Localization and involvement in development of residual recoverable reserves of a multilayer oil field
- 1 — Ph.D. Head of Department Almetyevsk State Technological University “Petroleum High School” ▪ Orcid
- 2 — Ph.D. Deputy Head of Department PAO TATNEFT ▪ Orcid
- 3 — Ph.D. Associate Professor Empress Catherine ΙΙ Saint Petersburg Mining University ▪ Orcid
- 4 — Ph.D. Deputy General Director OOO “RTC NEFTEGAZDYNAMIKA” ▪ Orcid
- 5 — Ph.D. Leading Researcher Almetyevsk State Technological University “Petroleum High School” ▪ Orcid
- 6 — Head of Department AO “Konsaltingovyi Tsentr” ▪ Orcid
- 7 — Head of Department PAO TATNEFT ▪ Orcid
Abstract
During waterflooding of a multilayer oil field there is a constant deterioration of the structure and composition of residual reserves due to geological and technological reasons. The largest share of residual reserves is localized in pillars, which arise from uneven development of the production facility and are undrained or poorly drained zones. The results of a quantitative assessment of the distribution of residual oil reserves in the Middle and Upper Devonian deposits of the Romashkinskoe oil field of the Republic of Tatarstan are presented. A retrospective method is proposed to identify reserves by analyzing and summarizing historical exploration data and the long history of reservoir development, and a calculation algorithm is proposed to quantify them. It has been established that residual oil reserves are localized in rows of dividing and injection wells, as well as in the central rows of producing wells in a three-line drive, in abandoned and piezometric wells, in the areas adjacent to the zones of reservoir confluence, pinch-out, oil-bearing contours, distribution of reservoirs with deteriorated porosity and permeability properties. Depending on geological conditions, algorithms for selecting geological and technical measures to include localized reserves in development and forecasting production profiles were proposed. According to the proposed method, residual recoverable reserves were identified and a number of wells were recommended for experimental works on their additional recovery: in well 16 (hereinafter in the text, conventional well numbers are used) after isolation of overlying high-water-cut formations, the additional perforation was carried out and oil flow was obtained. Additional perforation in well 6 resulted in oil recovery during development as well. Thus, the developed approaches to identifying residual recoverable reserves and patterns of their spatial distribution can be recommended in other multilayer oil fields with a long history of development.
Introduction
The multilayer field includes dozens of oil deposits in reservoirs of different age, composition, properties, hydrodynamic connectivity and reservoir depth. The largest deposits represent independent single- or multilayer natural reservoirs with their own exploration and development systems, but even with their help it is not possible to fully explore and account for all types of geological heterogeneity. For this reason, during long-term waterflooding of fields, residual oil reserves are inevitably formed in stagnant areas not covered by the displacement process, remaining behind the water-oil displacement front, often referred to as pillars [1, 2]. Undrained or poorly drained reserves may also be formed in low-permeability interlayers [3]. Various methods are used to determine residual recoverable reserves (RRR). They can be divided into direct and indirect. Direct methods include seismic, geophysical, hydrodynamic and geochemical well testing technologies. Vertical seismic profiling is carried out to predict oil and gas prospectivity of local zones, for further appraisal and identification of new hydrocarbon deposits [4], etc. Pulse neutron-neutron and carbon-oxygen logs (C/O log) [5] are used to determine the current oil saturation in production wells. Methods of pulse-code interference testing allow for the assessment of RRR in the environment of the wells surveyed [6, 7]. Geochemical methods include the method of determining the optical properties of oil in the ultraviolet, visible and near-infrared radiation to estimate the amount of residual oil reserves and their spatial arrangement [8]. A similar task of localization and quantitative assessment of residual recoverable reserves of oil is solved by monitoring changes in the component composition of associated petroleum gas as part of a neural network forecast of reserves in the interwell space [9]. Indirect methods for determining the RRRs include the neural network approach described above and retrospective analysis of historical data from geological exploration and development of the field [9, 10].
The resulting layer-by-layer and areal uncertainty in the distribution of RRR makes it difficult to plan geological and technological measures (GTM) to incorporate them into development. The complex of such geological and technological measures (GTM) may consist of a simple reperforation or additional perforation of missed intervals in individual wells (simple optimization). New drilling, sidetrack drilling combined with bottom-hole treatment (BHT) or hydraulic fracturing (HF) (integrated optimization) can be used to involve large pillars into production.
The object of the study is a Devonian terrigenous natural reservoir of one of the areas of the Romashkinskoe oil field, which includes reservoir systems of the Eifelian, Givetian and Frasnian stages. The field has been developed for more than 70 years using various types of waterflooding. Strong heterogeneity and irregularity of reservoirs contributed to the formation of residual reserves. The aim of the work is to develop and test the method of identification and localization of RRR, study the regularities of their spatial location in relation to the waterflooding system used in the field, and develop algorithms for selecting and evaluating the effectiveness of GTM.
Patterns of formation and methods of studying residual reserves
Many technological and geological factors contribute to the formation of RRR in a multilayer field. In wells that penetrate high- and low-permeable formations with a single filter, productive intervals with high filtration properties are usually drained [11, 12]. A complicating factor in the process of oil reserves development is also the presence of various types of cavernosity within the deposit [13]. The injectivity profile turns out to be extremely uneven, injectivity of low-permeability interlayers is often zero. Waterflooding in low-permeability reservoirs contributes to the formation of pillars, which leads to a decrease in the final oil recovery factor [14]. Uneven reservoir pressure within an oil field with complex mining and geological conditions can be associated with variability of formation-pressure conductivity, which also leads to different rates of reserve recovery [15]. Areas of undeveloped reserves arise due to the formation of linear filtration channels between production wells when the direction of filtration flows changes [16]. In all these cases, low-permeable formations are not stimulated and significant RRR are formed in them. Areas of sharp decrease or increase in thickness, irregular rise of oil-water contact (OWC) are often excluded from the drainage process due to the rapid contraction of the inner oil-water contact in combination with the high relative viscosity of the oil. RRR can be formed in the edge areas of oil-water zones (OWZ), oil-water contacts and watering cones [17]. The formation of pillars is facilitated by the deterioration of properties of both oil and rocks in the bottomhole zone of producing wells, high water cut of extracted products, aging of well stock, low rates of extraction of hard-to-recover reserves at the initial stages of development [18]. Formation of RRR occurs due to anthropogenic disturbance of the reservoir, for example, deterioration of porosity and permeability properties when water with a temperature lower than the reservoir temperature is injected into the formation. Viscosity of formation oil may increase [19, 20], crystallization of paraffins, deposition of resins and asphaltenes in the porous medium may occur [21, 22], which leads to changes in oil composition, recorded, for example, using X-ray tube and three-layer perceptron [23]. When mixing injected and reservoir water, salt deposits are often observed, which also worsen the reservoir properties [24]. The formation of RRR is facilitated by behind-casing and inter reservoir flows, water breakthroughs through thin shale barriers, zones of deteriorated reservoir properties or formations confluence. The condition for maintaining current production levels and selective recovery of residual reserves from the pillars of conditioned reservoirs, reservoirs with reduced permeability and previously unaccounted for oil-saturated intervals is their localization, which consists in geometrization of their spatial position in the structure of the natural reservoir under deve-lopment [25, 26].
The methods of RRR exploration are constantly improving – production logging test (PLT), hydrodynamic and interpretation tests, seismic, lithofacies, modeling and complex methods [8]. PLT and hydrodynamic testing of wells are devoted to the historical study of crossovers that contribute to the formation of pillars [27]. In dispersed waterflood systems, different approaches to assessing the shape and size of residual pillars and types of pressure interference tests are used. Hydrodynamic testing allows revealing the degree of hydrodynamic connectivity of wells in the formation and various types of geological barriers in the interwell space, to estimate the displacement coverage factor of oil reservoirs drained by a regular well network. Numerical methods, algorithms and programs for processing hydrodynamic well measurements are being developed for stable estimation of reservoir properties in local areas [28]. Processing of data obtained during the study of the process of formation reserves development allows to determine the current location of OWC, outer and inner OWC and the washed out area of the reservoir. Direct methods of control of oil reserves movement include observations of well operation modes, dynamics of watering of extracted product, electrometric and radiometric methods of well log surveys. Seismic data are used to describe the interwell space, geometry and morphology of sedimentary bodies by modeling the conditions and environment of sedimentation, reservoir properties in three-dimensional space [29]. Lithological and facial modeling is used in conjunction with 3D modeling of complex sand bodies of alluvial or coastal-marine type, as well as carbonate structures of various types, since in these cases an understanding of the depositional environment is necessary. Borehole seismology, including vertical seismic profiling, are used to differentiate terrigenous sediments by degree of clay content (sandiness), which is necessary to predict zones of sandstone distribution with improved reservoir properties [30]. Seismic methods are used to study the density distribution of carbonate sediments, which makes it possible to identify intervals of increased fracturing. The concept of digital core modeling technology is used to determine the terrigenous reservoirs properties for the purpose of modeling pore space and filtration processes in problems of estimating geological oil reserves [31]. Application of the presented methods in the developed fields helps to build refined geologic models and identify residual previously undrained or unknown oil pillars. The use of complex characteristics of heterogeneity, taking into account the variability of formations characteristics, makes it possible to group individual geological objects. Identification of separate formations and deposits, thus, allows increasing the efficiency of reservoir management [32].
Quantitative assessment of residual reserves is carried out based on actual data obtained during the exploration process. To calculate reserves, depending on geological conditions, various methods are used – volumetric, loss of reservoir pressure, dissolved gas, material balance, statistical, etc. There is also a possibility that the estimated oil reserves may not be confirmed. Therefore, it is necessary to assess the risks and strategic sustainability of the project for additional production and extraction of residual oil [33].
Object of study
The geological structure of one of the areas of the Romashkinskoe oil field in the Republic of Tatarstan includes sedimentary rocks of the Devonian, Carboniferous and Permian systems [34]. One of the first in the field was the development of Devonian formations in the 1950s, which included several productive oil-bearing horizons. Residual oil reserves were localized and quantified in them. In the Pashiy D1 horizon there are nine productive formations, which are united into two consolidated benches: the top bench, including formations a, b1, b2, b3, v, which are currently under active development; the bottom bench – formations g1, g2 g3+d. The development of the area started from the bottom bench. Despite significant watercutting of the extracted products, it contains significant residual reserves. Oil-bearing formations of the Mullin D2, Ardatov D3 (formations a, b, v), Vorobiev D4 (a, b) and Biysk D5 horizons lie lower in the section. Formations D1 and D2 form a single hydrodynamic system, in which the OWC is provided at levels of 1485-1486 m (the values are specified in modulus), confined to the lower part of the D1d formation, and is less frequently isolated in the upper part of the D2 formation. In Vorobiev D4 horizon, formations a, b form a system with OWC within 1525-1528 м. In Biysk D5 horizon, the OWC is established in rare wells below 1565 m (see Table). Thus, well 31 shows a system of Devonian formations, D1 and D2 are expressed by oil-bearing sandstones with the OWC at the mark of 1501 m, D3 and D4 are composed of water-saturated sandstones (Fig.1). According to the general development project, the study area is divided by rows of injection wells into blocks 1-8, within which, due to strong facies variability of rocks, a focal flooding system was implemented, and over time, the external dividing ring and rows of dividing injection wells ceased to exist. Water flooding in the area is carried out by individual injection wells representing relics of block and focal systems.
Markings of oil-water contact by formations
Horizon |
Index |
Mark, m |
|
Justified |
Variation interval |
||
Pashiy, Mullin |
D1, D2 |
1485-1486 |
1479-1496 |
Ardatov |
D3 |
1525-1528 |
1524-1539 |
Vorobiev |
D4 |
1530-1536 |
1528-1542 |
Biysk |
D5 |
1565 |
|
Research methods
The retrospective method was used to identify RRR, which consisted in the analysis and generalization of historical data on exploration and long-term development history of the studied formations. For this purpose, the current state, perforations, monthly operational reports (MOR), and logging information for all categories of wells, including abandoned and piezometric wells, were analyzed in available information systems. A total of 2,605 wells were analyzed. Each of the reservoir formations with RRR was assigned a category from 1 to 6. Category 1 included reserves in previously unexplored formations or individual intervals of missed conditional reservoirs lying above the OWC. For example, category 1 includes reserves of the D2 formation for the well shown in Fig.1. Category 2 includes reserves of formations with increased content of shale and siltstone material (more than 0.2) [35], with lower values of oil saturation factor (less than 0.6), as well as those identified below the justified OWC. Basing on previously developed formations, well MORs are analyzed to identify their prospectivity.
These formations could be shutoff with water cut values of less than 98 % [36] and at sufficiently high flow rates (assigned to category 3). Category 4 includes reserves of formations currently being developed. Category 5 includes developed formations in production wells and injection facilities in injection wells. Water-bearing formations, non-reservoirs and zones of pinch-out belong to the unpromising category 6 (formations g-d, D3 and D4 in the well shown in Fig.1) [10].
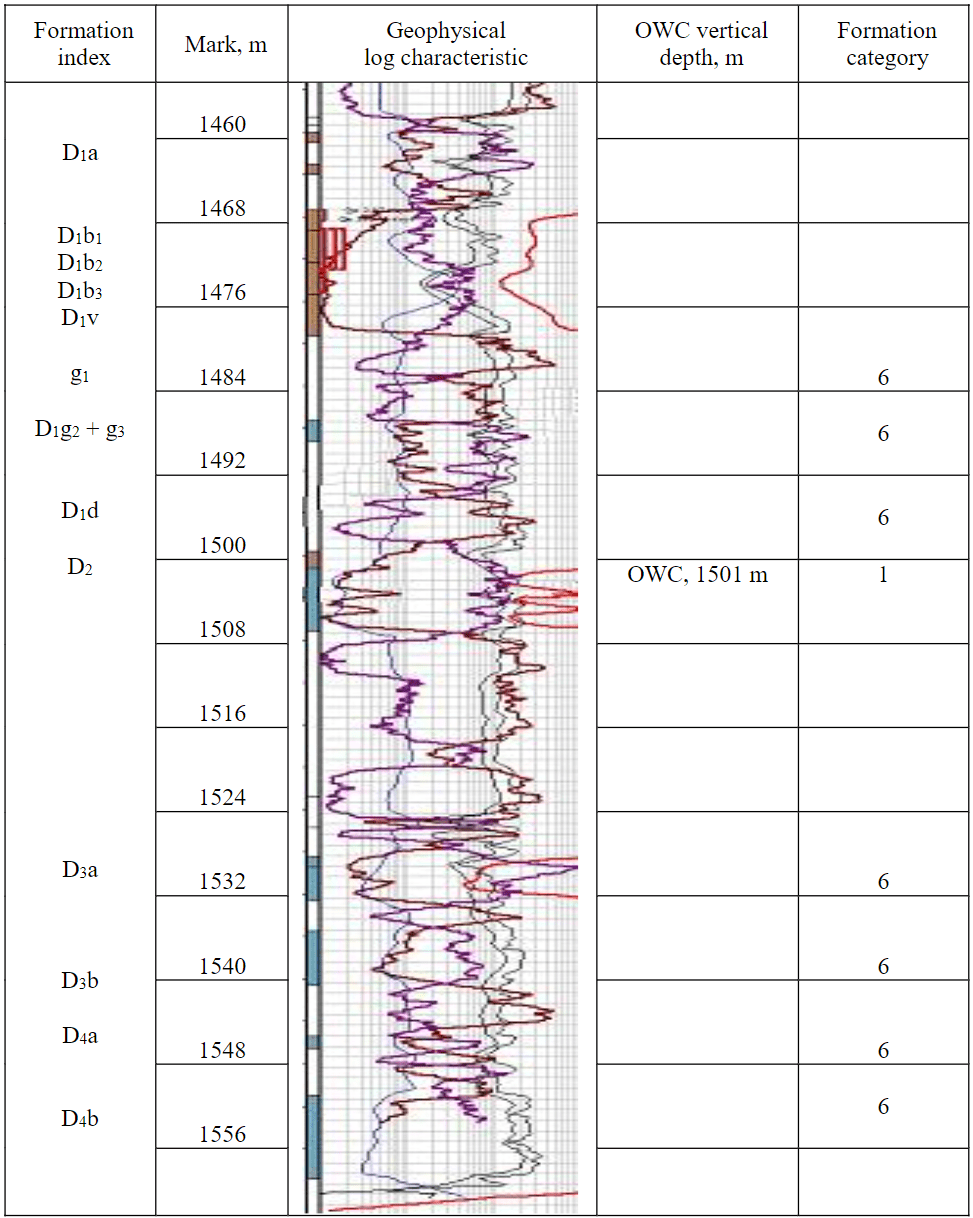
Fig.1. Devonian formations system and OWC determination in the Mullin horizon
In order to quantify the residual reserves, select geological and technological measures for involvement in the development and forecast production profiles, the method of algorithms was applied. These algorithms present a sequence of developed instructions and standard rules to effectively achieve the set goals, which is especially important when analyzing a bulk of historical well data. Thus, quantitative assessment of residual reserves was performed by the volumetric method based on the collected actual data on the filtration area (assumed equal to the Voronoi cell), capacitive characteristics of rocks and properties of residual oil according to the algorithm presented in Fig.2 [37]. The missing information was filled in by analogies with neighboring wells located in the same structure-facies zone with the analyzed one. Total reserves of localized zones are determined by simple summation of reserves by wells. The calculated reserves were plotted on maps of reserve categories and sizes. The maps were constructed separately for each reservoir to be analyzed together with lithological maps to identify patterns of spatial distribution of RRR.
For each category of RRR, we propose an algorithm for selecting GTM depending on the type of reserves localization. For reserves identified in individual wells, simple optimization methods are used including additional perforation and reperforation of formations. For pillars involving several wells, complex optimization methods are used (Fig.3). When selecting GTM the values of initial and residual reserves, cumulative oil production Qcum, presence of watered intervals in the wells, current technical condition of the well and the necessity to perform wireline (WL) and workover (WO) operations are taken into account. Due to their low prospects, wells with category 5 reserves are assessed as candidates for sidetracking in order to involve localized RRRs identified in the vicinity into development. Threshold values X and Y (Fig.3) for RRR are set by GTM and technological departments of oil and gas producing companies.
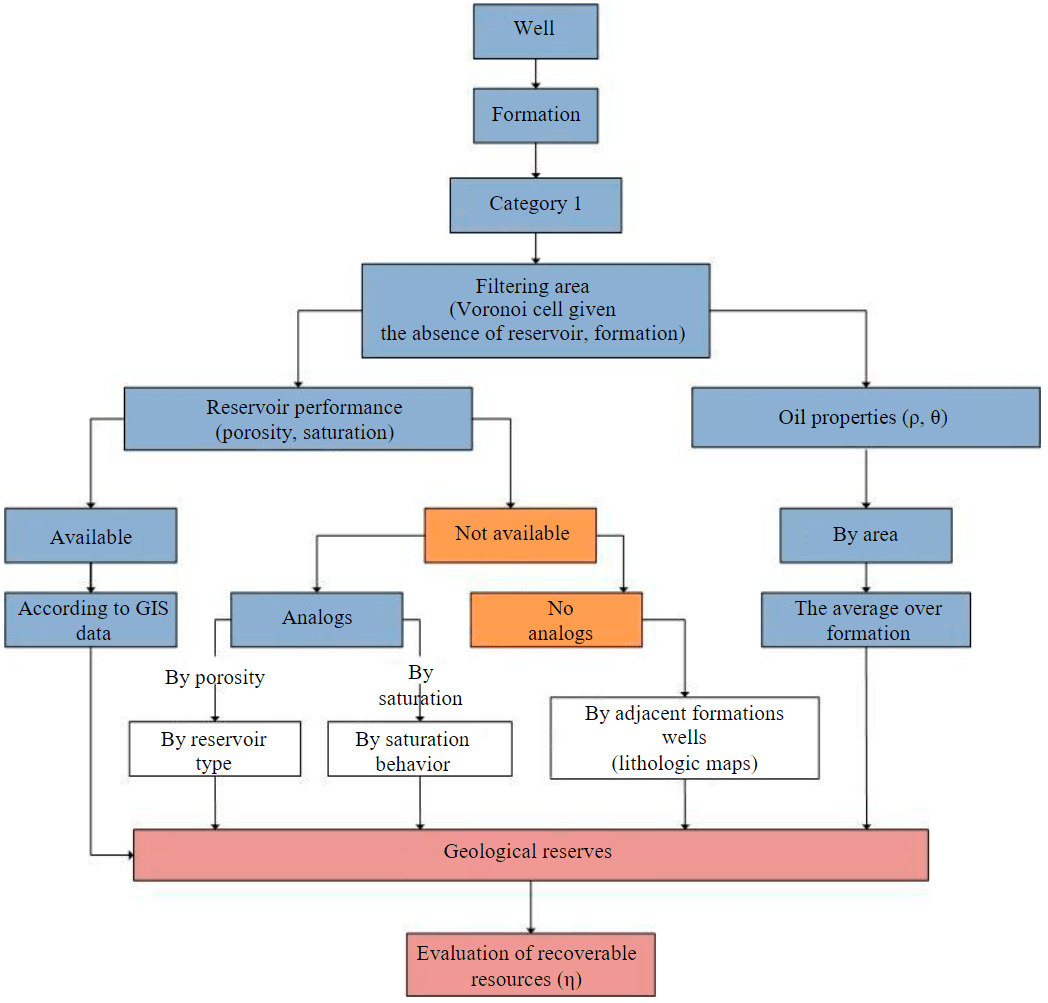
Fig.2. Algorithm of RRR calculation
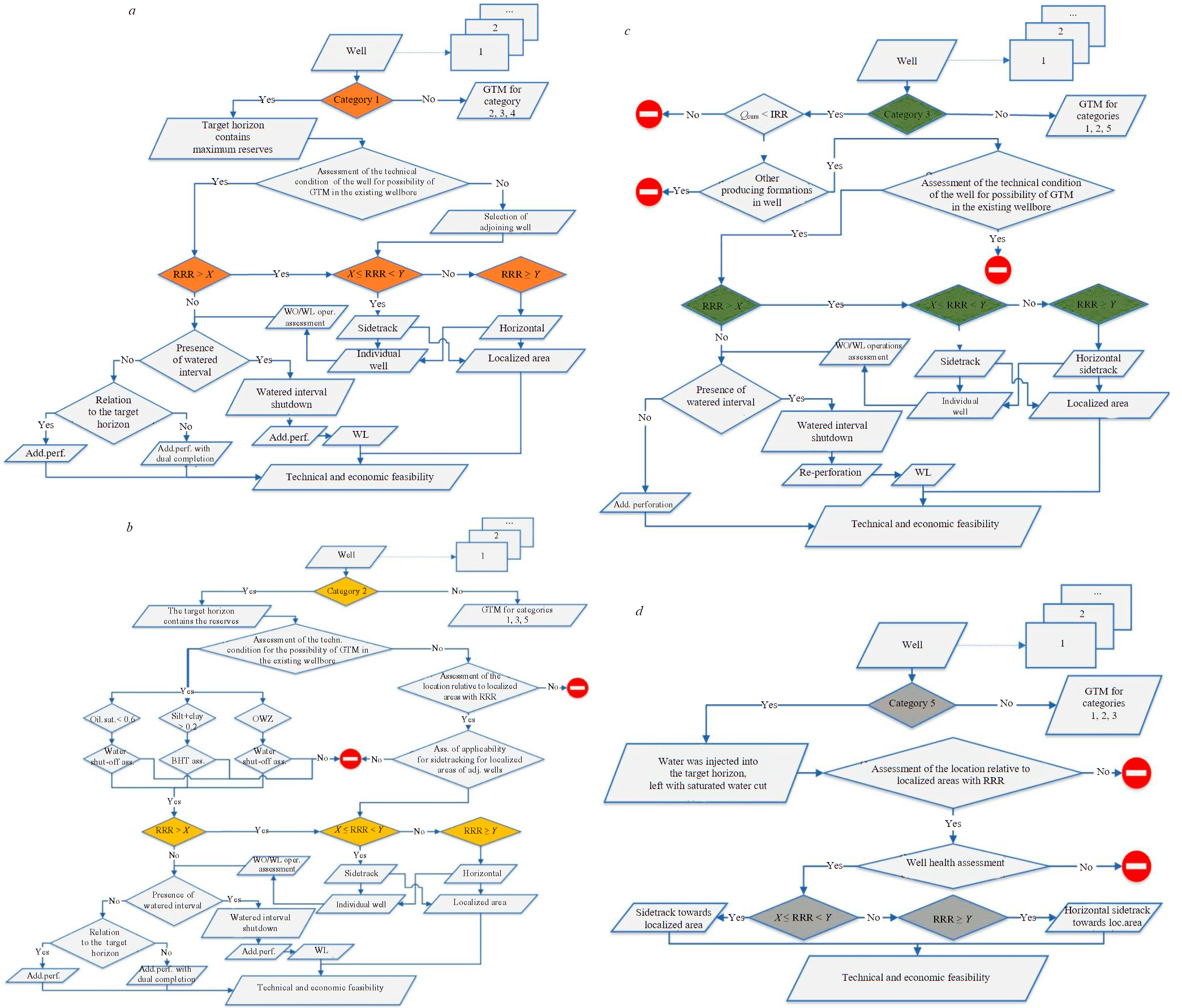
Fig.4. Algorithm for predicting production profiles
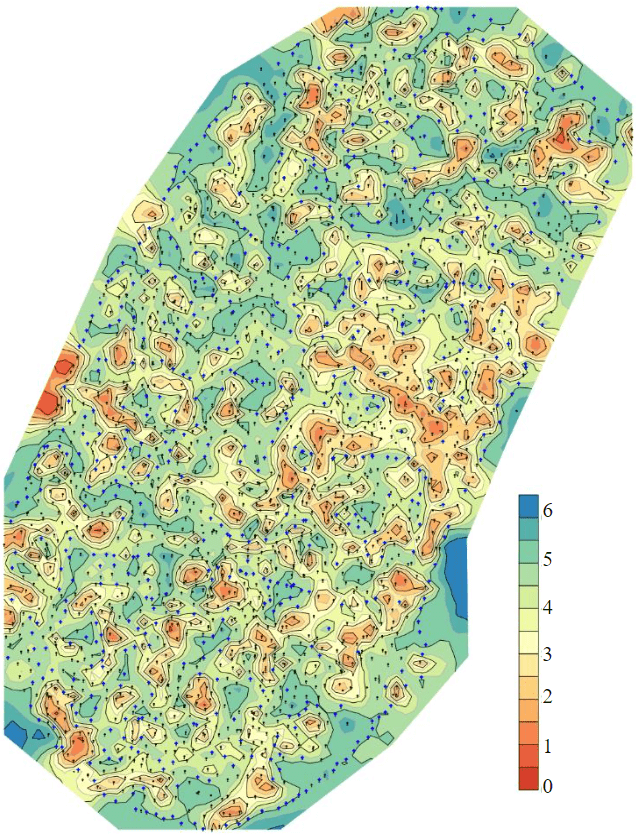
Fig.5. Category map for g2 formation
To forecast the production profile, the Arps method was used, which consists in constructing production decline curves based on historical data to approximate them for the forecast period. In order to effectively apply the method, an algorithm for forecasting production profiles is proposed (Fig.4). To construct a production decline curve the availability of actual historical data on the performance of formations in a well is assessed, and in case of their absence, for example in injection or abandoned wells, the possibility of selecting analogous wells.
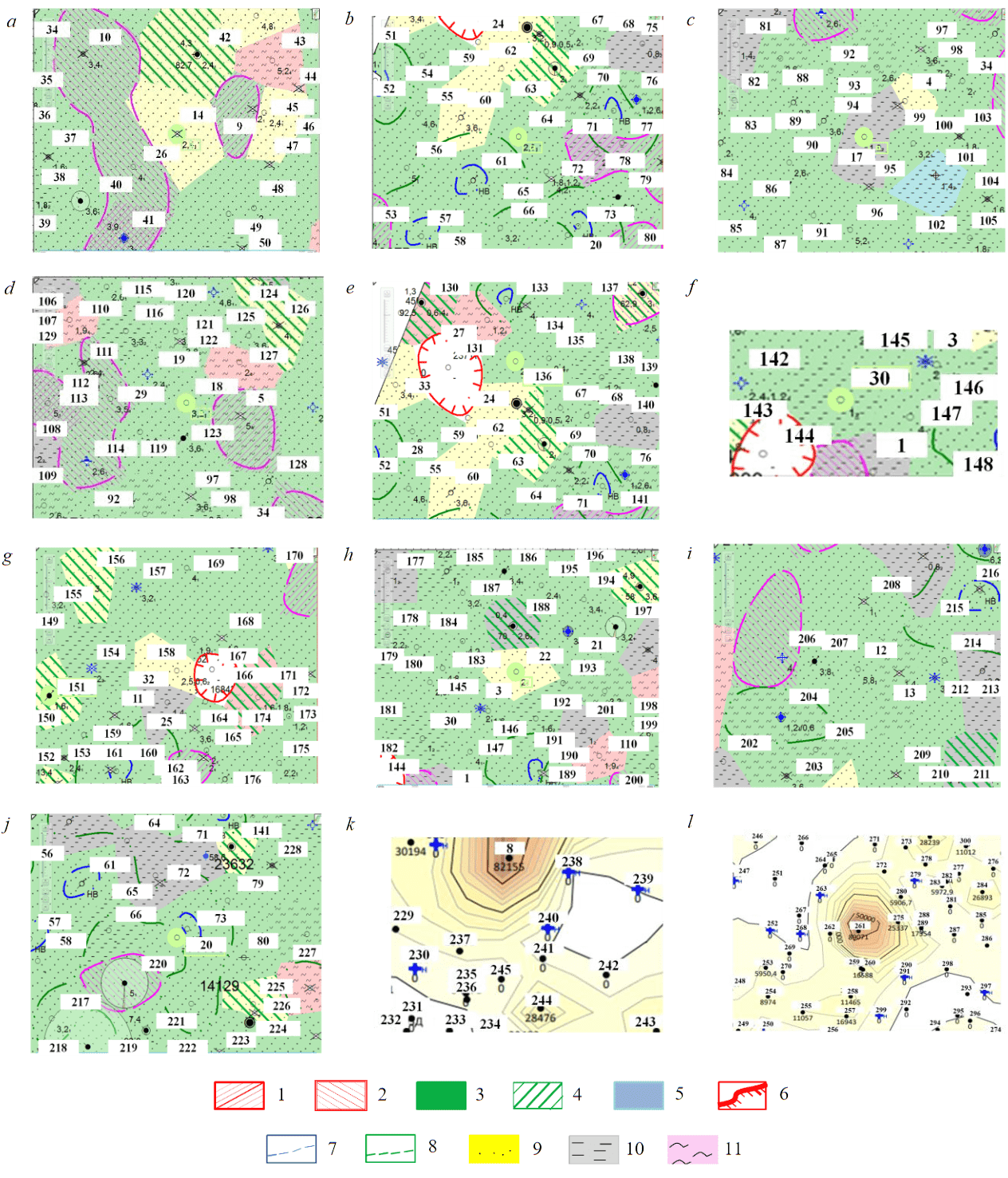
Fig.6. Regularities of spatial distribution of RRR
1, 2 – zones of junction with the formation: 1 – upper, 2 – lower; 3-5 – waterflooding: 3 – with injected water, 4 – fractional, 5 – with formation water; 6 – pinch-out line; 7, 8 – oil-water contact: 7 – outer, 8 – inner; 9-11 – lithotypes: 9 – sandstone, 10 – siltstone, 11 – clayey sandstone
Discussion of results
The identified residual reserves by area exceeded the average annual production of PAO TATNEFT and distributed among formations as follows, %: g1 – 19.53; g2 – 23.33; g3 – 22.03; d – 19.26; D2 – 4.37; D3a – 4.23; D3b – 3.12; D3v – 2.43; D4a – 1.36; D4b – 0.16. The largest share of reserves, over 96 % belongs to category 1. By blocks, taking into account total reserves of borderline wells, %: 1 – 13.82; 2 – 13.16; 3 – 18.57; 4 – 12.40; 5 – 14.15; 6 – 12.05; 7 – 12.03 and 8 – 3.82. The greatest prospects for detection and geological confirmation of RRR in the area are associated with block 3, and through the section with D1 g-d, formations even despite their high depletion and water cut. On the category map, which gives an areal representation of the distribution of RRR in g2 formation, localized RRR zones in category 1 are shown in brown, and depleted and unproductive areas are shown in blue (Fig.5).
Many factors influenced the formation and localization of the RRR in the study area. Since oil-bearing reservoirs below the justified OWC may not have been perforated at all, they may still contain significant residual reserves. For example, in well 31 in the D2 formation, the reserves of which are classified as category 1, the OWC was identified at 1501 m (see Fig.1), which is significantly lower than the average justified OWC level (see Table). The distribution of residual oil saturation is influenced by well spacing and density. Residual pillars formed in central row of producing wells in a three-line drive, between wells in producing rows and injection and adjacent producing rows, in the central parts of multi-row systems, farthest from the dividing rows, especially in low-permeability clayey or calcareous, poorly drained areas [38]. Another type of residual oil saturation formed due to the insufficient impact of injection wells on the reservoir. In case of a wide injection wells spacing, there is incomplete horizontal sweep of the reservoir due to the need to maintain a reservoir pressure gradient [39]. The discontinuity of such reservoirs also leads to a violation of the direct relationship between the amount of fluid coming from a separate interlayer and its conductivity. The following regularities in the spatial distribution of residual reserves have been revealed (Fig.6):
- In the zone of formations junction RRRs are formed due to their insufficient involvement in the drainage process (Fig.6, a). Thus, in well 14, significant RRR were identified in the g2 formation, represented by sandstones in the area between the zone of junction of the formation with the upper g1 (well 9) and lower g3 formations (wells 26 and 10).
- In the areas adjacent to the inner oil-water contact, RRR are formed when the outer oil-water boundary advances (Fig.6, b). In well 64 in the sandstone g2 formation, adjacent to the inner oil-water contact, the RRR were justified and recommended for involvement in development.
- In reservoirs with deteriorated properties, residual reserves arise due to advanced oil displacement from interlayers and areas with improved reservoir properties (Fig.6, c). A significant residual reserves zone is localized in the area of wells 17 and 4 (g2 formation), and in well 17 the formation is composed of siltstones with deteriorated reservoir properties.
- In areas adjacent to junction zones of reservoirs near injection wells (Fig.6, d), the formation of RRR may be associated with uneven distribution of the displacing agent across formations. In well 18 (sandstone formation g2), residual reserves were detected between the zones of junction with the upper (in well 29) and lower (in well 5) formations. The area is adjacent to injection well 19 but has not been waterflooded because g2 formation in well 18 was not perforated. It can be assumed that the injected water from well 19 flowed into the neighboring zones of reservoir junction.
- In the areas adjacent to the pinch-out line (Fig.6, e), the formation of residual reserves is associated with the presence of a hydrodynamic barrier. For example, g2 formation in wells 24, 28 and 33 is composed of sandstones and is directly adjacent to the fringe zone of reservoir, while in well 27 it is composed of clayey sandstone. A similar example is given for g2 formation in wells 1, 30 and 142 (Fig.6, f). It should be noted that the reserves in the fringe zone (wells 24, 28, 33) are an order of magnitude higher than the reserves in the localized zone (wells 1, 30, 142).
- The area with RRR, adjacent to the pinch-out line near the inner oil-water contact, arises under influence of two unfavorable factors of hydrodynamic screening and uneven contraction of the oil-water contact (Fig.6, g). A group of wells containing residual reserves in the g2 formation, including wells 25, 32 and 166, is illustrated. In well 11, the formation is adjacent to the inner oil-water contact and additional RRRs were noted.
- In the producing row (Fig.6, h), RRRs are formed when there is no formation pressure gradient between the bottoms of neighboring wells. In well 22, located between injection wells 21 and 3, reserves were found in the g2 formation. The g2 formation was not perforated in the well, so the draw down pressure necessary for the injected water front to advance towards it along the formation was not created.
- Formation of RRR occurs in the oil and oil-water zones, in the reservoir with deteriorated properties (Fig.6, i). In wells 12 and 13 in the clayey sandstone layer, residual reserves were identified in the oil zone of the formation. Well 12 contains more than 80 % of the reserves identified in the area, and well 13 contains about 20 %. In well 20 the reserves are concentrated in the water-oil zone of the g3 formation (Fig.6, j).
- In the dividing row (Fig.6, k), RRRs may occur if there is no formation pressure gradient between neighboring injection wells. The reserves in well 8 were found in the g2 formation, which has not been perforated before.
- In central rows of producing wells in a three-line drive, RRRs are formed due to insufficient influence of injection wells in the absence of a formation pressure gradient (Fig.6, l). In producing well 261 the g2 formation was not perforated and significant residual oil reserves are substantiated.
The purpose of identifying RRR is their further inclusion in development to increase the oil recovery factor [40] based on forecasting production profiles [41]. Various technical solutions have been proposed, such as horizontal sidetrack well drilling or sidetracking [42, 43], hydraulic fracturing [44, 45], dual string production and dual injection [46].
Conclusion
Identification of residual oil reserves in a multilayer field with a long history of development is the most important condition for selecting effective GTM in order to involve them in development and increase the oil recovery rate. There are no generally accepted ideas about the nature of residual oil distribution in water-flooded formations. Construction of a map of total residual reserves for all layers of a multilayer object, taking into account the retrospective analysis of the drilled well stock, regardless of their purpose, allowed to identify the most promising wells for the selection of effective technologies for stimulation of production and enhanced oil recovery, planning the sequence of GTM. The work proposes algorithms for classifying residual reserves by category, and constructs maps of categories and residual reserves by layers. It has been established that RRR localize in the dividing rows of injection wells, in central rows of producing wells in a three-line drive, in abandoned production, injection and other wells, often in the areas adjacent to the zones of reservoir confluence, pinch-out, oil-water contacts, distribution of reservoirs with deteriorated reservoir porosity and permeability properties.
The variants of geological and technical measures for the purpose of recovery of residual reserves by categories and an algorithm for forecasting production profiles according to Arps are proposed. Simple optimization methods include additional perforation and re-perforation of formations, if necessary in combination with various types of well workovers. However, maintaining production levels and achieving high final oil recovery occurs through the improvement and implementation of technologies.
Geological and technical activities are associated with difficulties related to well wear during long-term operation. Residual reserves are often predicted in wells, but their inclusion in development is hampered by the technical condition of the wellbore, production string or casing annulus. Test surveys began in well 16, where the current production target is b2 formation. In the well, the proposed algorithm revealed significant category 1 RRRs in the g2 formation with a net oil thickness of 2.79 meters. The following interventions were carried out: the overlying highly watered a and b2 formations were isolated using a packer; the underlying g2 formation, from which the initial oil influx was obtained, was completed. Next steps to stimulate the resulting influx are planned. The 4.88 m thick D3b+v formation was additionally perforated in idle well 6. The reservoir was developed by swabbing, oil was recovered, work continues. As part of the work presented herein, work is continuing on the development of GTM to involve the identified RRR into development with simultaneous forecasting of production profiles to assess their technical and economic efficiency.
The developed algorithms and the revealed patterns in the spatial distribution of RRR of the studied area can be applied to the well stock of other areas of the Romashkinskoe oil field, which includes tens of thousands of units. The peculiarities of the field are a large number of wells and formations with a long history of development, which require a comprehensive retrospective analysis and consideration of the influence of geological conditions on the selection of technologies. The proposed algorithms, which include a typical sequence of operations to identify RRR and select technologies for their inclusion in development, greatly facilitate the work of a field engineer.
References
- Strakhov P.N., Davydova E.A., Skachek K.G., Eremin Yu.G. Complexing information about different heterogeneity in the geological modeling of oil and gas deposits. Oil Industry Journal. 2020. N 7, p. 82-87 (in Russian). DOI: 10.24887/0028-2448-2020-7-82-87
- Chudinova D.Yu., Zakirova E.A., Yanova A.V. The geological heterogeneity its influence on development of residual reserves of oil of layer. Oil and gas technologies and new materials. Problems and solutions: collection of scientific papers. Ufa: Monografiya, 2018. Vol. 7 (12), p. 5-11 (in Russian).
- Podoprigora D., Byazrov R., Sytnik J. The Comprehensive Overview of Large-Volume Surfactant Slugs Injection for Enhancing Oil Recovery: Status and the Outlook. Energies. 2022. Vol. 15. Iss. 21. N 8300. DOI: 10.3390/en15218300
- Lenskii V.A., Zhuzhel A.S., Sharafutdinov T.R. Modern condition of a well seismic survey (WSS) in Russia. Geology, geophysics and development of oil and gas fields. 2019 N 1, p. 29-36 (in Russian). DOI: 10.30713/2413-5011-2019-1-29-36
- Mashkin K.A., Korotchenko A.G., Glukhov V.L. et al. Experience of Neutron Lifetime Log in the Estimating of Current Oil Saturation in Wells at Deposits of ООО Bashneft-Dobycha. Neft. Gas. Novacii. 2019. N 2, p. 32-37 (in Russian).
- Ganiev B., Lutfullin A., Karimov I. et al. Mature Fields Oil Production Enhancement by Pulse Code Testing. SPE Annual Caspian Technical Conference, 15-17 November 2022, Nur-Sultan, Kazakhstan. OnePetro, 2022. N SPE-212156-MS. DOI: 10.2118/212156-MS
- Sabzabadi A., Masoudi R., Arsanti D. et al. Verifying Local Oil Reserves Using Multi-Well Pressure Pulse Code Testing. Offshore Technology Conference Asia, 20-23 March 2018, Kuala Lumpur, Malaysia. OnePetro, 2018. N OTC-28601-MS. DOI: 10.4043/28601-MS
- Burkhanov R.N., Lutfullin A.A., Ibragimov I.I., Maksyutin A.V. Estimation and Localization of Residual Recoverable Oil Reserves by the Complex of Filtration, Optical and Field Research. SPE Russian Petroleum Technology Conference, 26-29 October 2020. OnePetro, 2020. N SPE-201914-MS. DOI: 10.2118/201914-MS
- Sudakov V.A., Safuanov R.I., Kozlov A.N. et al. Localization and development of residual oil reserves using geochemical studies based on neural network algorithms. Georesources. 2022. Vol. 24. N 4, p. 50-64 (in Russian). DOI: 10.18599/grs.2022.4.4
- Burkhanov R.N., Lutfullin A.A., Maksyutin A.V. et al. Retrospective analysis algorithm for identifying and localizing residual reserves of the developed multilayer oil field. Georesources. 2022. Vol. 24. N 3, p. 125-138 (in Russian). DOI: 10.18599/grs.2022.3.11
- Palyanitsina A., Safiullina E., Byazrov R. et al. Environmentally Safe Technology to Increase Efficiency of High-Viscosity Oil Production for the Objects with Advanced Water Cut. Energies. 2022. Vol. 15. Iss. 3. N 753. DOI: 10.3390/en15030753
- Duryagin V., Nguyen Van Thang, Onegov N., Shamsutdinova G. Investigation of the Selectivity of the Water Shutoff Technology. Energies. 2023. Vol. 16. Iss. 1. N 366. DOI: 10.3390/en16010366
- Martyushev D.A., Galkin V.I., Ponomareva I.N. Study of regularities of distribution of filtering properties within complexly constructed carbonate reservoirs. Bulletin of the Tomsk Polytechnic University. Geo Аssets Engineering. 2021. Vol. 332. N 11, р. 117-126 (in Russian). DOI: 10.18799/24131830/2021/11/3069
- Chen X., Paprouschi A., Elveny M. et al. A laboratory approach to enhance oil recovery factor in a low permeable reservoir by active carbonated water injection. Energy Reports. 2021. Vol. 7, p. 3149-3155. DOI: 10.1016/j.egyr.2021.05.043
- Galkin S.V., Krivoshchekov S.N., Kozyrev N.D. et al. Accounting of geomechanical layer properties in multi-layer oil field development. Journal of Mining Institute. 2020. Vol. 244, p. 408-417. DOI: 10.31897/PMI.2020.4.3
- Grachev S.I., Korotenko V.A., Kushakova N.P. Study on influence of two-phase filtration transformation on formation of zones of undeveloped oil reserves. Journal of Mining Institute. 2020. Vol. 241, p. 68-82. DOI: 10.31897/PMI.2020.1.68
- Poletaeva I.A., Rybichev A.A., Terekhina N.M. Development of the scheme for residual oil recovery from highly watered reservoirs. Experience, actual problems and prospects of oil and gas complex development: Materialy XI Mezhdunarodnoi nauchno-prakticheskoi konferentsii obuchayushchikhsya, aspirantov i uchenykh, posvyashchennoi 40-letiyu filiala TIU v g. Nizhnevartovske, 22 aprelya 2021, Nizhnevartovsk, Russia. Tyumen: Tyumenskii industrialnyi universitet, 2021, p. 145-147 (in Russian).
- Zingel E.M. Residual Oil: Challenges and Procedures. Neft. Gas. Novacii. 2012. N 6 (161), p. 71-74 (in Russian).
- Beloglazov I., Morenov V., Leusheva E., Gudmestad O.T. Modeling of Heavy-Oil Flow with Regard to Their Rheological Properties. Energies. 2021. Vol. 14. Iss. 2. N 359. DOI: 10.3390/en14020359
- Leusheva E., Brovkina N., Morenov V. Investigation of Non-Linear Rheological Characteristics of Barite-Free Drilling Fluids. Fluids. 2021. Vol. 6. Iss. 9. N 327. DOI: 10.3390/fluids6090327
- Nurgalieva K.S., Saychenko L.A., Riazi M. et al. Improving the Efficiency of Oil and Gas Wells Complicated by the Formation of Asphalt–Resin–Paraffin Deposits. Energies. 2021. Vol. 14. Iss. 20. N 6673. DOI: 10.3390/en14206673
- Korobov G.Y., Vorontsov A.A. Study of conditions for gas hydrate and asphaltene-resin-paraffin deposits formation in mechanized oil production. Bulletin of the Tomsk Polytechnic University. Geo Assets Engineering. 2023. Vol. 334. N 10, p. 61-75 (in Russian). DOI: 10.18799/24131830/2023/10/4181
- Mayet A.M., Alizadeh S.M., Nurgalieva K.S. et al. Extraction of Time-Domain Characteristics and Selection of Effective Features Using Correlation Analysis to Increase the Accuracy of Petroleum Fluid Monitoring Systems. Energies. 2022. Vol. 15. Iss. 6. N 1986. DOI: 10.3390/en15061986
- Baldenkova D.I., Shangaraeva L.A. Prevention of scale in the downhole equipment and the formation of Priobskoe oil field. Readings of A.I.Bulatov: Materials of I International scientific and practical conference, 31 March 2017, Krasnodar, Russia. Krasnodar: Izdatelskii dom – Yug, 2017. Vol. 2, p. 27-29 (in Russian).
- Yartiev A.F., Khakimzyanov N.N., Petrov V.N., Idiyatullina Z.S. Improving technologies for the development of oil reserves from heterogeneous and complex reservoirs of the Republic of Tatarstan. Kazan: Ikhlas, 2016, p. 192 (in Russian).
- Muslimov R.Kh. The New Classification of Reserves and Resources of Oil and Combustible Gas – Movement Onward or Backward? Georesources. 2016. Vol. 18. N 2, p. 80-87. DOI: 10.18599/grs.18.2.1
- Aslanyan A., Grishko F., Krichevsky V. et al. Assessing Waterflood Efficiency with Deconvolution Based Multi-Well Retrospective Test Technique. SPE Europec featured at 81st EAGE Conference and Exhibition, 3-6 June 2019, London, UK. 2019. N SPE-195518-MS. DOI: 10.2118/195518-MS
- Zhdanov I., Kotezhekov V., Margarit A. The Method of Localization of Residual Oil on the Basis of Complex Multi-Well Diagnostics and Calibration of the Hydrodynamic Model. SPE Russian Petroleum Technology Conference, 15-17 October 2018, Moscow, Russia. OnePetro, 2018. N SPE-191590-18RPTC-MS. DOI: 10.2118/191590-18RPTC-MS
- Egorov A.S., Prischepa O.M., Nefedov Y.V. et al. Deep Structure, Tectonics and Petroleum Potential of the Western Sector of the Russian Arctic. Journal of Marine Science and Engineering. 2021. Vol. 9. Iss. 3. N 258. DOI: 10.3390/jmse9030258
- Zaripov B.F., Ryzhov V.A. The Application of Machine Learning for Quality Assessment of Raw Field Data of Passive Seismic Surveys During the Hydrocarbon Deposit Exploration by LFS Technology. Neft. Gas. Novacii. 2020. N 3 (232), p. 11-15 (in Russian).
- Belozerov I.P., Gubaydullin M.G. Concept of technology for determining the permeability and porosity properties of terrigenous reservoirs on a digital rock sample model. Journal of Mining Institute. 2020. Vol. 244, p. 402-407. DOI: 10.31897/PMI.2020.4.2
- Mukhametshin V.Sh., Khakimzyanov I.N. Features of grouping low-producing oil deposits in carbonate reservoirs for the rational use of resources within the Ural-Volga region. Journal of Mining Institute. 2021. Vol. 252, p. 896-907. DOI: 10.31897/PMI.2021.6.11
- Cherepovitsyn A., Tsvetkova A., Komendantova N. Approaches to Assessing the Strategic Sustainability of High-Risk Offshore Oil and Gas Projects. Journal of Marine Science and Engineering. 2020. Vol. 8. Iss. 12. N 995. DOI: 10.3390/jmse8120995
- Muslimov R.Kh. On program of development of priority research studies in geological prospecting and reservoir engineering by independent oil companies for the period 2016-2015. Neftyanaya provintsiya. 2018. N 4 (16), p. 1-17 (in Russian). DOI: 10.25689/NP.2018.4.1-17
- Khusainov V.M. Increasing recoverable oil reserves at the late stage of development of a large oil field (theory, geological basis, practice): Avtoref. dis. … d-ra tekhn. nauk. Moscow: Nauchnyi tsentr nelineinoi volnovoi mekhaniki i tekhnologii RAN, 2011, p. 50 (in Russian).
- Rocheva E.V. Causes of premature flooding of wells and the importance of geologic factor in flooding control. The New Science: Experience, Tradition, Innovation. 2017. N 1-3 (123), p. 25-27 (in Russian).
- Akhmetyanova A.I., Mashkova E.A. Estimation of oil reserves by volumetric method. Oil and gas technologies and new materials. Problems and solutions: annual collection of scientific papers. Ufa: Monografiya, 2019. Vol. 8 (13), p. 54-58 (in Russian).
- Burkhanov R.N., Lutfullin A.A., Maksyutin A.V., Ibragimov I.I. Distribution and exploration of residual oil reserves in flooded formations. Achievements, problems and prospects for the development of the oil and gas industry: Materialy IV Mezhdunarodnoi nauchno-prakticheskoi konferentsii, 16-18 oktyabrya 2019, Almetevsk, Russia. Almetevsk: Almetevskii gosudarstvennyi neftyanoi institut, 2019, p. 42-48 (in Russian).
- Grigorev B.A., Orov D.M., Savchenko N.V., Ryzhov A.E. Study of threshold pressure gradients at filtration through low-permeability reservoir rocks. Vesti Gazovoy Nauki. 2013. N 1 (12), p. 119-125 (in Russian).
- Galimzyanov A., Naydensky K., Huseby O.K. Selection and Justification of Technologies for Enhanced Oil Recovery Methods Using Inter-Well Tracer Survey. SPE Russian Petroleum Technology Conference, 12-15 October 2021. OnePetro, 2021. N SPE-206489-MS. DOI: 10.2118/206489-MS
- Nazarenko M.Yu., Zolotukhin A.B. Application of machine learning for probabilistic production forecasting and ultimately recoverable reserves estimation. Oil Industry Journal. 2020. N 9, p. 109-113 (in Russian). DOI: 10.24887/0028-2448-2020-9-109-113
- Bakirov D.L., Mazur G.V., Babushkin E.V. Improving technology of horizontal sidetracking in complicated geological-technical conditions. Oil Industry Journal. 2019. N 8, p. 40-43 (in Russian). DOI: 10.24887/0028-2448-2019-8-40-43
- Yurova M.P. The Role of Horizontal Wells when Developing Low-Permeable, Heterogeneous Reservoirs. Georesources. 2017. Vol. 19. N 3. Part 1, p. 209-215. DOI: 10.18599/grs.19.3.10
- Kravchenko A.N., Vasilev V.V., Salimov O.V., Samoilov M.I. Hydraulic fracturing. Special aspects and potential of process type assignment. Neftyanaya provintsiya. 2022. N 2 (30), p. 134-149 (in Russian). DOI: 10.25689/NP.2022.2.134-149
- Salimov V.G., Nasybullin A.V., Salimov O.V. Applied tasks of hydraulic fracturing technology. Kazan: Izdatelstvo “Fen” Akademii nauk Respubliki Tatarstan, 2018, p. 380 (in Russian).
- Burkhanov R., Ibragimov I., Khannanov M., Lutfullin A. Investigation of Optical and Rheological Properties of Natural and Recombined Hydrocarbon Mixtures. SPE Russian Petroleum Technology Conference and Exhibition, 24-26 October 2016. OnePetro, 2016. N SPE-181979-MS. DOI: 10.2118/181979-MS