Finite element analysis of slope failure in Ouenza open-pit iron mine, NE Algeria: causes and lessons for stability control
- 1 — Postgraduate Student University of Bejaia, Faculty of Technology, Mining and Geology Department ▪ Orcid
- 2 — Ph.D., Dr.Sci. Deputy Head of Department University of Bejaia, Faculty of Technology, Mining and Geology Department ▪ Orcid ▪ Scopus ▪ ResearcherID
- 3 — Ph.D., Dr.Sci. Professor University of Bejaia, Faculty of Technology, Mining and Geology Department ▪ Orcid
- 4 — Ph.D., Dr.Sci. Professor University of Bejaia, Faculty of Technology, Laboratory of Construction Engineering and Architecture (LGCA) ▪ Orcid
Abstract
Slope failures in mining engineering pose significant risks to slope stability control, necessitating a thorough investigation into their root causes. This paper focuses on a back analysis of a slope failure in the Zerga section of the Ouenza – Algeria open-pit iron mine. The primary objectives are to identify the causes of slope failure, propose preventive measures, and suggest techniques to enhance stability, thereby providing crucial insights for monitoring slope stability during mining operations. The study commenced with a reconstruction of the slopes in the affected zones, followed by a numerical analysis utilizing the Shear strength reduction method within the Finite element method (SSR-FE). This approach enables the examination of slope stability under both static and dynamic loads. The dynamic load assessment incorporated an evaluation of the vibrations induced by the blasting process during excavation, introducing seismic loading into the finite element analysis. The findings reveal that the primary triggering factor for the landslide was the vibration generated by the blasting process. Furthermore, the slope stability was found to be critically compromised under static loads, highlighting a failure to adhere to exploitation operation norms. The challenging geology, particularly the presence of marl layers where maximum shear strain occurs, contributed to the formation of the landslide surface. The study not only identifies the causes of slope failure but also provides valuable lessons for effective slope stability management in mining operations.
Introduction
Slope failures in open-pit mines, common occurrences during exploitation, carry substantial implications for safety and economics, depending on their location and extent [1-3]. To design stable slopes, a comprehensive geotechnical investigation is imperative [4-6]. Typically, slope stability is evaluated by determining the safety factor, a ratio of passive to active forces acting on the slope. A safety factor above one indicates stability, but uncertainties may lead to instability even with a value above one [7-10]. Open pit slope design guidelines highlight critical conditions with safety factors below 1.3 and 1.1 for static and dynamic loads, respectively [11].
A thorough understanding of the geological, hydrogeological, seismological, and geotechnical aspects of a site, along with awareness of adapted methods, is essential for effective slope stability investigation [12-14]. Among numerical methods, the Finite element method (FEM) is widely used in stability analysis [15]. While the accuracy of FEM results relies on selecting appropriate geotechnical models and parameters [16-18], its advantage lies in minimal assumptions about slip surfaces and internal force distribution [19-21].
In mining engineering, controlling slope stability necessitates a deeper understanding of potential threats, often achieved through the analysis of past slope failure events [22-25]. Back-analysis, involving the reconstruction of observed failures, is a common practice during the production stage when signs of instability emerge [26]. Sakurai's work [27] emphasizes the use of sensitivity analysis in 2D slope stability software for back-analysis. This method identifies the material property exerting the most significant influence on slope stability [22]. The initial step to understanding natural or human-induced landslides is to accurately determine the geometry of the failure surface [28-31].
Our study aims to conduct a back analysis on a slope failure in the Zerga section of the Ouenza – Algeria open-pit iron mine, identifying causes and proposing preventive techniques. Initial steps involve reconstructing the slope in affected zones, followed by a numerical analysis using Shear strength reduction by Finite element method (SSR-FE) through Phase2 Finite element software developed by Rocscience Inc*. The SSR-FE method facilitates slope stability examination under static and dynamic conditions, including the impact of vibrations from blasting through seismic loading analysis. This approach enhances our understanding of slope failure mechanisms and contributes to improved stability control in mining operations.
Methods
Numerical methods are an effective and robust method for solving a wide range of engineering problems, with the FEM being one of these effective methods. Our study employed the software Phase2 to conduct these analyses. We obtained the safety factor (SF) using the shear strength reduction by Finite element (SSR-FE) method. The SSR-FE has gained significant attention thanks to the advancements in computer technology. When the slope reaches the failure state using SSR-FE, an unlimited plastic flow will be produced by the displacement on the sliding surface [32-34]. Many works demonstrated the efficiency of the SSR-FE method in determining the safety factor of sloping soils and rock masses [35-38], the use of this method is vast and adopted in several research studies [39-41]. The SSR-FE method is well-suited for analyzing complex slope conditions and provides insights into displacement, stress, and water pressure details.
In the SSR-FE method, the parameters of shear strength of a rock C or soil material φ are reduced by one reduction factor F*. Thus, new parameters (C* and φ*) can be obtained and are reduced by another reduction factor again. Until the slope is in the limit equilibrium state. At this moment, the corresponding reduction factor is termed as Strength Reduction Factor (SRF). In other words, this method involves successive reductions (by some trial factors) in the shear strength of slope materials (C and φ) until instability is induced in the slope. The SSR-FE method usually assumes the Mohr – Coulomb strength for slope materials. This failure criterion is commonly used because it is linear, which greatly speeds up calculation times. The equations for this method are as follows:
where τ, C and φ are shear stress, cohesion and internal friction angle of slope materials before reduction, respectively; τ*, C* and φ*areshear stress, cohesion and internal friction angle of slope materials after reduction, respectively; s is normal stress of slope materials; F* is the reduction factor.
Another important feature when doing an SSR-FE analysis is the ability to plot maximum deformation versus SRF, which can automatically define the failure surface. Also, we can determine the effect of seismic vibrations on slope stability by adding seismic coefficients in the finite element method. The seismic coefficients represent the maximum vibration acceleration as a fraction of the acceleration due to gravity. When it is defined, an additional body force will be applied to each finite element in the mesh. The vibrations produced by the blasting process are a combination of horizontal and vertical vibrations with a specific acceleration.
Methodology
The methodology adapted to analyze the landslide that happened in the iron open pit mine of Ouenza, Algeria, takes the following steps. First, we reconstruct the slopes of the two zones where the sliding occurred. Next, a geotechnical analysis is performed using the SSR-FE method. This slope stability analysis is done in the static and dynamic loads. The dynamic load in our study case is represented by the effect of the vibrations generated by the blasting process on the slope stability; its impact is estimated by including a seismic loading in the finite element analysis.
Stability Analysis. The site backgrounds
The Zerga site is formed by a hill called Koudiat Ez-Zerga, located about 3.5 km southwest of the city center of Ouenza. It is administratively linked to the municipality of Ouenza, province of Tebessa (Fig.1). The Zerga site is the last part of the mineralized axis of the Ouenza iron mine deposit, its structure is simple, and it consists of a monocline of Aptian limestone, some levels of which have been replaced by iron ore. Outcrops of marl in continuity with this monocline are found on this site. The Aptian limestone is intersected by vein mineralization, mainly fluorite and barite. A geological reserves estimated 3196457 t still to exploit with an iron percentage (content) of 42.7 % [42, 43].
The exploitation of the Zerga site started in 1974. For several years, in the structures, successive landslides have been occurring, especially in the zone located above the northern part of the ore body. The studies on this subject make it possible to mention some significant slides in this mine: The first visible manifestations date back to the end of 1978 in the central part of the site. Another landslide that occurred in 1985 reached the northwestern part of the first bench in the surrounding rocks. This landslide reached the northeastern part of the second bench in 1986 and 1987. This mine recently suffered another landslide in 2012. The two distinct zones of the landslides will be the subject of our study.
The zone 1 is located in the northern part of the Zerga site. The landslides in this area extend from the 826 m level to the 731 m level (Fig.2, a), and the zone 2 is located in the western part of the site. The movement in this part started at the upper level of 865 m to reach a level of 755 m (Fig.2, b). The overall sliding surface is estimated at approximately 30000 m2.
The presence and behavior of groundwater in the Ouenza iron mine area play a pivotal role in determining the stability of slopes within this site. While the various formations in the deposit generally lack significant underground aquifers, the specific characteristics of each geological layer significantly influence the movement and availability of groundwater.
The Triassic diapiric layer, serving as a local impermeable stratum, hinders the circulation of water, making it challenging for groundwater to permeate. In contrast, the massive limestone formations, though exhibiting high fissuration when exposed, allow for the infiltration of groundwater, albeit with a relatively slow recharge rate. The marl series, on the other hand, presents impermeable qualities, causing rainwater to run off or evaporate, except in the presence of thin limestone intercalations, which are usually of limited extent.
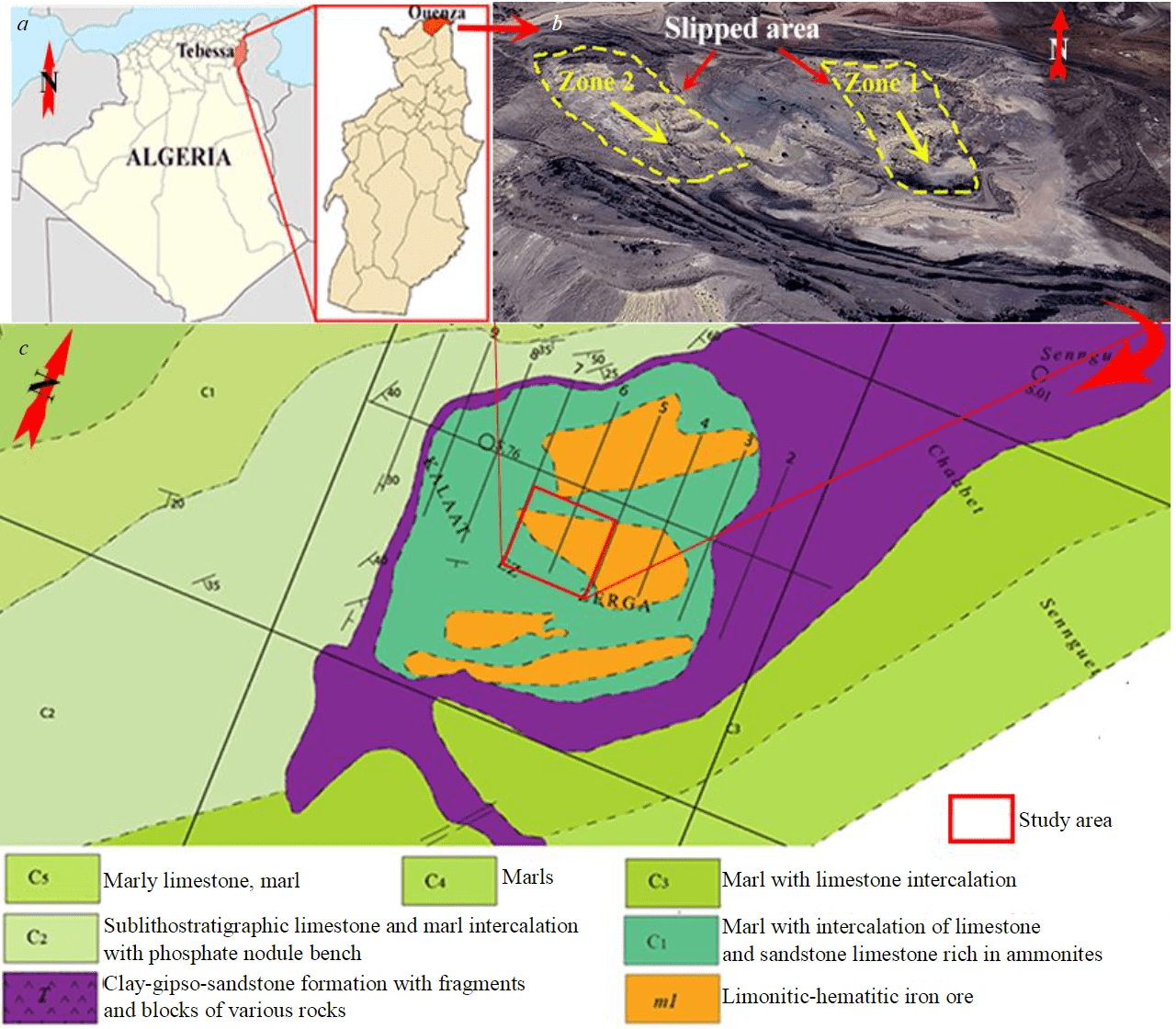
Fig.1. Location map (a), slipped surface (b), and geological area (c) of the Zerga-Ouenza iron mine
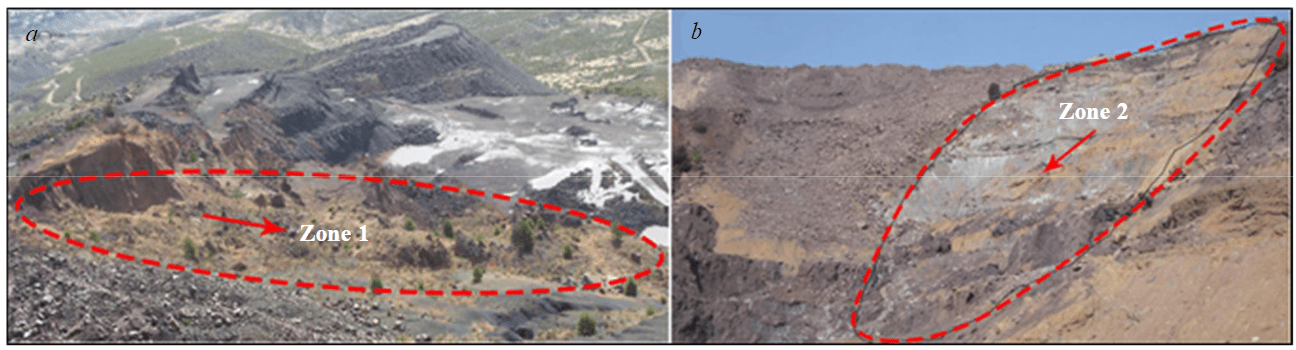
Fig.2. Geological section of the studied area after sliding: zone 1 (a) and zone 2 (b)
In a region with an average annual rainfall of around 400 mm, the hydrogeological dynamics are crucial to consider. Moreover, the elevated water stress situation in the Ouenza region underscores the significance of understanding and managing groundwater, with the city of Ouenza relying on water transfers from the Ain Dalia reservoir, located approximately 60 km to the north, to fulfill its drinking water requirements. These factors collectively underscore the essential role of groundwater in shaping slope stability within the Ouenza iron mine, making it a vital consideration in both mining and environmental management.
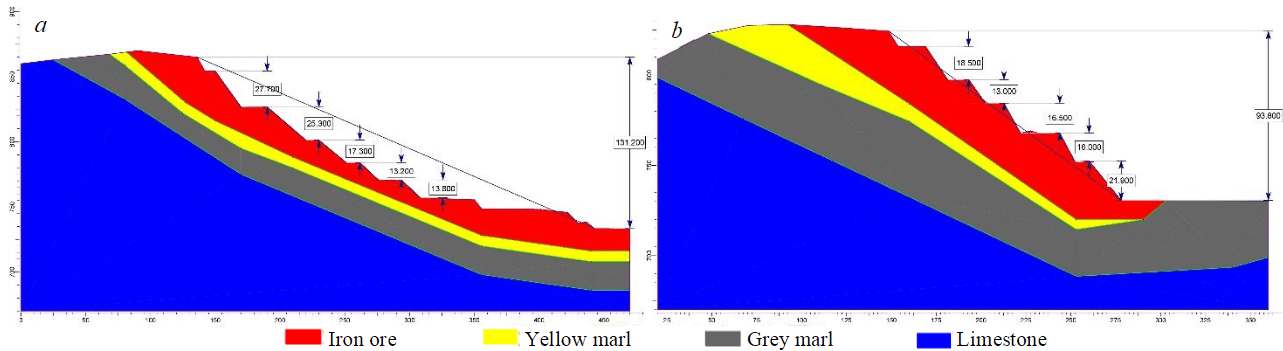
Fig.3. Geotechnical models for zone 2 (а) and zone 1 (b) in the Zerga-Ouenza
Observation of the landslide area and Geotechnical model
Based on the direct observations of the landslide, analyzing the layers in the geological section of the studied area (Fig.2), and examining its structural composition along with documentation communicated and research findings, the characteristics of the failure surface can be outlined as follows:
- the terrain affected by the landslide consists mainly of the exploited iron ore layer mixed with deposits of marl (yellow and grey);
- mobilization of a significant volume of land and pairing of benches are observed in two zones;
- the existence at the base of the iron ore layer of a Pelitic and clay formation of the lower Thanetian represents a weak resistance compared to the iron ore layer;
- the presence of tectonic deformation surfaces in marl and limestone.
Based on those above, it can be inferred that the sliding surface is situated at the marl layer's level and that its shape follows the topography thereof.
Fig.3 represents the numerical model that integrates the topographic and geological data. Based on the topographic and boreholes data, including direct observation of the site, the geological formation of landslide areas in the Zerga deposit was constructed from four main layers, iron ore layer, yellow and grey marl layers, and limestone layer.
In this paper, the selected rock slope was modeled as a continuum material using a two-dimensional, plane strain approach, based on a Mohr – Coulomb elastic-plastic formulation that is dilation upon yield. The numerical analyses were carried out using a finite element formulation provided in the Phase2. Shear strength reduction or SSR method is used to find out the factor of safety of slopes. Table 1 presents the rock mass properties used in the analysis. Roller boundary conditions are assumed along the lateral sides of the model such that no displacement is allowed in the lateral direction. At the base of the numerical model, the boundary is fixed such that no movement is allowed in either direction.
Table 1
Geotechnical parameters of soils
Material |
Unit compressive strength σcs,МPа |
Dry unit weight γd, kN/m3 |
Young’s modulus of rock mass Erm, МPа |
Cohesion C, МPа |
Internal friction angle φ, deg |
Dilatancy ψ, deg |
Poisson ratioν |
Iron ore |
14.53 |
29.33 |
1822 |
0.199 |
25.9 |
0 |
0.3 |
Limestone |
34.36 |
26.09 |
6548.8 |
0.332 |
30.7 |
0.7 |
0.3 |
Yellow marl |
13.11 |
24.71 |
784.9 |
0.166 |
21.6 |
0 |
0.28 |
Grey marl |
11.47 |
25.95 |
677.1 |
0.128 |
18.9 |
0 |
0.28 |
Reflection on the possible causes of the sliding
Effectively managing slope stability in mining engineering requires a thorough understanding of potential triggers for landslides. These triggers encompass a range of factors, including hydrological and hydrogeological conditions, geometric parameters such as slope height and dip angle, and dynamic effects resulting from earthquakes or blasting processes. In our specific case study, hydrological and hydrogeological considerations were not taken into account due to the predominantly arid nature of the Ouenza iron mine area, specifically the Zerga section. The arid classification of the Ouenza iron mine area, particularly in the Zerga section, is supported by the absence of significant aquifers.
Considering dynamic effects from earthquakes and blasting processes, the seismic zoning map, following Algerian seismic regulation RPA99/Version 2003, designates the Tebessa province, including our study area, as seismic zone 1. This classification implies that seismic waves associated with earthquakes in this region are weak and have a negligible role in destabilizing slopes. Therefore, seismic considerations were not considered significant contributors to the slope failure in our studied case.
In contrast, blasting emerges as a significant factor due to its adoption as the primary exploitation method in the Ouenza mine. The use of explosives in blasting generates vibrations that directly impact rock massifs, compromising their integrity and weakening their resistance to compression. Consequently, the potential for triggering a landslide through this mechanism becomes a crucial consideration in our study, emphasizing the importance of assessing and mitigating the effects of blasting on slope stability in mining operations.
Results and Discussions
Stability analysis in the static and dynamic loads
The FEM was used to perform a back analysis on the slope stability in the static state. The slopes were modeled by the Phase2 software (Fig.3). The Mohr – Coulomb failure criterion has been chosen for the analysis, and the geotechnical parameters for each layer are shown in Table 1. The Shear strength reduction technique allows us to determine a slope’s safety factor. In this method, the value of SRF at the point of instability is taken as the safety factor of the slope. First, and for both areas in their natural state, we investigated the stability of the slope before excavation to determine whether the initial rock mass of the relief was stable. Next, we examine the stability of the reconstructed slope after excavation under static and dynamic loading (Fig.4).
The results of natural slope analysis show an SF = 1.88 and SF = 2.05 for the zones 1 and 2, respectively (Fig.4, a). Both values of SF are above 1.3, which means that in the static state, the initial massif rock is stable and safe for the exploitation process of the iron ore in the long term. The reconstructed slope analysis result in the zone 1 static state shows an SF = 1.2, and the maximum shear strain is located in the marl layers beginning from level 828 to level 731 (Fig.4, b). The zone 2 shows an SF = 1.16, and the maximum shear strain is also located in the marl layers forming a circle surface that started from level 868 to 757 (Fig.4, b). From these results, even if both values of SF are over than one and theoretically the slopes of both zones appear stable, the slope stability is considered critical since the SF is less than 1.3 in the static condition, according to John and Peter [11].
We can deduce that the factors responsible for this critical condition are related to the failure to respect the mining standards. First, the concentration of the excavation works on the lower part of the slope led to increasing the total dip of the flank; this puts more pressure on the marl layers that have a low cohesion and friction angle, elevating the chance of forming a probable sliding surface as shown in Fig.3, b. In addition, not respecting the Algerian norms of the bench height that put 15 m as a limit, the bench height of our site reaches some levels of more than 27 m and with an inclination angle that varies from 39 to 62°. Despite that, these factors place the slope stability in a critical condition; they are not the direct causes that triggered the landslide.
Since the slope is in a critical condition in the static state, but the factors that directly triggered the slide are yet to find, an investigation of other influencing factors is carried out. As mentioned before, the vibrations generated from earthquakes and the groundwater factors have no role in triggering the landslide because our site is considered an arid area and non-seismic, but considering the exploitation method adopted in our study site is the drilling and blasting method, the vibrations generated by the blasting are investigated using the finite element method by adding seismic coefficients.
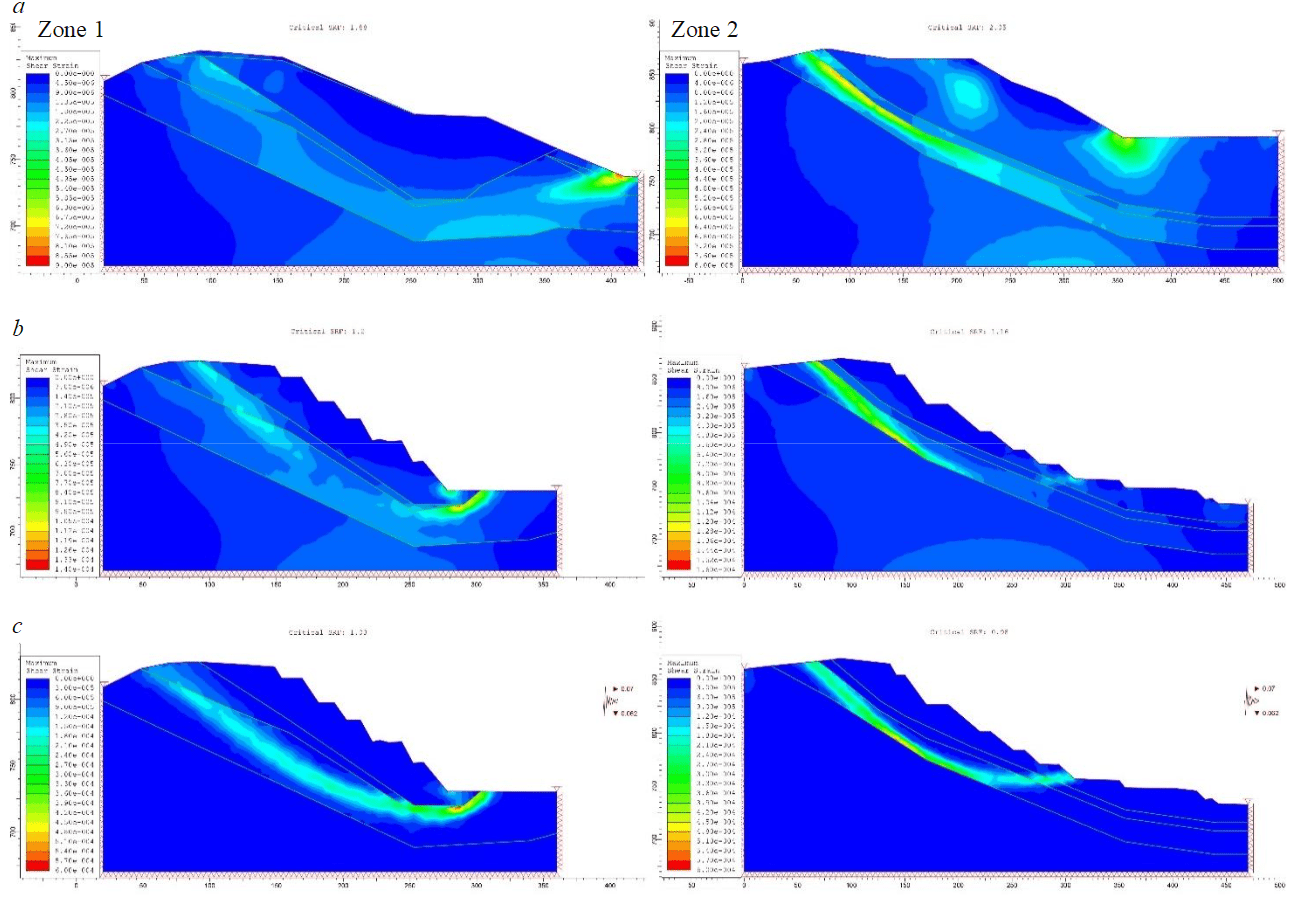
Fig.4. Maximum shear stress and safety factor of the rock mass for geological zones before excavation (a), after excavation, static load (b), and after excavation, dynamic load (c)
The seismic coefficients represent the maximum vibration as a fraction of the acceleration due to gravity. When defined, an additional body force will be applied to each finite element in the mesh. The vibration waves produced by the blasting process combine horizontal and vertical waves. According to the velocity and frequency of waves measurement performed using a seismograph in our site, the values of the horizontal and vertical acceleration vary between 0.02 to 0.07g and 0.02 to 0.062g, respectively; for our analysis, we take the highest values to determine the impact rate that can be reached.
The results of the slope stability analysis under the dynamic load show a remarkable decrease in the SF value in both zones. For the zone 1, the SF = 1.03, and the maximum shear strain is located in the marl layers forming the landslide surface beginning from level 828 to level 731 (Fig.4, c). The zone 2 show an SF = 0.98 and the maximum shear strain is also located in the marl layers forming the surface, which started from level 868 to 757 (Fig.4, c).
From the results, SF < 1 for the zone 2 and SF ≈ 1 for the zone 1, which means that the slope is unstable under the dynamic load and the landslide will occur according to the surface formed by the maximum shear strain in the phase model as in Fig.4, c. The vibrations generated by the blasting process are the direct trigging factor of the landslide.
Analyzing the effect of blasting vibration on the safety factor value
Evaluation of the impact of vibration produced by the blasting process on slope stability is very important to understand the degree of damage that can be reached; this evaluation can be done by performing an analysis of the influence of the horizontal and vertical accelerations of the vibration’s waves generated by the blasting process on the safety factor values. Each time in the phase software modelization and for both zones, the horizontal and vertical acceleration is varied in uniform increments between the minimum and maximum values. At the same time, other input parameters are held constant at their main values (Fig.5).
The findings indicate a significant decrease in the safety factor value occurred with the increase in the acceleration of horizontal vibration in both zones. On the other hand, increasing the acceleration of vertical vibration have a low effect in decreasing the value of the safety factor; its impact is almost negligible. We can conclude that the horizontal vibrations generated by the blasting process are the main parameter in the dynamic load analysis that has an important effect on the safety factor and, consequently, the slope stability. From the line graphs, the slope stability will be in a critical condition if the horizontal vibrations value reaches or surpasses 0.044g for the zone 1 and 0.024g for the zone 2.
Solutions that could prevent the landslide
This study indicates that the triggering factor of the landslide is the blasting vibrations produced by the explosive in the blasting process and that its impact on the slope stability can be severe; this leads to an understanding that controlling and good monitoring of the blasting operations is very important to maintain the slope stability. But for our case, the explosive caused the landslide only because the slope was in critical condition in its static state, according to John and Peter in the guidelines of an open pit slope design [11]. As mentioned before, the factors responsible for this critical condition are related to the failure to respect the mining standards by concentrating the excavation works from the bottom to the top of the edge; also, the bench height that has been adapted in the studied case consider critical, reaching up more than 27 m in certain levels. So, in this part of our work and for both zones, we propose an exploitation method of the initial slope with five phases of excavation that respects a sinking direction from top to bottom, including a bench height of 15 m according to the Algerian norms with an inclination angle of 70° as shown in Fig.6.
The slope is analyzed in each phase of the exploitation operation under the dynamic load that triggered the landslide in the first place (Fig.7).
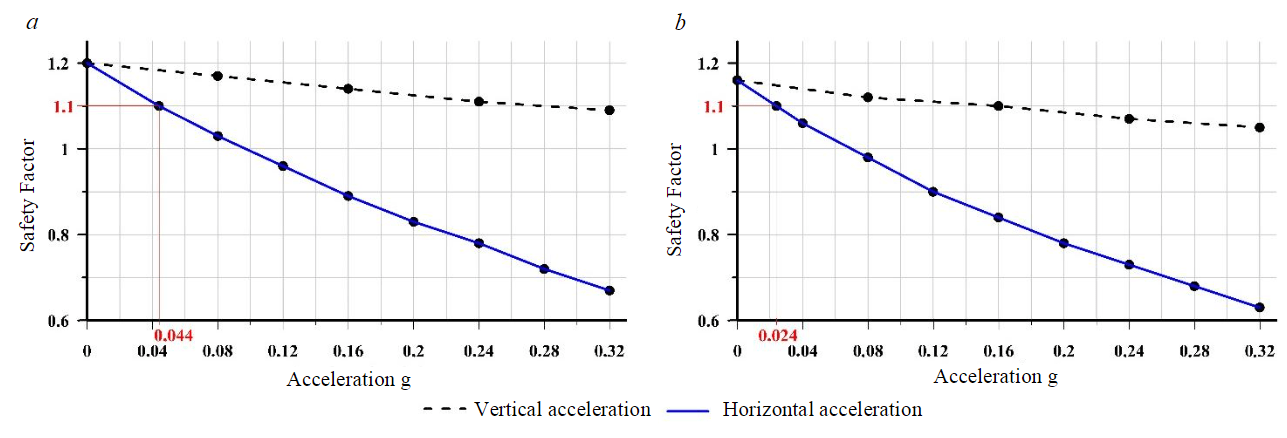
Fig.5. Line graphs of the effect of blasting vibration on the safety factor value: a – zone 1; b – zone 2
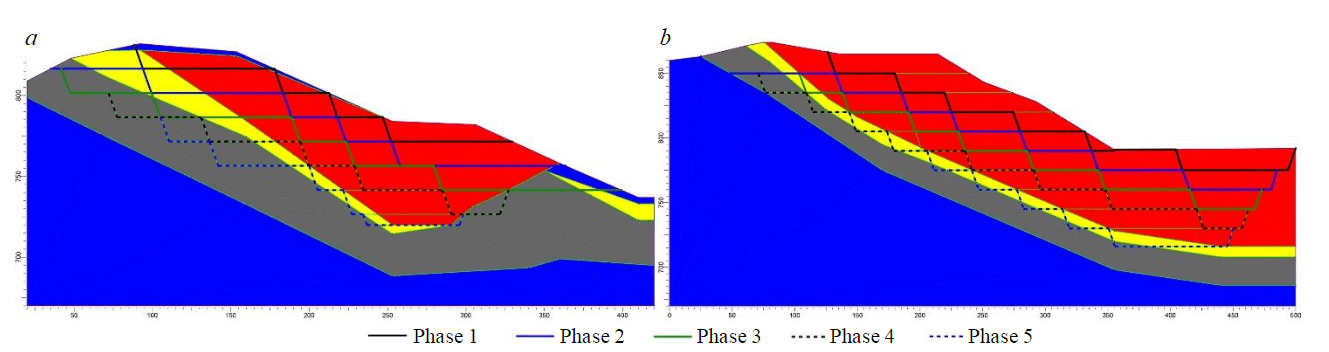
Fig.6. Excavation sequences for geological zones: a – zone 1; b – zone 2
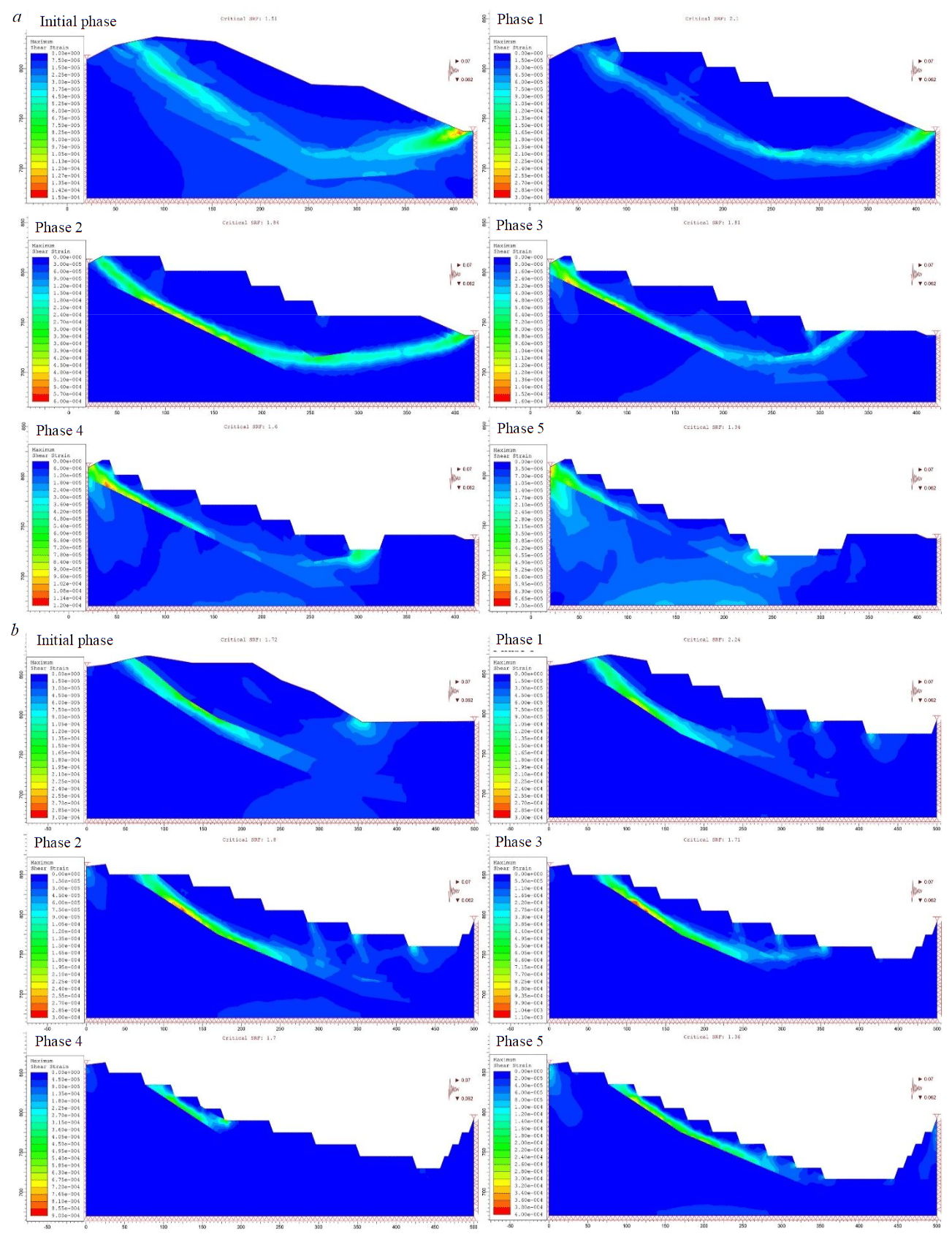
Fig.7. Maximum shear stress and safety factor for each excavation phase in zone 1 (a) and zone 2 (b)
The results of the safety factor calculation for both zones are summarized in Table 2. The initial relief showed a safety factor under the dynamic load of 1.51 for the zone 1 and 1.72 for the zone 2 since these values are more than the minimum admitted under the dynamic load (SF ≥ 1.1), the initial relief is stable in the long term.
Table 2
Safety factors for different phases under dynamic conditions
Zone |
Initial phase |
Phase 1 |
Phase 2 |
Phase 3 |
Phase 4 |
Phase 5 |
Zone 1 |
1.51 |
2.1 |
1.84 |
1.81 |
1.6 |
1.34 |
Zone 2 |
1.72 |
2.24 |
1.8 |
1.71 |
1.7 |
1.36 |
All phases of the exploitation operation presented an SF of 1.34 to 2.24 under the dynamic load for both zones; these values are also way greater than the minimum admitted under the dynamic load. We conclude that this proposed exploitation method ensures the safe mining of the iron ore without any danger of a landslide occurring in the slope until the total extraction of the ore body. Plus, the actual vibration generated by the blasting process is acceptable and presents no danger to slope stability.
Conclusion
This paper undertakes a back analysis utilizing the finite element method to investigate a slope failure in the Zerga section of the Ouenza – Algeria open-pit iron mine. Initial examination of the massif rock's stability before excavation reveals a static SF of 1.88 and 2.05 for the zones 1 and 2 respectively, both exceeding 1.3, indicating stability for long-term ore exploitation. However, subsequent static analysis of the reconstructed slope yields SF values of 1.2 and 1.16 for the zones 1 and 2, respectively, indicating a critical condition as SF falls below 1.3.
Factors contributing to this critical state include deviations from mining norms, particularly the concentration of excavation at the lower slope, elevating total dip, and exerting increased pressure on marl layers. Although bench height exceeds Algerian norms, it did not directly trigger the landslide.
The study then explores the impact of explosive-generated vibrations on slope stability, incorporating seismic coefficients. Results demonstrate a significant SF decrease (1.03 for the zone 1 and 0.98 for the zone 2), indicating instability under dynamic load. Horizontal vibrations notably decrease SF, whereas vertical vibrations have a lesser impact.
The primary trigger for the landslide is identified as blasting vibrations, with severe consequences for slope stability. However, the landslide only occurs due to the pre-existing critical static condition, attributable to non-compliance with mining standards. Analysis of a proposed exploitation method with a top-to-bottom sinking direction indicates SF values between 1.34 and 2.24 under dynamic load, affirming its safety for long-term iron ore mining.
Both direct and indirect factors, including blasting-generated vibrations and non-compliance with exploitation standards, contribute to landslide occurrence. Proper monitoring and control of blasting processes are crucial for slope stability, emphasizing the need for strict adherence to mining operation norms.
To enhance slope stability, it is imperative to strictly adhere to mining standards, focusing on excavation concentration and bench height. Ensure that excavation practices align with recommended norms to mitigate potential destabilizing effects on the slope.
Additionally, implement a comprehensive geotechnical monitoring system for continuous slope stability assessments, enabling the early detection of instability and timely intervention.
Furthermore, effective blasting management practices should be developed and implemented. This includes optimizing explosive usage, monitoring vibration levels, and adopting techniques to minimize the impact of vibrations on slope stability.
Consider adopting the proposed exploitation method with a sinking direction from top to bottom, following recommended bench heights and inclination angles, as it has demonstrated improved safety factors under dynamic loads, proving crucial for preventing future landslides. Looking forward, future research should embrace a holistic approach to slope stability assessments, incorporating geological, hydrological, and geotechnical factors to enhance the accuracy of predictions and preventive measures. Additionally, exploring emerging technologies, establishing long-term monitoring programs, increasing public awareness, fostering collaborative research initiatives, and integrating environmental impact assessments into studies will contribute to sustainable mining practices.
References
- Kolapo P., Oniyide G.O., Said K.O. et al. An Overview of Slope Failure in Mining Operations. Mining. 2022. Vol. 2. Iss. 2, p. 350-384. DOI: 10.3390/mining2020019
- Bednarczyk Z. Slope Stability Analysis for the Design of a New Lignite Open-Pit Mine. Procedia Engineering. 2017. Vol. 191, p. 51-58. DOI: 10.1016/j.proeng.2017.05.153
- Roy N., Kumar A., Rao K.S. Stability Analysis of Failed Slope in Tindharia Region, West Bengal, India – A Case Study. Journal of the Geological Society of India. 2021. Vol. 97. Iss. 8, p. 887-892. DOI: 10.1007/s12594-021-1787-y
- Sarkar S., Pandit K., Dahiya N., Chandna P.Quantified landslide hazard assessment based on finite element slope stability analysis for Uttarkashi–Gangnani Highway in Indian Himalayas. Natural Hazards. 2021. Vol. 106. Iss. 3, p. 1895-1914. DOI: 10.1007/s11069-021-04518-x
- Yaohui Gao, Yan Zhang, Chunchi Ma et al. Failure process and stability analysis of landslides in Southwest China while considering rainfall and supporting conditions. Frontiers in Environmental Science. 2023. Vol. 10. N 1084151. DOI: 10.3389/fenvs.2022.1084151
- Obregon C., Mitri H. Probabilistic approach for open pit bench slope stability analysis – A mine case study. International Journal of Mining Science and Technology. 2019. Vol. 29. Iss. 4, p. 629-640. DOI: 10.1016/j.ijmst.2019.06.017
- Mojtahedi S.F.F., Tabatabaee S., Ghoroqi M. et al. A novel probabilistic simulation approach for forecasting the safety factor of slopes: a case study. Engineering with Computers. 2019. Vol. 35. Iss. 2, p. 637-646. DOI: 10.1007/s00366-018-0623-5
- Shuai Li, Zeming Zhao, Boyi Hu et al. Hazard Classification and Stability Analysis of High and Steep Slopes from Underground to Open-Pit Mining. International Journal of Environmental Research and Public Health. 2022. Vol. 19. Iss. 18. N 11679. DOI: 10.3390/ijerph191811679
- Xiaojie Yang, Zhenli Hao, Gaotong Ma, Gan Li. Research on Slope Stability Evaluation Based on Improved Set Pair Analysis Method: A Case of Tonglvshan Open-Pit Mine. Shock and Vibration. 2021. Vol. 2021. N 6713581. DOI: 10.1155/2021/6713581
- Shiferaw H.M. Study on the influence of slope height and angle on the factor of safety and shape of failure of slopes based on strength reduction method of analysis. Beni-Suef University Journal of Basic and Applied Sciences. 2021. Vol. 10, p. 31. DOI: 10.1186/s43088-021-00115-w
- Wesseloo J., Read J.Acceptance criteria. Guidelines for open pit slope design. CSIRO Publishing, 2009, p. 221-235.
- Chaoqun Hou, Rongjian Zhang, Yongxin Li et al. Comparisons of Safety Factors for Slope in Nonlinear Soils. KSCE Journal of Civil Engineering. 2021. Vol. 25. Iss. 10, p. 3737-3749. DOI: 10.1007/s12205-021-0298-0
- Yiyun Yang. Grey Relational Analysis on Influencing Factors of Highway Slope Safety in Ankang Mountain Area. International Journal of Safety and Security Engineering. 2020. Vol. 10. N 5, p. 721-726. DOI: 10.18280/ijsse.100518
- Yunyong He, Ziyin Cai, Fuming Wang et al.Numerical investigation on slope stability influenced by seismic load and discontinuity with a continuous-discontinuous method. Bulletin of Engineering Geology and the Environment. 2023. Vol. 82. Iss. 3. N 70. DOI: 10.1007/s10064-023-03090-x
- Karthik A.V.R., Manideep R., Chavda J.T. Sensitivity analysis of slope stability using finite element method. Innovative Infrastructure Solutions. 2022. Vol. 7. Iss. 2. N 184. DOI: 10.1007/s41062-022-00782-3
- Stetjuha V.A., Zheleznjak I.I.Methodology for calculating the stability of the polymer operating string in permafrost. Journal of Mining Institute. 2020. Vol. 241, p. 22-28. DOI: 10.31897/PMI.2020.1.22
- Fredj M., Hafsaoui A., Boukarm R. et al. Numerical Modelling of Slope Stability in Open Pit Phosphate Mines, Algeria: A Comparative Study. IOP Conference Series: Earth and Environmental Science. 2019. Vol. 221. N 012020. DOI: 10.1088/1755-1315/221/1/012020
- Rybak J., Khayrutdinov M.M., Kuziev D.A. et al. Prediction of the geomechanical state of the rock mass when mining salt deposits with stowing. Journal of Mining Institute. 2022. Vol. 253, p. 61-70. DOI: 10.31897/PMI.2022.2
- Weijun Cen, Jiarui Luo, Jishun Yu, M. Shamin Rahman. Slope Stability Analysis Using Genetic Simulated Annealing Algorithm in Conjunction with Finite Element Method. KSCE Journal of Civil Engineering. 2020.Vol. 24. Iss. 1, p. 30-37. DOI: 10.1007/s12205-020-2051-5
- Koca T.K., Koca M.Y. Comparative analyses of finite element and limit-equilibrium methods for heavily fractured rock slopes. Journal of Earth System Science. 2020. Vol. 129. Iss. 1. N 49. DOI: 10.1007/s12040-019-1314-3
- Baryakh A.A., Devyatkov S.Yu., Denkevich E.T.Mathematical modelling of displacement during the potash ores mining by longwall faces. Journal of Mining Institute. 2023. Vol. 259, p. 13-20. DOI: 10.31897/PMI.2023.11
- Fredj M., Hafsaoui A., Riheb H. et al. Back-Analysis study on slope instability in an open pit mine (Algeria). Naukovyi visnyk Natsionalnoho hirnychoho universytetu. 2020. N 2, p. 24-29. DOI: 10.33271/nvngu/2020-2/024
- Gadri L., Hadji R., Zahri F. et al. The quarries edges stability in opencast mines: a case study of the Jebel Onk phosphate mine, NE Algeria. Arabian Journal of Geosciences. 2015. Vol. 8. Iss. 11, p. 8987-8997. DOI: 10.1007/s12517-015-1887-3
- Fredj M., Hafsaoui A., Khadri Y., Boukarm R. Influence of the failure surface choice on the safety factor value during slope stability studies. Naukovyi visnyk Natsionalnoho hirnychoho universytetu. 2018. N 3, p. 3035. DOI: 10.29202/nvngu/2018-3/3
- Wei-Hai Yuan, Kang Liu, Wei Zhang et al.Dynamic modeling of large deformation slope failure using smoothed particle finite element method. Landslides. 2020. Vol. 17. Iss. 7, p. 1591-1603. DOI: 10.1007/s10346-020-01375-w
- Ng S.M., Ismail M.A.M., Abustan I. Back Analysis of Slope Failure Using Finite Element with Point Estimate Method (FEM-PEM). Journal of Civil Engineering Research. 2014. Vol. 4. N 3A, p. 3135. DOI: 10.5923/c.jce.201402.04
- Sakurai S. Back Analysis in Rock Engineering. London: CRC Press, 2017, p. 240. DOI: 10.1201/9781315375168
- Xuesong Chu, Liang Li, Yung-ming Cheng.Risk Assessment of Slope Failure Using Assumption of Maximum Area of Sliding Mass and Factor of Safety Equal to Unit. Advances in Civil Engineering. 2019. Vol. 2019. N 6268079. DOI: 10.1155/2019/6268079
- Saadoun A., Hafsaoui A., Khadri Y., Fredj M. Numerical modelling of slope stability in Chouf Amar limestone quarry (M’sila, Algeria). Naukovyi visnyk Natsionalnoho hirnychoho universytetu. 2018. N 5, p. 2429. DOI: 10.29202/nvngu/2018-5/3
- Qi Li, Yimin Wang, Kunbiao Zhang. Failure Mechanism of Weak Rock Slopes considering Hydrological Conditions. KSCE Journal of Civil Engineering. 2022. Vol. 26. Iss. 2, p. 685-702. DOI: 10.1007/s12205-021-1198-z
- Benyoucef A.A., Gadri L., Hadji R. et al. Mining operations and geotechnical issues in deep hard rock mining – case of Boukhadra iron mine. Geomatics, Land management and Landscape. 2022. N 4, p. 27-46. DOI: 10.15576/GLL/2022.4.27
- Saadoun A., Yilmaz I., Hafsaoui A. et al. Slope Stability Study in Quarries by Different Approaches: Case Chouf Amar Quarry, Algeria. IOP Conference Series: Materials Science and Engineering. 2020. Vol. 960. N 4. N 042026. DOI: 10.1088/1757-899X/960/4/042026
- Abdellah W.R., Hussein M.Y., Imbabi S.S. Rock slope stability analysis using shear strength reduction technique (SSRT) – case histories. Mining of Mineral Deposits. 2020. Vol. 14. Iss. 2, p. 16-24. DOI: 10.33271/mining14.02.016
- Sysala S., Hrubesova E., Michalec Z., Tschuchnigg F. A rigorous variant of the shear strength reduction method and its geotechical applications. 16th International Conference on Computational Plasticity (COMPLAS 2021), 7-10 September 2021, Barcelona, Spain. Scipedia, 2022. Vol. CT14 – Geomechanics, p. 8. DOI: 10.23967/complas.2021.007
- Fredj M., Boukarm R., Saadoun A., Nakache R. Slopes stability studies excavated in a rock masses. Case of Ahmed El-Hadj quarry (Lafarge). 23rd International Multidisciplinary Scientific GeoConference SGEM 2023, 3-9 July 2023, Albena, Bulgaria. STEF92 Technology. 2023. Vol. 23. Iss. 1.1, p. 233-242. DOI: 10.5593/sgem2023/1.1/s02.28
- 36. Nie Zhibao, Zhang Zhihong, Zheng Hong. Slope stability analysis using convergent strength reduction method. Engineering Analysis with Boundary Elements. 2019. Vol. 108, p. 402-410. DOI: 10.1016/j.enganabound.2019.09.003
- Hussain G., Singh Y., Bhat G.M. et al. Geotechnical Characterisation and Finite Element Analysis of Two Landslides along the National Highway 1-A (Ladakh Region, Jammu and Kashmir). Journal of the Geological Society of India. 2019. Vol. 94. Iss. 1, p. 93-99. DOI: 10.1007/s12594-019-1272-z
- Yong Hong, Zhushan Shao, Guangbin Shi, Jiabao Liu. Stability and Countermeasures for a Deposit Slope with Artificial Scarp: Numerical Analysis and Field Monitoring. Advances in Civil Engineering. 2020. Vol. 2020. N 8822080. DOI: 10.1155/2020/8822080
- Jiang Zhao, Xiangrui Duan, Lina Ma et al. Importance sampling for system reliability analysis of soil slopes based on shear strength reduction. Georisk: Assessment and Management of Risk for Engineered Systems and Geohazards. 2021. Vol. 15. Iss. 4, p. 287-298. DOI: 10.1080/17499518.2020.1780618
- Tianlong Zhang, Peng Zeng, Rafael Jimenez et al. System reliability analysis of soil slopes using shear strength reduction and active-learning surrogate models. Arabian Journal of Geosciences. 2022. Vol. 15. Iss. 6. N 470. DOI: 10.1007/s12517-022-09718-8
- Aziz K., Sarkar S., Sahu P.Geo-investigation and Slope Stability Analysis of Debris Slides along Ramban-Gool Road Network, Jammu and Kashmir, India. Journal of the Geological Society of India. 2023. Vol. 99. Iss. 7, p. 986-994. DOI: 10.1007/s12594-023-2419-5
- Zerzour O., Gadri L., Hadji et al. Geostatistics-Based Method for Irregular Mineral Resource Estimation, in Ouenza Iron Mine, Northeastern Algeria. Geotechnical and Geological Engineering. 2021. Vol. 39. Iss. 5, p. 3337-3346. DOI: 10.1007/s10706-021-01695-1
- Zerzour O., Gadri L., Hadji R. et al. Semi-variograms and kriging techniques in iron ore reserve categorization: application at Jebel Wenza deposit. Arabian Journal of Geosciences. 2020. Vol. 13. Iss. 16. N 820. DOI: 10.1007/s12517-020-05858-x