and the standard error of the mean value was calculated.
Determination of static exchange capacity and degree of pollutant recovery from solution:
where Ci is the initial concentration of ions in solution, mg/l; Ce, the equilibrium (residual) concentration of copper ions in leachate determined in water after mixing water and substrate, mg/l; V – volume of model solution added to waste, l; m, mass of dry weighed quantity of the substrate, g.
Biotesting of wastewater
The seed germination experiment was performed according to the procedure in SanPin 2.1.7.573-96. Watercress Lepidium sativum L. seeds (30 pcs.) were placed evenly on filter paper in a Petri dish. Then, 5 ml of wastewater obtained after interaction with waste was poured into each dish. As control – wastewater from the Kaban-1 deposit with initial elemental composition (concentrations before purification using iron-magnesium production waste: Cd2+ – 0.20 (MPC = 0.005); Zn2+ – 50.12 (MPC – 0.01); Mg2+ – 322.50 (MPC – 40); Cu2+ – 78.10 (MPC – 0.001); Fe3+ – 147.71 (MPC – 0.1); Co2+ – 1.73 (MPC – 0.01); Ca2+ – 33.20 (MPC – 180); K+ – 5.26
(MPC – 50) and distilled water [8]. Dishes were covered with lids and left for 72 h at room temperature. The experiment was repeated three times. Root length and germination power of seeds as well as the contents of the investigated chemical elements in individual plants were measured on completing the experiment. Before the chemical analysis, the seeds were washed with distilled water. The seeds were considered germinated if their length was at least 2 mm. Based on these data, the indicators of relative seed germination power (RGP) and relative root growth (RRG) were obtained:
where Gs – is the number of germinated seeds in the sample; and Gc – the number of germinated seeds in control (distilled water);
Ls – root length of germinated seeds in the sample; Lc – root length of germinated seed in control (distilled water).

Fig.2. Effect of initial concentration of metal ions in solution on the HPW wastewater purification process (monometallic solutions)
Discussion of results
To confirm the possibility of using the HPW as a material for afterpurification of wastewater from metal ions, the influence of initial concentration of metals in solution, contact time between the material and the solution, dosage of the HPW and acid-alkaline state of the aqueous medium (pH) were studied. Analysis of the influence degree of each factor helps to prove the efficiency and potential of the HPW for wastewater purification from metals [26-28]. The results obtained can serve as a rationale for the use of production waste as a material for purification and afterpurification of industrial wastewater in terms of developing the efficient measures on environmental rehabilitation of water bodies.
Influence of initial concentration of metal ions on wastewater purification
Initial concentration of metal ions controls the removal of metal ions from aqueous solution. This allows evaluating the influence of metal concentration in solution on the interaction processes and to understand, what concentrations are most important for an efficient removal from solutions [29]. The dependence of the static exchange capacity of the HPW with respect to the metals under study (Cu2+, Zn2+, Cd2+, Mg2+) relative to their initial concentration in monosolution is shown in Fig.2. State standard samples were used to dilute solutions from 5 to 1,000 mg/l.
The graph in Fig.2 shows that the processes of removal of metals such as copper, cadmium, magnesium, and zinc have a certain dynamics. The initial stage (from 5 to 50 mg/l) is characterized by a rapid recovery of metals (Cu2+, Zn2+), which points to a high formation rate of metal compounds with waste [30, 31]. After this stage, there is a period (50-200 mg/l) of a marked decrease for Cu2+, Zn2+. As a result (from 500 mg/l), the equilibrium sets in when the absorption rate of metals and their removal becomes approximately the same, which signals the completion of this process and the attainment of a certain stability of the system. The process of magnesium and cadmium absorption by the HPW is increasing with growing initial concentration of these elements in solution, which is shown in Fig.2 by a concave curve.
Therefore, it can be stated that it is impossible to use the HPW for purification of wastewater containing high concentrations of the elements under study (Cu2+, Zn2+ more than 50 mg/l); however, they can be used at the final stages for bringing the quality of purified water to the MPC level of commercial fishing importance.
The mechanism for removing elements from wastewater can be described in several stages. At the first stage, metal ions move from solution to the outer surface of waste. This process corresponds to film diffusion, when metal ions move from solution surface to the waste surface. At the second stage, metal ions penetrate into the waste structure. This process involves movement of metal ions through internal channels or pores of the material, which can lead to their deeper fixation inside the material [32, 33]. All this plays the key role in understanding the processes of metal removal as well as for the development of efficient water purification technologies in terms of introducing measures on environmental rehabilitation of water bodies including the elimination of discharge of highly mineralized water into them.
Effect of contact time and dosage
The study of the process of ion recovery from solution included a change in the waste mass from 0.1 to 2 g in 50 ml of solution with constant concentration. This process was performed at a constant stirring speed of 99 rpm and lasted 120 min at room temperature. To evaluate the recovery of metal ions from wastewater, underspoil water of the Kaban-1 deposit treated with iron-magnesium production waste was used as model solution [8]. The results are shown in Table 1 and Fig.3.
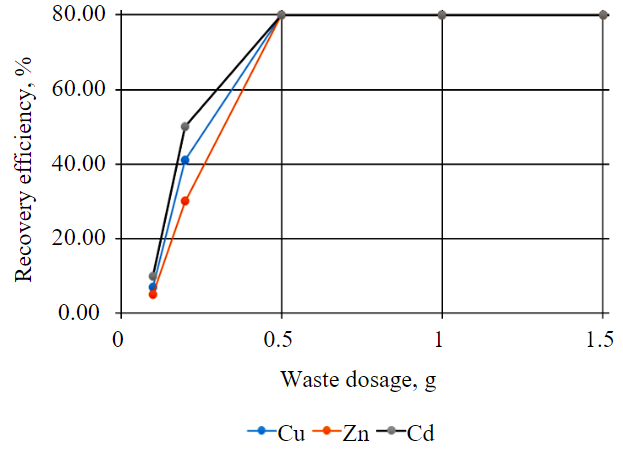
Fig.3. Influence of waste dosage on degree of metal ions recovery from solution
Table 1
Results of chemical analysis of obtained leachates (50 ml wastewater, contact time 120 min)
Weighed quantity, g |
Content of chemical elements in leachate, mg/l |
pH |
|||
Cd2+ |
Zn2+ |
Mg2+ |
Cu2+ |
||
0.1 |
0.007±0.001 |
0.15±0.02 |
953.14±55.16 |
0.073±0.01 |
6.25±0.01 |
0.2 |
0.003±0.001 |
0.1±0.02 |
857.11±47.21 |
0.039±0.01 |
6.78±0.01 |
0.5 |
< 0.0015 |
< 0.0016 |
931.10±45.24 |
< 0.0012 |
7.03±0.01 |
1.0 |
< 0.0015 |
< 0.0016 |
932.05±43.42 |
< 0.0012 |
6.99±0.01 |
1.5 |
< 0.0015 |
< 0.0016 |
946.41±40.56 |
< 0.0012 |
6.9±0.01 |
2.0 |
< 0.0015 |
< 0.0016 |
992.94±44.15 |
< 0.0012 |
6.78±0.01 |
The results of the study showed that the efficiency of removing these metals with the use of the HPW increased with its growing dosage, until almost complete purification (100 %) was achieved. This suggests that a higher dosage of the HPW favoured the increasing number of active sites for metal binding, which, in turn, increased the overall efficiency of the removal process [34-36]. Further, the ability of the HPW to remove copper, cadmium and zinc from solution does not change with increasing dosage. Considering the presence of silica in the source raw material for the production of potassium humate (diatomite), virtually insoluble compounds form as a result of the HPW interaction with wastewater.
At the next stage of research, the influence of duration of contact between the HPW and wastewater on the efficiency of metal ions removal was determined, which makes it possible to verify the optimal exposure time to the HPW for purifying wastewater from the investigated elements to the MPC level.
Table 2
Results of chemical analysis of obtained leachates (50 ml of wastewater, 0.2 g weighed quantity)
Contact time, min |
Content of chemical elements in leachate, mg/l |
рН |
|||
Cd2+ |
Zn2+ |
Mg2+ |
Cu2+ |
||
5 |
0.009±0.001 |
0.18±0.03 |
995.45±52.14 |
0.08±0.02 |
6.05±0.01 |
10 |
0.007±0.001 |
0.17±0.02 |
994.51±54.21 |
0.07±0.02 |
6.11±0.01 |
15 |
0.006±0.001 |
0.15±0.02 |
854.25±56.12 |
0.05±0.02 |
6.25±0.01 |
30 |
0.005±0.001 |
0.12±0.02 |
966.32±50.11 |
0.05±0.02 |
6.52±0.01 |
60 |
0.005±0.001 |
0.11±0.01 |
854.55±56.87 |
0.04±0.01 |
6.70±0.01 |
120 |
0.003±0.001 |
0.10±0.02 |
857.11±47.21 |
0.03±0.01 |
6.78±0.01 |
180 |
0.003±0.001 |
0.10±0.02 |
905.84±67.14 |
0.04±0.01 |
6.82±0.01 |
An experimental study of the effect of contact time on wastewater afterpurification process was conducted at constant solution concentration (Table 2) within different time intervals from 5 to 180 min at room temperature (Fig.4).

Fig.4. Effect of contact time on recovery rate of metal ions from solution
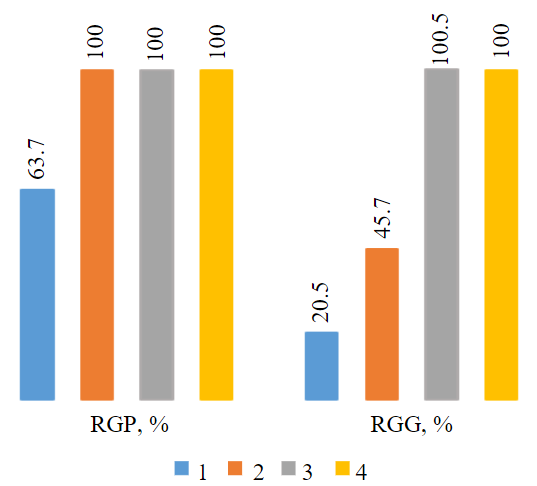
Fig.5. Effect of different wastewater compositions (1-4 in Table 4) on germination power (RGP) and root growth (RGG) of watercress Lepidium sativum L.
Elemental composition of leachates obtained as a result of the HPW interaction with wastewater allows evaluating changes in concentration of different elements in leachates depending on contact time, which is important for understanding the dynamics of the process and determination of the optimal conditions for wastewater purification to the MPC level (Table 2). Residual concentration of metals in leachates after interaction at these contact time conditions varied from
0.009 to 0.003 mg/l for cadmium, from 0.18 to 0.10 mg/l for zinc, from 995.45 to 854.55 mg/l for magnesium, and from 0.08 to 0.04 mg/l for copper.
It can be concluded that in the time interval from 120 to 180 min. the maximum values of the efficiency indicator for copper, cadmium and zinc are recorded; further increase of the interaction time of solutions with the HPW will not lead to major changes in the investigated indicator. It should be noted that magnesium values in the resulting leachates were higher than the initial magnesium values in wastewater. This indicates that the process of removing magnesium ions from polymetallic wastewater is not as efficient as in artificially created model solutions from the SSS, which indicates the need for further research related to formation of a cascade of biological ponds considering the material composition of the bed and filtration dam sensitive to this chemical element.
Further, to assess the risk of secondary pollution when using the HPW, the chemical analysis of the samples obtained after interaction with wastewater was accomplished. Concentrations of metals in the material before the start of the experiment are given (Table 3).
Table 3
Results of chemical analysis of HPW after interaction with wastewater
Element |
Gross forms, mg/kg (before interaction) |
Gross forms, mg/kg (after interaction) |
Mobile, mg/kg (after interaction) |
MPC, mg/kg* |
|
Cu |
0.81±010 |
9.68±1.58 |
1.53±0.25 |
33/3 |
|
Zn |
4.38±0.98 |
21.75±4.58 |
4.25±0.89 |
55/23 |
|
Cd |
< 0.0015 |
< 0.0015 |
< 0.0015 |
0.5/Undetermined |
|
Co |
0.20±0.05 |
4.35±1.25 |
0.98±0.10 |
–/5.0 |
|
Mg |
1,504.31±354.44 |
1,305.40±299.45 |
602.93±154.45 |
Undetermined |
|
Mn |
21.27±3.47 |
22.03±3.33 |
4.03±0.78 |
1,500/700 |
|
K |
36,540.38±3,548.21 |
3,628.68±454.68 |
1,245.39±248.45 |
Undetermined |
|
Fe |
2,485.93±384.49 |
2,490.38±335.24 |
1,300.58±224.87 |
Undetermined |
* SanPiN 1.2.3685-21 “Hygienic standards and requirements for ensuring the safety and (or) harmlessness of environmental factors to humans”.
Initially, the HPW contains a large amount of magnesium – 1,504.31 mg/kg; potassium – 36,540.38 mg/kg; manganese – 21.27 mg/kg and iron – 2,485.93 mg/kg. At the end of experiment,
a decrease in gross forms of magnesium to 1,305.40 mg/kg, potassium to 3,628.68 mg/kg was recorded, iron and manganese remained at the same values. At the same time, there is a significant accumulation of copper from 0.81 to 9.68 mg/kg; zinc – from 4.38 to 21.75 mg/kg, cobalt – from 0.20 to 4.35 mg/kg.
Experiments on afterpurification of pre-treated underspoil water from the Kaban-1 deposit, when determining the optimal conditions and time parameters for the purification processes, indicate the range within which the process reaches its highest efficiency – from 120 to 180 min, at material dosage 10 g/l (0.5 g weighed quantity).
Biotesting of wastewater
Despite a major excess of the MPC of commercial fishing importance for magnesium, in this study, a biotesting technique was applied for in-process monitoring of acute toxicity of samples including the use of watercress as a test object. This method is based on the study of behavioural response of plants to exposure to wastewater. Response of plants (seed germination rate, root system development, and total biomass) can serve as an indicator of the negative impact of various pollutants on the ecosystem.
One of the main advantages of using watercress as a test object is a relatively short duration of experiments due to the rapid cycle of plant growth and deve-lopment [37, 38].
- Seeds of Lepidium sativum L. were germinated in Petri dishes (Fig.5) on filter paper within 72 h with addition of the investigated aqueous solutions:
- wastewater from the Kaban-1 deposit (untreated);
- wastewater from the Kaban-1 deposit (after interaction with iron-magnesium production waste) [8];
- wastewater from the Kaban-1 deposit (after interaction with iron-magnesium production waste and the HPW);
- distilled water.
Table 4
Influence of different wastewater compositions on germination power and growth of watercress, elemental analysis of individual plants after interaction
Element |
Untreated wastewater |
Wastewater after interaction with iron-magnesium Waste dosage – 10 g/l, |
Wastewater after interaction with iron-magnesium Waste dosage – 10 g/l, |
Distilled water |
1 |
2 |
3 |
4 |
|
Number of germinated seeds, pcs. |
19.00±2.00 |
30.00±0.00 |
30.00±0.00 |
30.00±0.00 |
Root length, mm |
4.30±4.00 |
9.64±2.65 |
21.11±6.00 |
21.06±1.53 |
Total biomass, mg |
15.00±2.89 |
27.15±4.04 |
40.09±4.36 |
45.66±1.15 |
RGP, % (germination percentage) |
63.33±2.00 |
100.00±0.00 |
100.00±0.00 |
100.00±0.00 |
RGG, % (relative root length) |
20.50±4.00 |
45.74±2.65 |
100.50±6.00 |
100.00±1.53 |
Cu, mg/kg |
662.67±30.00 |
16.80±1.33 |
8.75±1.02 |
8.40±1.11 |
Zn, mg/kg |
1,478.40±218.04 |
174.32±6.56 |
53.10±3.11 |
45.12±1.85 |
Cd, mg/kg |
1.87±0.46 |
1.00±0.27 |
< 0.0015 |
< 0.0015 |
Fe, mg/kg |
1,442.47±117.90 |
234.80±33.50 |
206.15±25.51 |
246.40±29.42 |
Mg, mg/kg |
2,042.13±211.00 |
15,064.00±667.18 |
11,956.00±468.01 |
1,996.00±127.42 |
K, mg/kg |
< 0.0008 |
13,108.00±452.28 |
15,419.50±709.10 |
13,704.00±394.22 |
pH |
2.33 |
5.95 |
7.05 |
6.51 |
Experimental studies revealed that Lepidium sativum L. seeds exposed to untreated wastewater from the Kaban-1 deposit displayed a major decrease in seed germination power, total biomass and root system development compared to control values (Table 4, Fig.5).
It was found that seed germination decreased by more than 30 %, and total biomass by 65 %, indicating a deterioration in seed viability under the influence of this wastewater. Development of the plant root system was 80 % less compared to control values. These data indicate the negative impact of wastewater on plant development, which is a serious signal of its potential toxicity and impact on living systems.
The study showed that compositions 2 and 3 containing wastewater taken after interaction with waste, demonstrated a high efficiency compared to composition 1 containing untreated wastewater. The number of germinated seeds in these solutions was similar to the control sample and amounted to 100 %.
In plants germinated in composition 2 (containing iron-magnesium production waste 10 g/l), relative length of the root system was 45.74 %, which is 2.2 times greater than in plants from composition 1. However, this length of roots was 2.1 times less than that of plants from composition 3 which included water prepared first using iron-magnesium production waste, and then waste from humic acids production.
When analysing the elemental composition of plants, it is important to note a decrease in the content of all studied metals in samples 2 and 3 compared to untreated wastewater, with the exception of magnesium and potassium. This indicates a potential impact of wastewater treatment process using waste on reducing concentrations of different metals in plants. It is potassium and magnesium that play an important role in vital activity of plants, especially in conditions of technogenically altered or polluted ecosystems. These elements are macronutrients and are necessary for plants in relatively large amounts compared to micronutrients [39, 40].
Potassium plays the key role in the process of photosynthesis, activates many enzymes, participates in regulation of plant water balance, and also affects plant resistance to stress conditions such as drought and frost. On the other hand, magnesium is no less important for plants, since it plays an important role in photosynthesis and chlorophyll synthesis, participates in metabolic processes, in the absorption and transport of phosphorus, which is important for ensuring plant growth and development [39, 40].
In conditions of anthropogenic influence on the environment, where plants can encounter unfavourable conditions, sufficient levels of potassium and magnesium in soil and their availability become critical for ensuring normal growth and development, and, therefore, is essential for the renewal and maintenance balance in such ecosystems, although it should be clarified that purified wastewater does not have any stimulating effect on Lepidium sativum L. plants [39, 40].
Also, iron is one of the most important and indispensable micronutrients for the plants. Insolubility of iron salts and difficulty of their transition into a soluble form create problems for the absorption of iron ions by plant roots. Iron is an important element for many reactions and processes in plants, and its deficiency or excess can negatively affect their development and productivity [41]. In wastewater samples from the Kaban-1 deposit (compositions 1 and 2), iron concentration is much higher than in other samples including compositions treated with waste.
It is especially important that cadmium was not found in composition 3, which indicates a possible decrease in cadmium availability for the plants when using the treatment process including iron-magnesium production waste and the HPW.
In the study of chemical composition of germinated watercress seeds, it was found that the plants exposed to untreated wastewater absorbed more micronutrients, such as iron, zinc, copper, and cadmium, compared to other samples. Since most plants have the root type of metal accumulation, the accumulation process, most likely, occurred in the underground part [42-44]. The detected high concentrations of iron, zinc, copper, and cadmium appeared to be toxic for plants, and this affected the characteristics of the germinated seeds, root length and total biomass.
In addition, in samples 1chlorosis was recorded associated with a lack of chlorophyll, which led to a change in colour (yellowing) of plants. Low pH level (2.33) in samples 1 also affected the availability of magnesium and potassium macronutrients which are essential for healthy plant growth [45, 46]. Macronutrients including nitrogen, potassium, calcium, magnesium, and sulfur are known to be better absorbed at pH values between 6.0 and 6.5. Micronutrients become less available to plants at higher alkaline pH values exceeding 7.0 [45, 46].
Potassium and magnesium micronutrients, being antagonists, compete with each other in the processes of assimilation of nutrients by plants [47]. In samples with low pH level plants do not receive enough essential macronutrients, such as potassium and magnesium, but accumulate an excess of iron, zinc, cadmium, and copper micronutrients. This imbalance has a negative effect on plant growth and development.
Therefore, maintaining the optimal soil pH level is the key to preventing chlorosis and ensuring healthy plant growth. The results obtained indicate a possibility of using wastewater purified by a complex of iron-magnesium production waste and the HPW of wastewater for irrigation of biological plant resources when initiating the processes of ecological rehabilitation of ecosystems disturbed by development of copper pyrite deposits.
Conclusion
In this study, the optimal conditions were identified for the efficient use of the HPW in afterpurification processes of pre-treated underspoil water of the Kaban-1 deposit from copper, cadmium, and zinc ions. The most efficient parameters include the following:
- Contact time ranging from 120 to 180 min, indicating the importance of prolonged exposure to attain the maximum efficiency of the purification process. Long contact time allows intensifying the interaction between waste and wastewater, which promotes a more complete removal of metal ions from solution.
- Waste dosage of 10 g/l indicates the optimal amount of waste to achieve the desired purification effect. This parameter affects the degree of interaction between waste and solution as well as the overall efficiency of the process.
Using waste, a neutral pH value (7.03) was attained compared to the initial value (5.95). However, waste appeared to be inefficient for the afterpurification of natural wastewater from magnesium ions, and the process of releasing Mg2+ into solution was also recorded. Purified wastewater, despite a significant amount of magnesium, does not have a negative effect on the growth and development of Lepidium sativum L. plants; however, no stimulating effect was recorded.
References
- Makarov A.B., Talalai A.G., Guman O.M., Khasanova G.G. Anthropogenic deposits and their impact on the natural environment. Minerals and Mining Engineering. 2022. N 3, p. 120-129 (in Russian). DOI: 10.21440/0536-1028-2022-3-120-129
- Sokolov I.V., Antipin Yu.G., Rozhkov A.A., Solomein Yu.M. Environmental geotechnology for low-grade ore mining with the creation of conditions for the concurrent disposal of mining waste. Journal of Mining Institute. 2023. Vol. 260, p. 289-296. DOI: 10.31897/PMI.2023.21
- Litvinova T.E., Suchkov D.V. Comprehensive approach to the utilisation of technogenic waste from the mineral resource complex. Mining Informational and Analytical Bulletin. 2022. N 6-1, p. 331-348 (in Russian). DOI: 10.25018/0236_1493_2022_61_0_331
- Ribnikova L.S., Ribnikov P.A. Processes of hydrosphere self-rehabilitation and mine water treatment in post mining period. Mining Informational and Analytical Bulletin. 2020. N 3-1, p. 488-500 (in Russian). DOI: 10.25018/0236-1493-2020-31-0-488-500
- Makovozova Z.E., Sokolov A.A., Fomenko V.A., Sarbaeva M.T. Influence of hydrogeology at Unal tailings pond on ecosystem pollution with heavy metals. Mining Informational and Analytical Bulletin. 2023. N 6, p. 126-138 (in Russian). DOI: 10.25018/0236_1493_2023_6_0_126
- Semyachkov A.I., Pochechun V.A., Semyachkov K.A. Hydrogeoecological conditions of technogenic groundwater in waste disposal sites. Journal of Mining Institute. 2023. Vol. 260, p. 168-179. DOI: 10.31897/PMI.2023.24
- Mochalova L.A., Sokolova O.G., Podkorytov V.N., Eremeeva O.S. Circulation industry cluster management within the mineral mining and processing sector. Mining Informational and Analytical Bulletin. 2021. № 11-1, p. 374-387 (in Russian). DOI: 10.25018/0236_1493_2021_111_0_374
- Antoninova N.Yu., Sobenin A.V., Usmanov A.I., Shepel K.V. Assessment of the possibility of using iron-magnesium production waste for wastewater treatment from heavy metals (Cd2+, Zn2+, Co2+, Cu2+). Journal of Mining Institute. 2023. Vol. 260, p. 257-265. DOI: 10.31897/PMI.2023.34
- Lyashenko V.I., Vorobiev A.E., Khomenko O.E., Dudar T.V. Development of Technologies and Technical Facilities to Reduce the Environmental Impact in the Area of Influence of the Tailings Dam. Vestnik of Nosov Magnitogorsk State Technical University. 2022. Vol. 20. N 1, p. 25-41 (in Russian). DOI: 10.18503/1995-2732-2022-20-1-25-41
- Pashkevich M.A., Korotaeva A.E., Matveeva V.A. Experimental simulation of a system of swamp biogeocenoses to improve the efficiency of quarry water treatment. Journal of Mining Institute. 2023. Vol. 263, p. 785-794.
- Xianshang Bai, Jianwei Lin, Zhibin Zhang, Yanhui Zhan. Immobilization of lead, copper, cadmium, nickel, and zinc in sediment by red mud: adsorption characteristics, mechanism, and effect of dosage on immobilization efficiency. Environmental Science and Pollution Research. 2022. Vol. 29. Iss. 34, p. 51793-51814. DOI: 10.1007/s11356-022-19506-2
- Ang Liu, Yingchen Bai, Chao Wang et al. Study on the removal of iron (II) and manganese (II) in acidic mine drainage by red mud: Performance and mechanism. Environmental Science and Pollution Research. 2023. Vol. 30. Iss. 55, p. 117970-117980. DOI: 10.1007/s11356-023-30378-y
- Fomenko A.I., Sokolov L.I. Use of Ashes from Thermal Power Plants for Effluents Treatment. Ecology and Industry of Russia. 2022. Vol. 26. N 1, p. 14-18 (in Russian). DOI: 10.18412/1816-0395-2022-1-14-18
- Guifang Wang, Jie Xiang, Guangchuan Liang et al. Application of common industrial solid waste in water treatment: a review. Environmental Science and Pollution Research. 2023. Vol. 30. Iss. 52, p. 111766-111801. DOI: 10.1007/s11356-023-30142-2
- Sobenin A.V., Antoninova N.Yu., Usmanov A.I., Shepel K.V. Influence of material composition of biological pond bed on waste water treatment in mining and metallurgy industries. Mining Informational and Analytical Bulletin. 2021. N 5-2, p. 273-282 (in Russian). DOI: 10.25018/0236_1493_2021_52_0_273
- Teran A.I. Kinetics of extraction from water solutions of Fe3+, Cu2+ and Pb2+ ions by filter loading, obtained by blinding plasters. Foundry Production and Metallurgy. 2019. N 4, p. 76-80 (in Russian). DOI: 10.21122/1683-6065-2019-4-76-80
- Sizirici B., Fseha Y.H., Yildiz I. et al. The effect of pyrolysis temperature and feedstock on date palm waste derived biochar to remove single and multi-metals in aqueous solutions. Sustainable Environment Research. 2021. Vol. 31. N 9. DOI: 10.1186/s42834-021-00083-x
- Runjuan Zhou, Ming Zhang, Shuai Shao. Optimization of target biochar for the adsorption of target heavy metal ion. Scientific reports. 2022. Vol. 12. N 13662. DOI: 10.1038/s41598-022-17901-w
- Madzin Z., Zahidi I., Raghunandan M.E., Talei A. Potential application of spent mushroom compost (SMC) biochar as low-cost filtration media in heavy metal removal from abandoned mining water: a review. International Journal of Environmental Science and Technology. 2023. Vol. 20. Iss. 6, p. 6989-7006. DOI: 10.1007/s13762-022-04617-7
- Bakhireva O.I., Sokolova M.M., Mankovskaya O.Yu., Pan L.S. Obtaining biosorbent based on vermiculite for additional purification of waste water from nickel ions. PNRPU Bulletin. Chemical Technology and Biotechnology. 2021. N 4, p. 5-15 (in Russian). DOI: 10.15593/2224-9400/2021.4.01
- Mosendz I.A., Kremenetskaya I.P., Drogobuzhskaya S.V., Alekseeva S.A. Sorption of heavy metals by the filtering containers with serpentine materials. Vestnik of MSTU. 2020. Vol. 23. N 2, p. 182-189 (in Russian). DOI: 10.21443/1560-9278-2020-23-2-182-189
- Afolabi F.O., Musonge P., Bakare B.F. Bio-sorption of a bi-solute system of copper and lead ions onto banana peels: characterization and optimization. Journal of Environmental Health Science and Engineering. 2021. Vol. 19. Iss. 1, p. 613-624. DOI: 10.1007/s40201-021-00632-x
- Rahman D.Z., Vijayaraghavan J., Thivya J. A comprehensive review on zinc(II) sequestration from wastewater using various natural/modified low-cost agro-waste sorbents. Biomass Conversion and Biorefinery. 2023. Vol. 13. Iss. 7, p. 5469-5499. DOI: 10.1007/s13399-021-01822-1
- Duwiejuah A.B., Amadu Y., Gameli B.H.R. et al. Spent Chinese Green Tea as an Adsorbent for Simultaneous Removal of Potentially Toxic Metals from Aqueous Solution // Chemistry Africa. 2022. Vol. 5. Iss. 6, p. 2107-2114. DOI: 10.1007/s42250-022-00459-5
- Ahmed M., Mavukkandy M.O., Giwa A. et al. Recent developments in hazardous pollutants removal from wastewater and water reuse within a circular economy. Clean Water. 2022. Vol. 5. N 12. DOI: 10.1038/s41545-022-00154-5
- Thakur A., Sharma N., Singh J. Synthesis of copper oxide (CuO) nanoparticles for the efficient removal of fluoride from an aqueous solution. Journal of Radioanalytical and Nuclear Chemistry. 2024. Vol. 333. Iss. 6, p. 2857-2865. DOI: 10.1007/s10967-023-09066-1
- Barragán-Peña P., Macedo-Miranda M.G., Olguin M.T. Cadmium removal from wastewater in a fixed-bed column system with modified-natural clinoptilolite-rich tuff. Chemical Papers. 2021. Vol. 75. Iss. 2, p. 485-491. DOI: 10.1007/s11696-020-01314-y
- Chang Y.S., Au P.I., Mubarak N.M. et al. Adsorption of Cu(II) and Ni(II) ions from wastewater onto bentonite and bentonite/GO composite. Environmental Science and Pollution Research. 2020. Vol. 27. Iss. 26, p. 33270-33296. DOI: 10.1007/s11356-020-09423-7
- Wong S., Ghafar N.A., Ngadi N. et al. Effective removal of anionic textile dyes using adsorbent synthesized from coffee waste. Scientific reports. 2020. Vol. 10. N 2928. DOI: 10.1038/s41598-020-60021-6
- Khadem M., Husni Ibrahim A., Mokashi I. et al. Removal of heavy metals from wastewater using low-cost biochar prepared from jackfruit seed waste. Biomass Conversion and Biorefinery. 2023. Vol. 13. Iss. 16, p. 14447-14456. DOI: 10.1007/s13399-022-02748-y
- Mladenović N., Kljajević L., Nenadović S. et al. The Applications of New Inorganic Polymer for Adsorption Cadmium from Waste Water. Journal of Inorganic and Organometallic Polymers and Materials. 2020. Vol. 30. Iss. 2, p. 554-563. DOI: 10.1007/s10904-019-01215-y
- Ambaye T.G., Vaccari M., van Hullebusch E.D. et al. Mechanisms and adsorption capacities of biochar for the removal of organic and inorganic pollutants from industrial wastewater. International Journal of Environmental Science and Technology. 2021. Vol. 18. Iss. 10, p. 3273-3294. DOI: 10.1007/s13762-020-03060-w
- Mathabatha T.I.K., Matheri A.N., Belaid M. Peanut Shell-Derived Biochar as a Low-Cost Adsorbent to Extract Cadmium, Chromium, Lead, Copper, and Zinc (Heavy Metals) from Wastewater: Circular Economy Approach. Circular Economy and Sustainability. 2023. Vol. 3. Iss. 2, p. 1045-1064. DOI: 10.1007/s43615-022-00207-4
- Filippov D.V., Fufaeva V.A., Shepelev M.V. Sorption of Heavy-Metal Ions from Aqueous Solutions by Mesoporous Nickel 2-Ethylimidazolate. Russian Journal of Inorganic Chemistry. 2022. Vol. 67. N 3, p. 375-381. DOI: 10.1134/S0036023622030081
- Faisal A.A.H., Nassir Z.S., Rashid H.M. et al. Neural network for modeling the capture of lead and cadmium ions from wastewater using date palm stones. International Journal of Environmental Science and Technology. 2022. Vol. 19. Iss. 11, p. 10563-10576. DOI: 10.1007/s13762-021-03883-1
- Saranya S., Gandhi A.D., Suriyakala G. et al. A biotechnological approach of Pb(II) sequestration from synthetic wastewater using floral wastes. SN Applied Sciences. 2020. Vol. 2. Iss. 8. N 1357. DOI: 10.1007/s42452-020-3172-7
- Elguera J.C.T., Barrientos E.Y., Wrobel K., Wrobel K. Effect of cadmium (Cd(II)), selenium (Se(IV)) and their mixtures on phenolic compounds and antioxidant capacity in Lepidium sativum. Acta Physiologiae Plantarum. 2013. Vol. 35. Iss. 2, p. 431-441. DOI: 10.1007/s11738-012-1086-8
- Bożym M., Król A., Mizerna K. Leachate and contact test with Lepidium sativum L. to assess the phytotoxicity of waste. International Journal of Environmental Science and Technology. 2021. Vol. 18. Iss. 7, p. 1975-1990. DOI: 10.1007/s13762-020-02980-x
- Zhurova V.G., Svetlichnaya M.S. Study of the influence of potassium, calcium and magnesium ions on the growth and development of plants. Dostizheniya nauki i obrazovaniya. 2018. N 14 (36), p. 13-15 (in Russian).
- Akanova N.I., Kozlova A.V., Mukhina M.T. Magnesium role in plant nutrition system. Agrochemical Herald. 2021. N 6, p. 66-72 (in Russian). DOI: 10.24412/1029-2551-2021-6-014
- Ivanishchev V.V. Availability of iron in the soil and its influence the plant growth and development. News of the Tula state university. Natural sciences. 2019. Iss. 3, p. 127-138 (in Russian).
- Riseh R.S., Vazvani M.G., Hajabdollahi N., Thakur V.K. Bioremediation of Heavy Metals by Rhizobacteria. Applied Biochemistry and Biotechnology. 2023. Vol. 195. Iss. 8, p. 4689-4711. DOI: 10.1007/s12010-022-04177-z
- Puhalsky Y.V., Loskutov S.I., Vorobyov N.I. et al. Impact of Heavy Metals on Changes in the Biochemical Profile of Pea Root Exometabolites. Russian Agricultural Sciences. 2023. Vol. 49. N 2, p. 189-201. DOI: 10.3103/S106836742302009X
- El-Moustaqim K., Tallou A., Mabrouki J. et al. Phytoremediation Processes for the Removal of Heavy Metals / Integrated Solutions for Smart and Sustainable Environmental Conservation. Cham: Springer, 2024, p. 33-46. DOI: 10.1007/978-3-031-55787-3_3
- Ferrarezi R.S., Lin X., Gonzalez Neira A.C. et al. Substrate pH Influences the Nutrient Absorption and Rhizosphere Microbiome of Huanglongbing-Affected Grapefruit Plants. Frontiers in Plant Science. 2022. Vol. 13. N 856937. DOI: 10.3389/fpls.2022.856937
- Çalişkan B., Çalişkan A.C. Potassium Nutrition in Plants and Its Interactions with Other Nutrients in Hydroponic Culture / Improvement of Quality in Fruits and Vegetables Through Hydroponic Nutrient Management. InTechOpen, 2018, p. 9-21. DOI: 10.5772/intechopen.71951
- Kailiu Xie, Ismail Cakmak, Shiyu Wang et al. Synergistic and antagonistic interactions between potassium and magnesium in higher plants. The Crop Journal. 2020. Vol. 9. Iss. 2, p. 249-256. DOI: 10.1016/j.cj.2020.10.005