Morphometric parameters of sulphide ores as a basis for selective ore dressing
- 1 — Ph.D. Associate Professor Saint Petersburg Mining University ▪ Orcid
- 2 — Ph.D., Dr.Sci. Head of Department Saint Petersburg Mining University ▪ Orcid
- 3 — Ph.D., Dr.Sci. Director of the Institute of Mineral Processing Machines Freiberg Mining Academy ▪ Orcid
- 4 — Ph.D. Associate Professor Saint Petersburg Mining University ▪ Orcid
Abstract
To assess the possibility of selective disintegration and reduction of overgrinding of hard-to-reproduce ores, optical microscopic and X-ray microtomographic studies were carried out and quantitative characteristics of morphological parameters of disseminated and rich cuprous ore samples from Norilsk-type Oktyabrsky deposit were identified. Among quantitative morphological parameters the most informative are area, perimeter, edge roughness, sphericity, elongation and average grain spacing for disseminated copper-nickel ores; area, perimeter, edge roughness and elongation for rich cuprous ores. The studied parameters are characterized by increased values and dispersion in ore zones, which is especially important for fine-grained ores, which are difficult to diagnose by optical methods. Three-dimensional modelling of the internal structure of sulphide mineralisation samples was carried out using computed X-ray microtomography, which allows observation of quantitative parameters of grains, aggregates and their distribution in the total rock volume and interrelationship with each other. The evaluation of rock pore space by computer microtomography made it possible to compare the results obtained with the strength characteristics of rocks and ores, including those on different types of crushers. The obtained quantitative characteristics of structural-textural parameters and analysis of grain size distribution of ore minerals allow us to evaluate the possibility of applying selective crushing at various stages of ore preparation
Introduction
In the modern world, the proportion of finely crushed, resistant raw materials is increasing, which requires the development of new approaches and technologies in the process of ore dressing [1, 2]. Disintegration processes applied at the initial stage of beneficiation allow extraction of the valuable components in a more economical and environmentally friendly way and thus increase the profitability of ores and deposits [3-5]. The complexity of finely disseminated sulphide ores processing is caused by the difficulty of opening of clusters of valuable components at disintegration for their extraction in the conditioned quality commercial products [6, 7]. It is known that the main task of disintegration of refractory ore is to break the object at interfaces without overgrinding at minimization of power inputs [8]. The currently known methods do not provide sufficient disclosure of grains of valuable components, and the destruction of material occurs in bulk [9, 10], which leads to losses of valuable components and reduces the technological indicators of further ore processing [11, 12].
To realise selective disintegration, a detailed study of the relationship between structural phase and material composition and the physical, physic-chemical and technological properties of minerals is necessary [13, 14]. Usually, geologists describe the structure and texture of rocks and ores through the analysis of thin sections or polished sections [15-17]. To obtain morphometric structural-textural characteristics of rocks and ores, the optical methodhas limitations, as it is two-dimensional and can only give a qualitative description. To obtain more detailed, including quantitative, information about minerals, it is necessary to find the relationships between the relevant fracture parameters, product characteristics and inherent technological-mineralogical and structural-textural features, which is an urgent task in the study of selective disintegration processes and the development of apparatuses for their implementation [18, 19].
Currently, new 3D modelling tools and measuring morphometric characteristics of rocks and ores are being actively developed [20, 21]. In this connection, the main purpose of this work was to investigate the possibilities of applying modern means of measurement and analysis of optical and microscopic data, Thixomet Pro software (Russia) [22] and computer X-ray microtomography data processing programs [23, 24] to study the structural and textural characteristics on the example of sulphide copper-nickel ores of the Norilsk ore district.
Geological structure of the Norilsk ore district
Typical deposits of sulphide copper-nickel ores in the Norilsk ore district were chosen as objects of study [25, 26]. The geological position of the Norilsk deposits has been well studied and described in numerous papers [27, 28].
The Norilsk ore district is located in northern Siberia at the junction of the Siberian Platform with the Tunguska syncline and theEnisei-Khatanga Trough (Fig.1). Sill-like intrusions of gabbro-dolerites, leucogabbro and gabbro-diorites, bearing platinum-copper-nickel mineralization, were introduced into the continental terrigenous and coal-bearing sediments of the Tunguska Group of age C2-P2, according to the trappean basalt formations of age P2 -T1 [29, 30]. The intrusive complexes distribution is controlled by the Norilsk-Kharaelakh fault: the Kharaelakh and the Talnakh intrusives are located in the southern part of the Kharaelakh depression. The Talnakh intrusive is located in the southern part of the Kharaelakh Trough and is associated with the cross junction of the Norilsk-Kharaelakh fault with the Pyasin dome-shaped uplift, while the Norilsk intrusive is located in the northern part of the Norilsk Trough.
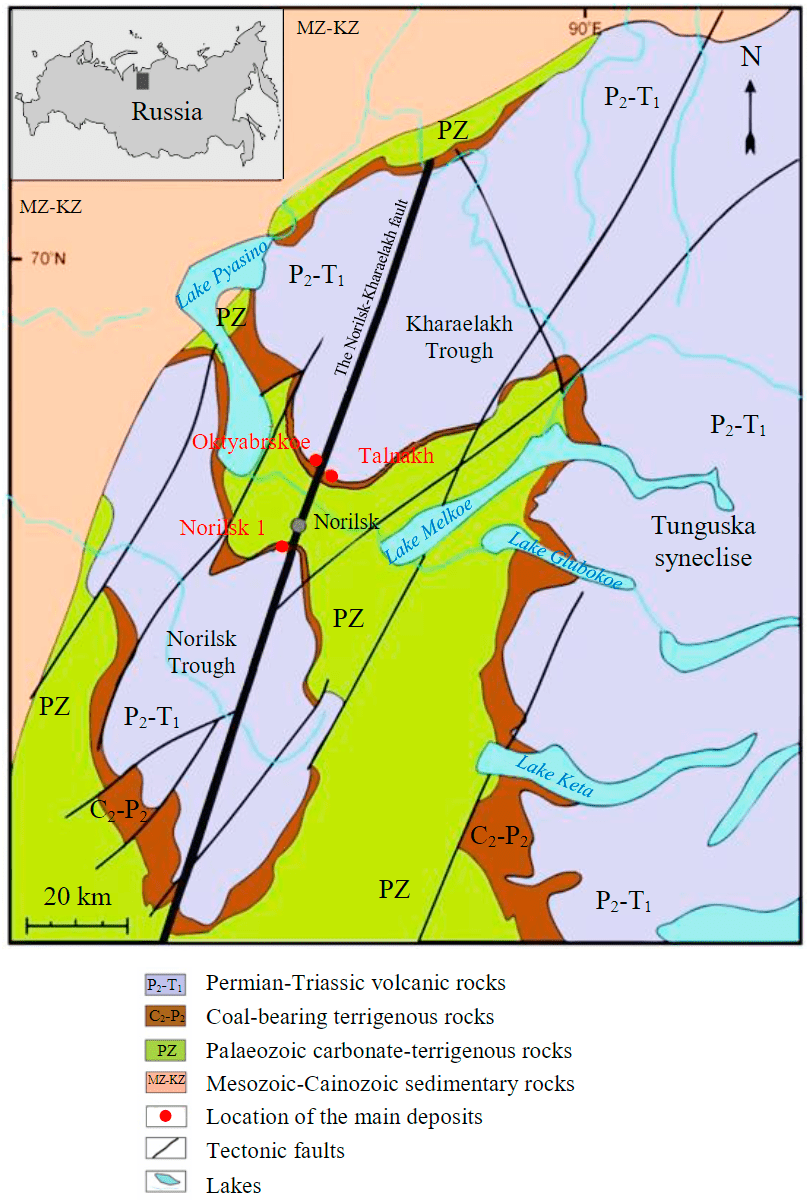
Fig.1. Geological structure of the Norilsk ore district [31, with changes], the inset shows the position of the Norilsk ore district in Russia
At present, 96 % of metals produced at the Norilsk Mining and Metallurgical Plant are extracted from ores of the Talnakh intrusive complex and only 4 % are extracted from poor ores of the Norilsk intrusive complex. Four types of ore-bearing intrusions are distinguished in the Norilsk ore district: Norilsk, Zubovsky, Kruglogorsky and Nizhnetalnakhsky [32]. Intrusive complexes of the Norilsk type of fully differentiated intrusions provide all commercial copper and nickel deposits in the Norilsk region, while the Zubovsky, Kruglogorsky and Nizhnetalnakhsky types accompany Norilsk, being less differentiated and ore-bearing, but more abundant. Intrusions of the Norilsk type are differentiated from gabbro-dolerites of the normal series to taxitic and picritic gabbro-dolerites with ore phenocrysts in the lower part of the intrusion and to leucogabbro and gabbro-diorites in the upper part. The lower part of differentiated intrusions of the Norilsk type is associated with bedded bodies of massive copper-nickel sulphide ores, located in the surrounding hornfels and the intrusion body as lenses and bedded deposits. There are also bodies of complex morphology, represented by massive, breccia-like, densely disseminated so-called “cuprous ores” [28, 30]. The main sulphide minerals in the ore bodies are chalcopyrite, pyrrhotite, pentlandite and pyrite [33, 34]. Secondary minerals include galena, valleriite, millerite, mackinawite, argentopentlandite and bornite. Ores of this composition are complex and diverse when studying morphometric parameters for selective extraction, which is especially complicated by the presence of fine grains of platinum minerals in the ore composition.
The present study analysed typical samples of disseminated copper-nickel and rich cuprous ores of the Norilsk type of the Oktyabrskoe deposit Norilsk ore district (central and western flanks of the Kharaelakh intrusion, respectively).
The disseminated copper-nickel ores of the central flanks of the Kharaelakh intrusion (21 samples, 63 thin sections) are differentiated mainly from poikilophytic to taxitic and picritic gabbro-dolerites with massive ore phenocrysts in the lower part of the intrusion. In the upper parts of intrusives, interstitial sulphide phenocrysts in leucogabbro; below – drop-shaped, less frequently sideronite phenocrysts in gabbro-dolerites and picrite dolerites; in the middle lower part of intrusives – nests of irregular shape in taxitic gabbro-dolerites; in the bottom parts of intrusives – massive pentlandite-talnakhite ore on the contact with brecciated hornfels.
The rich cuprous ores of the western flanks of the Kharaelakh intrusion (23 samples, 69 thin sections) are less differentiated compared to the central parts of the Kharaelakh intrusion. With approaching the massive sulphide ore, ore minerals form large allotriomorphic-grained aggregates that take the form of interstitials between pyroxene crystals and plagioclase leists, individual ore minerals are drop-shaped and begin to accumulate and form nests. In the marginal parts of the intrusive in the described section, there are no leucocratic varieties, and taxitic varieties predominate.
Methodology
Samples of disseminated copper-nickel and rich massive ores were studied using optical and microscopic methods with subsequent image processing using Thixomet Pro Software, as well as computer X-ray microtomography methods.
Opto-microscopic investigations were carried out using an Axio Imager A2m (Zeiss) optical polarisation microscope. The obtained images were then processed and analysed using Thixomet Pro image analysis Software, which has been metrologically certified as a measuring instrument [35]. For the study, oriented petrographic thin sections were made in mutually perpendicular slices according to the method [13].
Then, the panoramas of the thin sections were created and the grains of the ore minerals were automatically captured. Then the morphometric parameters of the grains quantitatively reflecting the structural-textural features of the ores were automatically calculated using the Thixomet Pro Software. The main of these parameters are: area A, μm2; perimeter P, μm; diameter (minimum, maximum, average), μm; length L and width B, μm (calculated with P = 2(L + B), A = LB); shape factor, a unit fraction (4πA/P2); continuity, a unit fraction (ratio of real and convex area); elongation, a unit fraction (L/B); edge roughness/roughness, a unit fraction (P/Rс, where Pс is convex perimeter); average distance between grain centres, µm (real area/number of grains).
X-ray tomography studies and visualisation of the internal three-dimensional structure of the oriented samples were performed using a Bruker SkyScan-1173 micro-CT scanner (Belgium), equipped with NRecon, DataViewer, CTAn and CTVox Software. Cylinders with a diameter of 2 cm were cut out for imaging. Imaging parameters: accelerating voltage 130 kV, current strength 60 mA, resolution 17 µm, glass filter 0.25 mm, rotation step 0.3 deg.
The methodology of computed X-ray tomography studies is described in detail in [36, 37]. The essence of the X-ray method is to reduce the power of X-ray radiation during its passage through the definite volume of the sample due to the photoelectric absorption of X-rays. The absorption is detected by the detector by creating shadow projections – graphic files in which each pixel contains information about the amount of absorbance at a specific point. The result of such scanning is a series of X-ray images, which are then converted into 3D images for further visual studies and morphometric analysis [7, 32]. Significant differences in the density of plagioclase (≈ 2.7 g/cm3), clinopyroxene (3.1-3.5 g/cm3), ore minerals (chalcopyrite ~4.2 g/cm3, pyrrhotite 4.6-4.7 g/cm3, ilmenite 4.8 g/cm3, magnetite to 5.2 g/cm3, marcasite 4.85-4.9 g/cm3, titanomagnetite 4.8-5.3 g/cm3), and platinum group minerals (18-21 g/cm3) made it possible to obtain a realistic picture of the distribution of the densest phases on a scale from a few centimetres up to 15-20 µm resolution [16, 25].
Morphometric parameters of grains calculated by computed X-ray microtomography: area, μm2; perimeter, μm; length L, μm (diameter of the circle of equivalent area/maximum distance between two points of the object); width B, μm (minimum distance between two points of the object); sphericity, unit fraction (ratio of ball surface of equivalent volume to the surface); specific surface (roughness), mm2/mm3 (ratio of total surface to volume); elongation, unit fraction (L/B); edge roughness/roughness, unit fraction (P/Rс); closed porosity, % (proportion of area completely enclosed); effective porosity, % (proportion of area not completely enclosed); total porosity, % (sum of closed and effective porosity).
The chemical composition of ores was determined by energy dispersive X-ray fluorescence analysis, and PGE (platinum group elements: platinum and palladium) contents were estimated by inductively coupled plasma mass spectrometry method using ELAN DRC mass spectrometer of Perkin Elmer company in the central chemical laboratory of MMC Norilsk Nickel. Laboratory tests were carried out at the benefication facilities of the St. Petersburg Mining University and Freiberg Mining Academy; the methodology is described in detail in [38]. All data obtained were processed using Statistica software (STATISTICA 10.0, StatSoft, Tulsa, OK, USA).
Results and discussion
Morphometric parameters of ore minerals in Norilsk-type ores of the Talnakh intrusive complex calculated using optical and microscopic images in the Thixomet Pro Software and analysis of computer X-ray microtomography data are presented in Table 1.
Comparative analysis of morphometric data shows that such parameters as elongation, and edge roughness are most closely determined by different investigation methods while determining the area and perimeter of ore minerals are diagnosed ambiguously. Thus, during interpretation, one of the main limitations of Thixomet Pro data, is the correct data capture, namely the difficulty of automated extraction of individual grains within polyphase ore aggregates. The Software can see aggregates as a single grain and calculate their overall morphometric parameters. A similar problem may arise when processing and interpreting microtomographic data; the tomograph Software most effectively distinguishes ore minerals with high X-ray density (native elements, sulphides and metal oxides) from silicate (olivines, pyroxenes, amphiboles, feldspars, quartz, etc.) or carbonate matrix, but poorly discriminates grains of minerals with similar X-ray density, e.g. sulphides between each other, by entirely isolating aggregates of sulphide grains. Nevertheless, when comparing the results obtained by the different methods, a high convergence and complementarity can be noted.
Ore minerals of disseminated copper-nickel ores form separate rather large nested clusters in biotite-bearing metasomatically altered gabbro-dolerites (Fig.2). All grains in them are elongated in the horizontal plane, have an irregular shape, sinuous grain boundary contours, and large distance between grains and grain aggregates. In fine-grained basalts, ore minerals form phenocrysts with minimum ore grain size and maximum elongation. The grains have irregular, sinuous boundaries and are spaced far apart (Table 1).
Table 1
Comparison of morphometric parameters of ore minerals
Parameter |
Opto-microscopic method Thixomet Pro |
Computed X-ray microtomography |
|||
1 |
2 |
1 |
2 |
||
Samples/Measurements |
21/6305 |
23/11100 |
21/21 |
23/23 |
|
Area, mm2 |
0.18±0.08 |
0.06±0.03 |
0.28±0.23 |
0.10±0.06 |
|
Perimeter, mm |
3.93±0.06 |
0.25±0.10 |
7.05±0.15 |
0.48±0.04 |
|
Shape factor / Sphericity, unit fraction |
0.19±0.07 |
0.18±0.05 |
0.23±0.10 |
0.25±0.05 |
|
Continuity, unit fraction |
0.58±0.11 |
0.59±0.10 |
n.d. |
n.d. |
|
Elongation, unit fraction |
33.2±5.7 |
33.7±8.1 |
28.2 |
32.1 |
|
Edge roughness / Roughness |
1.96±0.25 |
2.1±0.51 |
3.61±0.58 |
2.3±0.08 |
|
Average distance between the centres of the grains, µm |
460.87 |
157.4 |
n.d. |
n.d. |
|
Closed porosity, % |
n.d. |
n.d. |
0.61 |
0.82 |
|
Effective porosity, % |
n.d. |
n.d. |
0.28 |
1.18 |
|
Total porosity, % |
n.d. |
n.d. |
0.89 |
2.0 |
|
Sphericity of pores, % |
n.d. |
n.d. |
0.82 |
0.70 |
Note. Mean values ± confidence interval at 95 % probability; 1 – disseminated copper-nickel ores from the central flanks of the Kharaelakh intrusion; 2 – rich cuprous ores from the western flanks of the Kharaelakh intrusion.
Ore minerals from the rich cuprous ores of the central flanks of the Kharaelakh intrusion form a fine-grained, drop-shaped sulphide phenocryst in the host rocks (Fig.3). The quantitative characteristics of the ore minerals vary to a lesser extent in three planes over the area and perimeter of the grains (Table 1). When comparing with disseminated ores, the granulometric differences of the ore minerals are of particular note: all grains are considerably smaller in size, have more sinuous boundaries, have smaller spacing between grains (aggregates), and irregular shapes.
In assessing the distribution of morphological parameters by rock type, it was found that the maximum size of ore minerals in the central part of the intrusion is characteristic of taxitic gabbro-dolerites, and in the marginal parts of the intrusion – of olivine porphyritic taxitic gabbro. The most productive (with increased PGE content) in the central and marginal parts of the intrusive are taxitic gabbro-dolerites. The average distance between olivine grains in the marginal parts of the intrusion has increased.
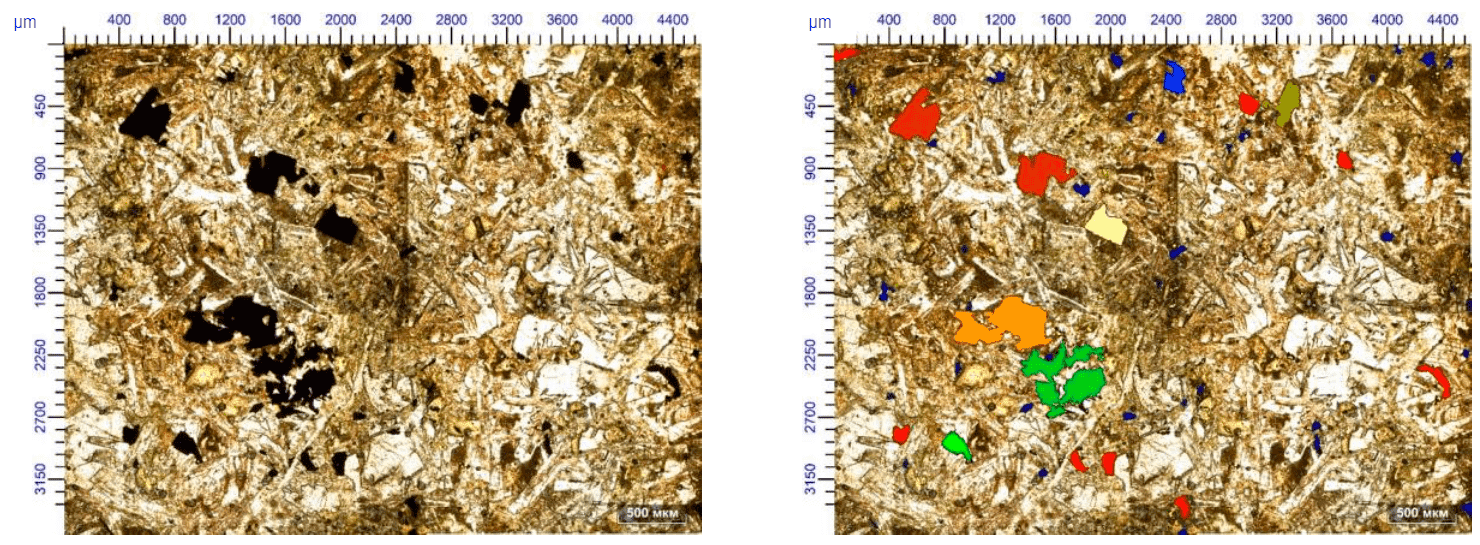
Fig.2. Disseminated copper-nickel ores in gabbro-dolerite. Transmitted light photos of thin sections, without analyzer (left), an example of automatic image capture of ore minerals in Thixomet Pro (right)
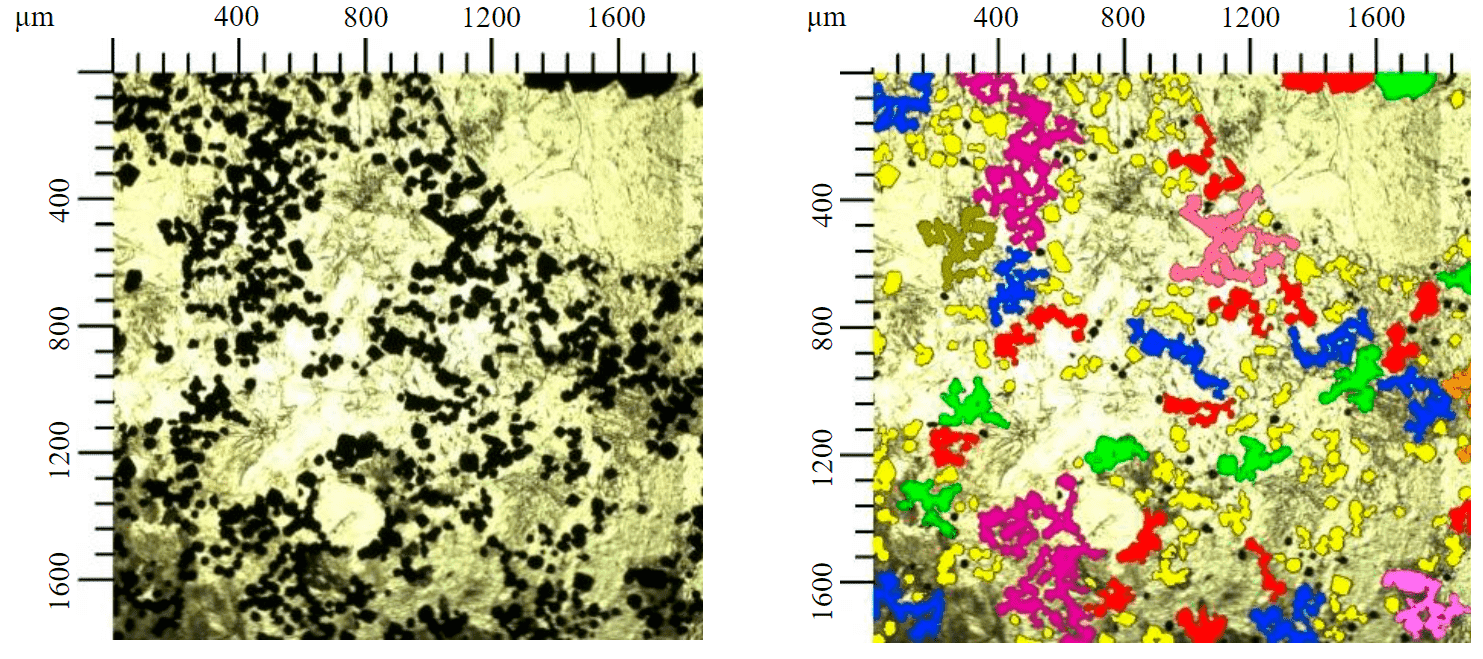
Fig.3. Rich cuprous ores in gabbro-dolerite. Transmitted light photos of the thin sections, without analyser (left), example of automatic image capture of ore minerals in Thixomet Pro (right)
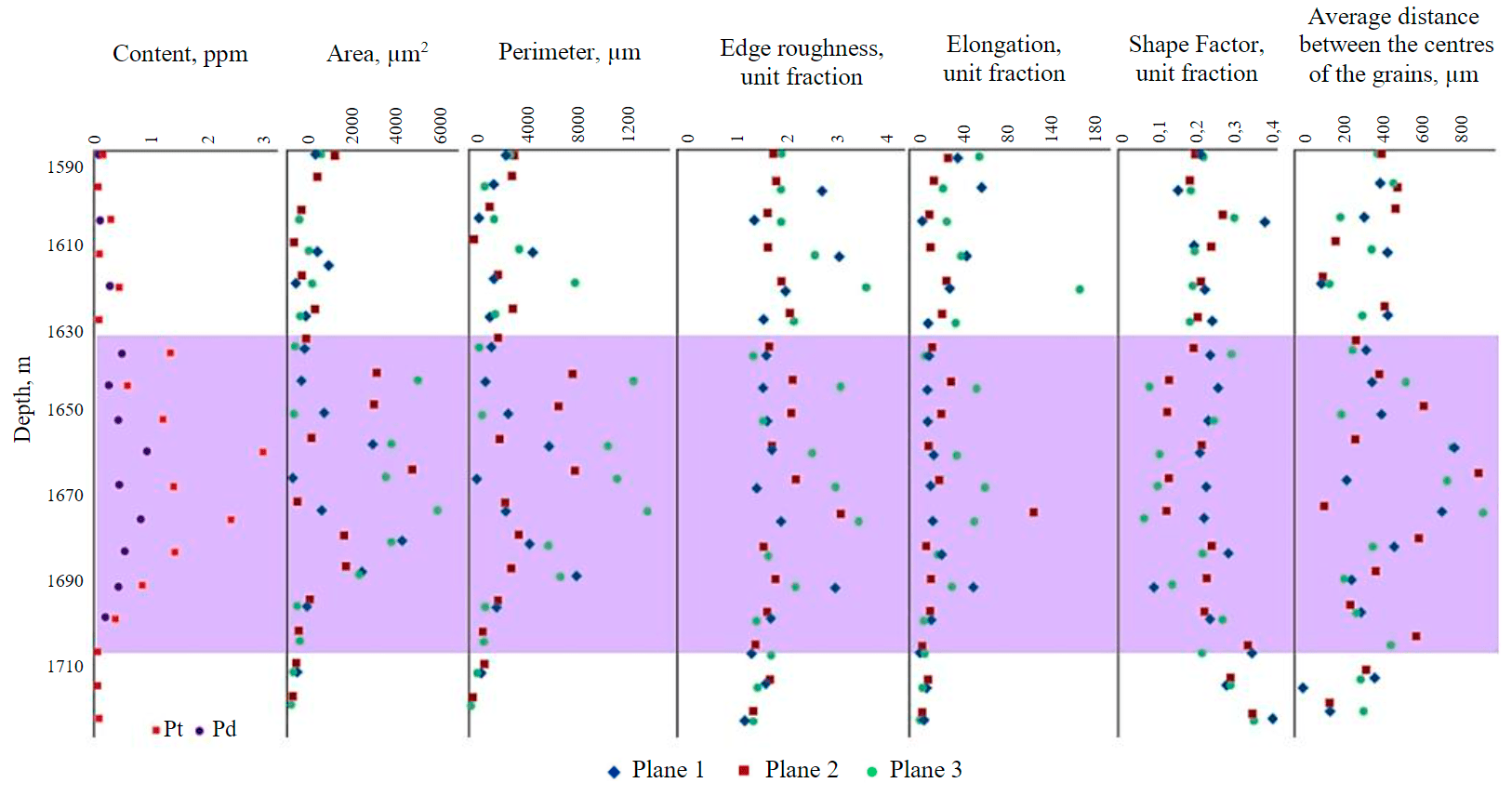
Fig.4. Distribution of ore mineral quantitative parameters in three planes along the borehole depth in the central part of the Kharaelakh intrusion. Disseminated copper-nickel ores. The colour highlights the zone with increased PGE concentrations
In the central part of the Kharayelakh intrusion, disseminated ore minerals have fairly isometric shapes in all three planes throughout the section, and only in one interval sharp scatter of parameters (area, perimeter, edge roughness, elongation) in three planes is observed, as well as the increase of the area and perimeter of grains and mainly in the horizontal plane (thin section/plane 3). The sphericity parameter in this interval, on the contrary, decreases. The rocks in this interval are dominated by taxitic and pycritic gabbro-dolerites with frequent leucogabbro autoliths that are not mineralized [32]. According to the chemical data exactly in this interval the platinum and especially palladium content increases (Fig.4).
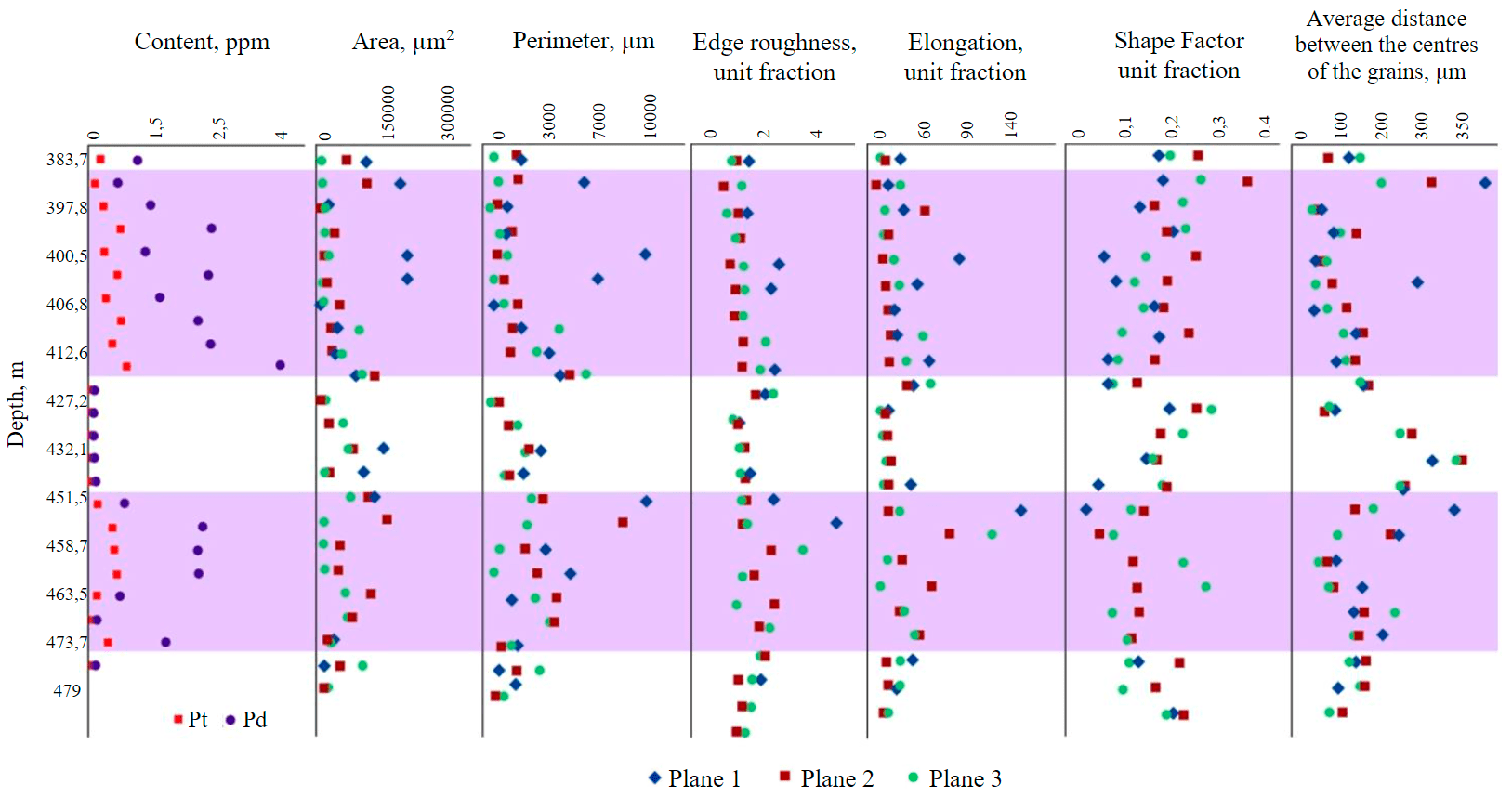
Figure 5. Distribution of ore mineral quantitative parameters in three planes along the borehole depth in the western flanks of the Kharaelakh intrusion. Rich cuprous ores. The colour highlights the zone with increased PGE concentrations
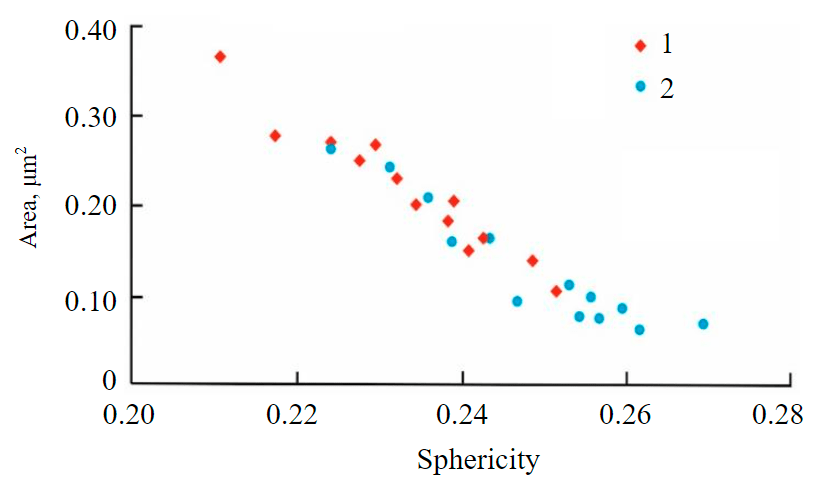
Fig.6. Dependence of ore minerals sphericity on their area according to computed X-ray microtomography 1 – disseminated ores; 2 – rich cuprous ores
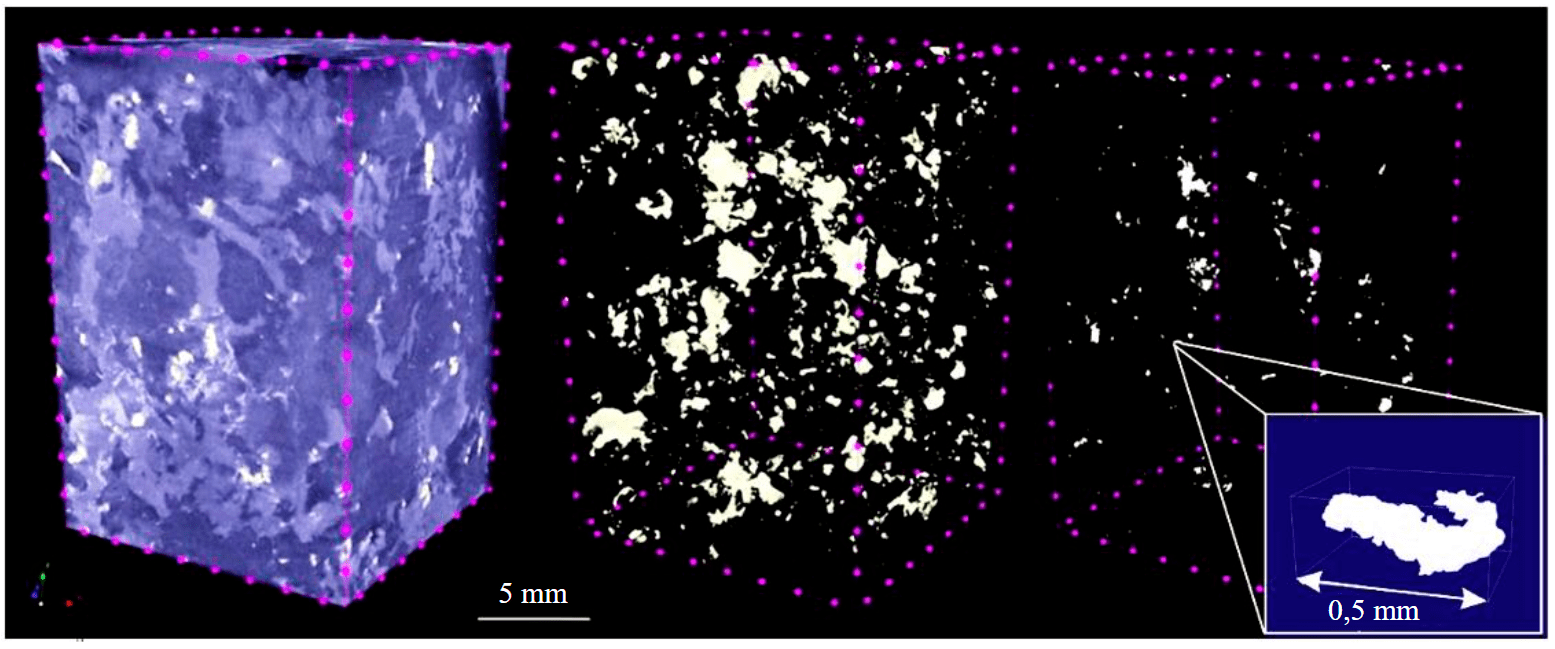
Fig.7. 3D X-ray imaging of disseminated copper-nickel ores (left), sulphide distribution in the rock volume (centre) and platinum group minerals (right)
Ore minerals of rich cuprous ores from the western flanks of the Kharaelakh intrusion are generally characterized by a smaller scatter of morphometric parameters, smaller sizes of ore grains and smaller spacing between them (Fig.5). However, in the ore intervals, a sharp scatter of almost all parameters is evident, except for the average distance between the centres of ore grains with an increased sphericity parameter, which is probably due to the presence of large aggregates of ore-free pyrite.
According to computed X-ray microtomography, the sphericity of ore minerals is inversely related to their area, which fully corresponds to the results of chemical analyses and optical-microscopic study, which show that the largest aggregates and clusters of ore minerals belong to xenomorphic complex branching skeleton crystals of pyrite, not bearing, as a rule, platinum-copper-nickel mineralization (Fig.6). It is also worth noting that large ore grains (or “clumped” aggregates of smaller grains) according to Fig.6 have a more complex morphology in contrast to the smaller, more idiomorphic grains.
The microtomography studies have also produced 3D images of the samples, which allow us to observe not only the size of the grains and their aggregates but also their distribution in the total rock volume and their relationships with each other, which is particularly important for studying the opening of aggregates during crushing and milling in the ore dressing process (Fig.7). The software allows to simulate the total rock volume, to separate silicate matrix from sulphide matrix and sulphide matrix from platinum group minerals. As the pores are easily distinguishable from minerals when separating, the X-ray computed microtomography method also determines the porosity of ores which shows the strength characteristics of rocks and affects the behaviour of rocks during disintegration, because fracture occurs along the weakest zones – fractures, intergrowth boundaries of individual mineral phases, etc. [39, 40]. The analysis of the pore space characteristics of the samples revealed a fairly low porosity, which indicates the high strength of the ores themselves [41, 42].
The results obtained were compared with the laboratory results on the physical and mechanical properties of ores and the data obtained by T.N.Aleksandrova et al. [38] (Tables 2, 3), which show that copper-nickel ores belong to the hard ore type (both in terms of low-energy fracture parameter and the complex parameter of high-energy fracture A·b) but are soft in terms of fracture resistance during impact crushing and are moderately abrasive. It should be noted that rich cuprous ores have higher effective porosity (see Table 1) and at the same time are less compressive and fracture resistant both in dry samples and in water-saturated ones (Table 2), which is connected with peculiarities of internal structure of both ores themselves (size, roughness of borders, distribution in rock space) and structural and textural features of host rocks (distribution of taxite textures, etc.).
Table 2
Physical and mechanical properties of the disseminated and rich ores
Parameter |
1 |
2 |
|
Volumetric weight, g/cm3 |
2.93-3.26 |
2.5-2.96 |
|
Compressive strength, kg/cm2 |
990±89 |
450±58 |
|
Compressive strength at water saturation, kg/cm2 |
750±76 |
215±35 |
|
Tensile strength, kg/cm2 |
70±9 |
26±7 |
|
Strain modulus according to static test diagrams, kg/cm3 |
500 •103 |
430 •103 |
|
Longitudinal wave velocity, m/s |
6200 |
4600 |
|
Poisson's ratio |
0.27 |
0.23 |
|
Anisotropy coefficient |
1.0 |
0.9-1.1 |
|
Dynamic Young's modulus |
|
|
|
For air-dry samples, kg/cm3 |
n.d. |
26±7 |
|
For water-saturated samples, kg/cm3 |
n.d. |
26±7 |
Note. Young's modulus is determined based on measured values of velocity and volume weight;
1, 2 – see notes to Table 1.
Table 3
Physical and mechanical properties of sulphide copper-nickel ores according to [38]
Parameter |
Sulphide copper-nickel ores |
JK DropWeight test results |
|
Parameter A |
99.90±4.95 |
Parameter b |
0.30±0.0015 |
Multiplication Ab |
30.00±0.64 |
Abrasive parameter ta |
0.30±0.06 |
Parameter SCSE*, kWh/t |
11.90±0.21 |
F. Bonds’ indexes determination |
|
Ball mill work index BWI, kWh/t |
11.96±0.54 |
Crushing work index CWI, kWh/t |
21.82±1.09 |
Allis Chalmers’ abrasive tests |
|
Abrasiveness index AI, g |
0.1653±0.008 |
Bulk density (with tamping), t/m3 |
2.01±0.09 |
The data obtained are also consistent with the results of laboratory tests [38, 43]. Thus, during the crushing of the mentioned ores in different types of crushers (jaw, roller and rotary crushers) it was revealed that the main ore components (mainly copper and nickel) are concentrated also in fine sieve classes with a simultaneous increase in silica and calcium content in the coarse sieve classes (table 4). All these studies allow us to justify the possibility of selective disintegration for different types of copper-nickel ores, both in terms of mineralogical and technical characteristics and in terms of experimental studies using different types of crushing plants.
Table 4
Distribution of copper, nickel, silicon and calcium in size classes in different types of crushers [38]
Sieve classes, mm |
Jaw |
Roll |
Impact |
Jaw |
Roll |
Impact |
|
Copper, % |
Nickel, % |
||||
–4+3.2 |
43.42 |
25.28 |
31.64 |
59.47 |
24.91 |
34.49 |
–3.2+1.6 |
13.69 |
24.98 |
14.05 |
11.06 |
27.70 |
13.34 |
–1.6+0.8 |
10.57 |
11.75 |
13.11 |
9.62 |
12.06 |
16.02 |
–0.8+0.4 |
8.77 |
9.11 |
9.63 |
5.13 |
10.01 |
10.35 |
–0.4+0.2 |
6.50 |
8.50 |
11.55 |
4.43 |
7.28 |
9.62 |
–0.2+0.1 |
7.06 |
7.08 |
11.32 |
3.49 |
6.06 |
9.43 |
–0.1+0 |
8.99 |
13.28 |
8.70 |
6.80 |
11.98 |
6.75 |
|
Silica, % |
Calcium, % |
||||
–4+3.2 |
71.86 |
37.53 |
61.54 |
71.06 |
34.94 |
67.70 |
–3.2+1.6 |
11.66 |
33.29 |
13.01 |
12.03 |
35.71 |
10.98 |
–1.6+0.8 |
6.90 |
13.10 |
9.13 |
7.20 |
13.91 |
8.00 |
–0.8+0.4 |
3.35 |
6.09 |
5.36 |
3.33 |
6.30 |
4.34 |
–0.4+0.2 |
2.48 |
4.19 |
4.68 |
2.56 |
4.19 |
3.96 |
–0.2+0.1 |
1.66 |
3.08 |
3.83 |
1.70 |
3.12 |
3.10 |
–0.1+0 |
2.09 |
2.72 |
2.45 |
2.12 |
1.83 |
1.92 |
Conclusions
Quantitative characteristics of morphological parameters of typical samples of disseminated and rich cuprous Norilsk-type ores within the Oktyabrsky deposit of the Norilsk ore district were identified as a result of optical-microscopic and X-ray microtomographic studies. Among the quantitative parameters measured using Thixomet Pro Software, the most informative for disseminated ores were grain area, perimeter, edge roughness, shape factor, elongation and the average distance between grains, and for rich cuprous ores – grain area, perimeter, edge roughness and grain elongation.
As a result of this study, the Norilsk-type intrusions are characterized by increasing values and dispersion in ore zones of parameters such as grain area and perimeter, as well as the average distance between grains. This pattern can be seen for both disseminated and rich cuprous ores. Thus, ore intervals can be predicted from the combined maximums and strong variability of morphological parameters in the three planes. However, when identifying ore zones, special attention should be paid to the occurrence of leucogabbro in the intervals, as these rocks have large sulphide segregations (main pyrite), are often non-bearing and may violate the identified patterns.
It is noted that the area of ore minerals increases at least 2-3 times in the ore zones, which is especially important for the marginal flanks of the Kharaelakh intrusion, where ore minerals are an order of magnitude smaller than similar differentiates in the central part and are difficult to diagnose by optical methods.
Quantitative analysis of ore minerals in the Thixomet PRO Software and using a computed X-ray microtomography is quite fast, so they can easily be used as preliminary express methods of substance study. Also, the method of computed X-ray microtomography is used for 3D visualization of the internal structureof sulphide mineralization samples, which allows observing not only quantitative parameters of grains and their aggregates but also their distribution in the total volume of rocks and their interrelation with each other. The undoubted advantage of computed tomography is the possibility of obtaining not only the quantitative characteristics of mineral phases but also the pore space of the rock, which affects the strength characteristics of rocks and ores.
In conclusion, it should be noted that the possibility to obtain statistical dispersion parameters of different morphometric characteristics, and their spatial distribution is an undoubted advantage of both used methods, since based on a large amount of the obtained data using mathematical-statistical modelling (correlation, factor analysis, etc.) it is possible to forecast dependences between the morphometric parameters of ores and properties of dressing products or system characteristics, such as wear and tear or energy consumption, which will allow to closely approach the issues of selective disintegration of Norilsk ores.
References
- Litvinenko V.S. Digital Economy as a Factor in the Technological Development of the Mineral Sector. Natural Resources Research. 2020. Vol. 29, p. 1521-1541. DOI: 10.1007/s11053-019-09568-4
- Matveeva V.A., Alekseenko A.V., Karthe D., Puzanov A.V. Manganese Pollution in Mining-Influenced Rivers and Lakes: Current State and Forecast under Climate Change in the Russian Arctic. Water. 2022. Vol. 14. Iss. 7. N 1091. DOI: 10.3390/w14071091
- Pronin E.M., Vasilev V.E., Tsvetkov V.Y. The factors defining the stability and sustainable development of mineral and raw materials enterprises and the factors’ influence оn the activity results evaluation. Journal of Mining Institute. 2011. Vol. 191, p. 176-182 (in Russian).
- Makhovikov A.B., Katuntsov E.V., Kosarev O.V., Tsvetkov P.S. Digital transformation in oil and gas extraction: Challenges and prospects. In Innovation-based development of the mineral resources sector. London: CRC Press, 2019. 614 p.
- Alekseenko V.A., Shvydkaya N.V., Alekseenko A.V. et al. Element Accumulation Patterns of Native Plant Species under the Natural Geochemical Stress. Plants. 2021. Vol. 10. Iss. 1. N 33. DOI: 10.3390/plants10010033
- Vorontsova N.I., Talovina I.V., Lazarenkov V.G. et al. Isotope ratio 87Sr/86Sr in rocks and ores of the Sakhara and Ufalei supergene nickel deposits in Urals. Journal of Mining Institute. 2013. Vol. 200, p. 179-185 (in Russian).
- Ilalova R.K., Gulbin Y.L. Thermometry of Nickel Bearing Chlorites from the Kolskii Massif (Northern Urals). Geology Ore Deposits. 2019. Vol. 61, p. 736-746. DOI: 10.1134/S107570151908004X
- Hesse M., Popov O., Lieberwirth H. Increasing efficiency by selective comminution. Minerals Engineering. 2017. Vol. 103-104, p. 112-126. DOI:10.1016/j.mineng.2016.09.003
- Arsentyev V.A., Gerasimov A.M., Dmitriev S.V., Samukov А.D. A study of mineral coal physical and mechanical properties changes in thermal modification process. Obogashchenie rud. 2016. N 3, p. 3-8 (in Russian). DOI: 10.17580/or.2016.03.01
- Vaisberg L.A., Kameneva E.E. Changes in rock structure with cyclic freezing and thawing. Obogashchenie rud. 2015. N 2, p. 28-31 (in Russian). DOI: 10.17580/or.2015.02.06
- Melnikova Т.N., Yatlukova N.G., Litvinova N.M. Upon the Issue of Ores Grinding Process Optimization. Obogashchenie rud. 2006. N 4, p. 5-7 (in Russian).
- Benndorf J., Buxton M. A Special Issue on Geomathematics for Real-Time Mining. Mathematical Geoscience. 2019. Vol. 51, p. 845-847. DOI: 10.1007/s11004-019-09828-2
- Popov O., Lieberwirth H., Folgner T. Quantitative characterisation of the solid rocks to predict the influence of the rock on relevant product properties and system parameters. Part 1: Application of quantitative microstructural analysis. AT Mineral Processing. 2014. Vol. 55, p. 76-88 (in German).
- Popov O., Lieberwirth H., Folgner T. Quantitative characterisation of the solid rocks to predict the influence of the rock on relevant product properties and system parameters. Part 2: Selected examples. AT Mineral Processing. 2014. Vol. 55. 54-63 (in German).
- Izoitko V.M. Technological mineralogy and ore evaluation. St. Petersburg: Nauka, 1997, p. 532 (in Russian).
- Raaz V. Charakterisierung der Gesteinsfestigkeit mit Hilfe eines modifizierten Punktlastversuches. Zeitschrift für Geologische Wissenschaften. 2002. Vol. 30. N 3, p. 213-226.
- Melnik A.E., Skublov S.G., Rubatto D. et al. Garnet and zircon geochronology of the Paleoproterozoic Kuru-Vaara eclogites, northern Belomorian Province, Fennoscandian Shield. Precambrian Research. 2021. Vol. 353. N 106014. DOI: 10.1016/j.precamres.2020.106014
- Klichowicz M., Frühwirt T., Lieberwirth H. New experimental setup for the validation of DEM simulation of brittle crack propagation at grain size level. Minerals Engineering. 2018. Vol. 128, p. 312-323. DOI: 10.1016/j.mineng.2018.08.033
- Aleksandrova T.N., Nikolaeva N.V., Lvov V.V., Romashev A.O. Ore processing efficiency im-provements for precious metals based on process simulations. Obogashchenie rud. 2019. N 2, p. 8-13 (in Russian). DOI: 10.17580/or.2019.02.02
- Vaisberg L.A., Kameneva Ye.Ye., Pimenov Yu.G., Sokolov D.I. Gneissogranite pore space structure study by the X-ray tomography method. Obogashchenie rud. 2019. N 3, p. 37-40 (in Russian).
- Popov O., Talovina I., Lieberwirth H., Duryagina A. Quantitative microstructural analysis and x-ray computed tomography of ores and rocks – Comparison of results. Minerals. 2020. Vol. 10. Iss. 2. N 129. DOI: 10.3390/min10020129
- Duryagina A., Heide G., Talovina I., Bravo A. Mineralogical and morphometric aspects of the rock analysis as the basis for choosing a scheme for ore preparation. VIII International Scientific Conference “Problems of Complex Development of Georesources”, 8-10 September 2020, Khabarovsk, Russian Federation. E3S Web of Conferences, 2020. Vol. 192. N 02023. DOI: 10.1051/e3sconf/202019202023
- Duryagina A.M., Talovina I., Shtyrlyaeva A., Popov O. Application of Computer X-Ray Microtomography for Study of Technological Properties of Rocks. Key Engineering Materials. 2018. Vol. 769, p. 220-226. DOI: 10.4028/www.scientific.net/KEM.769.220
- Shtyrlyaeva A.A., Zhuravlev A.V., Gerasimova A.I. Prospects and Problems of Computer Microtomography using for Core Samples Studies. Petroleum Geology – Theoretical and Applied Studies. 2016. Vol. 11, N 1, р. 12 (in Russian). DOI: 10.17353/2070-5379/8_2016
- Genkin A.D., Distler V.V., Gladyshev G.D. Sulphide copper-nickel ores of Norilsk deposits. Moscow: Nauka, 1981, p. 234 (in Russian).
- Distler V.V. Platinum mineralisation of Norilsk deposits. In Geology and genesis of platinum metal deposits. Moscow: Nauka, 1994, p. 7-35 (in Russian).
- Eckstrand O.R., Hulbert L.J. Magmatic Nickel-copper-platinum Group Elements Deposits // Mineral deposits of canada: a synthesis of major deposit types, district metallogeny, the evolution of geological provinces, and exploration methods: geological association of Canada, mineral deposits division, Special Publication. Ottawa, Ontario: Geological Survey of Canada, 2007. Vol. 5, p. 205-222.
- Naldrett A.J.Magmatic Sulfide Deposits: Geology, Geochemistry and Exploration. Berlin/Heidelberg: Springer Science & Business Media, 2013, p. 728. DOI: 10.1007/978-3-662-08444-1
- Sittner J., Brovchenko V., Siddique A. et al. Three-Dimensional Distribution of Platinum Group Minerals in Natural MSS-ISS Ores From the Norilsk One Deposit, Russia. Frontiers in Earth Science. 2022. Vol. 10. N 860751. DOI: 10.3389/feart.2022.860751
- Tolstykh N., Krivolutskaya N., Safonova I. et al. Unique Cu-rich sulphide ores of the Southern-2 orebody in the Talnakh Intrusion, Noril’sk area (Russia): Geochemistry, mineralogy and conditions of crystallization. Ore Geology Reviews. 2020. Vol. 122. N 103525. DOI: 10.1016/j.oregeorev.2020.103525
- Chayka I., Zhitova L., Antsiferova T. et al. In-Situ Crystallization and Continuous Modification of Chromian Spinel in the “Sulfide-Poor Platinum-Group Metal Ores” of the Norilsk-1 Intrusion (Northern Siberia, Russia). Minerals. 2020. Vol. 10. N 498. DOI: 10.3390/min10060498
- Radko V.A. Facies of intrusive and effusive magmatism of the Norilsk region. St. Petersburg: Kartograficheskaya fabrika VSEGEI, 2016, p. 226 (in Russian).
- Barnes S.J., Le Vaillant M., Godel B., Lesher C.M. Droplets and Bubbles: Solidification of Sulphide-rich Vapour-saturated Orthocumulates in the Norilsk-Talnakh Ni–Cu–PGE Ore-bearing Intrusions. Journal of Petrology. 2019. Vol. 60. Iss. 2, p. 269-300. DOI: 10.1093/petrology/egy114
- Barnes S.J., Mungall J.E., Le Vaillant M. et al. Sulfide-silicate textures in magmatic Ni-Cu-PGE sulfide ore deposits: Disseminated and net-textured ores. American Mineralogist. 2017. Vol. 102. N 3, p. 473-506. DOI: 10.2138/am-2017-5754
- Kazakov A.A., Kiselev D. Industrial Application of Thixomet Image Analyzer for Quantitative Description of Steel and Alloys. Metallography, Microstructure, and Analisis. 2016. Vol. 5. Iss. 4, p. 294-301. DOI: 10.1007/s13632-016-0289-6
- Evans C.L. Quantifying mineral grain size distributions for process modelling using X-ray micro-tomography. Minerals Engineering. 2015. Vol. 82, p. 78-83. DOI: 10.1016/j.mineng.2015.03.026
- Willson C.W., Lu N., Likos W.J. Quantification of grain, pore and fluid microstructure of unsaturated sand from X-Ray CT images. Geotechnical Testing Journal. 2012. Vol. 35. N 6. N PA 19428-2959. DOI: 10.1520/GTJ20120075
- Aleksandrova T., Nikolaeva N., Afanasova A. et al. Selective disintegration justification based on the mineralogical and technological features of the polymetallic ores. Minerals. 2021. Vol. 11. Iss. 8. N 851. DOI: 10.3390/min11080851
- Alexandrova T.N., O’Connor C. Processing of platinum group metals ores in Russia and South Africa: Current state and prospects. Journal of Mining Institute. 2020. Vol. 244, p. 462-473. DOI: 10.31897/PMI.2020.4.9
- Gupta A., Yan D.S. Mineral Processing Design and Operations: An Introduction. Amsterdam: Elsevier, 2016, p. 882. DOI: 10.1016/C2014-0-01236-1
- Zolotukhina L.V., Nosova O.V., Narbekova T.N. Behavior of noble metals when enriching Noril’sk ore. Scientific Bulletin of the Norilsk Industrial Institute. 2014. Vol. 14, p. 42-52 (in Russian).
- Litvinova N.M., Melnikova T.N., Yatlukova N.G., Danilov E.I. Intensification of milling process of resistant gold ores of Albazino deposit. Gornyi zhurnal. 2006. N 11, p. 63-64 (in Russian).
- 43.Aleksandrova T.N., Kuznetsov V.V., Ivanov E.A. Investigation of the water hardness ions impact on the copper-nickel ores flotation probability. Mining Informational and Analytical Bulletin. 2022. Vol. 6-1, p. 263-278 (in Russian). DOI: 10.25018/0236_1493_2022_61_0_263