Analysis of the problems of high-quality drinking water extraction from underground water intakes on Vysotsky Island in the Vyborg district of the Leningrad region
- 1 — Ph.D. Mining University, Saint Petersburg ▪ Orcid
- 2 — Ph.D. Mining University, Saint Petersburg ▪ Orcid
- 3 — Manager OOO“SPETS-TRANS” ▪ Orcid
- 4 — Commercial Director Commercial Director ▪ Orcid
- 5 — Ph.D. Leading Specialist Saint Petersburg State University ▪ Orcid
Abstract
This article presents the results of drilling, experimental filtration work and laboratory studies aimed at assessing the resources and quality of groundwater in the licensed area of Vysotsky Island located in the Leningrad region, in the Gulf of Finland in accordance with the requirements of regulatory documents. Analysis of the results of hydrochemical studies and their comparison with data on water intakes in adjacent areas gives the right to conclude that it is possible to classify a hydrogeological unit as a different type of resource formation than those located in the surrounding areas. Groundwater in this area is confined to an unexplored deep fractured regional high-pressure zone. According to the received data, the explored water intake can be attributed to a unique groundwater deposit, which has an uncharacteristic composition of groundwater in the north of the Leningrad region, which may be due to the mixing of modern sediments and relict waters of the Baltic glacial lake. The stability of groundwater characteristics is confirmed by long-term monitoring.
Introduction
The problem of obtaining high-quality drinking water for the purposes of domestic and drinking water supply to the population and manufacturers, meeting all sanitary and epidemiological standards and requirements, in the territory of the Vyborg district of the Leningrad region is very relevant [1-7]. The importance of this problem is caused not only by the hydrogeological and hydrochemical features of the area, but also by the mistakes made by drilling organizations during the construction of water intake wells and their commissioning [8-13]. The low quality of well drilling has been noted for a long time and is caused primarily by the market economy, when the main decision criterion is the reduction of the cost of work, and not the correct construction of the well. The most characteristic design flaw is the lack of cementation of the annular space of the production casing, which is provided for by the requirements of Sanitary Regulations and Norms (SanPiN) 2.1.4.1110-02 “Sanitary protection zones for water supply sources and drinking water pipelines”. The lack of cementation makes it impossible to isolate the exploited aquifer from pollution due to overflow from overlying horizons and from the day surface, and also significantly reduces the life of the well [14-17]. Additional difficulties in providing consumers with drinking water are caused by the increased natural iron content in water, which is a hydrochemical feature of the area (Fig.1) [1, 15].
Groundwater on Vysotsky Island is confined to the fractured aquifer zone of Archean-Proterozoic crystalline rocks and Quaternary sediments. On the periphery of the island, water intake structures are represented by wells and shaft wells with a depth of 10-20 m, exploiting Quaternary horizons, while in the central part these structures are represented in the form of water intake wells with a depth of 70-150 m, provided with equipment in order to extract water from fractured zones in crystalline rocks. Table 1 shows data on existing water intake structures on Vysotsky Island that have a license for groundwater extraction.
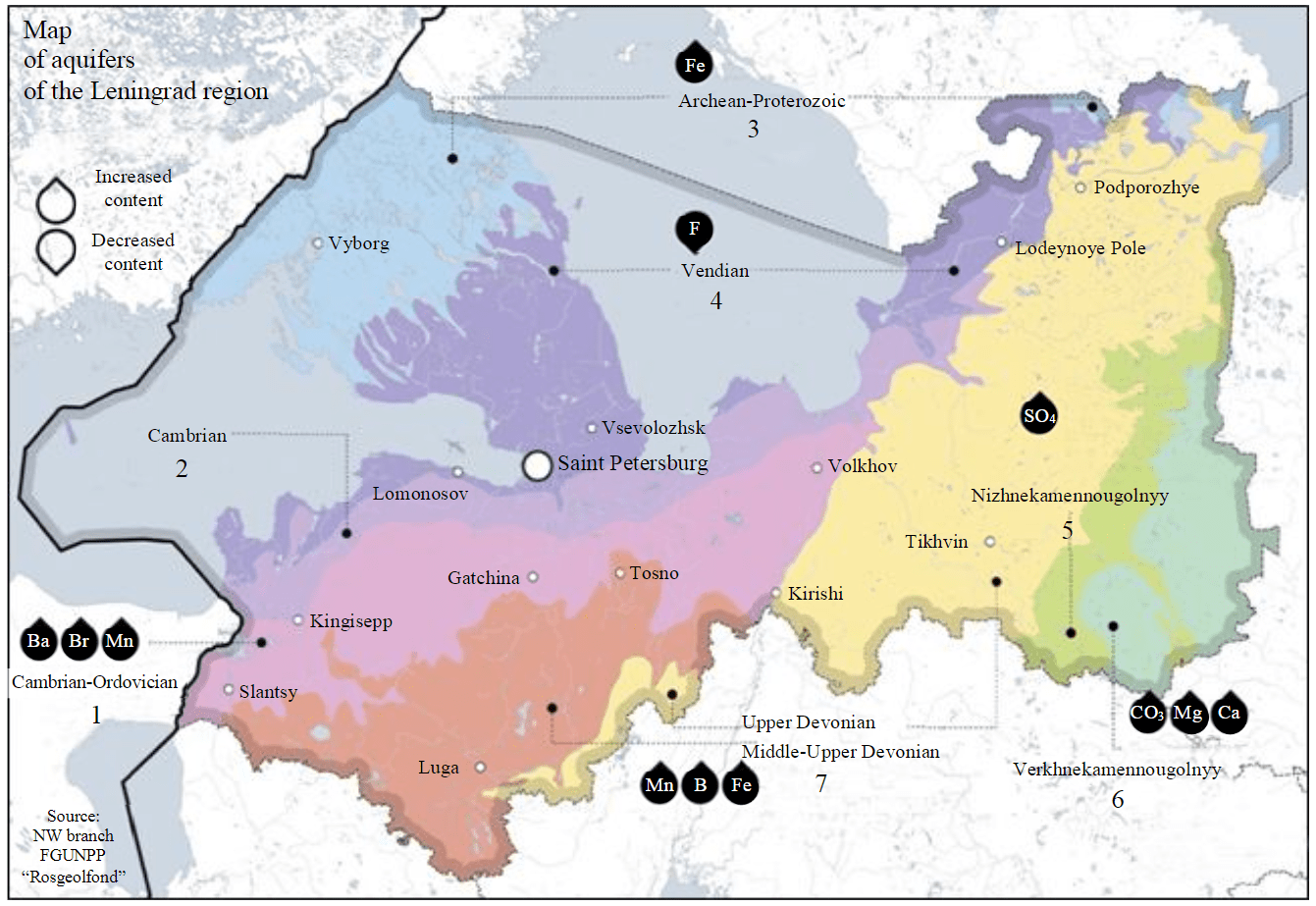
Fig.1. Map of aquifers of the Leningrad region [15] 1 – overestimated natural content of bromine, barium and manganese; 2 – reserves are limited and have little prospects due to low water abundance; 3 – constant excess of iron content; 4 – low fluorine content is observed in the waters of most water intakes; 5 – pressure groundwater, it is moderately hard, in the south of the territory in the lower part of the complex there is water with a high content of sulfates, in the east the mineralization reaches 13.9 g/dm3; 6 – groundwater is predominantly low-pressure, its chemical composition is hydrocarbonate magnesium-calcium, moderately hard; 7 – widespread excess of iron content; increased natural content of boron and manganese was recorded in Devonian waters
Table 1
Main characteristics of existing water intakes in the area of Vysotsky Island
Water user |
Licensed waterintake, m3/day |
Aquifer subdivision |
Sampling interval, m |
Well depth, m |
Year of drilling |
License number |
RPK-Vysotsk |
105 |
АR-PR1 |
9-14,30-45 |
50 |
2003 |
02268 ВЭ |
OAO “RZHD”, Vysotsk railway station |
87 |
АR-R1 + f,lgIIIos |
11-20 |
20 |
1992 |
02308 ВЭ |
Sawmill and woodworking enterprise OOO “Petrotrans” |
2.75 |
АR-PR1 |
10-20,70-110 |
150 |
1995 |
2484 ВЭ |
OOO “Port-Vysotsk” |
35.0-40.9 |
АR-PR1 |
– |
72, 90 |
2008 |
02674 ВП |
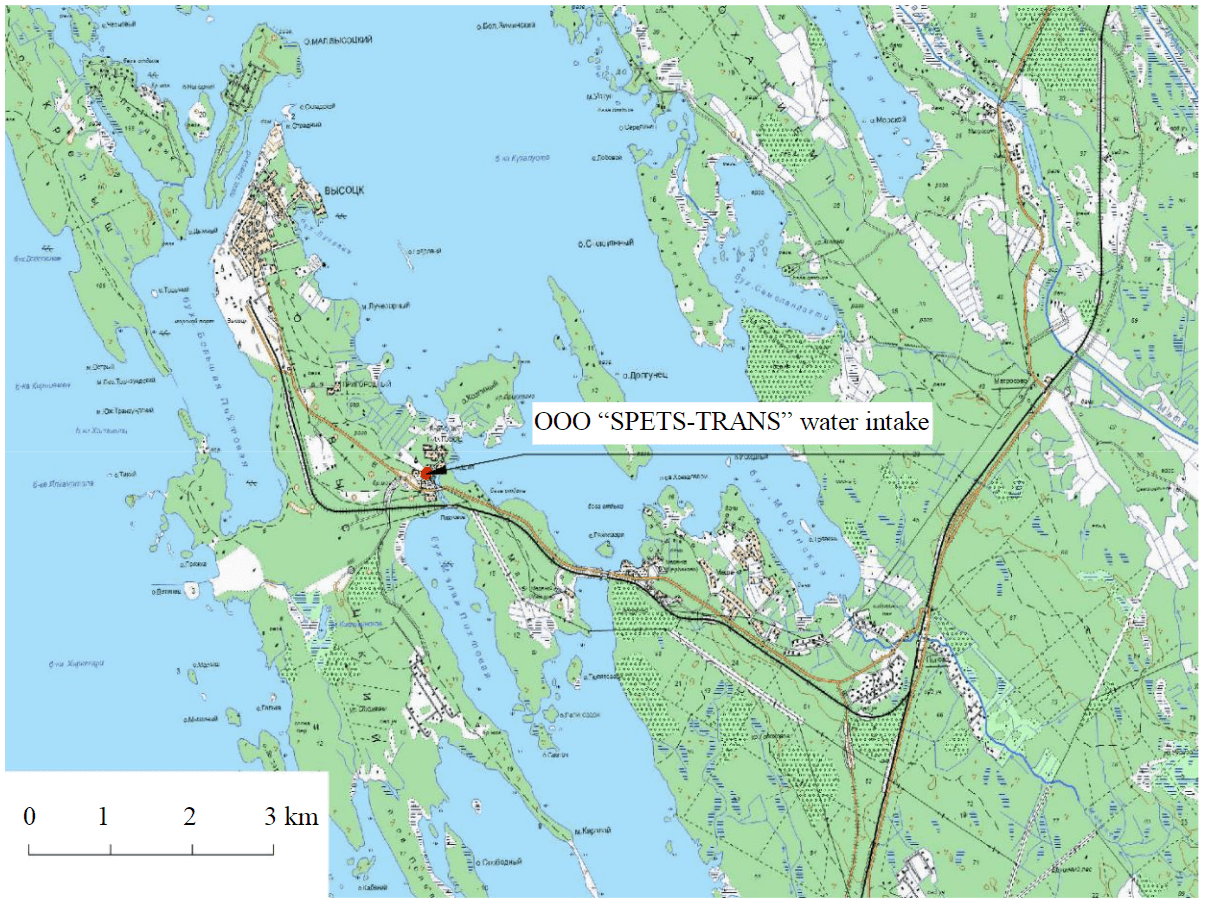
Fig.2. Water intake location plan for OOO “SPETS-TRANS”
Groundwater mixed in chemical composition: hydrocarbonate; sulfate-hydrocarbonate; chloride-hydrocarbonate; sulfate calcium-sodium. Mineralization varies from 115 to 670 mg/l, averaging 280 mg/l. For the main components, the composition of water at the above water intakes complies with the standards (SanPiN 2.1.3684-21), except for iron 1.34-13.3 mg/l (maximum allowable concentration (MAC) 0.3 mg/l), manganese up to 0.41 mg/l (MAC 0.1 mg/l), oxidability up to 39 mgO2/dm3 (MAC 5 mgO2/dm3), sometimes fluorine up to 3.1 mg/l (MAC 1.5 mg/l), increased radon content in some wells up to 573.5 Bq/l (intervention level 60 Bq/l) [3].
Methods
In such areas, it is recommended to focus work on searching for overdeepened valleys and (or) zones of increased fracturing in crystalline rocks [18, 19]. For Quaternary deposits, it is recommended to select aquifers associated with fluvioglacial and marine deposits as the main options [20, 21]. During the analysis of archive materials, the fact was revealed that in these conditions the generally accepted search methodology does not give a complete result. As an example to solve the problem of drinking water quality, the water intake owned by OOO “SPETS-TRANS” was examined, which is located in the eastern part of Vysotsky Island. The location of the water intake of OOO “SPETS-TRANS” is shown in Fig.2, and Fig.3 shows a hydrogeological map of Vysotsky Island with a section.
In 2018, two wells (1 and 2) were drilled in the water intake area of OOO “SPETS-TRANS” and the obtained data revealed a zone of increased fracturing in Archean-Proterozoic granites. The upper part of the wells is secured with casing pipes with annular cementation, and an open hole is left from the bottom of the casing to the bottom of the well [22]. Specialists from OOO “Eco-Expert” carried out two cluster pumpings in turn from wells 1 and 2. The submersible pump JILEX Vodomet 110/110 and the motor pump DDE PTR80 were used as water-lifting equipment during the experiment. Flow was measured using a Groen DRC Du-50 water meter; the water level was measured using a hydrostatic pressure sensor with built-in Solinst Levelogger M20 recorders, control measurements were carried out using an electric level gauge. The examined wells produced a significant flow rate for this area. The received data is shown in Table 2.
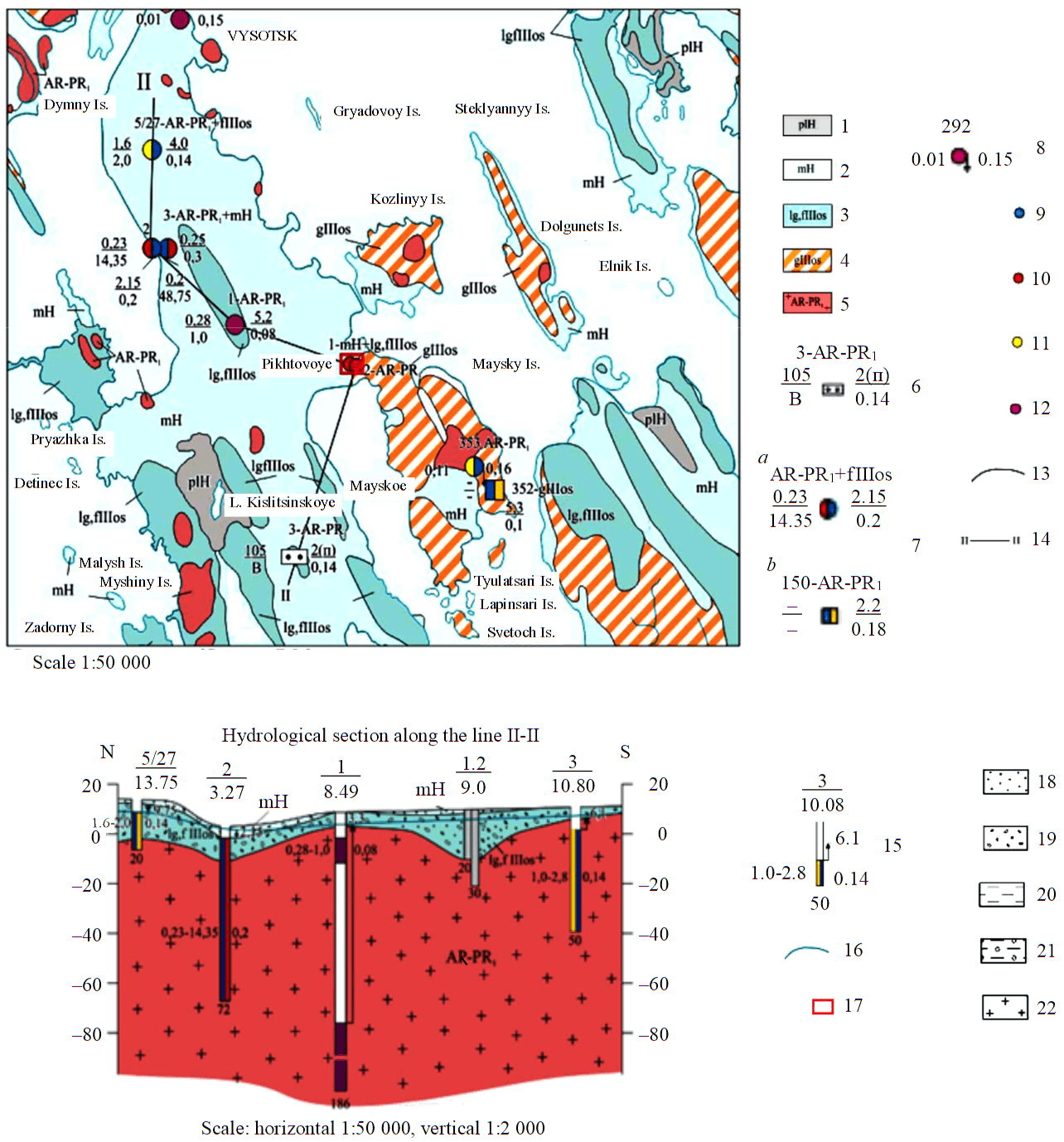
Fig.3. Hydrogeological map of Vysotsky Island with section [15] 1 – modern bog aquifer (peat); 2 – modern marine aquifer (sands, sandy loams, less often loams with ribbon-type layering); 3 – Ostashkovsky lacustrine-glacial and fluvioglacial aquifer (sands of different grains, coarse-grained, less often sandy loam with gravel and pebbles); 4 – Ostashkovsky moraine relatively water-resisting horizon (clayey sands, silty sands with gravel, pebbles and boulders, sometimes boulder sandy loam); 5 – aquiferous zone of fracturing of Archean-Proterozoic rocks (granites, gneisses); 6 – water intake (at the top – the number on the map and the index of the aquifer; on the left in the numerator – reserves (m3/day), in the denominator – mineralization (g/l); on the right – the number of wells in the water intake, in brackets – the index of water type according to its use); 7 – at the top – map number and index of the aquifer; on the left in the numerator – flow rate (l/s), in the denominator – reduction; on the right in the numerator – the depth of the standing water level (m), in the denominator – water salinity (g/l); the shading corresponds to the chemical composition of groundwater (a – well, b – dug well); 8 – descending spring (above – number, on the left – flow rate, on the right – mineralization); 9-12 – chemical type of water: 9 – hydrocarbonate, 10 – chloride, 11 – sulfate, 12 – mixed; 13 – boundaries of hydrogeological units; 14 – line of geological and hydrogeological section; 15 – on sections – well (above: numerator – well registration number, denominator – wellhead elevation (m), the arrow corresponds to the groundwater pressure, the number at the arrow is the absolute elevation of the level (m), to the left of the sampling interval: flow rate (l/s) – depression (m), on the right – mineralization (g/l); 16 – groundwater level; 17 – work area; 18-22 – lithological designations: 18 – sand, 19 – gravel-pebble (pebble-boulder) sediments, 20 – loams, clays, 21 – boulder loams, sandy loams, 22 – granites
Table 2
Basic information about experimental pumping
Well number |
Duration of pumping/recovery, h |
Average pumping flow rate, l/s |
Depth of static level, m |
Maximum drop, m |
1 |
15.5 |
2.66 |
2.23 |
0.93 |
2 |
7.0 |
2.59 |
2.28 |
1.59 |
Groundwater samples in accordance with the “Working program for monitoring the quality of drinking water”, approved by Rospotrebnadzor TU in the Leningrad Region, were examined in accredited testing laboratories to determine their compliance with the established requirements: SanPiN 2.6.1.2523-09 “Radiation Safety Standards” (NRB-99/2009), SanPiN 2.6.1.2800-10 “Hygienic requirements for limiting exposure of the population due to natural sources of ionizing radiation” and MU 2.6.1.1981-05 (with amendment N 1 MU 2.6.1.2719-10), “Radiation control and hygienic assessment of sources of drinking water supply and drinking water according to radiation safety indicators”, SanPiN 1.2.3685-21 “Hygienic standards and requirements for ensuring the safety and (or) harmlessness of environmental factors to humans”, SanPiN 2.1.3684-21 and EAEU TR 044/2017.
The isotopic composition of groundwater (deuterium content – 2H and oxygen-18 – 18O) was determined in the Science Park of Saint Petersburg State University, the results are given in ppm relative to the SMOW standard. The measurement error is ±0.1 ‰ for δ18O and ±1 ‰ for δ2H.
Results and discussion
Experimental filtration testing
Basic information about the progress of pumping is given in Table 2. Indicator graphs of pumping and the water level recovery are presented in Fig.4.
The results of interpretation of filtration experiments are given in Table 3.
Hydraulic parameters calculated from reduction graphs are distorted due to significant unsteadiness of pumping flow rates. At the same time, due to high piezoconductivity, level fluctuations in the disturbing well are largely reflected in the observation well.
The calculation of the parameters that most reliably characterize the properties of the medium was carried out by restoring the levels of the water. When pumping from well 1, the water level in it was restored after stopping the pumps operation almost instantly, so it was decided to process only the graph for well 2. After pumping from well 2, the level in it recovered by more than 80 % in the first minute, which indicates the influence of the skin effect. This graph was processed in two versions using the solutions of Theis and Mench. In both options, a water conductivity coefficient of 940 m2/day was obtained, but Mench’s solution gave a more realistic value of piezoconductivity of the order of 106 m2/day. Thus, based on the experimental results, the water conductivity of the tested Archean-Proterozoic aquifer zone is in the range of 940-980 m2/day, and the piezoconductivity is (1.2-2.3)∙106 m2/day. To assess the groundwater reserves of the subsurface site under consideration, the most unfavorable ones should be taken as the calculated values of filtration parameters: water conductivity 940 m2/day, piezoconductivity 2.3·106 m2/day, elastic water yield (m* = T/a*) – 4.1·10–4. The value of elastic water yield, obtained during conducted experiments, is in good agreement with the elastocapacity characteristic of fractured rocks of the order of 10–5 m–1, with the thickness of the fractured zone of Archean-Proterozoic rocks on the order of a few tens.
Determining the specific flow rate of wells is complicated by the unsteadiness of the operation of water-lifting equipment. However, stabilization of levels at the end of pumping over a long period of time allows to roughly estimate this parameter:
- for well 1, the specific flow rate is 3.9 l/s·m, with a decrease of 0.74 m and an average pumping flow rate of 250 m3/day (2.89 l/s);
- for well 2, the specific flow rate is 2.17 l/s·m, with a decrease of 1.2 m and an average pumping flow rate of 225 m3/day (2.6 l/s).
It can be assumed that after drilling additional wells, the water intake will be able to provide groundwater flow in a volume of up to 15000 m3/day.
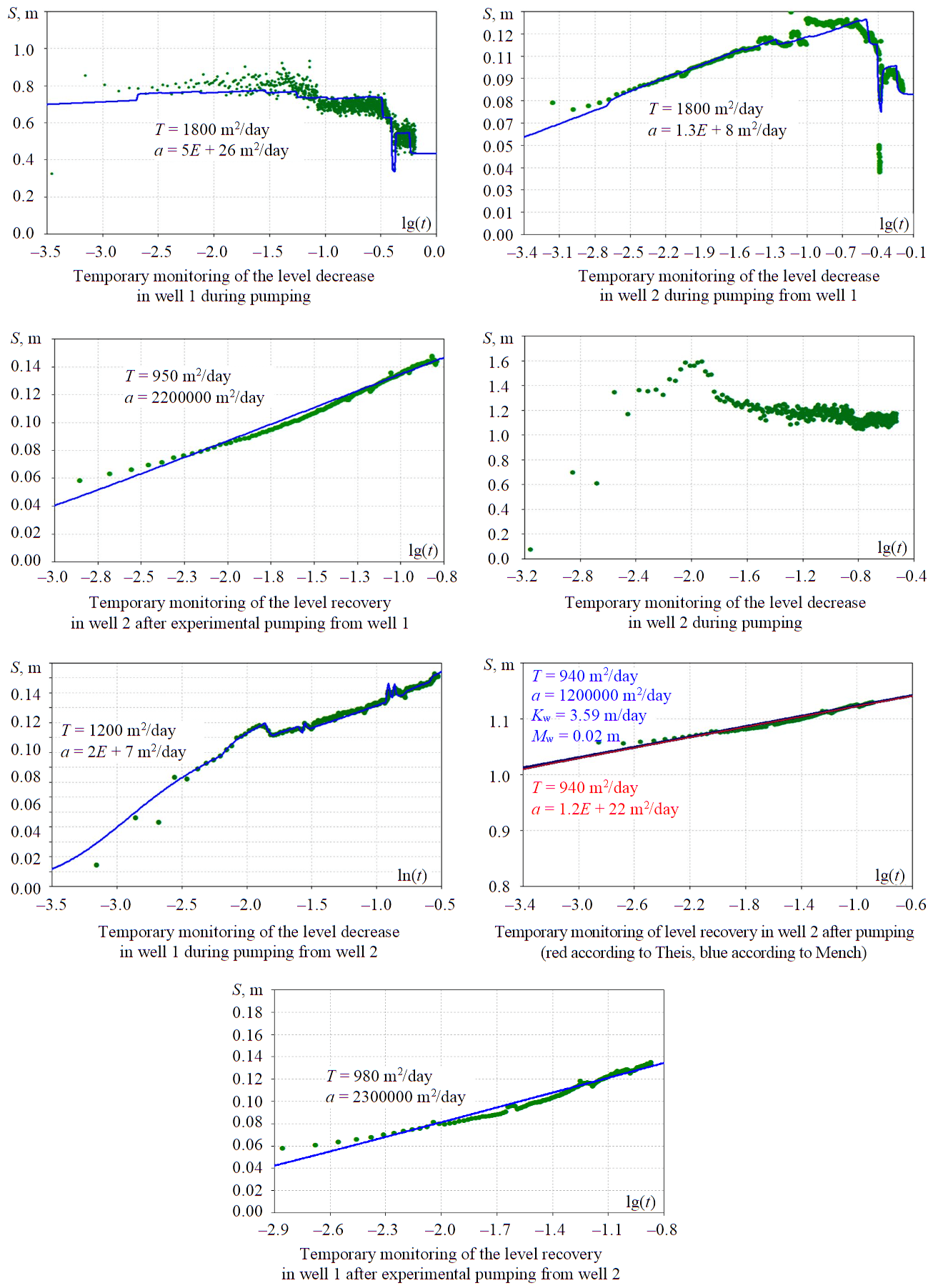
Fig.4. Indicator graphs of pumping and level recovery in wells 1 and 2
Table 3
Results of interpretation of filtration experiments
Centralwellnumber |
Experiment name |
Monitoring well number |
Water permeability Т, m2/day |
Piezoconductivity а*, m2/day |
1 |
Pumping |
1 |
– |
– |
2 |
1800 |
1.3·108 |
||
Recovery |
1 |
– |
– |
|
2 |
950 |
2.2·106 |
||
2 |
Pumping |
1 |
1200 |
2·107 |
2 |
– |
– |
||
Recovery |
1 |
980 |
2.3·106 |
|
2 |
940/940 |
1.2·1022/1.2·106 |
Hydrochemical testing.
The qualitative composition of water from fractured Archean-Proterozoic crystalline rocks is characterized as sulfate-hydrocarbonate-chloride of the mixed cationic composition. The mineralization of these waters equals to 105-132 mg/l (on average 121 mg/l) and a typical chemichal formula
The waters are close to ultra-sweet, slightly acidic with a pH of 6.0-6.8 (average 6.2); soft – total hardness 1.4-2.3 mgEq/l (average 1.8 mgEq/l). Average content of hydrocarbonates – 28.3 mg/l, sulfates – 16 mg/l, chlorides – 19.5 mg/l, sodium + potassium – 14.4 mg/l, calcium – 13.7 mg/l, magnesium – 4.8 mg/l, nitrates – 6 mg/l, ammonium < 0.001 mg/l. Components indicating anthropogenic pollution are: NO2, NO3, NH4, PO4, Al, Pb, petroleum products and phenols are contained in minimal concentrations or are absent.
The results of a detailed radiological analysis of the water samples showed compliance of the activity values of alpha- and beta-emitting radionuclides with the sanitary standards established by the next requirements: SanPiN 2.6.1.2523-09 “Radiation Safety Standards” (NRB-99/2009), SanPiN 2.6.1.2800-10 “Hygienic requirements for limiting public exposure due to natural sources of ionizing radiation” and MU 2.6.1.1981-05 (as amended N 1 MU 2.6.1.2719-10), “Radiation monitoring and hygienic assessment of sources of drinking water supply and drinking water on radiation safety indicators”. According to microbiological and virological characteristics of the water samples, the water of the observed horizon is healthy.
Groundwater fully meets the requirements of SanPiN 2.1.3684-21, SanPiN 2.1.3685-21, SanPiN 2.6.1.2523-09 (NRB-99/2009) and EAEU TR 044/2017 (Table 4).
Table 4
Groundwater analysis results
Indicator |
Well 2min-max |
Well 1min-max |
Requirements according to SanPiN 2.1.3684-21 |
Requirements according to EAEU TR 044/2017 |
|
Organoleptic properties |
|||||
Smell, points |
0 |
0 |
2 |
0-1 |
|
Colouration, deg |
1.2-2.6 |
1.5-5.0 |
20 |
< 5.0 |
|
Turbidity, mg/dm3 |
0.5-0.6 |
0.58-0.67 |
2.6 |
1.0 |
|
Continuation of Table 4 |
|||||
Indicator |
Well 2min-max |
Well 1min-max |
Requirements according to SanPiN 2.1.3684-21 |
Requirements according to EAEU TR 044/2017 |
|
Remaining taste, points |
0 |
0 |
0 |
0 |
|
Generalized indicators |
|||||
pH value |
6.0-6.8 |
6.0-6.8 |
6.0-9.0 |
4.5-9.5 |
|
Total hardness, mgEq/dm3 |
0.55-1.9 |
0.6-2.3 |
7.0 |
7.0 |
|
Permanganate oxidability, mgO2/dm3 |
1.0-2.0 |
1.1-2.1 |
5.0 |
3.0 |
|
Petroleum products, mg/dm3 |
< 0.005 |
< 0.005 |
0.1 |
0.05 |
|
Phenolic index, mg/dm3 |
< 0.005 |
< 0.005 |
0.25 |
– |
|
SS (anionic), mg/dm3 |
0.01-0.025 |
0.01-0.025 |
0.5 |
0.05 |
|
Volatile phenols, µg/dm3 |
< 0.0005 |
< 0.0005 |
0.1 |
0.5 |
|
Components of the general chemical composition |
|||||
Dry residue, mg/dm3 |
108.0-150.0 |
105.1-140.0 |
1000 |
< 1000 |
|
Total iron, mg/dm3 |
0.04-0.14 |
0.05-0.15 |
0.3 |
0.3 |
|
Nitrates, mg/dm3 |
0.1-2.8 |
0.1-2.8 |
45 |
20 |
|
Nitrites, mg/dm3 |
< 0.003 |
< 0.003 |
3.3 |
0.5 |
|
Sulfates, mg/dm3 |
20.0-40.0 |
20.0-40.0 |
500 |
250 |
|
Chlorides, mg/dm3 |
18.4-28.0 |
21.1-31.0 |
350 |
250 |
|
Cyanides, mg/dm3 |
< 0.01 |
< 0.01 |
0.07 |
0.035 |
|
Calcium, mg/dm3 |
9.7-27.0 |
13.7-17.0 |
Not standardized |
Not standardized |
|
Magnesium, mg/dm3 |
3.8-4.9 |
4.8-12.8 |
50 |
Not standardized |
|
Sodium, mg/dm3 |
9.2-12.2 |
9.1-13.0 |
200 |
200 |
|
Potassium, mg/dm3 |
4.6-5.3 |
4.6-5.3 |
Not standardized |
– |
|
Phosphates, mg/dm3 |
< 0.05 |
< 0.05 |
3.5 |
3.5 |
|
Inorganic matter |
|||||
Molybdenum, mg/dm3 |
< 0.025 |
< 0.025 |
0.25 |
0.07 |
|
Barium, mg/dm3 |
< 0.024 |
< 0.024 |
0.1 |
0.7 |
|
Beryllium, mg/dm3 |
< 0.0001 |
< 0.0001 |
0.0002 |
– |
|
Copper, mg/dm3 |
0.001-0.003 |
0.001-0.004 |
1.0 |
1.0 |
|
Cadmium, mg/dm3 |
< 0.001 |
< 0.001 |
0.001 |
0.001 |
|
Aluminum, mg/dm3 |
< 0.055 |
< 0.04 |
0.2 |
0.2 |
|
Manganese, mg/dm3 |
0.005-0.046 |
0.007-0.01 |
0.1 |
0.05 |
|
Arsenic, mg/dm3 |
< 0.005 |
< 0.005 |
0.01 |
0.01 |
|
Zinc, mg/dm3 |
< 0.0102 |
< 0.091 |
1 |
5 |
|
Boron, mg/dm3 |
< 0.05 |
< 0.05 |
0.5 |
1 |
|
Lead, mg/dm3 |
< 0.002 |
< 0.002 |
0.01 |
0.01 |
|
Nickel, mg/dm3 |
< 0.0075 |
< 0.067 |
< 0.1 |
0.02 |
|
Mercury, mg/dm3 |
< 0.0001 |
< 0.0001 |
0.0005 |
0.0005 |
|
Strontium, mg/dm3 |
< 0.1 |
< 0.1 |
7 |
7 |
|
Fluorides, mg/dm3 |
0.12-1.2 |
0.11-1.4 |
1.5 |
1.5 |
|
Selenium, mg/dm3 |
< 0.005 |
< 0.005 |
0.01 |
0.01 |
|
Titanium, mg/dm3 |
< 0.001 |
< 0.001 |
0.1 |
– |
|
Chromium, mg/dm3 |
< 0.001 |
< 0.005 |
0.05 |
– |
|
Cyanides, mg/dm3 |
< 0.005 |
< 0.01 |
0.07 |
0.035 |
|
Organic matter |
|||||
Gamma- HCH (lindane) |
< 0.00001 |
< 0.00001 |
0.002 |
0.5 |
|
DDT (sum of isomers) |
< 0.00001 |
< 0.00001 |
0.002 |
0.5 |
|
2.4-D, µg/dm3 |
< 0.0002 |
< 0.03 |
0.03 |
1.0 |
|
Ammonia and ammonium ion |
< 0.1 |
< 0.1 |
1.5 |
0.1 |
|
Microbiological properties |
|||||
Total coliform bacteria, CFU/100 ml |
n.d. |
n.d. |
Not allowed |
– |
|
Thermotolerant coliform bacteria, CFU/100 ml |
n.d. |
n.d. |
Not allowed |
– |
|
Total microbial count, CFU/ml |
0.0-3.0 |
0.0-2.0 |
< 50 |
20-100 |
|
Coliphages, PFU/100 ml |
n.d. |
n.d. |
Not allowed |
– |
|
Enterococci, CFU/100 ml |
n.d. |
n.d. |
Not allowed |
– |
|
E.coli, in 100 cm3 |
n.d. |
n.d. |
Not allowed |
– |
|
Radiation indicators |
|||||
Total volumetric activity of alpha-emitting radionuclides, Bq/l |
0.02-0.08 |
< 0.08 |
0.2 |
0.2 |
|
Total volumetric activity of beta-emitting radionuclides, Bq/l |
0.14-0.32 |
0.2-0.32 |
1 |
1 |
|
Radon-222 |
7.0-31.0 |
7.00-8.00 |
60 |
– |
|
Virological indicators |
|||||
Group A rotavirus RNA |
n.d. |
n.d. |
Not allowed |
||
Norovirus genotype 2 RNA |
n.d. |
n.d. |
Not allowed |
||
Astrovirus RNA |
n.d. |
n.d. |
Not allowed |
||
Enterovirus RNA |
n.d. |
n.d. |
Not allowed |
||
Hepatitis A virus |
n.d. |
n.d. |
Not allowed |
Note. n.d. – not detected.
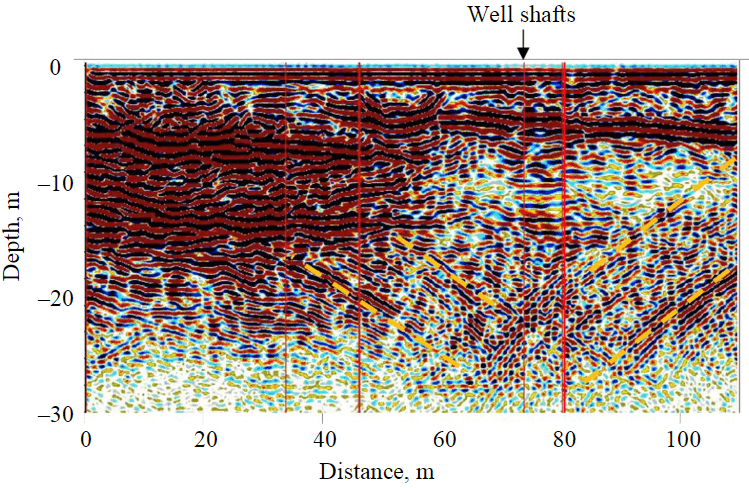
Fig.5. Georadiolocation section of the water intake across the placement of wells 1 and 2 in the middle of the distance between them
The results of chemical analysis of groundwater sampled at the water intake of OOO “SPETS-TRANS”, when compared with data on groundwater samples extracted from other water intakes, that are located on Vysotsky Island or in other adjacent areas, indicate that in the licensed area the conditions for the formation of resources are different by their nature from the areas located nearby. Groundwater at the site does not exceed the maximum allowable concentration for iron, manganese, fluorine, permanganate oxidation and radiological indicators, while other water intakes on Vysotsky Island are characterized by iron content values of 1.34-13.3 mg/l (MAC 0.3 mg/l), manganese values up to 0.41 mg/l (MAC 0.1 mg/l), oxidation index values up to 39 mgO2/dm3 (MAC 5 mgO2/dm3) and radon activity values up to 573.5 Bq/l (intervention level 60 Bq/l). The composition of the groundwater samples obtained from the wells of OOO “SPETS-TRANS” is similar to the samples of the water captured by the “Domik Lesnika” spring, which is located 4.5 km in a northeast direction from the city of Vyborg along the Vyborg – Svetogorsk highway.
At the end of 2019, a georadar survey was carried out in the water intake area using the method of continuous scanning at the central frequency of probing electromagnetic pulses of 50 MHz with the ABDL “Triton” antenna unit [23-25]. The results that were obtained made it possible to identify intensive intervals of disturbed rocks and outline areas that are promising for further drilling of production wells in the area (Fig.5).
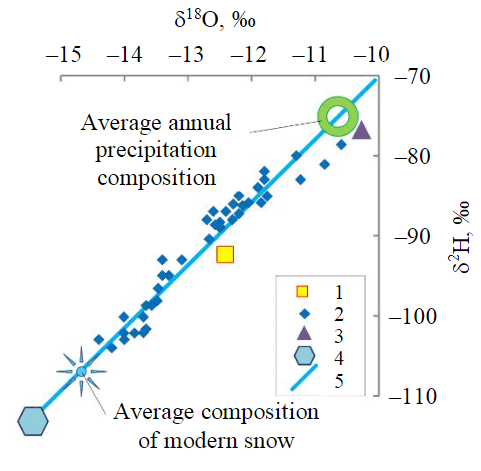
Fig.6. Isotopic composition of groundwater on Vysotsky Island 1 – isotopic composition of oxygen and hydrogen in water extracted from wells 1 and 2 of the water intake of OOO “SPETS-TRANS” relative to the SMOW standard; 2 – water intake wells on the Karelian Isthmus (the lightest compositions correspond to wells near the mouth of the Sestra River); 3 – average long-term isotopic composition of water in the Neva River; 4 – isotopic composition of groundwater of the Vendian-Riphean complex in the Ladoga graben at a depth of 250 m; 5 – local line of meteoric waters (trend of changes in the isotopic composition of precipitation in the annual cycle of observations)
The isotopic composition of groundwater in the Karelian Isthmus and Kronstadt Island was studied over the period of 2012-2019 (Fig.6). Groundwater with modern nourishment, which is reliably established by the presence of tritium, turns out to be isotopically lighter than modern atmospheric precipitation, for which the long-term average values of δ18O = –10.7 ‰ and δ2H = –76 ‰ (Fig.6). The waters of groundwater horizons are characterized by compositions of about δ18O = –11.3 ‰ and δ2H = –80 ‰, since infiltration nutrition is formed due to isotopically light precipitation of the cold season (melted snow and autumn-spring rains). The waters of the Vendian aquifer complex on the Karelian Isthmus near the mouth of the Sestra River have an isotopic composition of up to δ18O = –14.2 ‰ and δ2H = –104 ‰, and the waters of the Vendian-Riphean complex in the Ladoga graben at a depth of about 250 m are lightened down to δ18O = –16.6 ‰ and δ2H = –119 ‰.
Waters with the indicated light isotopic compositions could only form in the climatic conditions when average annual temperatures were noticeably lower than modern average annual temperatures, which are about 6.3 °C. According to the calculation by the formula derived by Willi Dansgaard in 1964 [26] using the lightest values of the isotopic composition of water, characteristic of the Vendian and Vendian-Riphean complexes, it was found that the climatic average annual temperatures during the formation of these waters reached –3.9 °C. Consequently, such waters could have been formed only during the period of the last ice age or immediately after its end. Cold climatic conditions with near-zero average annual temperatures prevailed in the north-west regions of Russia at the end of the last cold spell, which was about 10-12 thousand years ago. During this period, the Saint Petersburg area represented a gulf of the Baltic glacial lake, the basin of which was filled with melted glacial water, which had a lighter (comparing to modern precipitation) isotopic composition [27-36].
For the water intake area of OOO “SPETS-TRANS”, based on the results of double sampling, the following isotopic characteristics of groundwater were found: δ18O = –12.4 ‰ and δ2H = –92.4 ‰, which corresponds to average annual temperatures of about 2 °C. Taking into account the design of the wells and the location of the work site, it should be assumed that the water samples obtained during pumping are mixtures of deep and near-surface components. If we use the indicated average isotope characteristics for groundwater δ18O = –11.3 ‰ and δ2H = –80 ‰ and relict waters of the Vendian-Riphean complex δ18O = –16.6 ‰ and δ2H = –119 ‰, then from the equation of two-component mixing, it can be calculated that the ratio of the volumes of the ancient and young components is approximately 0.27. If we use the isotopic composition of groundwater in the area of the Sister River mouth as an extreme term, than the ratio of the volumes of the ancient and young components will equal 0.4. The calculations carried out based on the data obtained allow to assert that at least one third part of the water extracted by the water intake wells of OOO “SPETS-TRANS” is relict water formed by infiltration feeding with a light isotopic composition.
Conclusion
Based on the results of the conducted drilling, geophysical, experimental filtration work and laboratory studies, it can be concluded that the explored water intake can be attributed to a unique groundwater deposit, which has water quality uncharacteristic for the north of the Leningrad region, which may be due to the mixing of modern precipitation and relict waters of the Baltic glacial lake. Such source of the water makes it possible to solve the problem of providing the population of Vysotsk and the manufacturers of Vysotsky Island with domestic and drinking water supply that meets all the requirements of SanPiN 2.1.3684-21, SanPiN 2.1.3685-21, SanPiN 2.6.1.2523-09 (NRB-99/2009) and EAEU TR 044/2017. It is also worth noting the annual prizes awarded to water samples from this water intake, for example, in May 2022, a water sample was awarded a silver medal at the annual competition with international participation “Water of the Year – 2022” in the category “Natural drinking water with unchanged composition.”
References
- Ananina I. Problematic waters. Delovoi Peterburg. 2014. N 133, p. 18 (in Russian).
- Litvinenko V.S., Sergeev I.B. Innovations as a Factor in the Development of the Natural Resources Sector. Studies on Russian Economic Development. 2019. Vol. 30. N 6, p. 637-645. DOI: 1134/S107570071906011X
- Erzova V.A., Rumynin V.G., Sudarikov S.M. et al. Influence of North-West nuclear complex facilities on groundwater contamination (Leningrad region). Bulletin of the Tomsk Polytechnic University. Geo Аssets Engineering. Vol. 332. N 9, p. 30-42. DOI: 10.18799/24131830/2021/9/3351
- Kovshov S.V., Yakubovskii M.M., Kovshov V.P. Aquicultural reclamation of sand quarries in the Leningrad region as a comprehensive way to solve environmental problems of the region. Journal of Mining Institute. 2014. Vol. 207, p. 112-115 (in Russian).
- Kotlov S.N., Shamshev A.A. Numerical geo-flow modeling of horizontal drainage holes. Mining Informational and Analytical Bulletin. 2019. N 6, p. 45-55. DOI: 25018/0236-1493-2019-06-0-45-55
- Golovina E.I., Grebneva А.V. Management of groundwater resources in transboundary territories (on the example of the Russian Federation and the Republic of Estonia). Journal of Mining Institute. 2021. Vol. 252, p. 788-800. DOI: 10.31897/PMI.2021.6.2
- Golovina E., Grebneva A.Some Aspects of Groundwater Resources Management in Transboundary Areas. Journal of Ecological Engineering. 2021. Vol. 22. Iss. 4, p. 106-118. DOI: 12911/22998993/134037
- Borevskii B.V., Drobnokhod N.I., Yazvin L.S. Groundwater reserves assessment. Kiev: Vyshcha shkola. Golovnoe izd-vo, 1989, p. 407 (in Russian).
- Cadastre of groundwater in the USSR. Leningrad region. Vol. 2, kn. 2. Moscow: Kopirovalno-kartograficheskoe predpriyatie vsesoyuznogo geologicheskogo fonda, 1962, p. 205 (in Russian).
- Classification of reserves and forecast resources of drinking, technical and mineral groundwater. Moscow: MPR Rossii, 2020, p. 9 (in Russian).
- Khaustov V.V., Ustiugov D.L. Formation of drainage waters of Tyrnyauz deposit in ecological aspect. IOP Conference Series: Earth and Environmental Science. 2017. Vol. 87. N 042006, p. 1-5. DOI: 1088/1755-1315/87/4/042006
- Norova L.P. Basic prerequisites for the formation and development of the Leningrad School of Engineering Geology. Global View of Engineering Geology and the Environment: Proceedings of the International Symposium and 9th Asian Regional Conference of IAEG, 25 September 2013, Beijing, China. 2013, p. 549-555. DOI: 1201/b15794-88
- Lebedeva Y., Kotiukov P., Lange I. Study of the geo-ecological state of groundwater of metropolitan areas under the conditions of intensive contamination thereof. Journal of Ecological Engineering. 2020. Vol. 21. Iss. 2, p. 157-165. DOI: 12911/22998993/116322
- Leonteva E., Grebneva A., Erzova V. The analysis of changing hydrodynamic conditions aiming to validate geo-migration model with insufficient benchmark data. International Multidisciplinary Scientific GeoConference Surveying Geology and Mining Ecology Management (SGEM), 28 June – 7 July 2019, Albena, Bulgaria. STEF92 Technology, 2019. Vol. 19. Iss. 1.2, p. 523-530. DOI: 5593/sgem2019/1.2/S02.067
- Nasonova L.D., Sammet E.Yu., Andreeva N.G. et al. A set of geological, hydrogeological and geoecological maps of the territory of St. Petersburg and its suburbs on a scale of 1:200,000 (with an explanatory note). Vserossiiskoe soveshchanie “Glavneishie itogi v izuchenii chetvertichnogo perioda i osnovnye napravleniya issledovanii v XXI veke”. St. Petersburg: VSEGEI, 1998, p. 324 (in Russian).
- Lalomov D.A., Glazounov V.V. Collaborative Interpretation of the Data Obtained by Resistivity and Ground Penetrating Radar Methods for Assessing the Permeability of Sandy Clay Soils. Journal of Mining Institute. 2018. Vol. 229, p. 3-12. DOI: 10.25515/PMI.2018.1.3
- Ivanov A. Mineralogical characteristics and spatial distribution of different age fractures in granites and pegmatites of the “Vozrozhdenie” deposit (“Kawantsaari”), the Vyborg massif. Journal of Mining Institute. 2015. Vol. 212, p. 21-29 (in Russian).
- Ustyugov D.L., Mironchuk D.P. Short-term pumping tests as an identification method of aquifer-surface interaction. Journal of Mining Institute. 2015. Vol. 212, p. 84-88 (in Russian).
- Kudelskii A.V., Norova L.P. About applied hydrogeochemistry. Geoekologiya, inzhenernaya geologiya, gidrogeologiya, geokriologiya. 2014. N 1, p. 91-96 (in Russian).
- Ustyugov D.L. Constantly working hydrodinamical model of Yakovlevsky rich iron-ores deposit (Kursk magnetic anomaly). Journal of Mining Institute. 2013. Vol. 200, p. 332-335 (in Russian).
- Tsinkoburova M.G., Norova L.P. Reflection of Northwestern Russia's Hydrogeological Features in Old Toponyms of the Region. Izvestiya Rossiiskoi Akademii Nauk. Seriya Geograficheskaya. 2021. Vol. 85. N 5, p. 750-762. DOI: 31857/S2587556621050101
- Blinov P.A., Nikishin V.V., Kozlov A.V. Hydroaeromechanics and heat transfer in drilling. St. Petersburg: Lema, 2015, p. 93 (in Russian).
- Danilieva N.A., Daniliev S.M., Bolshakova N.V. Allocation of a deep-lying brine aquifer in the rocks of a chemogenic section based on the data of geophysical well logging and 2D seismic exploration. Journal of Mining Institute. 2021. Vol. 250, p. 501- DOI: 10.31897/PMI.2021.4.3
- Daniliev S.M., Danilieva N.A., Isakova E.P., Ashkar G.K. Research of cracking at facing stone deposit using the georadar method. Bulletin of the Tomsk Polytechnic University. Geo Assets Engineering. 2020. Vol. 331. N 9, p. 140-145 DOI: 18799/24131830/2020/9/2816
- Mardashov D., Duryagin V., Islamov S. Technology for improving the efficiency of fractured reservoir development using gel-forming compositions. Energies. 2021. Vol. 14. Iss. 24. N 8254. DOI: 3390/en14248254
- Dansgaard W. Stable isotopes in precipitation. Tellus. 1964. Vol. 16, p. 436-468.
- Andrén T., Andrén E., Björck S., Conley D.J. The Development of the Baltic Sea Basin During the Last 130 ka. The Baltic Sea Basin. Berlin Heidelberg: Springer-Verlag, 2011, p. 75-97.
- Hyttinen O. Sedimentological and chronological aspects of the Younger Dryas – Holocene transition record in southern Finland and northern Baltic. Helsinki: Unigrafia, 2012, p. 38.
- Geochemistry of Baltic sea surface sediments. Ed. by S.Uscinowicz. Warszawa: Polish Geological Institute – National Research Institute, 2011, p. 356.
- Uscinowicz S. Southern Baltic area during the last deglaciation. Geological Quarterly. 1999. Vol. 43. N 2, p. 137-148.
- Vassiljev J., Saarse L. Timing of the Baltic Ice Lake in the eastern Baltic. Bulletin of the Geological Society of Finland. 2013. Vol. 85. N 1, p. 9-18.
- Tikkanen M., Oksanen J. Late Weichselian and Holocene shore displacement history of the Baltic Sea in Finland. Fennia – International Journal of Geography. 2002. Vol. 180. N 1-2, p. 9-20.
- Bennike O., Jensen J.B. A Baltic Ice Lake lowstand of latest Allerød age in the Arkona basin, southern Baltic sea. Geological Survey of Denmark and Greenland. 2013. Vol. 28, p. 17-21.
- Wohlfarth B., Björck S., Funder S. et al. Quaternary of Norden. Episodes. 2008. Vol. 31. N 3, p. 73-81.
- Kemp C., Horton B.P., Engelhart S.E. Sea-Levels, Late Quaternary. Late Quaternary Relative Sea-Level Changes at Mid-Latitudes. Encyclopedia of Quaternary Science (Second Edition). Elsevier, 2013, p. 489-494. DOI: 10.1016/B978-0-444-53643-3.00140-0
- Lemke, Harff J. Europe. Holocene. Encyclopedia of Geology. Elsevier, 2005, p. 147-160. DOI: 10.1016/B0-12-369396-9/00470-6