Improvement of concentrate quality in flotation of low-rank coal
- 1 — Ph.D., Dr.Sci. Chief Researcher Chinakal Institute of Mining, Siberian Branch, Russian Academy of Sciences ▪ Orcid
- 2 — Postgraduate Student Chinakal Institute of Mining, Siberian Branch, Russian Academy of Sciences ▪ Orcid
Abstract
Percentage of high-rank coal with low content of ash, moisture, and sulfur in total coal production output is low. Most of the produced coal has a low quality (lignite, bituminous coal: long-flame and fiery). Under increasing requirements for ecological cleanness of coal, the efficient use of coal products is only possible after improvement of their processing properties. The authors discuss the enhancement of flotation efficiency of low-rank coal using the mechanism of physisorption of a collecting agent in particle – bubble attachment. It is explained why the yield of concentrate with low ash content increases as a result of combination of collectors having different physical properties. It is shown that the surface activity of a heteropolar agent relative to the gas – liquid interface and the adsorption density of the agent govern its collecting properties. Based on the recovery – surface activity relationship, the correlation is found between the collecting activity of a chemical compound and the structure of its molecules. The combination of the collectors with different surface activity enables adjusting collectability and selectivity of the blend. The physisorption mechanism of collectors can be a framework for developing recommendations on modification of concentrate yield and ash content, and on selection of optimized ratios of surface activities of miscible collectors relative to the gas – liquid interface.
Introduction
The electric power sector in Russia in recent decades features a steady trend toward a decline in the quality of coal as a consequence of the growing scale of its gross production. Low-rank coals used in power generation in Russia differ drastically by composition and qualities which govern efficiency of coals in terms of energy, ecology, and economy. Ash content of low-rank fuel exceeds 40 %. It is unlikely that the quality of coal can improve in the future as it is planned to increase production of power-station coals by open-pit mining of lower rank coal deposits.
In Russia run-of-mine coal is mostly used in industrial power generation. The use of low-rank coals features lower combustion heat and higher fuel consumption [1]. Combustion of low-rank coal slack involves increased emission of toxic pollutants, as well as elevated cost of transportation and storage of much ash and slag. The long-term development program approved by the Russian Government for the coal industry in Russia up to 2030 requires improvement of energy efficiency and industrial and ecological safety. It is only possible to make low-rank coal a high-rank fuel by beneficiation. Flotation is an efficient way of dressing coal slag and upgrading quality of low-rank coal.
Flotation of low-rank coal slag consumes much more conventional collectors. The process selectivity drops as conventional collectors fed at increased consumption are adsorbed at ash [2-5]. To increase coal recovery and decrease ash content of concentrate, a combination of collectors is commonly used. For instance, a mixture of residual fuel oil containing long-chain hydrocarbons with kerosene substantially improved flotation of oxidized coal [6, 7]. The use of mixed dodecane and 4-dodecylphenol allowed increasing extraction of lignite [8]. The higher yield of concentration can be explained by the increased hydrophobicity of coal as a result of sorption of 4-dodecylphenol preferably at oxidized hydrophilic surfaces, while dodecane adsorbed at hydrophobic surfaces.
Supposedly, higher hydrophobicity of low-rank coal enhances selectivity of its recovery. To this effect, the surface tension of a main collector is reduced by addition of surfactants. Many researchers think that its finer emulsification occurs and allows better spreading and flattening of microdrops attached to coal [9, 10]. It is also possible that an apolar collector spreads over a heteropolar collector attached to oxidized areas of coal [11-13].
It is stated in [14] that reagents containing apolar compounds, which are more akin to aromatic components of coal of medium and high metamorphic degree and are capable of fixing on the coal surface in a droplet form rather than in molecular form at the coal surface, are more effective. It is also believed that a physisorbed collector should attach as microdrops [15].
Insoluble collectors find application not only in coal flotation. The use of insoluble hydroxamic acid (AERO 6493) added with aliphatic alcohols С6-С13 allowed improving the efficiency of apatite ore concentration. A single flotation stage produced a concentrate with a Р2О5 content of 31 % and the recovery of 95 %, while the flotation feed only contained 5 % Р2О5 [16, 17]. High selectivity of extraction of apatite ore was explained by spreading of a microdrop of the insoluble complex collector over the mineral surface [18]. Flotation of phosphate from nepheline ore using fat acids and surface-active oxyethylated isotridecanol produced in a single process stage a high-quality concentrate containing 31 % Р2О5 at the recovery of 95 % [19].
Allyl ethers of xanthates are the best selective agents amongst sulfide collectors. These agents are therefore insoluble in water and are usually fed in the cycle of milling. For making a target mineral hydrophobic, xanthate is fed in the cycle of flotation. A small consumption of xanthate, insufficient to extract the target mineral, ensures hydrophobization, which is sufficient for the target mineral and insufficient for the waste rock minerals. For this reason, insoluble allyl ether of xanthate attaches mostly to hydrophobizated sites of the target mineral, which provides high selectivity of flotation [20].
This study aims to examine the influence exerted by the structure and composition of molecules of a collector on its collectability, and to analyze the effect of the physisorption mechanism of a single or mixed-type collector on coal recovery and concentrate quality.
Methods. The study used the available experimental materials on the influence exerted by the structure and composition of molecules of a collector on its flotation activity and selectivity. To this effect, the flotation activity of various collecting agents was correlated with the structure of their molecules. Then, transition from the structure and composition of the collector molecules to the collector activity in flotation was carried out using the physisorption mechanism of a collector [21]. The analysis of coal recovery in concentrate in correlation with the ash content of coal also used the physisorption mechanism.
First, the role of physisorption in the particle – bubble attachment was determined. The rupture of a water film between a particle and an air bubble involves formation of a local meniscus with an advancing contact angle θА. To dry the surface of the coal particle facing the bubble, it is necessary to fulfill the inequality
where σSG is the surface tension at the solid – gas interface; σSLis the surface tension at the solid – liquid interface; σLG is the surface tension at the liquid – gas interface.
Inequality (1) holds true when the contacting angle θА, formed after the water film rupture, reaches the value of the dynamic receding angle
The surface tension gradient of physisorbed surfactants at particles and bubbles defines the spreading rate of physisorbed collector on the water film surface. The surface tension gradient decomposes into two cofactors: G = (ꝺσ/ꝺС) (ꝺС/ꝺх). Here, ꝺσ/ꝺС characterizes the surface activity of long-chain (oxyhydryl, sulfhydryl, cation) collectors, and ꝺС/ꝺх describes the concentration gradient (ΔС) in the direction of the film movement. The concentration gradient is governed by the consumption of a collector and by the difference between the adsorption densities of the collector physisorption at the particle and at the air bubble. In this manner, the spreading rate is proportional to the surface activity of a physisorbed collector and to the difference of the physisorbed collector concentrations at the particle and at the bubble at the moment of the water film rupture between them:
where k is the constant of proportionality.
It follows from relation (2) that spreading rate increases with the higher surface activity of derivatives of a collecting agent and the greater its sorption on the extracted mineral with a simultaneous decrease on the bubble [22]. In flotation machines, the gas environment undergoes continuous refresh, and the surface concentration at the bubble may be assumed as zero for this reason. A high rate of spreading of a collector over the gas – liquid interface and the high rate of removal of water from the film interlayer reduces the induction time which unambiguously defines floatability. According to [23], the time of water removal from the film interlayer is the dominant factor in the mechanism of the particle – bubble attachment. Experiments have proved the connection between the time of induction and the combustible matter extraction in concentrate [24].
Connection of ash content and extractability of coal from the analysis of physisorption mechanism
In flotation,it is possible to improve the quality of a concentrate by using a weaker collector or by reducing the collector consumption [14, 22]. Let us discuss the way of increasing the flotation selectivity by changing consumption of a collector while maintaining its surface activity (ꝺσ/ꝺС const). An apolar collector at a smaller consumption will exhibit attachment in the form of drops predominantly to higher rank coal particles. The local rupture of the water film will take place at the physisorbed collector attachment site, i.e. at higher quality coal. A small consumption of a collector means no physisorption at ash particles and reduced adsorption at lower rank coal particles. As follows from equation (2), the low concentration of a collector will decrease ΔС, and will extend the transition time
An increased consumption of an apolar collector will increase its adsorption density at higher rank coal, but the sorption on oxidized coal will also increase. Due to the increased adsorption of the collector at coal and owing to its zero adsorption at air bubbles, ΔС will grow together with the higher recovery of all coal components. If its consumption is increased more, the apolar collector attachment at lower rank coal will grow, the latter will get in the concentrate, and the flotation froth will contain much ash. An increase in consumption of dodecane from 1 to 4 kg/t increased coal extraction, but at the same time at a dodecane consumption of more than 3 kg/t, the ash content of concentrate increased (Fig.1) [2].
Flotation of low-rank coal uses heteropolar collectors, for example, carboxylic acids. They increase recovery of combustibles by minimum 30 % compared to alkanes at the same consumption of the collectors (Fig.1) [2]. Increasing consumption of carboxylic acids can increase the concentrate yield. An increase in consumption of octenoic and dodecanoic acids improved the yield of the combustible matter but also substantially increased the ash content of the concentrate (Fig.1). This effect is especially noticeable in case of surface-active acids having a high rate of spreading over the gas – liquid interface (Fig.2).
A physically sorbable agent attaches preferably to hydrophobic surfaces and is a selective collector. It is effective at the gas – liquid interface irrespective of the coal surface condition and is not selective relative to target particles in this regard. Nonselectivity of physisorption grows with the increasing rate of a physisorbed collector spreading over water surface.
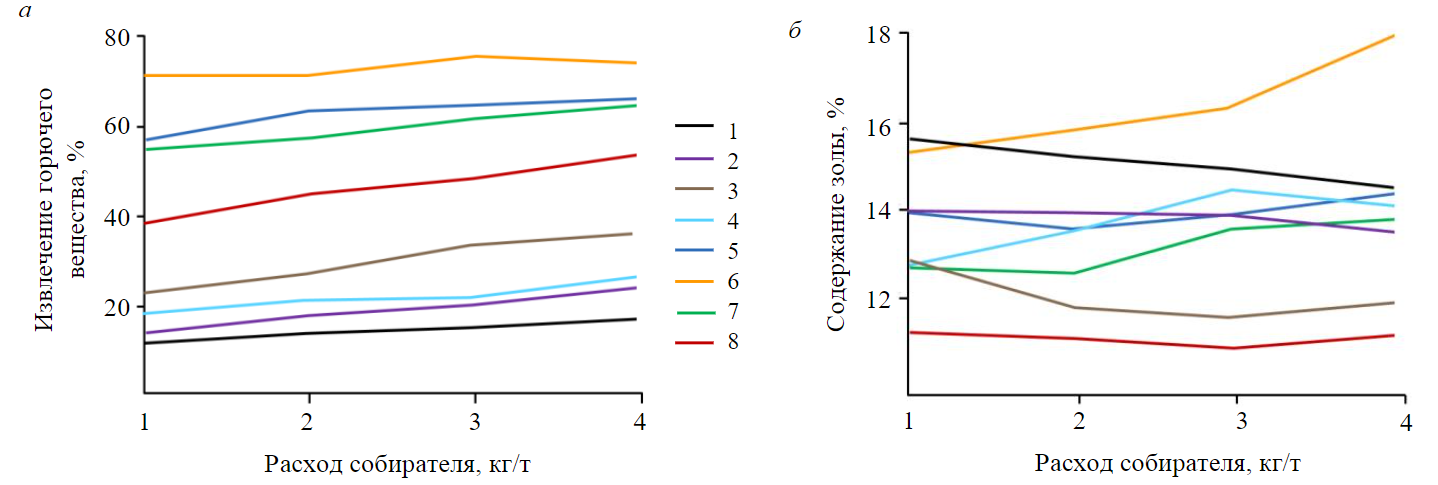
Fig.1. Flotation of coal with apolar collector and carboxylic acids:a – recovery of combustible matter; b – ash content of concentrate [2] 1 – n-pentane; 2 – n-octane; 3 – dodecane; 4 – n-hexadecane; 5 – n-pentanoic acid; 6 – n-caprylic acid; 7 – dodecanic acid; 8 – palmic acid
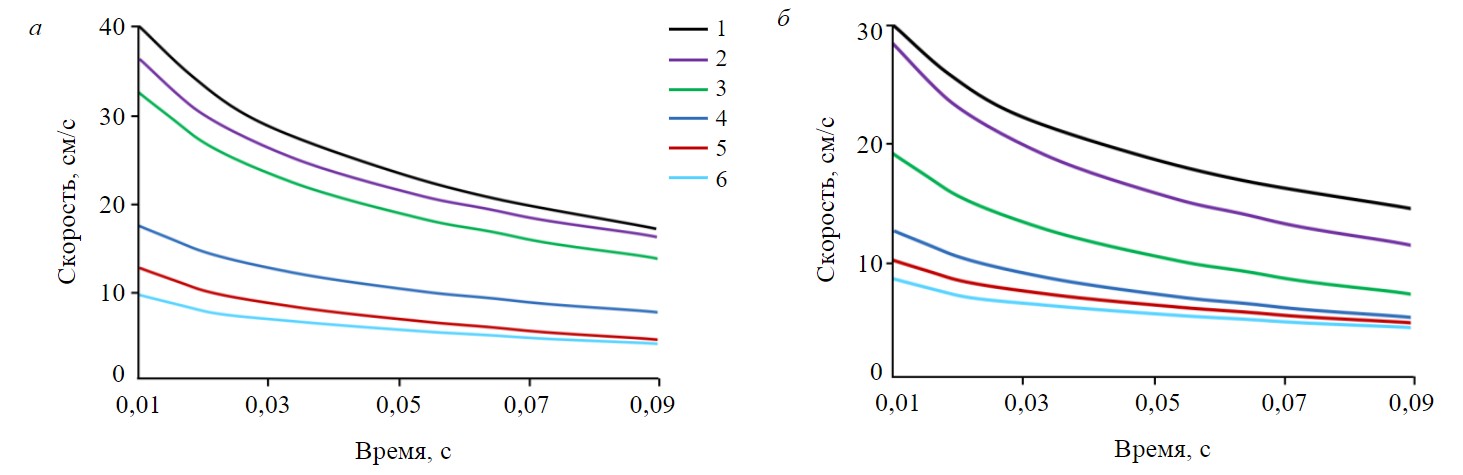
Fig.2. Spreading rate of carboxylic acids over water surface versus time: a – pH = 7; b – pH = 10 [25] 1 – hexoic acid; 2 – octenoic acid; 3 – decanoic acid; 4 – dodecanoic acid; 5 – tetradecanoic acid; 6 – palmitic acid
lowest content of ash in the concentrate among all test acids (see Fig.1, b). The low spreading rate and the long induction time result in the decreased recovery of the combustible matter (see Fig.1, a).
Octenoic acid, which has the maximal spreading rate (ꝺС/ꝺх and ΔС have optimal va-lues), offered the maximal extraction of the combustible matter and, because of nonselectivity of its physisorption, the high content of ash in the concentrate.
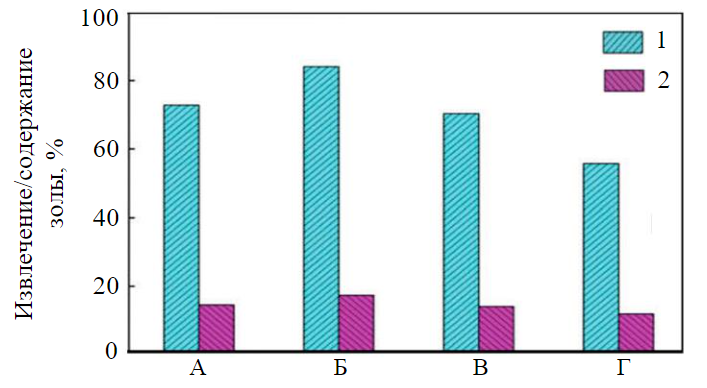
Fig.3. Collectability of carboxylic acids versus carbon atoms in hydrocarbon chain [2] A – n-pentanoic acid; B – n-octenoic acid; C – dodecanoic acid; D – tetradecanoic acid; 1 – extraction of combustible matter; 2 – ash content
Let us consider an approach to stimulating selectivity by changing the flotation activity of a collector at the preserved constant consumption ( const). The influence of hydrocarbon chain length (surface activity) of carboxylic acids on flotation performance was studied in [2]. The increase in the number of carbon atoms in hydrocarbon chain of an acid enhanced extraction of the combustible matter from 72 % in case of pentanoic acid and to 84 % in case of octenoic acid (Fig.3). The higher collecting ability results from the higher surface activity ꝺσ/ꝺС of acids with a longer hydrocarbon chain containing 10-12 atoms of carbon, and from the required value of ΔС being achieved. Carboxylic acids perform most actively in the neutral or weakly alkaline range of pH. For instance, oleinic acid generates surface-active dimers which achieve the maximal concentration when рН ~8. These dimers weaken the surface tension of the solution, generate the higher surface pressure at the moment of the water film rupture and, accordingly, accelerate water removal from the film.
Flotation of gas and coking coals using α-olefins was tested in [26]. The maximal yield of the concentrate was achieved with olefin containing 10 carbon atoms in hydrocarbon chain in case of gas coal and with olefin with 12 carbon atoms in case of coking coal [21]. The maximal yield of the concentrate was also obtained with a 12 carbon atoms chain in the radical [9]. Olefins with hydrocarbon fragments С11 and С12 showed an increased extraction of coal by 2-2.5 % as compared with alkanes [27]. As per the physisorption mechanism, an increase in extraction of a useful element is connected with the higher surface activity ꝺσ/ꝺС and, as a consequence, with the higher spreading rate of a surfactant over the gas – liquid interface (curve (2) in Fig.2), and with the sufficient value of ΔС. The transition time
Connection of molecule structure and composition with collectability and selectivity of collectors
Alkanes contain no hydrophilic groups and have a low surface tension: it varies from 20.3 mN/m for heptane to 25.35 mN/m for dodecane [28], which points at the higher surface pressure. However, there is no interaction between molecules of alkanes with zero dipole moment and polar molecules of water. For this reason, alkanes show no spreading over the gas – liquid interface [22]. The physisorption activity defined by the surface activity ꝺС/ꝺх and by the spreading rate is low. An advantage of alkanes consists in their selective attachment at hydrophobic surfaces according to the rule of polarity balancing between adjoining media. The strength of the physisorption attachment is not of vital importance. After the water film rupture, a physisorbed collector goes to bubble surface. When the content of mineral matters is low and the degree of oxidation is high, it is necessary to select a collector with the increased donor – acceptor interaction in surface tension [29].
It is found that a monoalkylate collector containing 89-98 % of isoparaffins features high selectivity [30]. The content of aromatic compounds and unsaturated hydrocarbons is less than 0.3 % and 1.1-1.7 %, respectively. Isoparaffins mostly represent branched compounds with non-zero dipole moment. High selectivity of such collectors is governed by the absence of hydrophilic groups.
Addition of a hydrophilic group into the collector molecule changes its interaction with water molecules, its solubility, and spreading rate. As a hydrocarbon fragment of saturated acids gets longer, their spreading rate lowers because of cohesion between molecules in the film (see Fig.2). The maximal spreading rate is a feature of the short-chain acid, but at small concentrations, the low molecular mass of the acids disables their adsorption at coal surface in a sufficient amount to ge-nerate the required value of ΔС. The increased molecular mass of the acids above 144 g/mole enables sufficient adsorption at coal and the increased ΔС. With further increase in the molecular mass and in the hydrocarbon chain length, the spreading rate of the acids over the gas – liquid interface will decrease (see Fig.2). The decrease in the spreading rate will increase the transition time
Initially, an increase in the surface activity (hydrocarbon chain length) to a certain value (octenoic and decanoic acids) leads to an increase in recovery (Fig.3). The further addition of atoms affects adversely on the recovery of combustible matter in concentrate due to cohesion of acid molecules and reduced spreading rate. The decrease in the spreading rate of acids over water surface weakens the physisorption effect in spreading. The chemisorption effect remains unchanged. The ratio of the chemisorption and physisorption effects grows; the quality of the concentrate improves accordingly, which is observed in flotation with dodecanoic and tetradecanoic acids (Fig.3) [31].
The dipole moment of a collector molecule characterizes the collector interaction with water molecules. For instance, in coal flotation with alkanes, alkenes and arenes (Table 1) [32], the rise of the dipole moment correlates with the yield of the concentrate and with the recovery of the combustible matter, while it has no correlation with the quality of the concentrate. The dipole moment characterizes the spreading rate of a collector over the gas – liquid interface, i.e. the physisorption activity.
Table 1
Performance of coal flotation with alkanes, alkenes and arenes
Collectors |
Yield, % |
Ash content, % |
Combustible matter recovery, % |
Dipole moment D |
Alkanes Olefins Arenes |
46.057.876.2 |
8.28.58.7 |
50.763.783.3 |
0 (octane)0.25 (octene)0.58 (ethyl benzene) |
The experimental results [2, 25, 31] imply: the increase of the surface activity and spreading rate over water surface in physisorption raises the ash content of concentrates.
A way of enhancing extraction of the combustible matter at the good quality of a concentrate is a combination of collectors having different values of ꝺσ/ꝺС. The flotation performance with alkane (dodecane) and ionic and anionic (oleinic acid) collectors is compared in [33]. Figure 4 depicts the combustible matter extraction and the concentrate ash content versus the collector consumption. The extraction with dodecane is comparatively low because of the weak surface activity of the latter re-lative to the gas – liquid interface. The collector spreading at the said interface takes place not due to interaction with water molecules but due to gravity. The increase in the consumption of dodecane only slightly enhances the yield of the concentrate as a consequence of the grown value of ΔС (Fig.4). The ash content of the concentrate lowers owing to the selective attachment of the apolar collector to the hydrophobic areas of coal surface and due to zero spreading of the collector over water surface. In case of oleinic acid, extraction is much higher thanks to the higher value of ꝺσ/ꝺС. The spreading rate of oleinic acid over water surface is more than 20 cm/s. The concentrate quality is low and the ash content is much higher than in flotation with dodecane as molecules of the acid are sensitive to oil and water and the acid physisorption is unselective. And, as said earlier, the physisorption effect is independent of the coal surface condition.
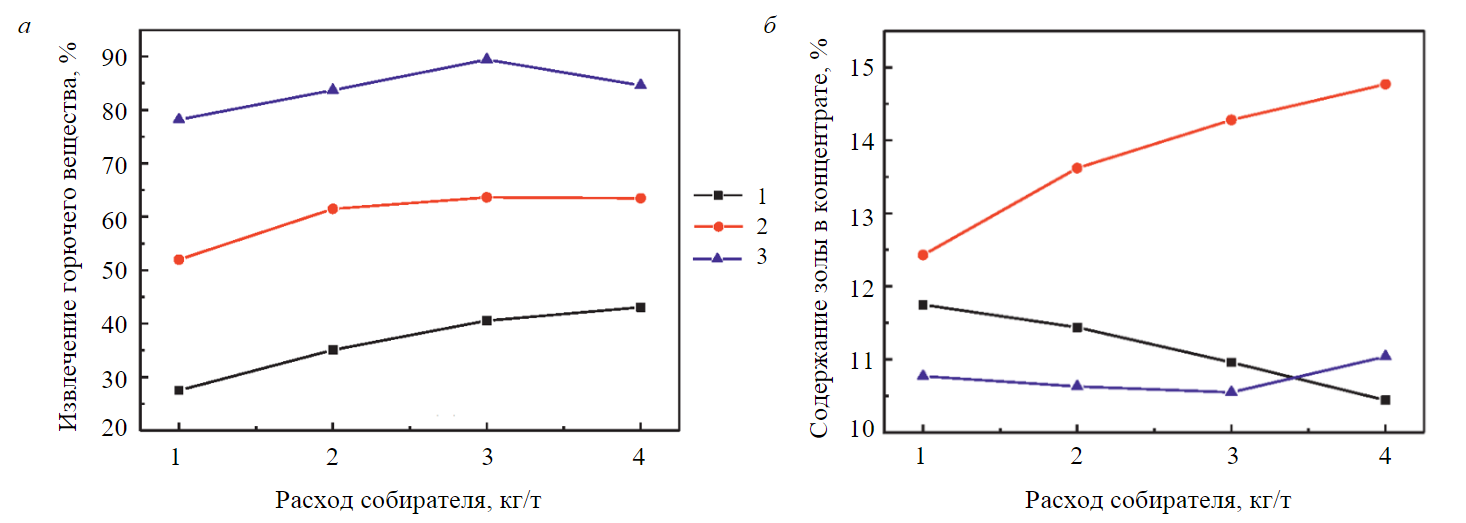
Fig.4. Performance of low-rank coal flotation versus consumption of a collector: a – extraction of combustible matter; b – ash content of concentrate [33] 1 – dodecane; 2 – oleinic acid; 3 – oleinic acid + dodecane
Selective extraction of low-rank coal Yozgat Ayridam was tested in flotation with kerosene, weakly soluble emulsifier, and nonionic surfactant Flotigol CS [12]. The surfactants somewhat promoted connection of the mixture with water molecules but supported no chemical interaction with the surface of hydrophobic coal. At the same time, the cation-active and anion-active substances lowered the concentrate quality. The use of nonionic surfactants is described in [34, 35].
A combination of collectors at moderate acid consumption increased the yield of concentrate and decreased its ash content (Fig.4). The reduced recovery of the combustible matter and the lower qua-lity of the concentrate at the collector consumption higher than 3 kg/t results from the entry of low-rank and impure coal in the froth product.
This example illustrates the fact that every collector performs its own function. Dodecane exhibits selective attachment in the form of drops to hydrophobic areas of coal surface. Oleinic acid with oil and water sensitive molecules is adsorbed by dodecane microdrops, i.e. at the oil – water interface, and enhances the surface activity of the mixture. The surface tension of dodecane – oleinic acid equals the acid surface tension [33]. This property of the mixture provides the wanted value of ꝺσ/ꝺС and the high spreading rate of the mix, i.e. the high extraction of the concentrate. Some acid molecules get attached to oxidized coal and enclosed minerals, and form linkages with their functional groups. In case of high acid consumptions, inorganic matters enter the concentrate. For dodecane and oleinic acid, their ratio in the mixture of collectors was selected as 4:1. In coal flotation with diesel fuel and oleinic acid, the maximal extraction of the combustible matter was reached at the collector ratio of 4:1 [36]. So, a heteropolar collector possessing high surface activity is adsorbed at the oil – water interface and improves the surface activity of a mixture.
The analytical reasoning shows that selectivity of an agent is governed by the ratio of different sorption mechanisms of the agent in removal of water from the film interlayer. An apolar agent is selectively adsorbed at the hydrophobic surface, but has a low surface activity and exhibits a low recovery. The heteropolar collector is attached at microdrops of an apolar collector, makes the mixture surface-active relative to the gas – liquid interface and enhances extraction. Optimizing the ratio of the sorption mechanisms allows a compromise between the quality and recovery in flotation [31, 37].
An increase in consumption of heteropolar frother Montanol 800 raised the ash content of the concentrates as the frother started interacting with rock-forming minerals and enhanced the surface activity ꝺσ/ꝺС of the frother and collector mixture [38]. The reduced extraction of the combustible matter in the concentrate at the increased consumption of the collector (more than 2.2 kg/t) is probably conditioned by the increased attachment of the collector at the target particles and by the decreased surface tension of bubbles down to minimum after attachment of the first particles. As a result, the surface tension gradient ΔС drops to a critical level when neither spreading of the agent nor removal of water from the film interlayer takes place.
The method of flotation improvement by combining collectors with different values of ꝺσ/ꝺС is also effective in case of composing apolar and cation-active collectors. The tests of low-rank coal floatability with diesel fuel combined with a cation-active collector of dodecyltrimethylammonium bromide (DTAB) proved low extraction in case of individual collectors [39]. The combination of the diesel fuel and cation-active collector at a ratio of 2:1 enabled the maximum yield of the concentrate with a low ash content of ~11 % (Fig.5).
The lab-scale tests of low-rank coal determined petrography and mineral composition of the combustible matter in the low-rank gas coal slack (Kuznetsk Coal Basin): ash content 45.10 %; reflectance 0.58 %; vitrinite 58 %; leuptinite 3 %; semivitrinite 8 %; fusinite 27 %; carbon 73.8 %; hydrogen 6.3 %; nitrogen 2.8 %; oxygen 17.5 %; mineral impurities. Tables 2 and 3 describe the grain size composition, fractional makeup of the test coal and the methods of testing, respectively.
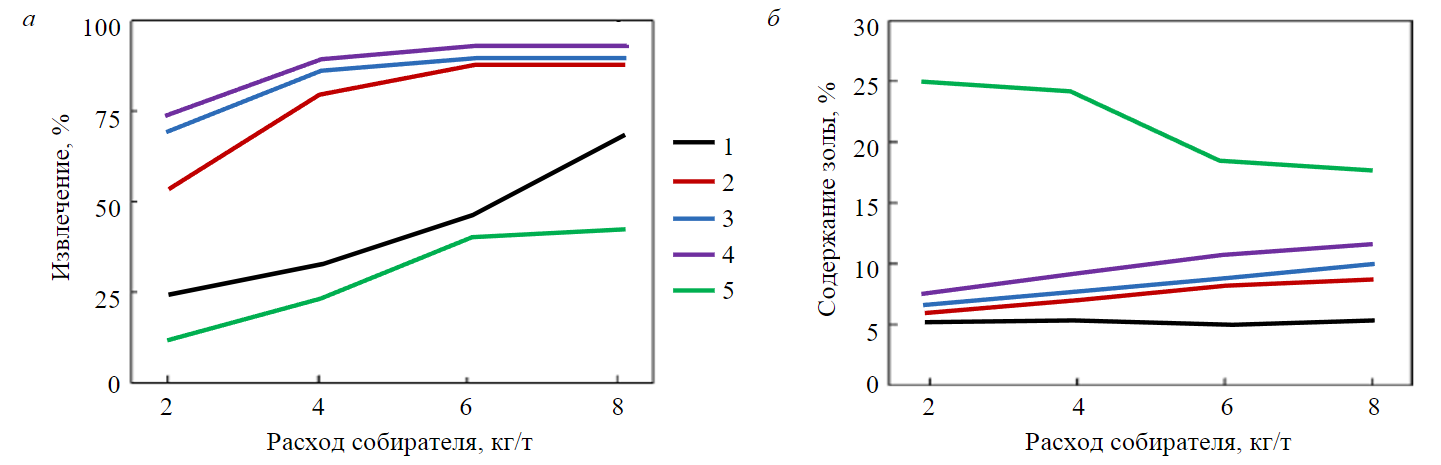
Fig.5. Flotation performance of low-rank coal versus consumption and ratio of collectors: a – recovery; b – ash content [39] 1 – 0:1; 2 – 1:10; 3 – 1:5; 4 – 1:2; 5 – DTAB
Table 2
Grain size composition and fractional makeup of low-rank gas coal slack (Kuznetsk Coal Basin)
Size grades, mm |
Yield, % |
Ash content, % |
Fraction density, kg/m3 |
Yield, % |
Ash content, % |
|
|
|
Less than 1400 |
27.00 |
5.30 |
0.2-0.5 |
37.00 |
44.42 |
1400-1500 |
12.78 |
9.78 |
0.1-0.2 |
17.40 |
49.80 |
1500-1600 |
5.23 |
20.40 |
0.05-0.1 |
7.70 |
45.60 |
1600-1800 |
1.79 |
36.44 |
0-0.05 |
37.90 |
43.50 |
More than 1800 |
53.20 |
76.51 |
Total |
100.00 |
45.10 |
Total |
100.0 |
45.10 |
Table 3
Lab-scale testing of low-rank coal
Test methods |
Equipment |
Coal slack petrology test in accordance with state standard GOST R 55662-2013 using SIAMS 620 analyzer in automatic mode. Liebig method estimation of carbon and hydrogen in accordance with state standard GOST 32979-2014 |
![]() |
Grain size distribution in accordance with state standard GOST 2093-82 using sieve analyzer MSA-W/D-200. Wet screening at aperture sizes of 0.5; 0.2; 0.1 and 0.05 mm
|
|
Fractional makeup of coal slack in centrifuge cup in accordance with state standard GOST 4790-2017; densities of 1300; 1400; 1500; 1600; 1800 kg/m3 |
|
Flotation tests on Mekhanobr machine 136A-FL-1, cell volume of 0.5 l, impeller speed 1500 rpm, constant pulp temperature in a range of 19-20 °С. Coal slack size not larger than 0.5 mm, solid content of 100 g/l. Pre-flotation soaking of all test samples for 5 min and loading together with soak water in flotation machine. Flotation time 10 min (complete flotation). One-time feed of agents. Sampling of frother product (flotation concentrate) as bulk concentrate. Flotation performance: flotation concentrate yield gc; flotation concentrate ash content Аdc; combustible matter extraction Еc.m; tailings ash content Adt, Аdc = (m3 – m1)/(m2 – m1), where m1 – mass of roasted boat, g; m2 – mass of boat with coal sample, g; m3 – mass of boat with post-roasting residue, g; Еc.m = γc(100 – Аdc)/(100 – Аdin), Аdin – ash content of initial coal slack, %; Аdt = (100Аdin – γсАdc)/gt, γt – flotation tailings, % |
|
Group analysis of agents using mass-fragmentographic assay (selective ion detection) and software Mass Spectrometry Data Handling System |
|
Estimation of agent spreading rate in water-filled pan placed under high-speed camera EVERCAM 1000-16-С. Video filming at resolution 1280×304 and speed of 3845 snap/s
|
|
The flotation tests of gas coal slack in the framework of this study proved the trend (Table 4). An apolar collector attaches more selectively to a hydrophobic surface, and a heteropolar collector enables the mixture to interact with water molecules, spreads over water surface and reduces the time of induction. Coal extraction to concentrate grew from 74.18 % in case of dodecane to 78.11 % in case of a mixture of the mentioned collectors, and the ash content of the concentrate lowered from 20.34 to 20.09 %.
Table 4
Flotation of gas coal slack with apolar and heteropolar collectors, and with their combination
Agents |
Agent consumption, g/t |
Initial ash content, % |
Concentrate |
Tailings |
Extraction of combustible matter to concentrate, % |
||
Yield, % |
Ash content, % |
Yield, % |
Ash content, % |
||||
Dodecane |
300 |
45.10 |
51.12 |
20.34 |
48.88 |
70.99 |
74.18 |
2-octanol |
300 |
45.10 |
36.72 |
22.49 |
63.28 |
58.22 |
51.84 |
Dodecane |
210 |
45.10 |
53.66 |
20.09 |
46.34 |
74.06 |
78.11 |
2-octanol |
90 |
||||||
Hexane |
300 |
45.10 |
9.06 |
20.96 |
90.94 |
47.50 |
13.04 |
Nonane |
300 |
45.10 |
42.74 |
21.23 |
57.26 |
62.92 |
61.32 |
Many studies attempt to enhance flotation performance by combining collectors with similar surface properties. For example, the study into effect of benzene ring in hydrocarbon fragment of the collector on flotation of coal used two surfactants – oxyethylated nonylphenol NPEO and oxyethylated dodecyl ether C12EO [4]. The main apolar collector was kerosene. Pretreatment of coal with NPEO and C12EO increased the extraction from 49.39 to 81.34 and to 83.96 %, respectively. The quality of the concentrate was improved too. Extraction of higher rank coal was somewhat higher with NPEO than with C12EO. In the listed agents, the oxyethyl groups are arranged similarly at the beginning of the hydrocarbon fragment. The hydrocarbon chain of C12EO contains 12 carbon atoms. The hydrocarbon chain of NPEO contains 9 atoms of carbon, but the benzene ring is at the beginning of radical, and the surface activity of the ring is equal to 3.5 СН2-groups of the main unbranched chain [40]. In this manner, the surface activities of these compounds are equal. Nearly similar extraction in case of these collectors is explained by coincidence of their ꝺσ/ꝺС and ΔС, i.e. by their equal spreading rates as per (2).
Results and discussion
The comparative analysis has exhibited correlation between flotation activity and molecular structure of different collectors. The physisorption mechanism in flotation activity allows transition from the structure of molecules to their composition. The flotation activity of an agent depends on the surface activity ꝺσ/ꝺС of the agent, on the difference of the agent adsorption density ΔС at particles and bubbles, and on the cohesion between molecules of the collecting agent. These characteristics are defined by the structure and composition of hydrocarbon fragment. The high flotation activity of collectors having 8-12 carbon atoms chains is explained by the optimal values of ꝺσ/ꝺС and ΔС, and by the low cohesive forces. The double linkage and hydrocarbon radical branching require more atoms of carbon in radical. Activity of many chemical compounds is either known or found experimentally, which enables prediction of their collecting properties. The hydrophilic group of a heteropolar collector governs its connection with water molecules, the value of ꝺσ/ꝺС, as well as the possibility to catch water and remove it from the film interlayer between coal particle and gas bubble. Chemical compounds lacking the hydrophilic group have low collectability because of zero interaction with water molecules.
It is possible to enhance selectivity of coal recovery by coal hydrophobization. This statement is proved by selective attachment of alkanes, with their molecules free from the hydrophilic groups, to the organic phase of coal (see Fig.1). Nonselective attachment of molecules of carboxylic acid worsens the concentrate quality, but their surface activity ꝺσ/ꝺС defines the spreading rate of a mixture of collectors and the velocity of water removal from the film interlayer between the objects of interaction in accordance to (2).
Combining collectors with different physical properties allows to achieve the wanted performance of concentrate yield and quality. An apolar collector at moderate consumption attaches selectively to hydrophobic surface of coal in accordance with the rule of polarity balancing between adjoining media. The spreading rate of such collector is relatively low, which explains its low collecting ability (see Fig.1). A heteropolar collector possesses a high surface activity and velocity of water removal from the film, which is reflective of its high collectability. At the same time, this collector exhibits attachment to mineral impurities in coal, which explains the high resultant ash content of the concentrate (see Fig.1). Adsorption of the heteropolar collector at the surface of the apolar collector at the oil – water interface adds the high surface activity and collectability to the mixture. Thus, the surface activity of a physically sorbable collector characterizes the recovery of the combustible matter, and the physically adsorbed apolar collector largely influences the concentrate quality.
Selectivity of a collector is governed by the ratio of the activities of the heteropolar and apolar compounds in the mixed-type collector relative to the gas – water surface, and by the ratio of their consumptions. The physisorption activity is understood as the efficiency of the physically adsorbable collector during its spreading, or as the thickness of its surface flow at the gas – water interface. The increase in the surface activity of the physisorbed heteropolar collector increases the ratio of functions performed by the heteropolar and apolar collectors in terms of removal of water from the film interlayer. The improved selectivity of a collector is achieved via changing the ratio of effects in water removal from the film by chemical compounds with different surface activities. Optimization of both ratios provides a compromise between the recovery and quality in flotation [37]. The illustration of the recovery – quality relation in a concentrate of the combustible matter is provided (see Fig.3).
Conclusion
The analysis of the experimental data on the physisorption mechanism has revealed the connection between the flotation activity of collectors and the structure and composition of their molecules. The combination of an apolar collector, attached as the drops to a hydrophobic surface, and a heteropolar collector, which adds up surface activity of the mix, enables a higher extraction of coal to concentrate at low ash content. The apolar collector at a moderate consumption exhibits attachment by the rule of polarity balancing of adjoining media and ensures selectivity of sorption, while the heteropolar collector, which has a high surface activity, is adsorbed at the oil – water interface and enhances recovery of the useful element.
Owing to the high surface activity, at the moment of rupture of a film between the coal particle and air bubble, the surfactant mixture of the heteropolar and apolar collectors removes water from the film, reduces the time of induction, and increases extraction of the combustible matter. The improved recovery of coal in concentrate with low ash content is achieved by optimizing the ratio of effects exerted on removing water from the film interlayer by the different sorption forms of an agent. Such function optimization provides a compromise between the recovery and quality in flotation [37].
References
- Linev B.I. Theoretical justification and development of rational processing technologies for coal-based fuels using an energy approach: Avtoref. dis. ... d-ra tekhn. nauk. M.: Izd-vo Moskovskogo gosudarstvennogo gornogo universiteta, 2003, p. 48 (in Russian).
- Quanzhi Tian, Yi Zhang, Guosheng Li, Yongtian Wang. Application of carboxylic acid in low-rank coal flotation. International Journal of Coal Preparation and Utilization. 2019. Vol. 39. Iss. 1, p. 44-53. DOI: 10.1080/19392699.2017.1297299
- Baofeng Wen, Wencheng Xia, Sokolovic J.M. Recent advances in effective collectors for enhancing the flotation of low rank/oxidized coals. Powder Technology. 2017. Vol. 319, p. 1-11. DOI: 10.1016/j.powtec.2017.06.030
- Bao Li, Jianying Guo, Shengyu Liu et al. Molecular insight into the mechanism of benzene ring in nonionic surfactants on low-rank coal floatability. Journal of Molecular Liquids. 2020. Vol. 302. N 112563. DOI: 10.1016/j.molliq.2020.112563
- Akdemir Ü., Sönmez İ. Investigation of coal and ash recovery and entrainment in flotation. Fuel Processing Technology. 2003. Vol. 82. Iss. 1, p. 1-9. DOI: 10.1016/S0378-3820(02)00248-5
- Jena M.S., Biswal S.K., Rudramuniyappa M.V. Study on flotation characteristics of oxidised Indian high ash sub-bituminous coal. International Journal of Mineral Processing. 2008. Vol. 87. Iss. 1-2, p. 42-50. DOI: 10.1016/j.minpro.2008.01.004
- Temel H.A., Majumder A.K. Investigation of the effectiveness of desliming and flotation in cleaning Malatya-Arguvan lignite. Energy Sources, Part A: Recovery, Utilization, and Environmental Effects. 2016. Vol. 38. N 8, p. 1048-1054. DOI: 10.1080/15567036.2015.1120819
- Wencheng Xia, Chao Ni., Guangyuan Xie. Effective flotation of lignite using a mixture of dodecane and 4-dodecylphenol (DDP) as collector. International Journal of Coal Preparation and Utilization. 2016. Vol. 36. Iss. 5, p. 262-271. DOI: 10.1080/19392699.2015.1113956
- Kadagala M.R., Nikkam S., Tripathy S.K. A review on flotation of coal using mixed reagent systems. Minerals Engineering. 2021. Vol. 173. N 107217. DOI: 10.1016/j.mineng.2021.107217
- Dey S. Enhancement in hydrophobicity of low rank coal by surfactants – A critical overview. Fuel Processing Technology. 2012. Vol. 94. Iss. 1, p. 151-158. DOI: 10.1016/j.fuproc.2011.10.021
- Jia R., Harris G.H., Fuerstenau D.W. Chemical reagents for enhanced coal flotation. Coal Preparation. 2002. Vol. 22. Iss. 3, p. 123-149. DOI: 10.1080/07349340213847
- Cebeci Y. The investigation of the floatability improvement of Yozgat Ayrıdam lignite using various collectors. Fuel. 2002. Vol. 81. Iss. 3, p. 281-289. DOI: 10.1016/S0016-2361(01)00165-X
- Zechen Liu, Yinfei Liao, Maoyan An et al. Enhancing low-rank coal flotation using a mixture of dodecane and n-valeraldehyde as a collector. Physicochemical Problems of Mineral Processing. 2019. Vol. 55 (1), p. 49-57. DOI: 10.5277/ppmp18109
- Kubak D.A., Petuсhov V.N., Semenov D.G. The investigation of the effect of complex reagents sectional chemical composition on coal flotation efficiency. Vestnik of Nosov Magnitogorsk State Technical University. N 4 (44), p. 5-10 (in Russian).
- Abramov A.A. Requirements to the choice and designing of selective reagents-collectors. Part 2. Requirements to physical-chemical properties of selective reagents-collectors. Tsvetnye Metally. N 5, p. 14-17 (in Russian).
- Miller J.D., Wang X., Li M. Bench Scale Flotation of Sedimentary Phosphate Rock with Hydroxamic Acid Collectors. Beneficiation of Phosphates: Advances in Research and Practice. Englewood: Society for Mining, Metallurgy & Exploration, 2002, p. 93-101.
- Miller J.D., Xuming Wang, Minhua Li. Patent N US6341697B1. Selective flotation of phosphate minerals with hydroxamate collectors. Publ. 29.01.2002.
- Xuming Wang, Nguyen A.V., Miller J.D. Selective attachment and spreading of hydroxamic acid-alcohol collector mixtures in phosphate flotation. International Journal of Mineral Processing. 2006. Vol. 78. Iss. 2, p. 122-130. DOI: 10.1016/j.minpro.2005.09.005
- Aleksandrova T.N., Elbendari A.M. Increasing the efficiency of phosphate ore processing using flotation method. Journal of Mining Institute. Vol. 248, p. 260-271. DOI: 10.31897/PMI.2021.2.10
- Kondratev S.A., Semyanova D.V. Relation between Hydrocarbon Radical Structure and Collecting Abilities of Flotation Agent. Journal of Mining Science. Vol. 54. N 6, p. 1024-1034. DOI: 10.1134/S1062739118065180
- Kondratev S.A. Selecting collecting agents for flotation. Journal of Mining Science. N 5, p. 109-124 (in Russian). DOI: 10.15372/FTPRPI20220511
- Kondratev S.A. Collectability and Selectivity of Flotation Agent. Journal of Mining Science. Vol. 57. N 3, p. 480-492. DOI: 10.1134/S1062739121030133
- Seongsoo Han, Nguyen A.V., Kwanho Kim et al. Quantitative Analysis of Attachment Time of Air Bubbles to Solid Surfaces in Water. Langmuir. 2021. Vol. 37. Iss. 2, p. 616-626. DOI: 10.1021/acs.langmuir.9b02773
- Lijuan Sun, Yaowen Xing, Haichang Yang et al. A New Experimental Approach to Evaluate Coal Particles Floatability: Bubble-Particle Attachment and Detachment Kinetics. ACS Omega. 2020. Vol. 5. Iss. 27, p. 16733-16738. DOI: 10.1021/acsomega.0c01630
- Kondratyev S.A., Semyanova D.V. A revisit of selection the efficiency criterion for flotation reagents of fatty acids class. Eurasian Mining. 2017. N 1, p. 24-29. DOI: 10.17580/em.2017.01.07
- Lavrinenko A.A., Svechnikova N.Y. The research of quantum-chemical characteristics in selection of coals flotation reagents. Vestnik Magnitogorsk State Technical University named after G.I.Nosov. N 1 (21), p. 85-87 (in Russian).
- Chuprova L.V. Studying of the mechanism of effect of reagents at floatation enrichment of coal slimes. International Journal of Applied and Fundamental Research. N 11. Part 5, p. 939-942 (in Russian).
- Ozdemir O., Karaguzel C., Nguyen A.V. et al. Contact angle and bubble attachment studies in the flotation of trona and other soluble carbonate salts. Minerals Engineering. 2009. Vol. 22. Iss. 2, p. 168-175. DOI: 10.1016/j.mineng.2008.06.001
- Xiahui Gui, Yaowen Xing, Tingxia Wang et al. Intensification mechanism of oxidized coal flotation by using oxygen-containing collector α-furanacrylic acid. Powder Technology. 2017. Vol. 305, p. 109-116. DOI: 10.1016/j.powtec.2016.09.058
- Petukhov V.N., Skorobogatova A.A., Ilyasova A.Z. Development reagent conditions of coal flotation using reagents-collectors of different chemical composition. The Theory and Process Engineering of Metallurgical Production. Vol. 20. N 1, p. 16-19 (in Russian).
- Kondratev S.A. Physisorption and its purpose in flotation. Novosibirsk: Nauka, 2018, p. 182 (in Russian).
- Osina N.Yu., Gorokhov A.V., Lakhtin S.V. Investigation of the influence of the group chemical composition of agents collectors on the efficiency of bituminous coal flotation. Gornyi informatsionno-analiticheskii byulleten. 2006. N 2, p. 393-396 (in Russian).
- Yinfei Liao, Xiaodong Hao, Maoyan An et al. Enhancing low-rank coal flotation using mixed collector of dodecane and oleic acid: Effect of droplet dispersion and its interaction with coal particle. Fuel. 2020. Vol. 280. N 118634. DOI: 10.1016/j.fuel.2020.118634
- Wencheng Xia, Jianguo Yang. Enhancement in flotation of oxidized coal by oxidized diesel oil and grinding pretreatment. International Journal of Coal Preparation and Utilization. 2013. Vol. 33. Iss. 6, p. 257-265. DOI: 10.1080/19392699.2013.816300
- Ceylan K., Küçük M.Z. Effectiveness of the dense medium and the froth flotation methods in cleaning some Turkish lignites. Energy Conversion and Management. 2004. Vol. 45. Iss. 9-10, p. 1407-1418. DOI: 10.1016/j.enconman.2003.09.011
- Dube R., Honaker R. Improving the flotation performance of an oxidized bituminous coal source. Minerals Engineering. 2019. Vol. N 105937. DOI: 10.1016/j.mineng.2019.105937
- Kondratev S.A., Rostovtsev V.I., Bochkarev G.R. et al. Justification and development of innovative technologies for integrated processing of complex ore and mine waste. Journal of Mining Science. Vol. 50. N 5, p. 959-973. DOI: 10.1134/S1062739114050160
- Alexandrova T.N., Kuskov V.B., Afanasova A.V., Kuznetsov V.V. Improvement of the fine coking coal flotation technology. Obogashchenie rud. N 3, p. 9-13 (in Russian). DOI: 10.17580/or.2021.03.02
- Rui Zhang, Yangchao Xia, Fangyu Guo et al. Effect of microemulsion on low-rank coal flotation by mixing DTAB and diesel oil. Fuel. 2020. Vol. 260. N 116321. DOI: 10.1016/j.fuel.2019.116321
- Rosen M.J. Reduction of Surface and Interfacial Tension by Surfactants. Surfactants and Interfacial Phenomena. Hoboken: John Wiley & Sons, 2004, p. 208-242. DOI: 10.1002/0471670561.ch5