New data on the composition of growth medium of fibrous diamonds from the placers of the Western Urals
- 1 — Junior Researcher Zavaritsky Institute of Geology and Geochemistry, Ural Branch of the RAS ▪ Orcid ▪ Scopus ▪ ResearcherID
- 2 — Ph.D., Dr.Sci. Director Zavaritsky Institute of Geology and Geochemistry, Ural Branch of the RAS ▪ Orcid ▪ Scopus
- 3 — Ph.D., Dr.Sci. Leading Researcher Saint Petersburg Mining University ▪ Orcid ▪ Scopus
- 4 — Ph.D., Dr.Sci. Leading Researcher Zavaritsky Institute of Geology and Geochemistry, Ural Branch of the RAS ▪ Orcid ▪ Elibrary ▪ Scopus
Abstract
This article presents the results of studying microinclusions of fluids/melts in diamonds from the placers of the Krasnovishersky District (western slope of the Middle/Northern Urals), which make it possible to establish the evolution of diamond-forming media in the subcontinental lithospheric mantle of the eastern margin of the East European craton. Impurity composition of the studied crystals reveals three different types of diamonds, the formation of which was associated with separated metasomatic events. Microinclusions in B-type diamonds containing A and B nitrogen defects reflect an older metasomatic stage characterized by the leading role of silicic and low-Mg carbonatitic fluids/melts. The second stage is associated with the growth of A-type diamonds containing nitrogen exclusively in the form of A-centers. At this stage, the formation of diamonds was related with low-Mg carbonatitic media, more enriched in MgO, CaO, CO2, and Na2O compared to B-type diamonds. The third stage probably preceded the eruption of the transporting mantle melt and led to the formation of C-type diamond containing A and C nitrogen defect centers and microinclusions of silicic to low-Mg carbonatitic composition. The recorded trend in the evolution of diamond-forming fluids/melts is directed towards more carbonatitic compositions. Fluids/melts are probably sourced from eclogitic and pyroxenitic mantle substrates.
Introduction
Mantle fluids/melts play an important role in the evolution of the subcontinental lithospheric mantle (SCLM), as they are involved in the mass transfer between different mantle domains and affect their composition, rheology, and oxidation state [1, 2]. The interaction of such fluids/melts with mantle rocks leads to the enrichment of primary rock-forming minerals with incompatible elements [3–5], as well as to the formation of secondary minerals containing volatiles [6, 7]. Modern concepts suggest that the formation of diamonds is associated with metasomatic events occurring with the active participation of deep fluids/melts interacting with mantle eclogites and peridotites [8–10]. The unique physical and chemical resistance of diamond to external processes determines the exceptional safety of captured material, which provides the important information about the composition, thermodynamic conditions and evolution of the Earth's mantle. Mineral inclusions and nitrogen aggregation state indicate that the vast majority of diamonds (> 90%) were formed in the subcontinental lithospheric mantle at the base of cratons, and only less than 5% are of superdeep (sublithospheric) origin [10–12]. The growth of some types of fibrous diamonds (cuboids and coated diamonds) with a low nitrogen aggregation state is usually associated with the formation of a proto-kimberlite melt. [9, 13, 14]. Such diamonds often contain microinclusions of daughter multiphase associations of diamond-forming high-density fluid (HDF), the properties of which are similar to volatile-rich melts [9]. Microinclusions in fibrous diamonds reflect the composition of the diamond-forming medium that existed in the subcontinental lithospheric mantle, which makes it possible to reconstruct the composition of deep fluids/melts responsible for mantle metasomatism, the formation of diamonds, and their subsequent ascent to the surface by kimberlites.
The Ural fold belt, which marks the geographic boundary between Europe and Asia, was formed in the Late Paleozoic as a result of the formation of the Pangea supercontinent [15]. The Urals is one of the key mining regions in Russia. Diamond-bearing placers of the western slope of the Middle and Northern Urals have been known since 1829, when the first documented Russian diamond was discovered here. Subsequent exploration work in the middle of the 20th century led to the discovery of diamondiferous placers in the Krasnovishersky District of the Perm Krai. The main source of diamonds in these placers is the Takata (Takaty) Formation, which belongs to the Emsian stage of the Lower Devonian (393–407 Ma) [16]. The Takata Formation is composed of quartz sandstones with interlayers of weakly cemented diamondiferous conglomerates and gravelstones in the basal member [17]. Despite the production of diamonds in the Urals, their primary sources have not yet been discovered. It is assumed that these sources may be concealed under a thick sedimentary cover of the East European craton (EEC) [16]. The 40Ar/39Ar eruption time of the transporting mantle melt, determined from clinopyroxene inclusions in diamonds, was 472 ± 28 Ma (O1-2), which indicates a gap of ~ 65 Ma between the eruption of the primary source and the formation of the diamond-bearing Takata Formation [18]. Such estimates exceed the age (D3-C1) of diamondiferous kimberlite pipes of the Siberian craton and the north of the East European craton (Arkhangelsk diamondiferous province) [19, 20]. The absence of reliable outcrops of primary sources of the diamonds in the Urals led to the emergence of a number of alternative hypotheses, among which the most well-known and debatable is the hypothesis of diamond-bearing “tuffizites” [21].
Most studies of diamonds from placers of the Urals describe their morphological and spectroscopic characteristics [22–24]. The composition of mineral inclusions in such diamonds from a few studies indicates a significant predominance of eclogitic paragenesis [18, 25]. This paper presents the results of the first study of the composition of fluid/melt microinclusions in the fibrous diamonds from placers of the Krasnovishersky District (Middle/Northern Urals). Together with the characteristics of the impurity composition, these results were used to reconstruct the chemistry and evolution of diamond-forming media in the mantle of the eastern margin of the East European craton.
Methods
Two cuboids from the placers of the Krasnovishersky District [24] were preliminary cut with a laser and polished on a diamond disk parallel to the {100} planes to plates 0.5–0.8 mm thick. One coated diamond was polished parallel to the {110} plane. Images of diamonds in the cathodoluminescence (CL) mode were obtained at the Laboratory of Evolution of Paleo-Oceans and Mantle Magmatism, Novosibirsk State University, using a CITL Mk5 cold cathode mounted on a Carl Zeiss Axio Scope.A1 polarizing microscope at 20 kV and 1 mA.
The impurity composition of diamonds was studied at the V.S. Sobolev Institute of Geology and Mineralogy Siberian Branch RAS, Novosibirsk, using a Bruker Vertex 70 IR spectrometer combined with a HYPERION 2000 IR microscope. The spectra were recorded in the wavelength range 600–4500 cm–1 with signal accumulation in 60 scans at a spectral resolution of 1 cm–1 and an aperture of 50 × 50 μm. The concentrations of nitrogen centers were calculated using the coefficients NC (ppm) = 37.5 × a1344 (cm−1), NA = 16.5 × a1282, NB = 29.4 × a1170 [26]. The presence of hydrogen-related defects (N3VH) was estimated from the absorption coefficient of the peak at 3107 cm–1 [27]. The spectra were processed using the OPUS spectroscopy software (version 5.0, Bruker, USA) and SpectrExamination software (developer O. Kovalchuk, ALROSA). The values of the carbonate mole fraction (CMF = CO2 / (H2O + CO2)) in the microinclusions were calculated from the maximum intensity of the absorption bands of water (~3420 cm–1) and carbonates (~1450 cm–1) using the coefficients from [28].
The composition of microinclusions was analyzed using an Oxford Instruments X-Max 80 energy dispersive spectrometer (EDS) mounted on a TESCAN MIRA LMS scanning electron microscope at the Common Use Center of the Ural Branch of RAS “Geoanalyst” (Zavaritsky Institute of Geology and Geochemistry). Identification of subsurface microinclusions was carried out in the electron backscattering (BSE) mode under the action of a focused electron beam (20 keV, 0.8 nA). The signal of each spectrum was accumulated for 30 s. The data were processed using Aztec v3.2 software. The chemical composition of microinclusions in each sample was calculated by averaging 20-25 analyzes of individual microinclusions, normalized to 100 wt.% (with stoichiometrically calculated oxygen without regard for carbon).
The composition of the mineral inclusion of clinopyroxene was obtained on a Cameca SX100 electron probe microanalyzer (EPMA) equipped with five WDS spectrometers at the Common Use Center of the Ural Branch of RAS “Geoanalyst” at a beam current of 20 nA and an accelerating voltage of 15 keV. The elements were calibrated using the mineral standards diopside (Mg, Ca), jadeite (Na, Al), and hematite (Fe).
Results and discussion
Diamonds from the placers of the Western Urals show predominance of rounded dodecahedroids (tetrahexahedroids) (70-90%), which are the final form of diamond dissolution [29, 30]. A few finds of diamonds with curved cubic faces were also reported [24]. In the cuboids from this study (602-66, 685-66), the central part is represented by a transparent zone, which is characterized by the mixed-habit growth of <100> and <111> sectors (Fig. 1, a, b). The outer zone saturated with microinclusions demonstrates curved concentric zonation corresponding to the <100> growth. The cathodoluminescent images of two diamonds also show systems of thin subparallel lines, probably reflecting the direction of plastic deformation of the crystals.
The coated diamond consists of four growth zones (Fig. 1c): (i) an inner highly luminescent octahedral core with rectilinear zoning reflecting layer-by-layer (tangential) growth; (ii) an outer weakly luminescent octahedral core with linear zoning; (iii) an inner coat showing curved concentric zonation marking the transition to <100> cubic growth; (iv) an outer coat consisting of fibers the direction of growth of which corresponds to <111>. The outer coat is characterized by a dull yellow-green luminescence and a yellow color in transmitted light. Microinclusions of diamond-forming fluids/melts were found both in the third and fourth zones.
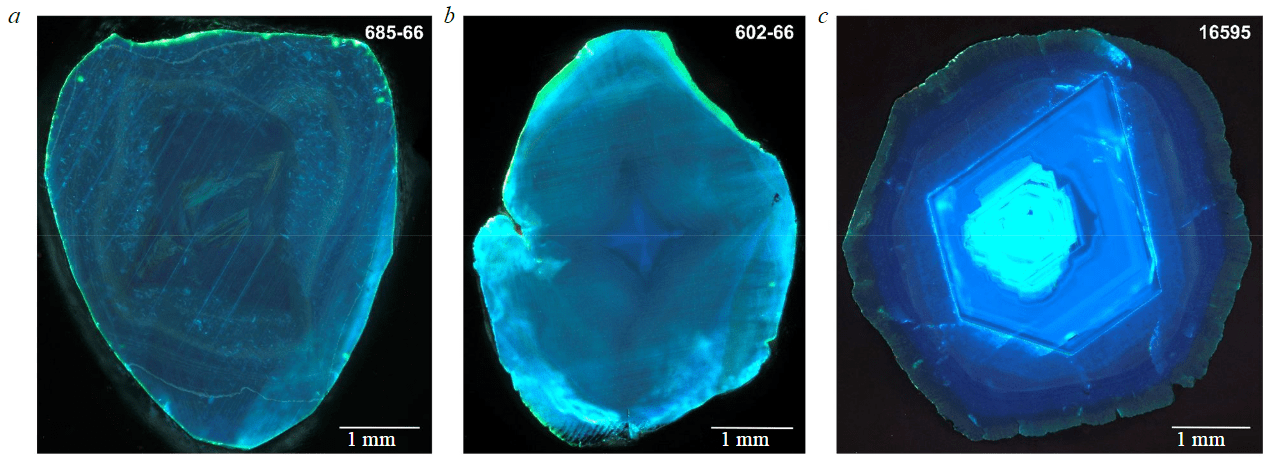
Fig.1. Cathodoluminescence images of diamond plates from placers of the Krasnovishersky District
Nitrogen is the most common structural impurity in diamond [26]. The form of nitrogen incorporation depends on the temperature and time of diamond annealing in mantle conditions [31, 32]. The most common diamonds contain A (two nitrogen atoms in neighboring positions) and highly aggregated B (four nitrogen atoms surrounding a vacancy) nitrogen defects in different ratio (type Ia) [33]. Much less common are diamonds containing low-temperature C (single nitrogen atom) defect centers (type Ib), or containing nitrogen in concentrations below the detection limits by IR spectroscopy (type IIa) [33]. The diamonds from the Urals placers belong to the IaAB type. The aggregation state (% B = 100 × B / (B + A)) and nitrogen content in these diamonds range from 30% to 60%, and from 400 to 900 ppm, respectively [23, 29, 34]. Rare diamonds of the cubic habit also contain low-aggregate C-centers in low concentrations [24].
Diamond 685-66 is characterized by a relatively uniform impurity composition (Fig. 2d-f). It belongs to the type IaA and contains exclusively A-centers. The total nitrogen content varies within a narrow range of 239-256 ppm. The absorption coefficient of the band at 3107 cm–1, which indicates the presence of hydrogen-containing defects (N3VH), shows slight variations from 3.4 to 4.1 cm–1 [27]. Such defects also appear as sharp peaks at 1405 and 2786 cm–1 [26].
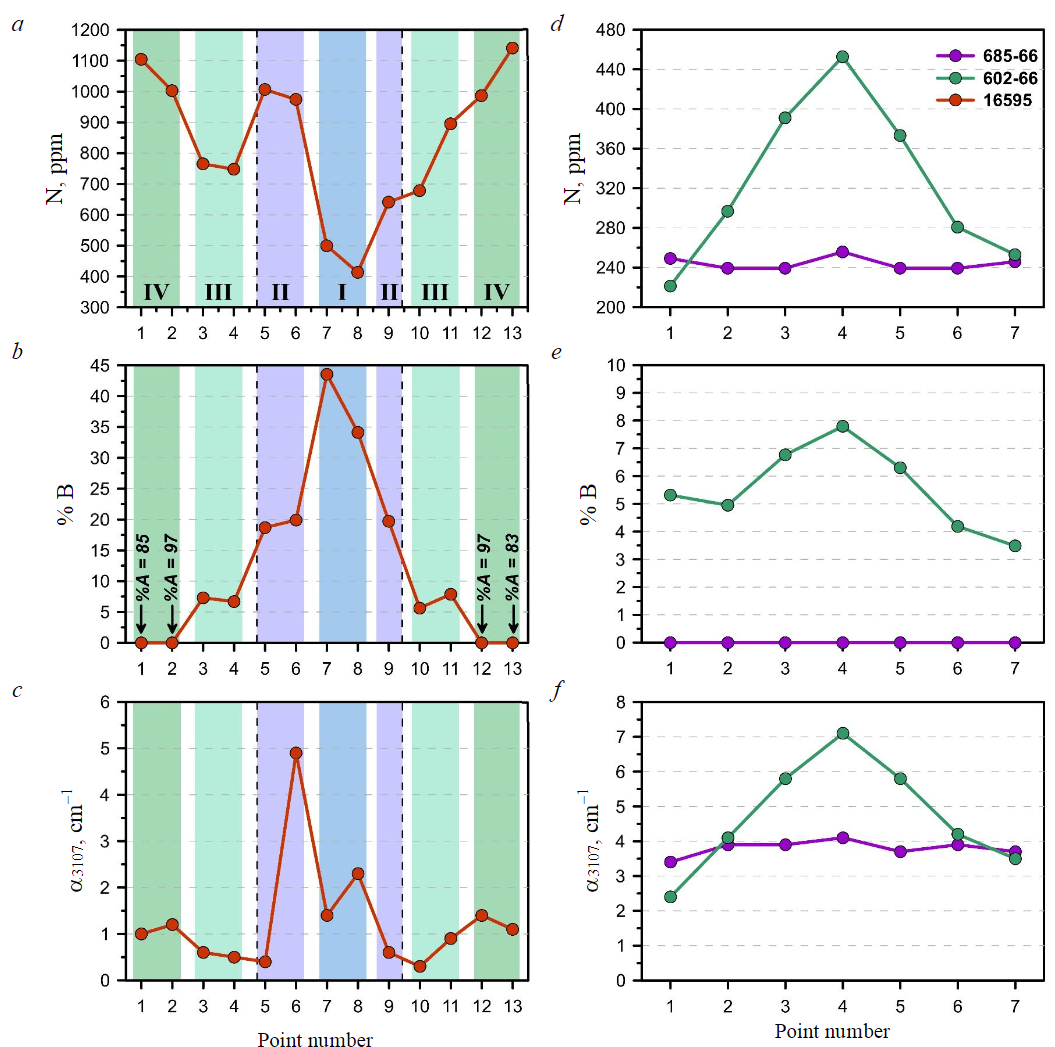
Fig.2. Profiles (rim-center-rim) of total nitrogen content (a, d), aggregation state % B = 100 × B / (A + B) (b, e) and absorption coefficient of hydrogen-containing centers at 3107 cm–1 (c, f) in diamonds from the placers of the Krasnovishersky District. Growth zones of coated diamond (sample 16595): I – inner core; II - outer core; III – inner coat; IV - outer coat. The content of A-centers relative to C-centers in the outer coat % A = 100 × A / (A + C).
Diamond 602-66 contains a small amount of B defects (3-8%) with a total nitrogen content of 210-417 ppm (Fig. 2d-f). The central part of the sample is enriched in nitrogen and B centers relative to rim. The same regularity was found for the absorption coefficient of the peak at 3107 cm–1 (2.4–7.1 cm–1), which indicates the implementation of growth conditions that promote the incorporation of both nitrogen and hydrogen into the diamond structure.
The diamond core 16595 demonstrates a non-uniform distribution of nitrogen content and B defects (Fig. 2, a-c). The inner core is characterized by a high aggregation state (34-44% B) and low nitrogen content (413-500 ppm), which indicates its longer annealing in mantle conditions [31]. The outer core show higher content of nitrogen (678-1007 ppm), and lower content of B centers (19-20%). The absorption coefficient of the peak corresponding to B’ defects (platelets) consisting of layers of interstitial carbon atoms [35] depends on the nitrogen aggregation state and varies in the range from 3.2 to 13.2 cm–1. The position of the B’ peak in the aggregated inner core corresponds to a wavelength of 1363 cm–1, while the position of the B’ band in the outer core is shifted by 1370–1372 cm–1. The position of the maximum reflects the linear size of the layers of B’ defects, which indicates their larger diameter in the inner core compared to the outer one [35]. The absorption coefficient of the peak at 3107 cm–1 varies from 0.4 to 4.9 cm–1. The inner coat of the diamond 16595 is characterized by low content of nitrogen (678–896 ppm) and B defects (6–8%). It also shows low absorption coefficients of the hydrogen-related peak (0.3-0.9 cm–1). The outer yellow-green coat contains A and low-aggregated C nitrogen defect centers. The concentrations of C-centers (100 × C / (A + C)) and nitrogen increase from 3% and 987-1003 ppm to 15-17% and 1104-1141 ppm, respectively, when moving to the rim.
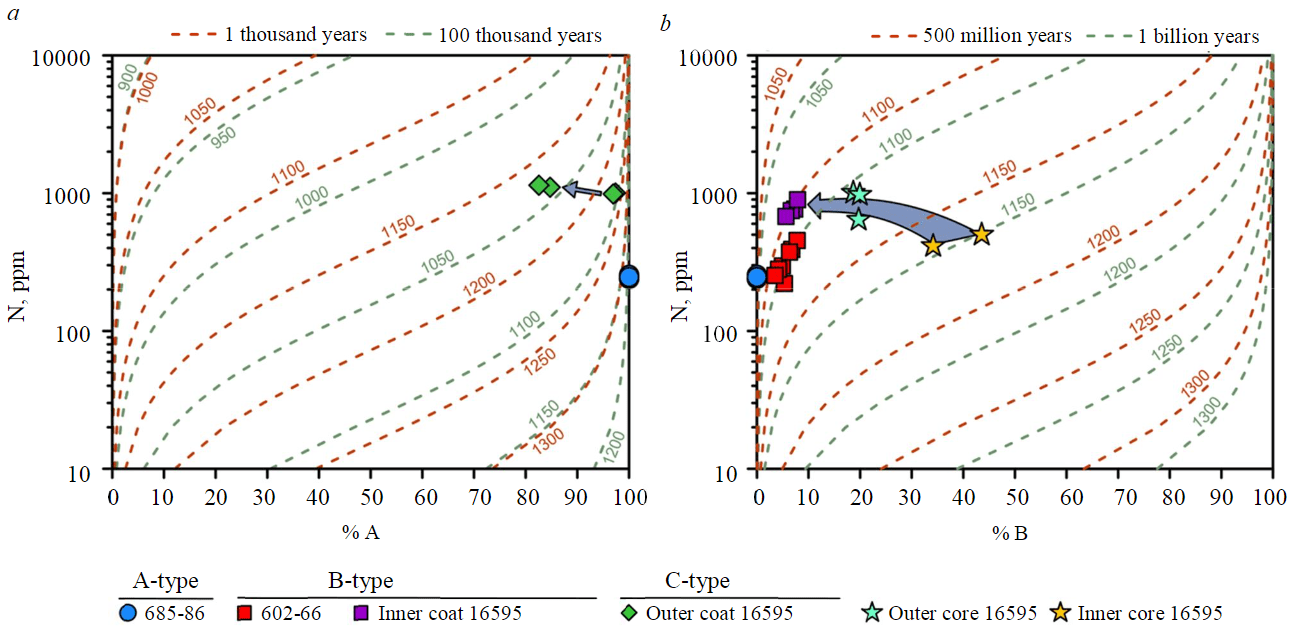
Fig.3. Impurity composition and temperature conditions of mantle annealing of diamonds from placers of the Krasnovishersky District. Isotherms were calculated based on the equations given in [31, 32] using kinetic parameters Ea = 6 eV, ln(A) = 18.81 for the transition of C centers to A centers (a) and Ea = 7 eV, ln(A) = 12.59 for the transition of A-centers to B-centers (b). The arrows show the growth evolution of diamond 16595
The impurity composition of the studied diamonds indicates their differences in time and/or temperature of residence in mantle conditions. The Re-Os systematics of eclogitic sulfide inclusions in diamonds from the Ural placers indicate that the age of their formation is 1280 ± 310 Ma [18]. The estimated 40Ar/39Ar age of the eruption of the transporting mantle melt (the ascent of diamonds to the surface), determined from inclusions of eclogitic clinopyroxene in diamonds, is 472 ± 28 Ma [18]. Taking into account the errors, the annealing time of the Ural dodecahedroids in mantle conditions was probably limited to 470-1146 Ma. To estimate the temperatures of mantle residence, we therefore used values of 500 million and 1 billion years (Fig. 3, b). With these time frames, diamond 602-66 experienced annealing at temperatures of ~1090-1120°C, which is higher than the values obtained for diamond 685-66 (~1000-1040 °C) [31]. This difference may be due to both higher annealing temperatures and longer mantle residence of sample 602-66. The impurity composition of the diamond core 16595 indicates annealing at temperatures of ~1110-1170 °С, while the inner aggregated core is characterized by higher values (~1150-1170 °С) compared to the outer core (~1110-1140 °С). The composition of the inner coat reflects the ambient temperatures in the range from ~1080 to 1100 °С. The outer coat contains C-centers, which quickly transform into more aggregated A-centers in the diamond stability field [32]. This indicates that the formation of the outer coat occurred shortly before it was carried to the surface by the mantle transporting melt. When assessing the mantle residence at 100 thousand years, the annealing temperature of the outer coat is ~1040-1100 °С, which is comparable to the interval obtained for the inner coat (Fig. 3, a) [32]. Thus, in the case of a relatively constant temperature conditions during the growth of different zones of diamond, the time gap between the formation of the outer and inner coat could be more than 400 million years. At higher temperatures (1170-1250 °С), the time of annealing of the outer coat is reduced from 100 to ~1 thousand years. Based on the characteristics of the impurity composition, three types of diamonds containing microinclusions can be distinguished, the formation of which was associated with three metasomatic events separated from each other in time. These types consist of diamonds containing exclusively A-centers (A-type; sample 685-66); A and B centers (B-type; sample 602-66 and inner coat of sample 16595); A and C centers (C-type; outer coat of sample 16595). Similar fibrous diamond populations have also been identified for the North Atlantic craton (NAC). [14].
The reported compositions of microinclusions of HDFs worldwide is limited by three end members: (i) high-Mg carbonatitic, rich in Fe, Mg and Ca carbonates; (ii) saline, enriched in K and Cl; (iii) silicic, characterized by high concentrations of Si, Al, K, and H2O [9, 13, 14]. Low-Mg carbonatitic group of HDFs is also distinguished between silicic and high-Mg carbonatitic end members. Intermediate compositions of the silicic-saline trend are currently not documented (Fig. 4, a).
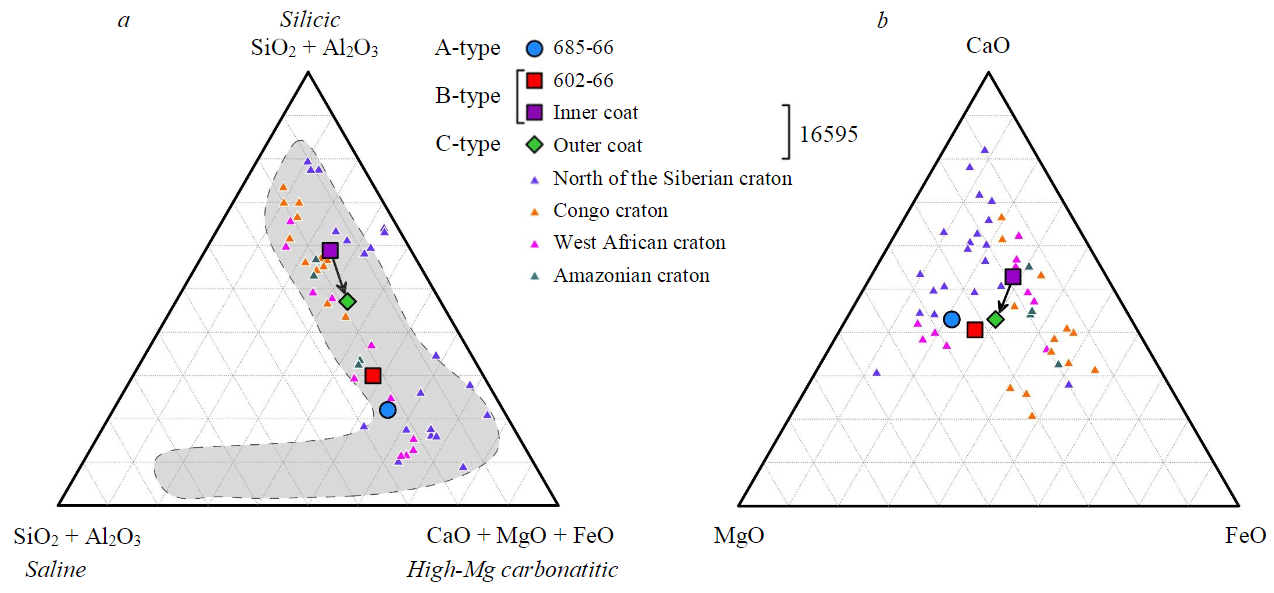
Fig.4. Composition of microinclusions of HDFs in diamonds from placers of the Krasnovishersky District. The grayish field represents documented data on fluid/melt compositions in fibrous diamonds worldwide [9]. The arrows indicate the evolution of fluids/melts during the formation of different growth zones of diamond 16595. The compositions of microinclusions in diamonds from placers of the north of the Siberian craton [36], Congo craton [37, 38], West African craton [39], and Amazonian craton [40] are plotted for comparison.
The formation of B-type diamonds reflects an ancient metasomatic event in the subcontinental lithospheric mantle of the eastern margin of the East European craton. The studied B-type diamonds show conspicuous differences in the composition of trapped fluids/melts (Figs. 4, 5). Diamond 602-66 (B-type) contains microinclusions of low-Mg carbonatitic HDFs. The divalent cations are the primary components of such microinclusions (CaO + MgO + FeO + ВаО = 44.7 wt.%) and are predominantly concentrated in carbonate phases, as evidenced by the high value of the ratio CMF = 0.25, determined using IR spectroscopy (see table). IR spectroscopy also revealed the presence of additional absorption bands corresponding to calcite (877 cm–1; 834 cm–1). CaO slightly predominates among the divalent cations in the microinclusions of sample 602-66 (see Fig. 4b). SiO2 and K2O content is 21.5 and 15 wt.%, respectively. The concentrations of other components (Al2O3, TiO2, Na2O, P2O5, SO3, and Cl) do not exceed 5.75 wt.%. The B-type inner coat of the diamond 16595 captured diamond-forming fluids/melts of silicic composition. They are characterized by the predominance of SiO2 (44.8 wt.%), Al2O3 (8.31 wt.%), CaO (11.9 wt.%) and K2O (11.3 wt.%). CaO significantly dominates over other divalent cations and probably accumulates in the phosphates and carbonates. The low value of the ratio CMF = 0.03 indicates a higher concentration of water in these microinclusions.
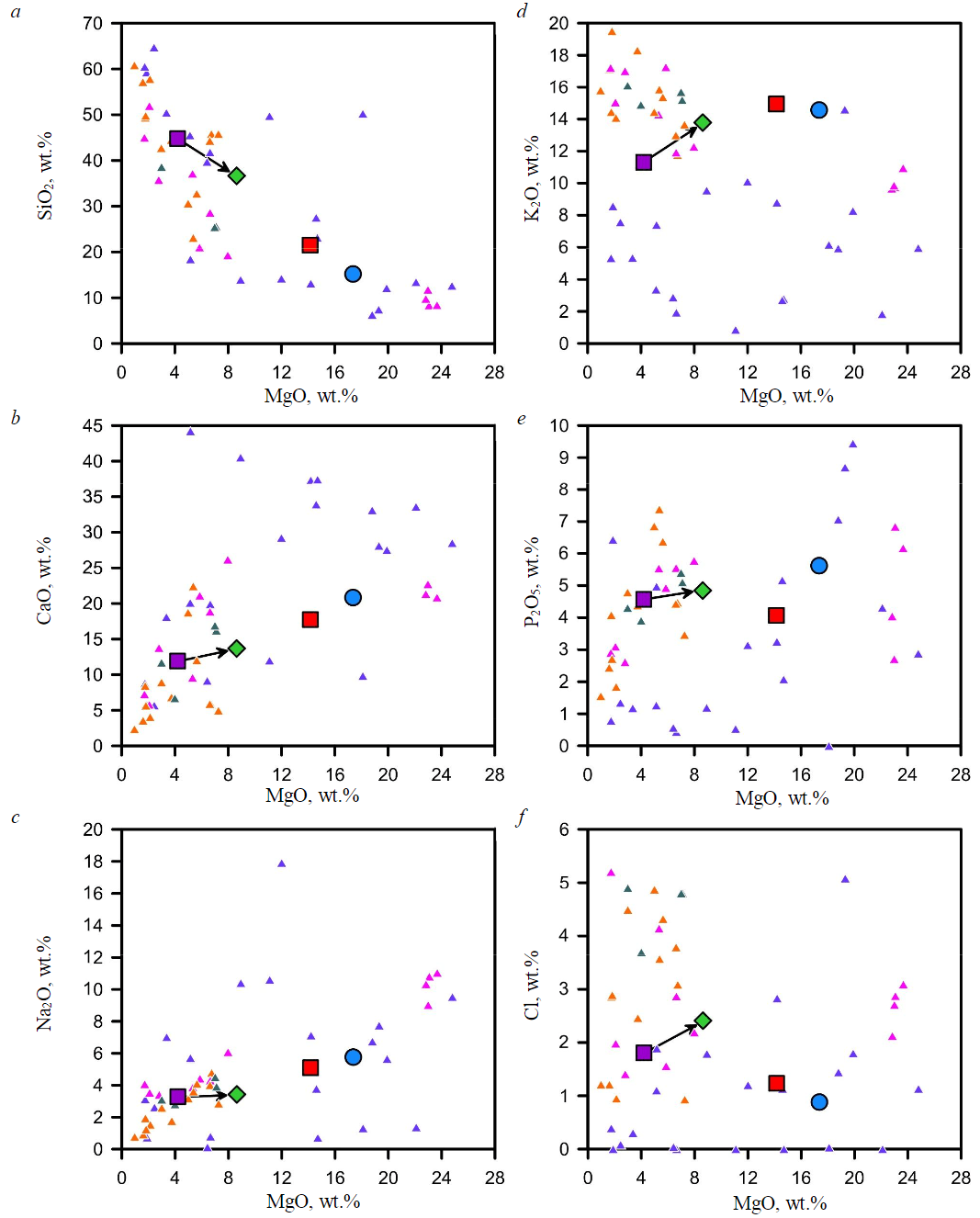
Fig.5. Two-component diagrams of HDFs compositions in diamonds from placers of the Krasnovishersky District. Variations of SiO2 (a), CaO (b), Na2O (c), K2O (d), P2O5 (e) and Cl (f) depending on the content of MgO in microinclusions are shown. The legend from Fig.4 is used.
Composition of microinclusions of fluids/melts in diamonds from placers of the Krasnovishersky region
Characteristics |
Sample |
||||
685-66 |
602-66 |
16595 |
|||
Type1 |
A |
B |
B |
C |
|
n2 |
20 |
25 |
23 |
20 |
|
Zone of diamond |
|
|
Inner coat |
Outer coat |
|
SiO23 |
15.2 (4.2) |
21.5 (5.4) |
44.8 (7.7) |
36.7 (6.3) |
|
TiO2 |
3.06 (2.2) |
2.18 (1.2) |
2.01 (1.4) |
– |
|
Al2O3 |
4.23 (2.3) |
5.75 (1.5) |
8.31 (1.7) |
7.00 (1.1) |
|
FeO |
10.2 (2.7) |
11.8 (1.8) |
6.42 (2.1) |
9.52 (1.8) |
|
MgO |
17.4 (3.4) |
14.2 (2.3) |
4.21 (1.5) |
8.63 (2.9) |
|
CaO |
20.8 (7.0) |
17.7 (6.1) |
11.9 (5.8) |
13.7 (3.1) |
|
Na2O |
5.76 (1.7) |
5.10 (1.7) |
3.28 (1.9) |
3.43 (2.7) |
|
K2O |
14.6 (2.8) |
15.0 (3.1) |
11.3 (3.5) |
13.8 (2.8) |
|
BaO |
1.59 (1.4) |
0.99 (0.8) |
1.24 (0.9) |
– |
|
P2O5 |
5.63 (3.1) |
4.07 (2.7) |
4.57 (3.2) |
4.85 (3.9) |
|
SO3 |
0.63 (0.6) |
0.57 (0.5) |
0.18 (0.1) |
– |
|
Cl |
0.88 (0.5) |
1.24 (0.6) |
1.81 (0.9) |
2.41 (1.9) |
|
CMF4 |
0.43 |
0.25 |
0.03 |
0.10 |
|
CO25 |
23.7 |
16.9 |
3.72 |
6.86 |
|
H2O5 |
12.8 |
20.7 |
49.2 |
25.3 |
|
1 Type of diamonds in accordance with their impurity composition: A – containing solely A nitrogen centers; B - containing A- and B-centers, C - containing A- and C-centers. |
Compositional diversity between microinclusions from two B-type diamonds may reflect the features of crystallization of cuboids and coated diamonds. It is known that an alkaline carbonate melt is a favorable medium for diamond nucleation [41]. The possibility of diamond nucleation in a silicate fluid/melt significantly depends on the content of H2O and CO2 and reaches a minimum in dry systems [41]. The impurity composition of cuboid 602-66 indicates its formation within one metasomatic event. Due to the need for nucleation, its growth occurred in the most favorable alkaline water-containing carbonate media. The ancient core in sample 16595 probably acted as a seed and contributed to the growth of the inner coat from less favorable silicate fluids/melts saturated with volatiles. A similar relationship between the forms of fibrous diamonds and the composition of microinclusions are also noted for other regions [13, 39].
Microinclusions in cuboid 685-66 (A-type) are characterized by compositions close to those described in diamond 602-66 (see table). The main components of such microinclusions are CaO (20.8 wt.%), MgO (17.4 wt.%), SiO2 (15.2 wt.%), K2O (14.6 wt.%), and FeO (10.2 wt.%). They also show high concentrations of Na2O (5.76 wt.%) and P2O5 (5.63 wt.%). The CMF = 0.43 ratio indicates a significant predominance of carbonates in the daughter phases. The key difference from diamond 602-66 (B-type) is the more carbonatitic composition of the trapped fluids/melts, which show a higher content of MgO, Na2O, CaO, and CO2 and a lower concentration of SiO2 (Figs. 4, 5).
The outer coat of sample 16595 (C-type) contains C-centers that are unstable in the diamond stability field [32]. Their presence indicates that the formation of the outer coat occurred shortly before the eruption of the transporting mantle melt. Microinclusions of HDFs have silicic to low-Mg carbonatitic composition, less enriched in SiO2 (36.7 wt.%) and Al2O3 (7 wt.%) relative to the inner coat (Fig. 4, 5). They also show higher concentrations of K2O (13.8 wt.%), CaO (13.7 wt.%), FeO (9.52 wt.%) and MgO (8.63 wt.%). The low value of the ratio CMF = 0.1 and the high concentrations of SiO2, Al2O3, and K2O indicate the predominance of water and silicate phases in the microinclusions (see table).
Microinclusions in fibrous diamonds with different characteristics of the impurity composition from the placers of the Krasnovishersky District of the Middle/Northern Urals reflect at least three metasomatic diamond-forming events separated in time. The first, more ancient, proceeded with the participation of silicic (inner coat 16595) and low-Mg carbonatitic fluids/melts (cuboid 602-66). The second stage of diamond formation was associated with HDFs similar in composition to those found in cuboid 602-66, but having a more carbonate composition with lower SiO2 content and higher concentrations of MgO, Na2O, CaO, and CO2. The third stage, which preceded the ascent of diamonds to the surface, led to the formation of the outer coat of diamond 16595 and was associated with silicic to low-Mg carbonatitic fluids/melts enriched in MgO, FeO, K2O, and CO2 and depleted in SiO2 relative to microinclusions in the inner coat. Thus, taking into account the possible differences in growth conditions between cuboids and coated diamonds, the general trend in the evolution of fluids/melts is directed from more silicic towards more carbonatitic compositions. A similar trend was also found in diamonds with radial variations in the composition of microinclusions of the HDFs from Brazil and Guinea [39, 40]. The compositional features of HDFs match those described in diamonds from placers of the West African, Amazonian, and north of the Siberian cratons [36, 39, 40]. The placers of the Congo craton with established kimberlite sources are dominated by silicic and silicic to low-Mg carbonatitic diamond-forming media close to those found in sample 16595 [37, 38]. All studied microinclusions from diamonds of the Krasnovishersky District fall into the compositional field of fluids/melts from kimberlite fibrous diamonds (see Fig. 4) [9].
Microinclusions from the studied diamonds fill the entire array between silicic and low-Mg carbonatitic end-members. The formation of such fluids/melts is associated with the partial melting of eclogites with different H2O and CO2 ratios [42]. This relationship is confirmed by findings of mineral inclusions of eclogitic paragenesis in HDF-bearing diamonds [13, 14, 43]. The low-Mg carbonatitic fluids/melts found in cuboids 602-66 and 685-66 probably sourced from CO2-enriched eclogites [42, 44]. Peridotite derived fluids/melts at similar SiO2 content are characterized by a significant predominance of MgO over CaO [44], which is not typical for the studied microinclusions. Mineral inclusion of pyroxenitic (websteritic) clinopyroxene was found in the inner coat of diamond 16595 (SiO2 = 54; TiO2 = 0.13; Al2O3 = 1.14; Cr2O3 = 0.22; FeO = 2.11; MgO = 17.3; CaO = 23 .8; Na2O = 0.43; K2O = 0.02; MnO = 0.02; NiO = 0.05; CoO = 0.03 wt %) [10]. Therefore, the formation diamond-forming fluids/melts could be associated with partial melting of a pyroxenitic or mixed eclogitic-pyroxenitic substrate [42, 44, 45]. Thus, the growth of cuboids and coated diamonds from the placers of the Krasnovishersky District of the Middle/Northern Urals is associated with eclogitic and pyroxenitic mantle rocks. A significant predominance of inclusions of eclogitic paragenesis is also characteristic of the Ural dodecahedroids [18, 25]. This indicates the important role of subduction processes in the formation of diamonds, which is also assumed for placers in the north of the Siberian craton [46].
Conclusion
Diamonds containing microinclusions from the placers of the Krasnovishersky District of the Middle/Northern Urals are characterized by different impurity composition, which makes it possible to distinguish at least three separated metasomatic events in the subcontinental lithospheric mantle of the eastern margin of the East European craton:
- The most ancient metasomatic stage led to the formation of B-type diamonds containing nitrogen in the form of A- and B -centers. The aggregation state (% B) of these diamonds is 3-8% and the nitrogen content varies between 210-896 ppm. The formation of B-type diamonds was associated with low-Mg carbonatitic (for cuboid) and silicic (for coated diamond) fluids/melts.
- The next metasomatic stage led to the formation of A-type cuboid containing nitrogen exclusively in the form of A-centers in concentrations of 239-256 ppm. This stage proceeded with the active participation of low-Mg carbonatitic fluids/melts, more enriched in MgO, CaO, Na2O and CO2 and depleted in SiO2 relative to B-type cuboid microinclusions.
- The last metasomatic stage preceded the eruption of the transporting mantle melt and led to the formation of C-type outer coat containing C and A nitrogen centers (C = 3-17%). The total nitrogen content in the coat varies in the range of 987-1141 ppm. The growth of this diamond population is associated with silicic to low-Mg carbonatitic fluids/melts, which have a more carbonatitic composition compared to the inner coat of the B-type.
The revealed trend in the evolution of the deep diamond-forming fluids/melts in the subcontinental lithospheric mantle of the eastern margin of the East European craton is directed towards more carbonatitic compositions enriched in MgO, CaO, Na2O, and CO2. The sources of the involved fluids/melts could be eclogitic and pyroxenitic mantle substrates with different H2O and CO2 ratios. Compositional features of studied microinclusions of HDFs match fluids/melts described in fibrous diamonds from placers and kimberlites worldwide.
References
- O’Reilly S.Y., Griffin W.L. Mantle Metasomatism. Metasomatism and the Chemical Transformation of Rock. Heidelberg: Springer, 2013, p. 471-533. DOI: 10.1007/978-3-642-28394-9_12
- Aulbach S., Massuyeau M., Garber J.M. et al. Ultramafic Carbonated Melt- and Auto‐Metasomatism in Mantle Eclogites: Compositional Effects and Geophysical Consequences. Geochemistry, Geophysics, Geosystems. 2020. Vol. 21. Iss. 5. N e2019GC008774. DOI: 10.1029/2019GC008774
- Skuzovatov S., Shatsky V.S., Ragozin A.L., Smelov A.P. The evolution of refertilized lithospheric mantle beneath the northeastern Siberian craton: Links between mantle metasomatism, thermal state and diamond potential. Geoscience Frontiers. 2022. Vol. 13. Iss. 6. N 101455, p. 1-17. DOI: 10.1016/j.gsf.2022.101455
- Melnik A.E., Korolev N.M., Skublov S.G. et al. Zircon in mantle eclogite xenoliths: a review. Geological Magazine. 2021. Vol. 158. Iss. 8, p. 1371-1382. DOI: 10.1017/S0016756820001387
- Ashchepkov I., Logvinova A., Spetsius Z. et al. Eclogite Varieties and Their Positions in the Cratonic Mantle Lithosphere beneath Siberian Craton and Archean Cratons Worldwide. Minerals. 2022. Vol. 12. N 1353. DOI: 10.3390/min12111353
- Kargin А.V., Sazonova L.V., Nosova A.A. et al. Phlogopite in mantle xenoliths and kimberlite from the Grib pipe, Arkhangelsk province, Russia: Evidence for multi-stage mantle metasomatism and origin of phlogopite in kimberlite. Geoscience Frontiers. 2019. Vol. 10. Iss. 5, p. 1941-1959. DOI: 10.1016/j.gsf.2018.12.006
- Mikhailenko D., Golovin A., Korsakov A. et al. Metasomatic Evolution of Coesite-Bearing Diamondiferous Eclogite from the Udachnaya Kimberlite. Minerals. 2020. Vol. 10. Iss. 4. N 383. DOI: 10.3390/min10040383
- Stachel T., Luth R.W. Diamond formation – Where, when and how? Lithos. 2015. Vol. 220-223, p. 200-220. DOI: 10.1016/j.lithos.2015.01.028
- Weiss Y., Czas J., Navon O. Fluid Inclusions in Fibrous Diamonds. Reviews in Mineralogy and Geochemistry. 2022. Vol. 88. Iss. 1, p. 475-532. DOI: 10.2138/rmg.2022.88.09
- Stachel T., Aulbach S., Harris J.W. Mineral Inclusions in Lithospheric Diamonds. Reviews in Mineralogy and Geochemistry. 2022. Vol. 88. Iss. 1, p. 307-391. DOI: 10.2138/rmg.2022.88.06
- Walter M.J., Thomson A.R., Smith E.M. Geochemistry of Silicate and Oxide Inclusions in Sublithospheric Diamonds. Reviews in Mineralogy and Geochemistry. 2022. Vol. 88. Iss. 1, p. 393-450. DOI: 10.2138/rmg.2022.88.07
- Smit K.V., Timmerman S., Aulbach S. et al. Geochronology of Diamonds. Reviews in Mineralogy and Geochemistry. 2022. Vol. 88. Iss. 1, p. 567-636. DOI: 10.2138/rmg.2022.88.11
- Gubanov N., Zedgenizov D., Sharygin I., Ragozin A. Origin and Evolution of High-Mg Carbonatitic and Low-Mg Carbonatitic to Silicic High-Density Fluids in Coated Diamonds from Udachnaya Kimberlite Pipe. Minerals. 2019. Vol. 9. Iss. 12. N 734. DOI: 10.3390/min9120734
- Elazar O., Grütter H., Weiss Y. The A B C's of metasomatism in the North Atlantic Craton during Pangea breakup; characterized by fluid inclusions in Chidliak diamonds. Lithos. 2022. Vol. 422-423. N 106725. DOI: 10.1016/j.lithos.2022.106725
- Puchkov V.N. The evolution of the Uralian orogeny. Geological Society, London, Special Publications. 2009. Vol. 327, 161-195. DOI: 10.1144/SP327.9
- Puchkov V.N. General features relating to the occurrence of mineral deposits in the Urals: What, where, when and why. Ore Geology Reviews. 2017. Vol. 85, p. 4-29. DOI: 10.1016/j.oregeorev.2016.01.005
- Konstantinovskii A.A. Epochs of Diamond Placer Formation in the Precambrian and Phanerozoic. Lithology and Mineral Resources. 2003. Vol. 38. N 6, p. 530-546. DOI: 10.1023/A:1027316611376
- Laiginhas F., Pearson D.G., Phillips D. et al. Re-Os and 40Ar/39Ar isotope measurements of inclusions in alluvial diamonds from the Ural Mountains: Constraints on diamond genesis and eruption ages. Lithos. 2009. Vol. 112. S. 2, p. 714-723. DOI: 10.1016/j.lithos.2009.03.003
- Sun J., Tappe S., Kostrovitsky S.I. et al. Mantle sources of kimberlites through time: A U-Pb and Lu-Hf isotope study of zircon megacrysts from the Siberian diamond fields. Chemical Geology. 2018. Vol. 479, p. 228-240. DOI: 10.1016/j.chemgeo.2018.01.013
- Ustinov V.N., Mikoev I.I., Piven G.F. Prospecting models of primary diamond deposits of the north of the East European Platform. Journal of Mining Institute. Vol. 255, p. 299-318. DOI: 10.31897/PMI.2022.49
- Lukyanova L.I., Zhukov V.V., Kirillov V.A. et al. On detecting a bedrock diamond source in the Urals. Regional Geology and Metallogeny. N 12, p. 134-157 (in Russian).
- Vasilev E.A., Klepikov I.V., Kozlov A.V., Antonov A.V. The Nature of the Elongated Form of Diamond Crystals From Urals Placers. Journal of Mining Institute. Vol. 239, p. 492-496. DOI: 10.31897/PMI.2019.5.492
- Nefedov Y.V., Klepikov I.V. Occurrence Regularities of Nitrogen Defects in the Ural Type Crystal Diamonds from Different Regions. Key Engineering Materials. 2018. Vol. 769, p. 201-206. DOI: 10.4028/www.scientific.net/KEM.769.201
- Klepikov I.V., Vasilev E.A., Antonov A.V. The Defect-Impurity Composition of Diamond Crystals with 〈100〉Growth Pyramids from Placers of the Krasnovishersk District, the Urals. Geology of Ore Deposits. 2020. Vol. 62. N 8, p. 743-753. DOI: 10.1134/S107570152008005X
- Sobolev N.V., Logvinova A.M., Tomilenko A.A. et al. Mineral and fluid inclusions in diamonds from the Urals placers, Russia: Evidence for solid molecular N2 and hydrocarbons in fluid inclusions. Geochimica et Cosmochimica Acta. 2019. Vol. 266, 197-219. DOI: 10.1016/j.gca.2019.08.028
- Zaitsev A.M. Optical Properties of Diamond. Heidelberg: Springer, 2001. 502 p. DOI: 10.1007/978-3-662-04548-0
- Goss J.P., Briddon P.R., Hill V. et al. Identification of the structure of the 3107 cm−1 H-related defect in diamond. Journal of Physics: Condensed Matter. 2014. Vol. 26. N 14. N 145801. DOI: 10.1088/0953-8984/26/14/145801
- Weiss Y., Kiflawi I., Navon O. IR spectroscopy: Quantitative determination of the mineralogy and bulk composition of fluid microinclusions in diamonds. Chemical Geology. 2010. Vol. 275. Iss. 1-2, p. 26-34. DOI: 10.1016/J.CHEMGEO.2010.04.010
- Vasilev E.A., Klepikov I.V., Lukianova L.I. Comparison of Diamonds from the Rassolninskaya Depression and Modern Alluvial Placers of the Krasnovishersky District (Ural Region). Geology of Ore Deposits. 2019. Vol. 61. N 7, p. 598-605. DOI: 10.1134/S1075701519070134
- Harris J.W., Smit K.V., Fedortchouk Y., Moore M. Morphology of Monocrystalline Diamond and its Inclusions. Reviews in Mineralogy and Geochemistry. 2022. Vol. 88. Iss. 1, p. 119-166. DOI: 10.2138/rmg.2022.88.02
- Taylor W.R., Jaques A.L., Ridd M. Nitrogen-defect aggregation characteristics of some Australasian diamonds: Time-temperature constraints on the source regions of pipe and alluvial diamonds. American Mineralogist. Vol. 75. N 11-12, p. 1290-1310.
- Taylor W.R., Canil D., Milledge H.J. Kinetics of Ib to IaA nitrogen aggregation in diamond. Geochimica et Cosmochimica Acta. 1996. Vol. 60. Iss. 23, p. 4725-4733. DOI: 10.1016/S0016-7037(96)00302-X
- Green B.L., Collins A.T., Breeding C.M. Diamond Spectroscopy, Defect Centers, Color, and Treatments. Reviews in Mineralogy and Geochemistry. 2022. Vol. 88. Iss. 1, p. 637-688. DOI: 10.2138/rmg.2022.88.12
- Fedorova E.N., Logvinova A.M., Luk’yanova L.I., Sobolev N.V. Typomorphic characteristics of the Ural diamonds (from FTIR spectroscopy data). Russian Geology and Geophysics. 2013. Vol. 54. N 12, p. 1458-1470. DOI: 10.1016/j.rgg.2013.10.013
- Speich L., Kohn S.C., Wirth R. et al. The relationship between platelet size and the B′ infrared peak of natural diamonds revisited. Lithos. 2017. Vol. 278-281, p.419-426. DOI: 10.1016/j.lithos.2017.02.010
- Zedgenizov D.A., Ragozin A.L., Shatsky V.S. et al. Fibrous diamonds from the placers of the northeastern Siberian Platform: carbonate and silicate crystallization media. Russian Geology and Geophysics. Vol. 52. N 11, p. 1298-1309. DOI: 10.1016/j.rgg.2011.10.003.
- Kosman C.W., Kopylova M.G., Stern R.A. et al. Cretaceous mantle of the Congo craton: Evidence from mineral and fluid inclusions in Kasai alluvial diamonds. Lithos. 2016. Vol. 265, p. 42-56. DOI: 10.1016/j.lithos.2016.07.004
- Timmerman S., Yeow H., Honda M. et al. U-Th/He systematics of fluid-rich ‘fibrous’ diamonds – Evidence for pre- and syn-kimberlite eruption ages. Chemical Geology. 2019. Vol. 515, p. 22-36. DOI: 10.1016/j.chemgeo.2019.04.001
- Weiss Y., Kessel R., Griffin W.L. et al. A new model for the evolution of diamond-forming fluids: Evidence from microinclusion-bearing diamonds from Kankan, Guinea. Lithos. 2009. Vol. 112, p. 660-674. DOI: 10.1016/j.lithos.2009.05.038
- Shiryaev A.A., Izraeli E.S., Hauri E.G. et al. Chemical, optical, and isotopic investigation of fibrous diamonds from Brazil. Russian Geology and Geophysics. 2005. Vol. 46. N 12, p. 1207-1222.
- Luth R.W., Palyanov Y.N., Bureau H. Experimental Petrology Applied to Natural Diamond Growth. Reviews in Mineralogy and Geochemistry. 2022. Vol. 88. Iss. 1, p. 755-808. DOI: 10.2138/rmg.2022.88.14
- Elazar O., Frost D., Navon O., Kessel R. Melting of H2O and CO2-be aring eclogite at 4-6 GPa and 900-1200 °C: implications for the generation of diamond-forming fluids. Geochimica et Cosmochimica Acta. 2019. Vol. 255, p. 69-87. DOI: 10.1016/j.gca.2019.03.025
- Klein-BenDavid O., Logvinova A.M., Schrauder M. et al. High-Mg carbonatitic microinclusions in some Yakutian diamonds – a new type of diamond-forming fluid. Lithos. 2009. Vol. 112. S. 2, p. 648-659. DOI: 10.1016/j.lithos.2009.03.015
- Hammouda T., Keshav S. Melting in the mantle in the presence of carbon: Review of experiments and discussion on the origin of carbonatites. Chemical Geology. 2015. Vol. 418, p. 171-188. DOI: 10.1016/j.chemgeo.2015.05.018
- Kogiso T., Hirschmann M.M., Pertermann M. High-pressure Partial Melting of Mafic Lithologies in the Mantle. Journal of Petrology. 2004. Vol. 45. N 12, p. 2407-2422. DOI: 10.1093/petrology/egh057
- Shatsky V.S., Zedgenizov D.A., Ragozin A.L., Kalinina V.V. Diamondiferous subcontinental lithospheric mantle of the northeastern Siberian Craton: Evidence from mineral inclusions in alluvial diamonds. Gondwana Research. 2015. Vol. 28. Iss. 1, p. 106-120. DOI: 10.1016/j.gr.2014.03.018