Defects of diamond crystal structure as an indicator of crystallogenesis
- Ph.D. Leading Engineer Saint Petersburg Mining University ▪ Orcid ▪ Elibrary ▪ Scopus
Abstract
Based on the study of a representative collections of diamonds from diamondiferous formations of the Urals and deposits of the Arkhangelsk and Yakutian diamond provinces, we established patterns of zonal and sectoral distribution of crystal structure defects in crystals of different morphological types, identified the specifics of crystals formed at different stages of crystallogenesis and performed a comprehensive analysis of constitutional and population diversity of diamonds in different formations. We identified three stages in the crystallogenesis cycle, which correspond to normal and tangential mechanisms of growth and the stage of changing crystal habit shape. At the stage of changing crystal habit shape, insufficient carbon supersaturation obstructs normal growth mechanism, and the facets develop from existing surfaces. Due to the absent stage of growth layer nucleation, formation of new {111} surfaces occurs much faster compared to tangential growth mechanism. This effect allows to explain the absence of cuboids with highly transformed nitrogen defects at the A-B 1 stage: they have all been refaceted by a regenerative mechanism. Based on the revealed patterns, a model of diamond crystallogenesis was developed, which takes into account the regularities of growth evolution, thermal history and morphological diversity of the crystals. The model implies the possibility of a multiply repetitive crystallization cycle and the existence of an intermediate chamber; it allows to explain the sequence of changes in morphology and defect-impurity composition of crystals, as well as a combination of constitutional and population diversity of diamonds from different geological formations.
Introduction
Diamond is unique in its variety of stable crystal structure defects (CSDs) [18, 6]. Irregularities in the volume distribution of impurities and CSDs allow to visualize internal crystal structure and serve as a basis for ontogenetic analysis [4, 21, 22], modeling of crystallogenesis [26-28] and mineral deposit genesis [23, 35, 39]. The fundamentals of diamond ontogeny were developed by V.V.Beskrovanov [2], who proposed an evolution pattern (crystallization cycle) of diamond crystal shape associated with a decrease in carbon supersaturation of the medium; he also identified three stages of growth, a destructive stage of dissolution and a regressive stage of coated crystal formation. At the first stage, crystals grow by a normal mechanism, at the second stage – by a tangential one, with contrast zoning; at the third stage, the growth is still driven by a tangential mechanism, and flat-faced octahedra are formed. The material for this study was comprised of octahedral crystals from the deposits of the Yakutian diamond province (YDP). At the deposits of the Arkhangelsk diamond province (ADP) and the Urals, crystals have wider morphological and constitutional diversity; further development of ontogenetic analysis is required to explain it. Over the past 20 years, a large amount of factual material has been accumulated [1, 3, 8], significant progress has been achieved in the study of structural specifics [5, 10] and regional typomorphism of diamonds [10, 12, 40], evolution of crystal morphology during growth and dissolution [25, 34], composition of diamond-forming medium [7, 17, 38], nature of various CSDs [5, 13, 36] and patterns of their transformation [19]. However, many aspects of anatomy, morphological and constitutional diversity of diamond crystals [16, 23, 24] remain unexplained in the scope of existing models of diamond formation and formation of kimberlite bodies [16, 31]. Hence, it is a relevant task to identify general patterns of CSD distribution in the volume of diamond crystals and determine the mechanisms of CSD transformation in natural processes. Consideration of these patterns is a crucial step in performing genetic interpretation of diamond research results. The results obtained over the past 20 years indicate that the classical concepts of natural diamond growth need to be revised.
Problem statement
To use the defects of diamond crystal structure as an indicator of crystallogenesis and to develop a CSD-based model of diamond crystallogenesis that will take into account patterns of growth evolution, thermal history and morphological diversity, it is necessary to address the following tasks: analyze the results of studying representative collections of diamonds from diamondiferous formations of the Urals, ADP and YDP deposits; establish patterns of zonal and sectoral CSD distribution in crystals of different morphological types; identify the specifics of crystals formed at different stages of crystallogenesis; perform comprehensive analysis of constitutional and population diversity of diamonds from different formations.
Methodology
Basic methods of this research included infrared (IR) absorption spectroscopy (IRS) and photoluminescence (PL) spectroscopy, optical and cathodoluminescence (CL) microscopy. IR absorption spectra were recorded on a Fourier spectrometer VERTEX-70 (Bruker) with a Hyperion2000 microscope. PL spectra were recorded on Horiba FL-3, InVia spectrometers (Renishaw). CL and SEI images were obtained with a scanning electron microscope CamScan MX2500 S at the Russian Geological Research Institute (VSEGEI).
The study involved analysis of the data, both obtained by the author and published in open sources, on more than 15,000 diamond crystals from the main primary and alluvial deposits of the YDP, ADP and various diamondiferous formations of the Urals. We studied diamonds from geological collections and current mining products of such companies as ALROSA, ALROSA-NURBA, Severalmaz, Almazy Anabara from deposits Udachnaya, Akhal, Internatsionalnaya, Mir, Dachnaya, Botuobinskaya, Nurbinskaya, Komsomolskaya, Sytykanskaya, Krasnopresnenskaya, Zapolyarnaya, Lomonosov – pipes Karpinsky 1 and 2, Arkhangelskaya, Snegurochka (collection of the Geological Faculty of Moscow State University); crystals from alluvial placers of the Krasnovishersky (AP), Aleksandrovsky and Gornozavodsky districts of the Rassolninskaya depression (RD) deposit (collections of VSEGEI, Mining University Museum, OOO “Permgeologodobycha”); crystals from the Ichetyu placer and various diamondiferous formations in Brazil (collection of the Institute of Geology, Komi Science Center of UB RAS); plates cut from the crystals of deposits Mir, Udachnaya, Aikhal, Botuobinskaya, Lomonosov, placers of the Anabar-Oleneksky interfluve (AOI), Timan, Urals.
Discussion
There is a long-term controversy regarding the nature of Ural-type dodecahedroids – whether they form during crystal growth or are a product of dissolution. The paradox of this discussion is that the growth surfaces of diamond crystals are often curvilinear [30], but the shape of formed individuals is not defined by them. Observed curved surfaces are a result of dissolution [25]. An ideal shape of a natural diamond crystal is a polyhedron bounded by {111} planes. However, actual crystals grow not only with 111 facets, but also with subparallel {100} surfaces, which manifest themselves in CL-zoning (Fig.1). Crystals of cubic habit do not have any flat facets; therefore, it is more correct to call them cuboids [17, 30]. Diamond crystals are formed by two growth mechanisms – the normal mechanism, which shapes cuboid surfaces, and the tangential one, which shapes octahedral facets. In a diamond, growth pyramids <100> and <111> are clearly separated and identified by their shape of zoning. In crystals composed of pyramids <100>, zonal irregularities have a wavy shape (Fig.1, a, c), in the limit they are concentric (Fig.1, b). In the volume of octahedral crystals, zonal irregularities have a shape of flat parallel layers. Zonal irregularities are most often accompanied by variations of nitrogen concentration Ntot, which define the difference between the zones in terms of concentration of nitrogen-vacancy centers of optical activity (COAs).
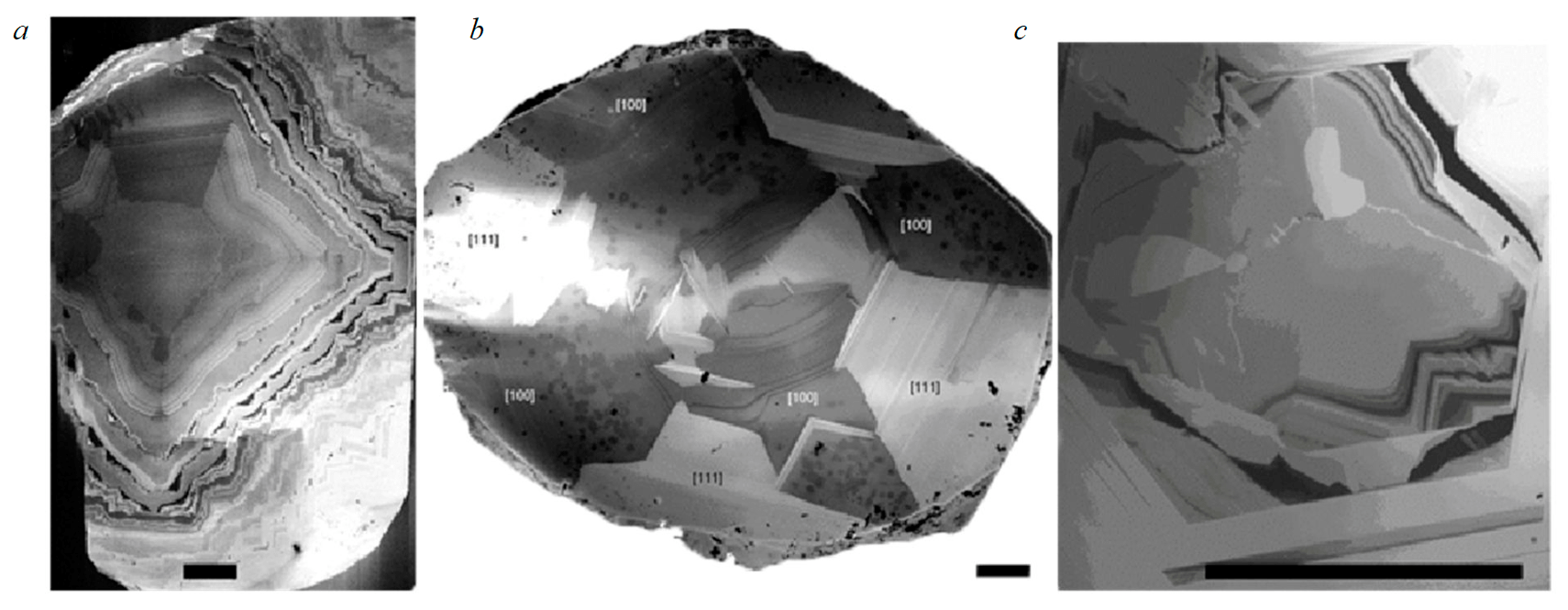
Fig.1. Manifestations of zonal and sectoral irregularities in the crystals with pyramids <100> from alluvial placers of the Krasnovishersky district (a, b) and the Ichetyu placer (c) in cathodoluminiscence. Plate orientation is close to {100}; scale size equals 0.3 mm
The stage of changing growth habit shape – refaceting from cuboid to octahedron – is critical for understanding the morphology of crystals and explaining their spectroscopic characteristics [17, 31]. The anatomy of crystals with refaceting zones is addressed in many studies [12, 17, 30]. At this stage, crystal morphology is usually described as “anti-skeletal octahedron” [29]; given such interpretation, relative area of the facet is defined by a ratio of normal and tangential components of growth velocity. Detailed study of such crystals demonstrates that their morphology is established by a regenerative growth mechanism. The key characteristic of the regenerative mechanism is the absence of layer nucleation stage: the facets grow from existing surfaces [15]. In terms of supersaturation range, regenerative growth occupies an intermediate position between normal and tangential ones. Regenerative growth begins with the formation of a flat-faced polyhedron and stops after the formation of a convex one. At the beginning of regenerative refaceting, cuboids become covered with {111} steps that form square pits. On cuboids and strongly dissolved crystals, these sculptures are usually interpreted as etching patterns [20]. On dissolved crystals, CL visualizes the areas of regenerative growth as “redoubt-shaped” irregularities. The effect of refaceting dramatically reduces the chance of identifying crystal growth forms by means of goniometry. Only examination of constitutional specifics of diamond crystals allows to draw a conclusion about which surfaces are individual facets and which ones are their micro-stepped combinations. In order to explain growth irregularities, morphology and spectroscopic specifics, it is necessary to consider an independent stage of crystallization cycle with a regenerative growth mechanism.
The first stage of diamond crystal growth is associated with high supersaturation, the fastest growth by the normal mechanism (normal velocity component V100┴) and formation of surfaces subparallel to {100} (Fig.2, a, b). At this stage, the dynamic form of growth is a skeletal cuboid. The earliest dynamic form of growth never corresponds to the surface of actual crystals. The curvature of growth surface depends on a number of factors (temperature, pressure, medium composition) and determines crystal habit in a range from a near-hexahedral shape to a spherocrystal. In the presence of strong defectiveness of the crystal structure and incorporation of multiple inclusions, crystal splitting may occur and form ballas-like diamonds. The first stage of growth cycle is the shortest, and diamond crystals can nucleate only during this stage [2]. Detailed examination reveals central, highly stressed areas in all of the crystals, but during mineralogical studies they can be visually indiscernible.
The next stage is characterized by the beginning of a regenerative growth – tangential build-up of facets from existing surfaces with a velocity Vr111║ (Fig.2, d, e). The velocity of such growth is lower than normal one (Vr111║ << V100┴), although with decreasing supersaturation the velocities may become close (Vr111║ = V100┴), which leads to formation of mixed habit crystals (Fig.3, c). Therefore, combined growth of pyramids <100> and <111> should be regarded not as a simultaneous occurrence of normal and tangential mechanisms, but rather an occurrence of normal and regenerative ones. As tangential regenerative growth continues, the crystal is refaceted to octahedron (Fig.2, f).
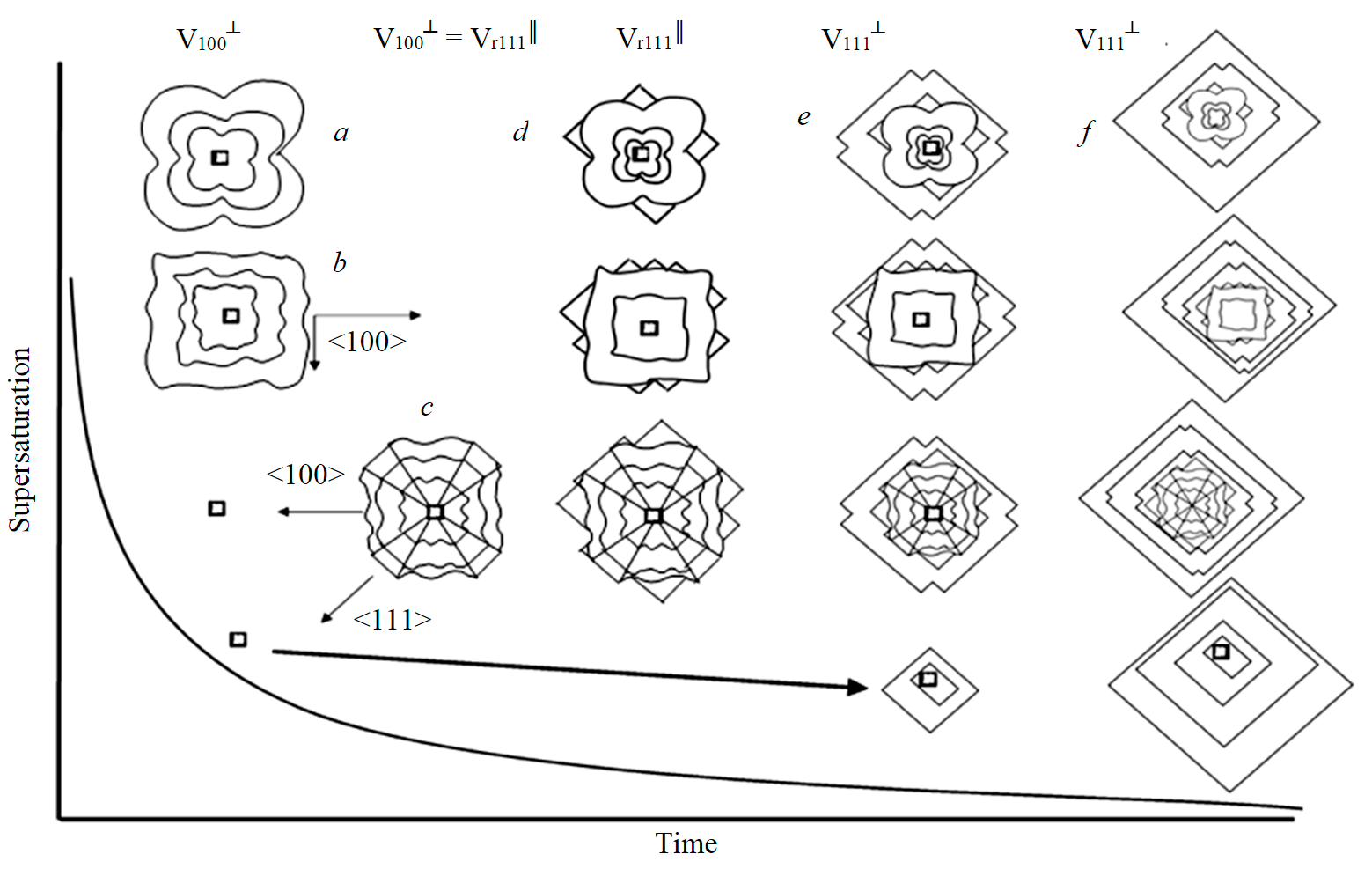
Fig.2. Changes in zoning of a diamond crystal in the crystallization cycle depending on the dominant growth mechanism (components of growth velocity that define the crystal shape): a – normal mechanism, concentric zoning; b – normal mechanism, wavy zoning; c – combined growth by normal and regenerative mechanisms; d – regenerative refaceting; e, f – tangential growth
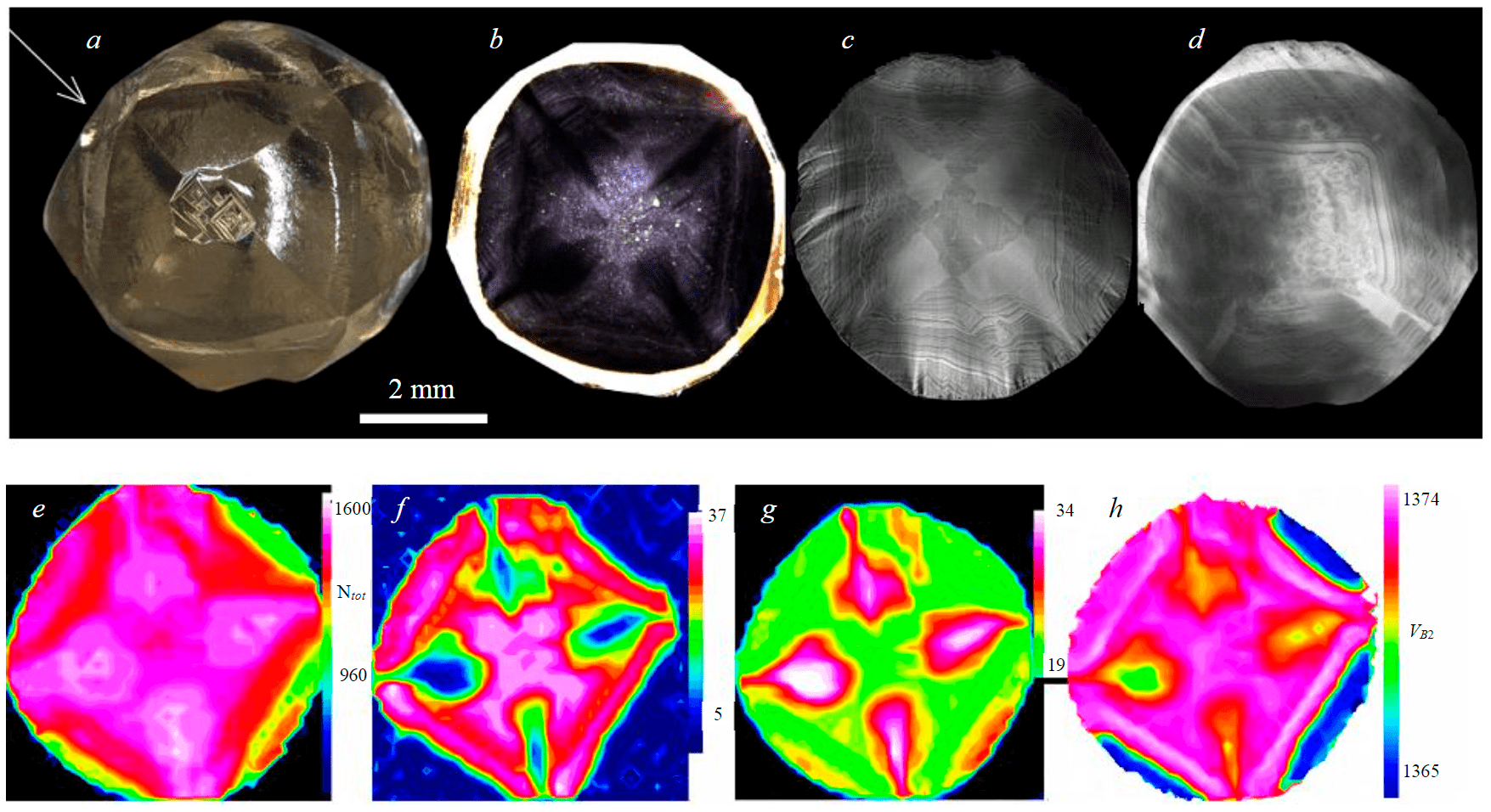
Fig.3. Crystal 123-76 of a mixed habit shape: a – general view, the arrow indicates a remainder of octahedron facet; b – dark-field image of a section plate {100} with a thickness of 0.71 mm, light areas are spotted with micro-inclusions; c-d – CL image of a plate from both sides; e-h – maximum and minimum values of parameters: e – maps of Ntot distribution; f – а3107; g – аВ2; h – vВ2
Subsequent decrease in supersaturation leads to termination of normal growth (V100┴ = 0) and beginning of regenerative refaceting due to tangential growth of 111 facets. The rate-limiting step of tangential growth mechanism is 2D nucleation [15]. Based on the analysis of internal crystal structure and general models of crystallization kinetics, it can be claimed that under the conditions that correspond to the occurrence of each mechanism, velocity components V100┴>>Vr111║>>V111┴ differ by orders of magnitude. The normal growth mechanism leads to incorporation of submicron inclusions by the growing surface, whereas synchronous zones of tangential growth contain no such inclusions. Therefore, the presence of submicron inclusions is not only a rationale for studying crystallization medium [17, 38], but also an indicator of growth mechanism.
During tangential growth in the same medium, crystals incorporate large monomineral inclusions, but remain free of submicron inclusions. A well-established pattern of nitrogen CSDs implies the following sequence: single substituent atom (C) → a couple of substituent atoms (А)→ four substituent atoms and a vacancy (В1) [19]. The duration of stages is reflected in the degree of А → В transformation in the corresponding areas. In the areas of crystals developed at the stages of normal and regenerative growth, no zoning by the degree of А → В transformation is observed, they have the same thermal history. At the first and second stages, the growth velocity is high, but the duration of stages is not enough for А → В1 transformation. At the long-lasting stage of slow tangential growth, there is sufficient time for А → В1 transformation, and we observe zoning by the share of nitrogen in the form of B1-defects (NBS). Hence, even in the same crystal, the degree of nitrogen aggregation can be constant in the areas of normal growth mechanism and decrease to the periphery in the areas of tangential growth.
A key distinction of natural diamond crystals from synthetic ones [34] and crystals of many other minerals [4] is the fact that their tangential growth occurs only with {111} facets. Cuboid surfaces in a natural diamond grow by a normal mechanism, the velocity of which is much higher than the tangential one [15]. Depending on the duration of growth stages by normal and tangential mechanisms, the stages of dissolution and subsequent regeneration, morphology and crystal habit vary in a wide range.
Growth irregularities in crystals can be associated either with defects and impurities (zonal and sectoral), or structural incoherence (orientational). In most cases, studied crystals with orientational irregularities have high values of nitrogen concentration (Ntot) and nitrogen share in the form of B1-defects (NBS), absorption coefficient of N3VH (а3107) and B2 band (аВ2). These data confirm a correlation between high defectiveness of the structure and growth splitting of the crystals [37]. Mixed habit crystals are a typical example of sectoral irregularities (Fig.3, а). In the central part of such individuals, there are <100> and <111> sectors (Fig.3, b-d).
Sectors <111> are characterized by narrow linear zoning, sectors <100> – by wavy zones. No zoning by Ntot, NBS and а3107 is observed in the sectors. Submicron inclusions are localized in sectors <100>. The periphery is dominated by the zoning of 111 steps. A sample 123-76 (Fig.3) allows to demonstrate several patterns, common for mixed habit crystals: inverse relationship between the distribution of а3107 and аВ2 (Fig.3, f, g), disproportionation of а3107 and Ntot (Fig.3, e), a change of growth mechanism at the final stage of crystal formation (Fig.3, c, d), localization of inclusions in pyramids <100>, homogeneity of sectors in terms of Ntot and VВ2 (Fig.3, h).
Areas with 111 orientation developed at the last stage of crystal growth and correspond to quick regenerative refaceting with the formation of transition shapes: cuboid-rhombic dodecahedron-octahedron. In some crystals, zonal irregularities are associated with repeated alternation of areas with normal and regenerative growth. Crystals of octahedral habit are characterized by linear {111} zoning in terms of all IRS parameters, irregular behavior of Ntot, decreasing NBS to the periphery of the crystals [1].
The last but rarely occurring stage of crystal growth is the formation of an outer zone with C-defects – a coating. The coating is usually colored yellow due to absorption of C-defects, but sometimes it is colorless, distinguished by a characteristic topography. For this area, typical features include carbonate inclusions, normal growth mechanism or combined growth of sections by normal and regenerative mechanisms. In the latter case, a normal mechanism leads to emergence of diverse positive topographic forms, whereas negative forms grow by the regenerative mechanism. The coating may form in crystals of all morphological types and different thermal history. It is observed to contain only A- and C-defects and their ionized and vacancy analogs H3, NV (575 nm), NV–(638 nm), H2. Sometimes on the boundary between the central area and the coating, there is a narrow band with an elevated value of а3107.
In the course of post-growth processes, crystals are subjected to natural etching, plastic deformation and irradiation. Under laboratory conditions, plastic deformation of crystals leads to formation of twin subindividuals [36]. Their presence in diamond crystals with a characteristic topography (deformation pattern) is confirmed by X-ray diffraction studies [41]. Separate layers that correspond to deformation pattern transverse the entire crystal, they are clearly distinguishable in CL and PL [14]. In the studied crystals, as well as in the ones described in literature, the planes of plastic deformation transverse the entire crystal, but they are never confined to internal areas. Deformation pattern is observed only on dissolved diamond crystals, while flat octahedral facets contain lines, decorated with negative inversely oriented trigonal sculptures. Certain dissolution of octahedral edges leads to formation of steps on curved surfaces, which are a continuation of lines decorated with trigons.
Natural post-growth etching under different temperature and duration makes primary growth irregularities more complicated. The simplest thermal history corresponds to the last growth stage – formation of a coating. The coating contains A- and C-defects, as well as their vacancy analogs in charged and neutral states [42]. Primary nitrogen-hydrogen CSDs are associated with the bands of 1353, 1374 cm–1. The majority of cuboids have a more complex growth and post-growth history because of the emergence of N3, N3VH centers. Subsequent etching transformation of these crystals leads to the occurrence of B1-defect, its vacancy analog H4, В2 and preceding polyatomic complexes, hydrogen-containing CSD with the line of 926 nm. This occurs in parallel with the development of diverse Ni-containing CSDs, active primarily in PL. Ni-containing centers can change transformation kinetics of nitrogen- and Ni-containing CSDs due to their additional contrast with the irregularity. As a share of B1-centers increases as a result of natural etching, it leads to the formation of specific COAs, identified in PL as lines of 537 and 575 nm, 660 and 700 nm. Further interaction of the indicated CSDs with dislocations and twinning result in the emergence of COAs that cannot be formed otherwise. The last stage of emergence and transformation of CSDs is irradiation in the bedrock or in the products of its processing.
Identification of plastic deformation traces is important for the analysis of crystal genesis, since the deformation is possible only in the solid rocks [36, 41]. The majority of crystals subjected to plastic deformation have traces of dissolution and etching, including the ones obtained in the stationary heterophase medium. Given diamond metastability, these conditions correspond to the parameters of an intermediate chamber. Crystals were deformed and dissolved in the cooled fluid-saturated substrate of the intermediate chamber, and their growth did not resume. The majority of studied crystals with traces of dissolution have visualized CL-lines of deformation slipping planes that transverse the entire crystal. No cases of slipping planes bounded by internal areas of the crystal were observed. Obtained results indicate a genetic relationship between plastic deformation and dissolution. Plastic deformation occurred at the last stage of crystallogenesis, after which crystal growth did not resume and no coating was formed.
Hence, in diamond crystals, orientational, zonal and sectoral irregularities are preserved, no traces of recrystallization and post-growth diffusive homogenization are detected. Normal or tangential growth mechanism is a basic distinction of crystals, which explains the specifics of their morphology and impurity-defect composition. Pyramids <100> incorporate more hydrogen and nickel as submicron inclusions, which determines the appearance of PL spectra and characteristics of IRS spectra. Tangential growth of pyramids <111> is primarily associated with incorporation of large monomineral inclusions. Growth disproportioning of nitrogen CSDs and the hydrogen-containing center N3VH between growth pyramids <100> and <111> is observed both in case of their simultaneous growth and in case of their successive alteration.
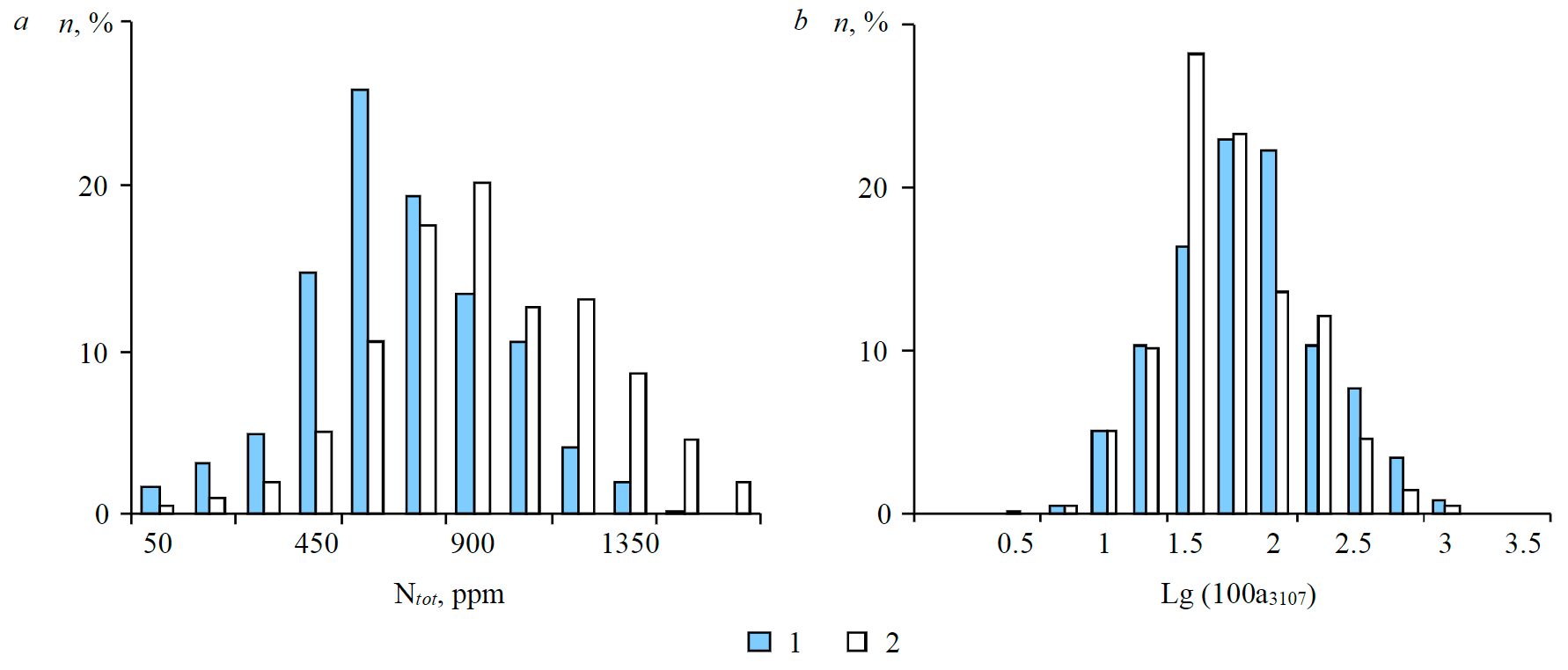
Fig.4. Distribution of Ntot (a) in linear coordinates and distribution of а3107 (b) in semilog coordinates for crystals from the pipes Dachnaya (486 pcs.) and Internatsionalnaya (199 pcs.) 1 – Dachnaya; 2 – Internatsionalnaya
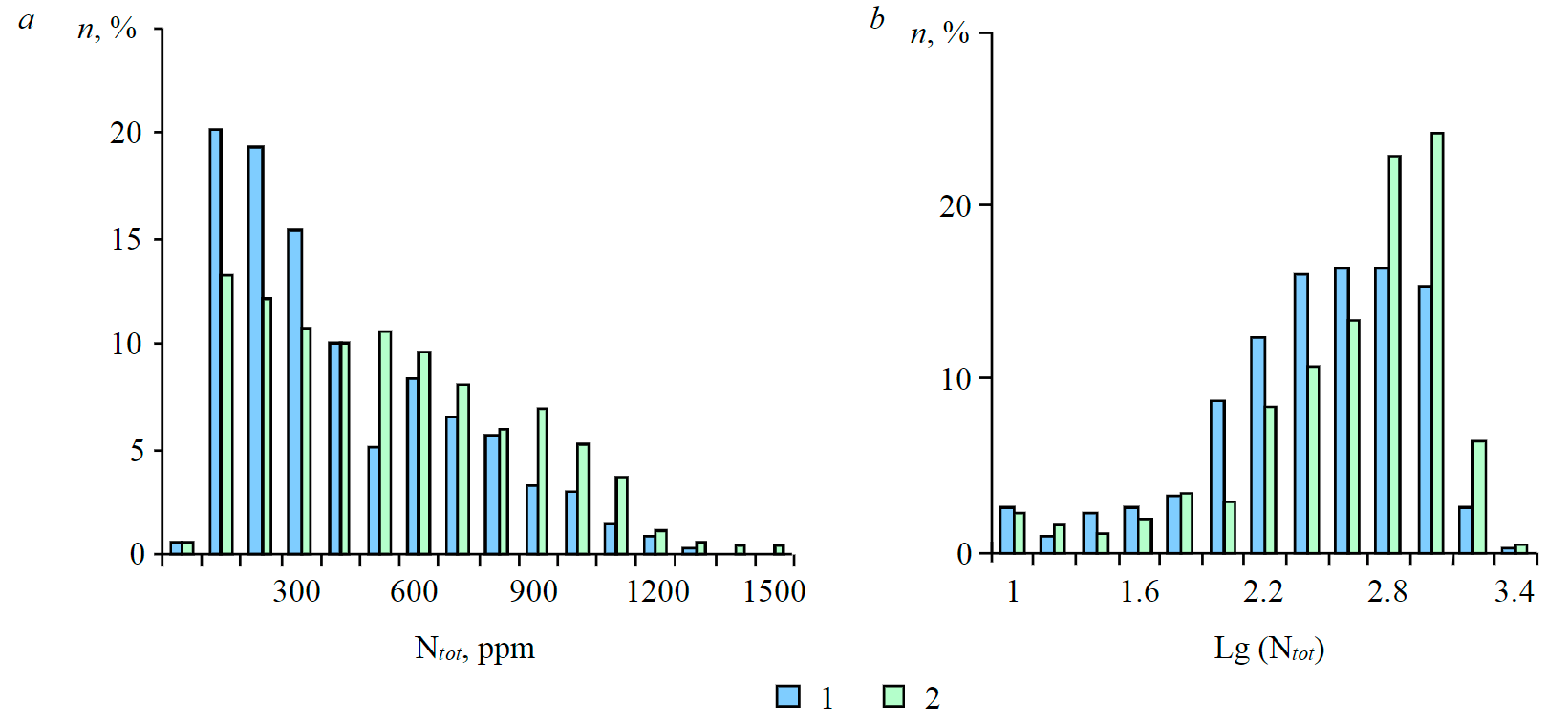
Fig.5. Distribution of Ntot in linear (а) and semilog (b) coordinates for crystals from the pipes Udachnaya (337 pcs.) and Zapolyarnaya (420 pcs.) 1 – Udachnaya; 2 – Zapolyarnaya
Primary deposits can contain several populations – groups that are homogenous in terms of some set of features [12]. Populations can be distinguished by their range of CSD concentrations, dominant specifics of morphology and thermal history. The quantity of octahedral crystals is subordinate in most kimberlite pipes of the Yakutian and Arkhangelsk diamond provinces [1, 9, 10], as well as deposits of other regions [8, 16, 28]. Compared to other deposits, kimberlite pipes Mir, Internatsionalnaya, Dachnaya are unique in their prevalence of octahedral-habit crystals and the absence of cuboids [1, 9]. The distribution of Ntot in the diamonds from these deposits is close to normal (Gaussian), i.e. mostly determined by a single factor (Fig.4, a), which is a sign of a single population. The distribution of а3107 is lognormal (Fig.4, b), multiplicative nature of lognormal distribution corresponds to a complex composition and multiple-stage formation of N3VH center.
At the deposits of Alakit-Markhinsky and Daldynsky kimberlite fields, given the diversity of morphological diamond types, the distribution of Ntot is lognormal (Fig.5, a), which corresponds to a combination of diamond populations from different crystallization stages. As the diversity of morphological types implies a larger share of crystals with pyramids <100>, these samples naturally have higher modal value of а3107 [11] (Fig.5, b).
Diamonds from the Lomonosov deposit are significantly different from crystals of other primary deposits in Russia and worldwide [10]: they contain around 15 % of cuboids and derivative forms of dissolution. The majority of such crystals are colored yellow due to low-temperature C-defects. According to their morphological specifics, crystals are divided into three groups, which can be associated with populations: I – octahedra and octahedroids, flattened trigontrioctahedroids; II – Ural-type dodecahedroids; III – cuboids and tetrahexahedroids. This division is based not only on morphological distinctions, it is primarily explained by the specifics of growth mechanism and thermal history. First of all, crystals of the three identified populations grew under different conditions; secondly, they have different post-growth history, degrees of plastic deformation and dissolution. In Western Urals, diamondiferous formations are divided into two types – modern alluvial placers (AP) and paleodepressions (tuffisite formations) [32, 33]. In order to study formations of the second type, in this work we examined diamonds from the deposit Rassolninskaya depression (RD). Despite the dominance of strongly dissolved Ural crystals in the formations of both types, AP diamonds are distinguished by a large share of cuboids, strong mechanical wear, a set of main CSDs. Among AP samples, we observed crystals with high nitrogen concentration, in their morphology close to V-VII varieties according to Yu.L.Orlov classification. In RD diamonds, distributions of Ntot and NBS are close to normal, which is a sign of a single population. Hence, judging by the nature of distribution of nitrogen defect concentration and morphological proximity, RD diamonds belong to a single population and originate from one primary source.
The following statements are based on performed studies and impose limitations on the model of crystallogenesis and formation of primary diamond deposits [16, 31]: crystal populations formed under the same conditions are characterized by normal distribution of nitrogen concentration; cuboids and mixed habit crystals formed at the first stage of crystallogenesis are always subjected to refaceting; for cubic habit crystals, duration and temperature of natural etching are minimal, cuboids form at the last stage of crystallogenesis; zones that correspond to Ural-type dodecahedroids are never observed in crystal anatomy; there is a possibility of repetitive crystallization cycles; crystal dissolution often occurs under stationary conditions in a heterophase system; there is a genetic relationship between plastic deformation and crystal dissolution; dissolution occurs after plastic deformation of crystals; no crystal growth occurs after plastic deformation; a kimberlite pipe can simultaneously contain dissolved crystals with a simple thermal history and slightly dissolved crystals subjected to natural etching.
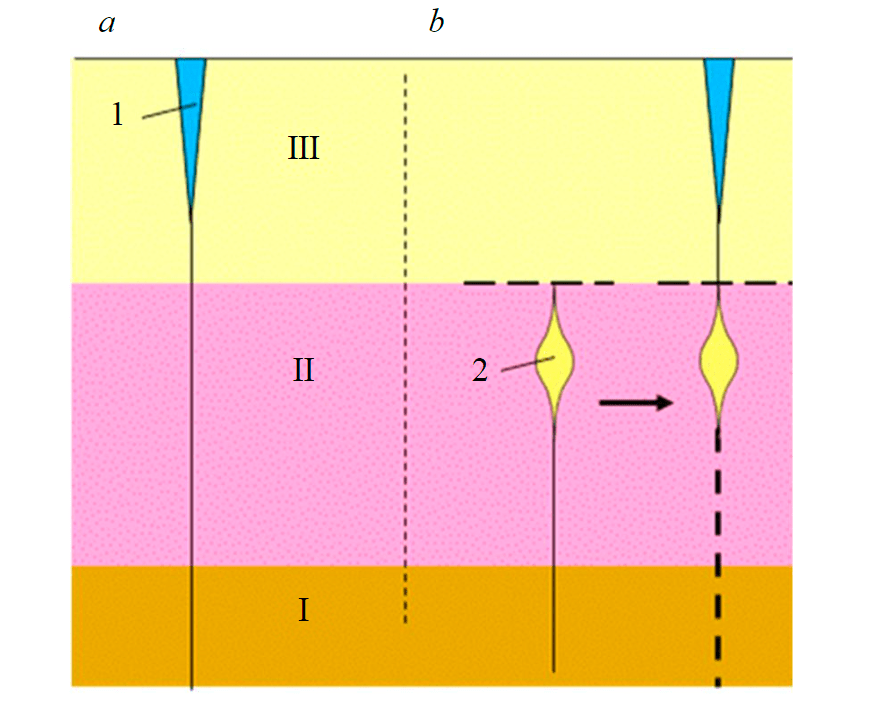
Fig.6. Formation of a kimberlite body with an intermediate chamber as a basis for morphological diversity and specifics of thermal history of the crystals: a – rapid ascent of protokimberlite melt and formation of a kimberlite pipe; b – halted ascent of protokimberlite melt with formation of an intermediate chamber, activation of kimerlite carry-over by the fluid and the next portion of the melt 1– kimberlite body; 2 – intermediate chamber I – region of diamond stability; II – region of diamond metastability, associated with plastic deformation and
Presented statements show that in actual systems, the general pattern of crystallogenesis is complicated by the following circumstances: multiple alteration of conditions with a repetition of crystallogenesis according to the general cycle of crystallization [2]; consequent differences in temperature and duration of post-growth etching; interruption of crystallogenesis and alteration of PT-conditions with partial solidification of the medium.
As we distinguish mantle and kimberlite stages of crystallogenesis, it can be claimed that crystal growth occurs at the first stage, whereas dissolution is associated with the second one: in crystal anatomy there are no forms corresponding to dissolution dodecahedroids, and the coating covers only growth surfaces, not dissolution ones.
All the indicated limitations are in agreement with the formation of kimberlite bodies according to the model of closed fracture dynamics [41] with the possibility of its termination at rheological boundaries and formation of an intermediate chamber; overall schematic that explains these aspects is presented in Fig.6. In the simplest case, after activation of kimberlite formation, a part of the melt with diamonds generated in a single cycle of crystallization starts its rapid ascent to the surface and forms kimberlite bodies. In this case, the deposit is dominated by a single population with minimal dissolution and plastic deformation of crystals (Fig.6, a).
Mir and Internatsionalnaya pipes are examples of deposits with a dominant population. These bodies are unique in their integrity and quality of crystals, relative simplicity of their anatomy, homogeneity of spectroscopic characteristics. In a more complicated case, protokimberlite melt contains several diamond populations that differ in terms of time or depth of their formation. Examples are kimberlite bodies from Alakit-Markhinsky and Daldynsky fields, in which morphological diversity of crystals is accompanied by a wide range of their model temperature [12].
As a portion of protokimberlite melt ascends, it may come to a temporary halt and form an intermediate chamber, where the melt cools and solidifies (Fig.6, b), while some of the crystals become deformed and dissolve. Examples of such formations are Zapolyarnaya pipe and Lomonosov deposit. After subsequent activation, the next portion of fluid or protokimberlite melt, which carries unmodified diamonds, leads to additional deformation and dissolution of crystals before they are brought to the surface. This stage is characterized by pressure and temperature insufficient for crystal growth. Complication of the pattern with the introduction of several populations, formed at different stages of crystallization, and existence of an intermediate chamber allow to explain morphological diversity of crystals, as well as a wide range of CSD concentrations and thermal history. In even more complicated cases, the intervals of parameters and morphological diversity of individual populations overlap. Apparently, such sources are exemplified by the crystals of placers from Anabar-Oleneksky interfluve and Western Urals.
Hence, proposed model based on the possibility of multiply repetitive crystallization cycle and existence of an intermediate chamber allows to explain the sequence of changes in morphology and defect-impurity composition of crystals, as well as a combination of constitutional and population diversity of diamonds in different geological formations.
Conclusions
It is established that a fundamental distinction of diamond crystals is the prevalence of pyramids, which have grown by normal or tangential mechanism: the dominant mechanism determines the specifics of crystal morphology and impurity-defect compositions. Pyramids <100> incorporate more hydrogen and nickel as submicron inclusions, which then determines the appearance of PL spectra and characteristics of IRS spectra. Tangential growth of pyramids <111> is primarily associated with incorporation of large monomineral inclusions. The stage of changing crystal habit shape is associated with quick refaceting of crystals and growth by a regenerative mechanism: this phenomenon explains the absence of cuboids with complex thermal history. Based on the study and systematization of constitutional and population diversity of diamonds, we developed a model of diamond crystallogenesis. This model takes into account a combination of observed specifics of anatomy, thermal history and morphology of diamond crystals from various geological formations. A promising direction for further research is identification of conditions associated with the normal mechanism of diamond growth, which corresponds to the first key stage of diamond formation.
References
- Kostrovitskii S.I., Spetsius Z.V., Yakovlev D.A. et al. Atlas of Primary Diamond Deposits of the Yakutian Diamond Province. Mirnyi: ALROSA, 2015, p. 480 (in Russian).
- Beskrovanov V.V. Diamond Ontogeny. Novosibirsk: Nauka, 2000, p. 264 (in Russian).
- Bogush I.N. Optical and Spectroscopic Properties of Diamonds as Criteria for Forecasting Their Primary Deposits: Avtoref. dis. ... kand. geol.-mineral. nauk. Yakutsk: Institut geologii almaza i blagorodnykh metallov Sibirskogo otdeleniya Rossiiskoi akademii nauk, 2004, p. 20 (in Russian).
- Brodskaya R.L., Marin Y.B. Ontogenic analysis of mineral individuals at micro- and nanolevel for the restoration of ore-forming conditions and assessment of minerals processing properties. Journal of Mining Institute. 2016. Vol. 219, p. 369-376. DOI: 10.18454/PMI.2016.3.369
- Eliseev A.P. Optically Active Nitrogen Centers in Diamonds: Spectroscopy, Structure, Mutual Transformation, Space Distribution: Avtoref. dis. ... d-ra fiz.-mat. nauk. Novosibirsk: Institut geologii i mineralogii im. B.C.Soboleva Sibirskogo otdeleniya Rossiiskoi akademii nauk, 2009, p. 41 (in Russian).
- Zhatnuev N.S. Transmantle (intratelluric) fluid flows: a new model for plumes and plume magmatism. Russian Geology and Geophysics. 2016. Vol. 57. N 8, p. 1135-1142. DOI: 10.1016/j.rgg.2016.08.002
- Zedgenizov D.A. Composition and Evolution of Crystallization Medium of Fibrous Diamonds from the Lithospheric Mantle of the Siberian Platform: Avtoref. dis. ... d-ra geol.-mineral. nauk. Novosibirsk: Institut geologii i mineralogii im. B.C.Soboleva Sibirskogo otdeleniya Rossiiskoi akademii nauk, 2011, p. 35 (in Russian).
- Zinchenko V.N. Morphology of diamonds from kimberlite pipes of Catoca field (Angola). Proceedings of the Russian mineralogical society. 2007. Vol. 136. N 6, p. 91-102 (in Russian).
- Zinchuk N.N., Koptil V.I. Typomorhism of Diamonds of the Siberian Platform. Мoscow: Nedra, 2003, p. 603 (in Russian).
- Kriulina G.Yu. Constitutional Characteristics of Diamonds from the Deposits of Arkhangelsk and Yakutian Diamond Provinces: Avtoref. dis. ... kand. geol.-mineral. nauk. Мoscow: Moskovskii gosudarstvennyi universitet, 2012, p. 24 (in Russian).
- Vyatkin S.V., Kriulina G.Y., Garanin V.K. et al. Morphology and defect-impurity composition of the Zapolyarnaya pipe diamonds. Vestnik Moskovskogo universiteta. Seria 4: Geologia. 2021. N 1, p. 99-109 (in Russian).
- Khachatryan G.K. Nitrogen and Hydrogen in Diamonds of the World as Indicators of Their Genesis and Criteria for Predicting and Searching for Primary Diamond Deposits: Avtoref. dis. ... d-ra geol.-mineral. nauk. Мoscow: Tsentralnyi nauchno-issledovatelskii geologorazvedochnyi institut tsvetnykh i blagorodnykh metallov, 2016, p. 48 (in Russian).
- Shiryaev A.A. Defects and Microinclusions in Diamonds as Indicators of Crystal-Forming Conditions and Post-Growth Changes: Avtoref. dis. ... d-ra khim. nauk. Мoscow: Moskovskii gosudarstvennyi universitet, 2013, p. 41 (in Russian).
- Gaillou E., Post J.E., Rose T., Butler J.E. Cathodoluminescence of Natural, Plastically Deformed Pink Diamonds. Microscopy and Microanalysis. 2012. Vol. 18. Iss. 6, p. 1292-1302. DOI: 10.1017/S1431927612013542
- Chernov A.A.Crystal growth science between the centuries. Journal of Materials Science: Materials in Electronics. 2001. Vol. 12, p. 437-449. DOI: 10.1023/A:1011839431276
- Gurney J.J., Helmstaedt H.H., Le Roex A.P. et al. Diamonds: crustal distribution and formation processes in time and space and an integrated deposit model. Economic Geology. 2005. Vol. 100, p. 143-177. DOI: 10.5382/AV100.07
- Zedgenizov D.A., Harte B., Shatsky V.S. et al. Directional chemical variations in diamonds showing octahedral following cuboid growth. Contributions Mineralogy Petrology. 2006. Vol. 151, p. 45-57. DOI: 10.1007/s00410-005-0044-5
- Dishler B.Handbook of spectral lines in diamond. Springer, 2012. Vol. 1, p. 467. DOI: 10.1007/978-3-642-22215-3
- Dobrinets I.A., Vins V.G., Zaitsev A.M. HPHT – Treated Diamonds: Diamonds Forever. Springer, 2013. Vol. 181, p. 257. DOI: 10.1007/978-3-642-37490-6
- Fedortchouk Y., Zhihai Zhang Diamond resorption: link to metasomatic events in the mantle or record of magmatic fluid in kimberlitic magma? The Canadian Mineralogist. 2011. Vol. 49. Iss. 3, p. 707-719. DOI: 10.3749/canmin.49.3.707
- Melnik A.E., Skublov S.G., Rubatto D. et al. Garnet and zircon geochronology of the Paleoproterozoic Kuru-Vaara eclogites, northern Belomorian Province, Fennoscandian Shield. Precambrian Research. 2021. Vol. 353. N 106014. DOI: 10.1016/j.precamres.2020.106014
- Gulbin Y.L., Mikhalsky E.V. Modeling of mineral parageneses and thermobarometry of metavolcanic rocks of the Ruker Group in the Southern Prince Charles Mountains, East Antarctica. Geology of Ore Deposits. 2020. Vol. 62, p. 584-598. DOI: 10.1134/S1075701520070053
- Kaminsky F.V., Khachatryan G.K. Сharacteristics of nitrogen and other impurities in diamond, as revealed by infrared absorption data. Cаnadian Mineralogist. 2001. Vol. 39. N 6, p. 1733-1745. DOI: 10.2113/gscanmin.39.6.1733
- Kaminsky F.V., Khachatryan G.K. The relationship between the distribution of nitrogen impurity centres in diamond crystals and their internal structure and mechanism of growth. Lithos. 2004. Vol. 77. Iss. 1-4, p. 255-271. DOI: 10.1016/j.lithos.2004.04.035
- Khokhryakov F., Palyanov Y.N. The evolution of diamond morphology in the process of dissolution: Experimental data. American Mineralogist. 2007. Vol. 92. N 5-6, p. 909-917. DOI: 10.2138/am.2007.2342
- Levashova E.V., Skublov S.G., Popov V.A. Distribution of Trace Elements Controlled by Sector and Growth Zonings in Zircon from Feldspathic Pegmatites (Ilmen Mountains, the Southern Urals). Geosciences. 2021. Vol. 11. Iss. 1. N7. DOI: 10.3390/geosciences11010007
- Machevariani M.M., Alekseenko A.V., Bech J. Complex characteristic of Zircon from Granitoids of the Verkhneurmiysky Massif (Amur region). Minerals. 2021. Vol. 11. Iss. 11. N 86. DOI: 10.3390/min11010086
- Mendelssohn M.J., Milledge H.J. Morphological Characteristics of Diamond Populations in Relation to Temperature-Dependent Growth and Dissolution Rates. International Geology Review. 1995. Vol. 37. Iss. 4, p. 285-312. DOI: 10.1080/00206819509465405
- Mironov V.P. Pyramids <001> and <011> in natural diamond // XVI International conference on luminescence and laser physics devoted to the 100th anniversary of Irkutsk State University, 2-7 July, 2018, Arshan, Russia. AIP Conference Proceedings. 2019. Vol. 2069. Iss. 1. N 040006. DOI: 10.1063/1.5089849
- Howell D., O'Neill C.J., Grant K.J. et al. μ-FTIR mapping: Distribution of impurities in different types of diamond growth. Diamond and Related Materials. 2012. Vol. 29, p. 29-36. DOI: 10.1016/j.diamond.2012.06.003
- Navon O. Diamond formation in the Earth's mantle. Proceedings of the 7th International Kimberlite Conference, 11-17 April, 1998, Cape Town, South Africa. Red Roof Design, 1999, p. 584-604.
- Nefedov Y.V., Klepikov Y.V. Occurrence Regularities of Nitrogen Defects in the Ural Type Crystal Diamonds from Different Regions. Key Engineering Materials. 2018. Vol. 769, p. 201-206. DOI: 10.4028/www.scientific.net/KEM.769.201
- Nefedov Y.V., Kochneva O.E., Heide G. The study of the ontogenesis of crystals of the Ural type diamonds by the method of IR-spectrometry. International Scientific and Technical Youth Conference “Perspective Materials of Constructional and Medical Purpose” 26-30 November, 2018, Tomsk, Russian Federation. IOP Conference Series: Materials Science and Engineering. 2018. Vol. 511. N 012041. DOI: 10.1088/1757-899X/511/1/01204
- Palyanov Yu.N., Sokol A.G., Sobolev N.V. Experimental modeling of mantle diamond-forming processes. Russian Geology and Geophysics. 2005. Vol. 46. N 12, p. 1271-1284.
- Pashkevich M.A., Alekseenko A.V. Reutilization prospects of diamond clay tailings at the Lomonosov mine, Northwestern Russia. Minerals. 2020. Vol. 10. Iss. 6. N 517. DOI: 10.3390/min10060517
- Howell D., Piazolo S., Dobson D.P. et al. Quantitative characterization of plastic deformation of single diamond crystals: A high pressure high temperature (HPHT) experimental deformation study combined with electron backscatter diffraction (EBSD). Diamond Related Materials. 2012. Vol. 30, p. 20-30. DOI: 10.1016/j.diamond.2012.09.003
- Ragozin A.L., Zedgenizov D.A., Kuper K.E., Shatsky V.S. Radial mosaic internal structure of rounded diamond crystals from alluvial placers of Siberian platform. Contributions to Mineralogy and Petrology. 2016. Vol. 110, p. 861-875. DOI: 10.1007/s00710-016-0456-0
- Kriulina G.Y., Iskrina A.V., Zedgenizov D.A. et al. The Compositional Peculiarities of Microinclusions in Diamonds from the Lomonosov Deposit (Arkhangelsk Province). Geochemistry International. 2019. Vol. 57, p. 973-980. DOI: 10.1134/S0016702919090052
- Gurney J.J., Hildebrand P.R., Carlson J.A. et al. The morphological characteristics of diamonds from the Ekati property, Northwest Territories, Canada. Lithos. 2004. Vol. 77. Iss. 1-4, p. 21-38. DOI: 10.1016/j.lithos.2004.04.033
- Fedorova E.N., Logvinova A.M., Lukyanova L.I., Sobolev N.V. Typomorphic characteristics of the Ural diamonds (from FTIR spectroscopy data). Russian Geology and Geophysics. 2013. Vol. 54. Iss. 12, p. 1458-1470. DOI: 10.1016/j.rgg.2013.10.013
- Titkov S., Krivovichev S., Organova N. Plastic deformation of natural diamonds by twinning: the evidence from X-ray diffraction studies. Mineralogical Magazine. 2012. Vol. 76. Iss. 1, p. 143-149. DOI: 10.1180/minmag.2012.076.1.143
- Zaitsev A.M. Optical Properties of Diamond: Data Handbook. Springer, 2001, p. 502. DOI: 10.1007/978-3-662-04548-0