Study of the dynamics for gas accumulation in the annulus of production wells
Abstract
Accumulation of associated petroleum gas in the annulus is one of the negative factors that impede the intensification of mechanized oil production. An increase in annular gas pressure causes growth of bottomhole pressure, a decrease in back pressure to the formation and the inflow of formation fluid. In addition, accumulation of gas in the annulus leads to displacement and a decrease in the liquid level above the submersible pump. Insufficient level of the pump submersion (rod or electric submersible) causes a number of complications in the operation of mechanized production units associated with overheating of the elements in pumping units. Therefore, the development of technologies for optimizing the gas pressure in the annulus is relevant. Method for calculating the intensity of gas pressure increase in the annulus of production wells operated by submersible pumps has been developed. Analytical dependence for calculating the time interval of gas accumulation in the annulus, during which the dynamic level decreases to the pump intake, is obtained. This value can be used to estimate the frequency of gas withdrawal from the annulus using compressors. It has been found that the rate of increase in annular gas pressure in time increases non-linearly with a rise in the gas-oil ratio and a decrease in water cut, and also linearly increases with a rise in liquid flow rate. Influence of the operating (gas-oil ratio) and technological (value of the gas pressure maintained in the annulus) factors on the flow rate of the suspended reciprocating compressor driven by the beam engine, designed for forced withdrawal and redirection of the annular gas into the flow line of the well is analyzed.
Introduction
Decrease in flow rates of production wells and an increase in the share of complicated wells are the defining trends in the modern oil and gas industry. Influence of associated petroleum gas is one of the factors hindering the achievement of the maximum flow rate potential of the wells. The gas separated at the intake of the submersible pump accumulates in the annulus, causing a gradual pressure increase in it [6]. An increase in annular gas pressure leads to a corresponding increase in bottomhole pressure and a decrease in back pressure on the reservoir. Negative effect of gas accumulation in the annulus is also associated with the displacement and reduction of the dynamic liquid level to the pump suspension depth. Insufficient liquid level above the pump causes complications in the operation of pumping units: an increase of the friction force in the plunger pair, jamming and thermal locking of the plunger in rod units; overheating and melting of the power cable of electric submersible pumps [1, 9, 24]. Furthermore, an insufficient liquid level above the pump can lead to a decrease in the efficiency of pumping units [5]. High values of the annular gas pressure as a component of the bottomhole pressure requires more accurate methods for recalculating the wellhead parameters when measuring the level recovery curve [6]. In general, problem of pressure growth at the intake of pumping units is relevant both for the fields of the Volga-Ural region [3] and Western Siberia [11]. Therefore, a number of investigations are aimed at studying the properties of gas and gas-liquid mixture in the annulus [10]. Field measurements of associated gas consumption for fields in Western Siberia were carried out, as well as chromatographic, physical and chemical analyzes for the properties of associated gas [16]. The work [11] analyzed the change in the gas factor in time.
Thus, the study of gas accumulation dynamics and the development of technologies for optimizing gas pressure in the annulus of wells is one of the relevant and key tasks of cost-effective development. To solve it, various technologies are used, including: redirecting the gas from the annulus to the flow line (pumping pipes) using a check valve [14], an ejector [4] and a jet pump [13]; forced withdrawal of annular gas by a pumping unit or compressor with subsequent collection into storage tanks or directing into a collector [12]. It is necessary to develop substantiated methods for predicting the dynamics of gas accumulation in the annulus, taking into account the individual operating conditions of the well and the rheological parameters of the pumped product, in order to ensure the effectiveness of various compression technologies and to calculate the optimal parameters for pumping the annular gas. An important task is to assess the frequency of gas sampling. Solution for the set tasks is based on mathematical modeling of the gas accumulation process in the annulus. Many works are devoted to these questions. Moreover, various models are used to describe wells without any complicating factors and wells with complex geological conditions, such as large well depth and high formation temperature. Calculations of the supercompressibility coefficient for the main components of natural gas by the method of molecular dynamics are shown in work [7]. Article [18] analyzes the annular pressure at an early stage of production in wells with high formation temperature and pressure. Work [34] analyzes the APB (Annular Pressure Build Up – increase of pressure in the annular space) – a model for the annular pressure increase in the well. Transient processes in the annulus of deep offshore wells are described in [22]. In addition to physical models, machine-learning models [26], modeling in software products, for example, in HYSYS [8] and experimental investigations [2] are used to solve the problems of describing the processes of annular pressure increase. Works [8] and [2] show the relationship between the gas content in water and the growth of the actual gas-oil ratio. Work [21] proposes a thermodynamic model based on Mathias's modification of the Soave – Redlich – Kwong equation of state, which was used to describe the dissolution of two-component systems of hydrocarbons in water. Article [33] shows the effect of wellbore heating on the increase of annular pressure. Influence of various factors on the process of gas release in the annulus led to the need to develop methods for predicting the values of annular gas pressure [17].
Many works are devoted to technical devices that allow increasing the operating efficiency of pumping units at high gas content. Results of studying the efficiency of jet devices for pumping gas from the annulus of a well are presented in [9]. Analysis [28] showed the effect of using devices for pumping gas from the annulus. Investigations of gas separators for rod pumping units have presented the area of their efficient application [20]. Work [25] highlights the effect of gas on the operation of electric submersible pumps. Review [15] shows tools for reducing the effect of gas on the operation of rod pumps and their efficiency in various operating conditions. Work [32] presents the results for the development of the technology for optimizing the operating mode of rod pumps when producing oil with a high gas content. Results for the development of the design for a multiphase rod pump are presented in [24]. Study [23] presents the results for testing a rod pump with electromagnetic valves for operation in wells with a high gas-oil ratio. It is noted [35] that the presence of gas significantly affects the operation of volume pumps, therefore, it is necessary to use various tools to improve their efficiency. Work [19] shows a significant effect from the introduction of technical and technological solutions to reduce the gas pressure in the annulus. Overview of methods for reducing pressure in the annulus is presented in [27]. One of the promising trends for reducing annular gas is the use of compressors for pumping gas driven by a beam engine [29-31].
Investigations
Mathematical model has been developed to assess the dynamics of annular gas pressure increase and decrease of the liquid level in the well over time and to assess the period of gas accumulation. Model takes into account the operating conditions: gas-oil ratio, water cut of the produced product, well flow rate for liquid and other parameters
Mathematical model
Gas in the annulus is described by the equation of state for a real gas. At the time t, the next equality is true [13]
where Pz, Vz – pressure (Pa) and volume (m3) of the gas in the annulus, respectively; m – gas mass, kg; μ – molar gas mass, kg/mol; R – universal gas constant, J·K–1·mol–1; z – supercompressibility coefficient of gas; T – gas temperature, К.
Then at the moment t + dt it can be written as follows:
Expanding this expression, the equation for the differentials Pz, Vz, m is obtained:
Let us assume that when the annular pressure and the dynamic level change, the intake pressure, which ensures the maximum volumetric gas content (equal to 25 %), remains constant [8]
where ρo – density of liquid in the annulus, kg/m3; g – acceleration of gravity, m/s2; Hp, Hd – pump descent depth and dynamic liquid level, respectively, m.
Maximum volumetric gas content can be calculated by solving the non-linear equation,
where Ql – flow rate of liquid, m3/day; B – water cut of the product, units; GF – gas-oil ratio of formation oil, m3/m3; Rs – gas solubility in oil, m3/m3; Bg – gas volumetric ratio; σ – annulus gas separation coefficient; in index corresponds to the pressure and temperature conditions at the pump intake.
Differentiating the expression (2), change in the gas volume in the annulus as a function of the pressure change is expressed as
where Sz – square of annulus area, m2.
Amount of gas per unit of time entering the annulus is determined by the formula [8]:
where ρin – gas density, kg/m3.
Substituting formulas (3) and (4) into expression (1), we obtain:
Equation (5) yields an equation for calculating the derivative of the gas pressure in the annulus with respect to time:
Expressing from equation (2) the volume of annular gas
and substituting it into formula (5), we obtain:
Accumulation period of annular gas is estimated, assuming that the lower boundary of the dynamic level corresponds to the pump intake with a certain minimum margin ΔHmin. Then the limit annular gas pressure Pz max, corresponding to a given position of the dynamic level, is
By integrating the left and right sides of equation (6) from the initial pressure Pz0 to the final limit annular gas pressure Pz max, an analytical expression for estimating the accumulation period of annular gas is obtained
Separation coefficient in formula (8) is calculated according to the known dependence [5]:
where Dc, Dt – casing inner diameter and tubing outer diameter, respectively, mm.
Known empirical correlations are used to calculate the physical properties of oil and gas [28]. In particular, solubility of gas in oil is calculated according to the formula:
where ρo0, ρg0 – density of oil and gas in normal conditions, respectively, kg/m3.
Results
Let us construct the dependencies for the dynamics of the gas pressure in the annulus and the gas accumulation period T on the gas-oil ratio, water cut, liquid flow rate for typical operating conditions (using the example of a model well). Well parameters and produced products: tubing outer diameter 62 mm; casing inner diameter 130 mm; oil density under normal conditions 800 kg/m3; gas density under normal conditions 1.3 kg/m3; molar mass of gas 0.029 kg/mol; pump descent depth 1000 m; intake pressure 2.5 MPa; liquid flow rate 5, 10, 20 m3/day; the minimum margin of the liquid level above the pump is 20 m; gas-oil ratio of formation oil 20-200 m3/m3; water cut 0-90 %.
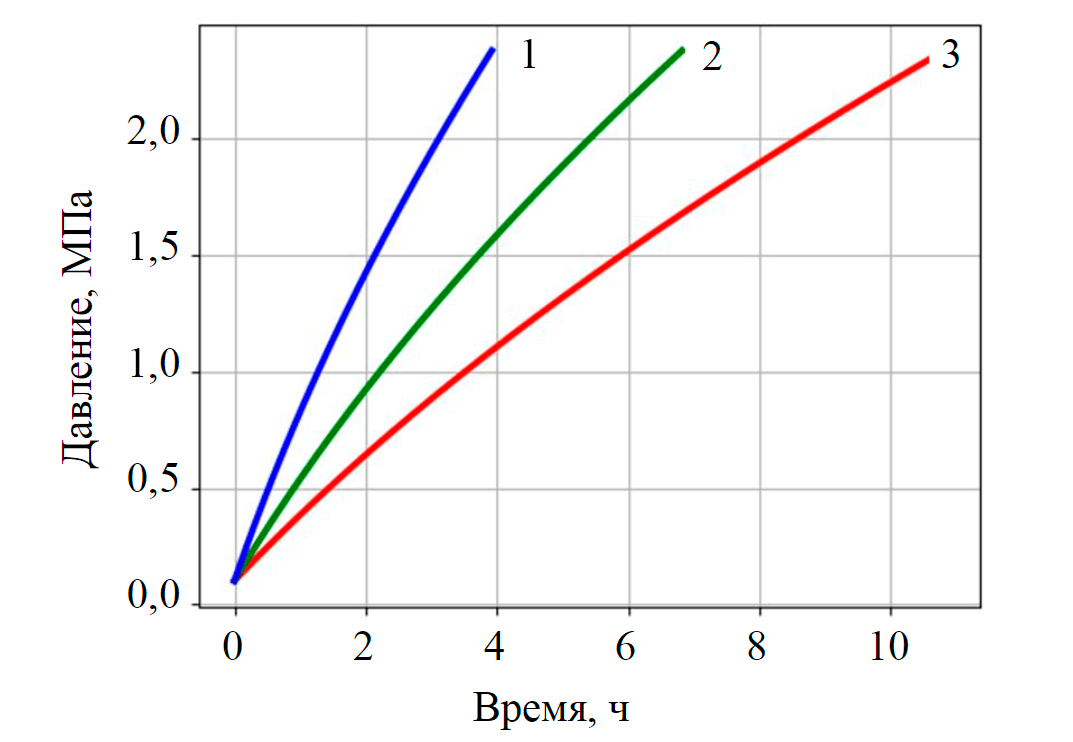
Fig.1. Rate of increase for the annular gas pressure at different gas-oil ratio (water cut 30 %, flow rate for liquid 20 m3/day) 1 – 150; 2 – 100; 3 – 75 m3/m3
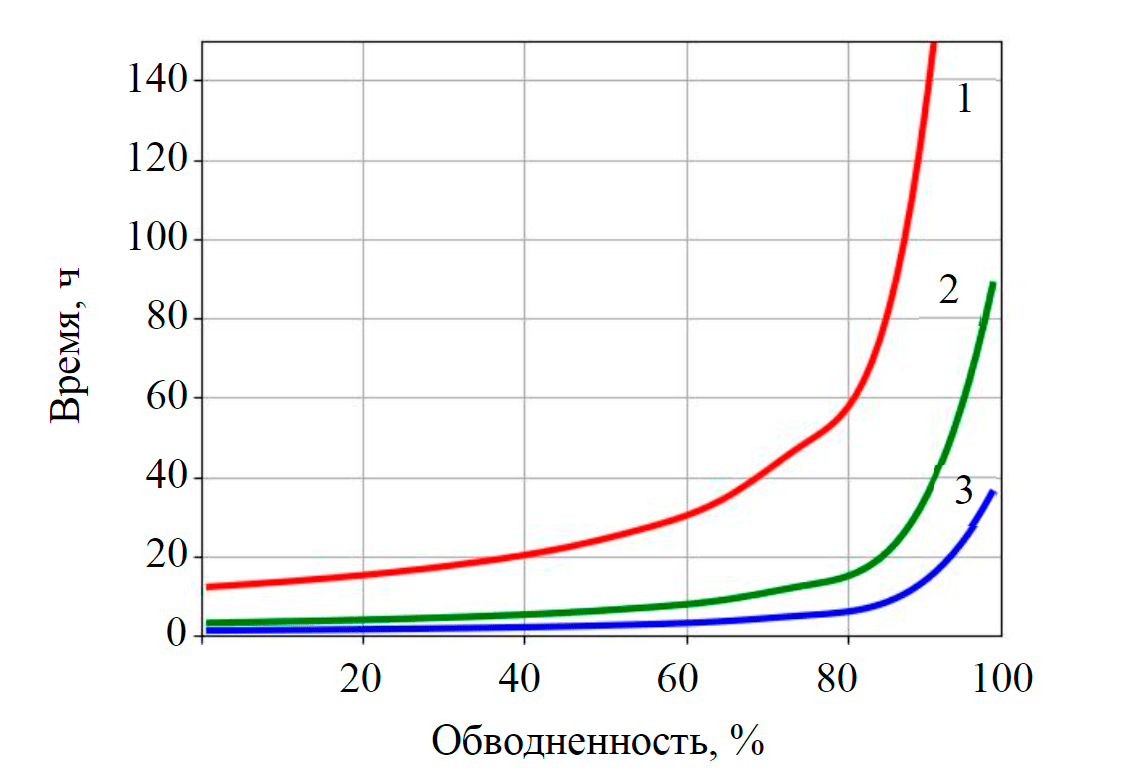
Fig.3. Dependence of the annular gas accumulation period on water cut for various gas-oil ratio (flow rate for liquid 20 m3/day) 1 – 50; 2 – 100; 3 – 200 m3/m3
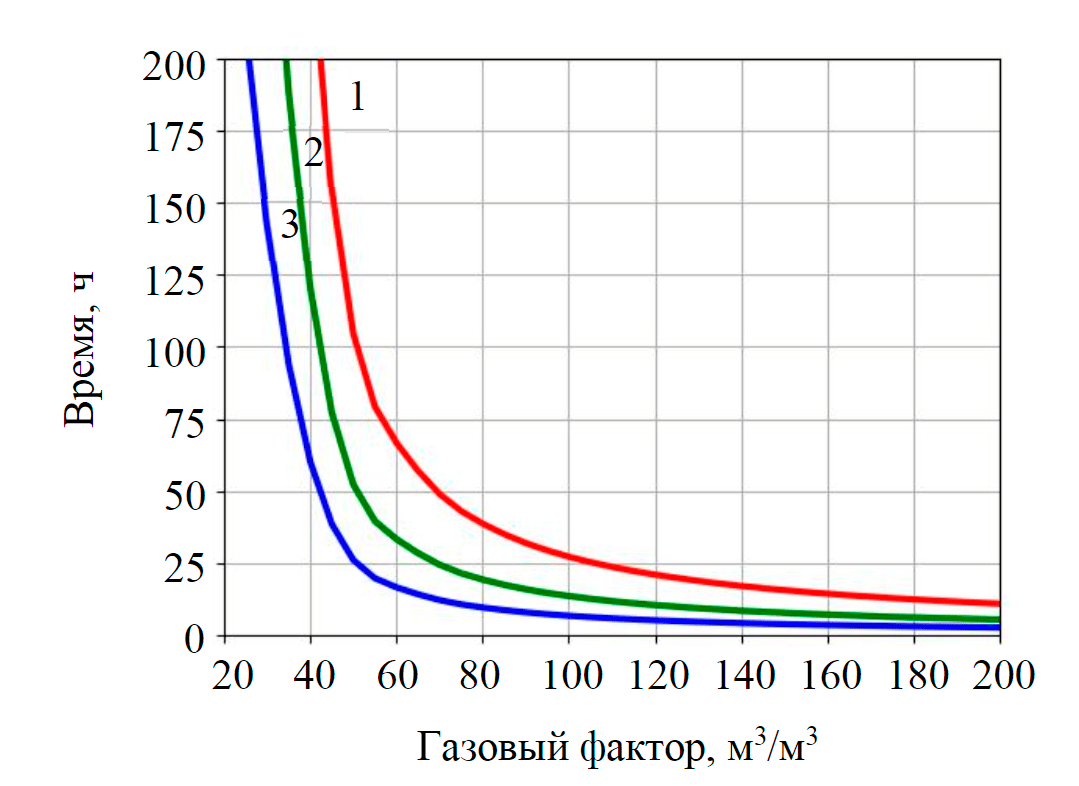
Fig.4. Dependence of the accumulation period on the gas-oil ratio, at liquid flow rate (water cut 30 %) 1 – 5; 2 – 10; 3 – 20 m3/day
Rate of increase in the annular gas pressure over time has a significant dependence on the water cut C and the gas-oil ratio G (Fig.1-3). Graphs are constructed taking into account the achievement of the maximum annular gas pressure equal to 2.4 MPa, at which the submersion of the pump under the dynamic level does not exceed 20 m, i.e. the dynamic level is approaching the pump intake. It can be seen (Fig.1) that the rate of increase for gas pressure rises non-linearly with an increase in the gas-oil ratio. Thus, with a gas-oil ratio of 75 m3/m3, the period for reaching the maximum annular gas pressure (equivalent to the period of decrease in the dynamic level to pump intake) is about 11 h, with an increase in the gas factor twice, up to 150 m3/m3, the period for reaching the maximum annular gas pressure decreases by more than 2.5 times, up to 4 h.
Rate of increase for the annular gas pressure decreases as the water cut rises (Fig.2, 3). With an increase in water cut from 10 to 80 %, annular gas accumulation period increases from 5 to 24 h, i.e. more than four times. It can also be seen at the graphs in figures 1, 2 that the gas pressure increases non-linearly with time – the intensity of increase in gas pressure decreases with time. Analysis of formula (7) shows that the dependence of the gas pressure in the annulus is a power function of the form on time, proportional to $\sqrt{t}$.
Graph (Fig.3) shows the results of calculating the accumulation period of annular gas at different values of water cut and gas-oil ratio. It can be seen that with an equal water cut, the duration of the annular gas accumulation period significantly decreases with an increase in the gas-oil ratio. With a gas-oil ratio of 50 m3/m3, the value of T increases by 12-150 h with an increase in water cut to 90 %, which is due to the low solubility of the gas in the aqueous phase (Fig.3). With an increase in the gas-oil ratio to 200 m3/m3, period T decreases significantly and in the water cut range of 0-90 % varies in the range of 1-36 h.
Rate of increase for annular gas pressure rises with an increase in the flow rate of the produced product with a dependence close to linear (Fig.4).
With a fourfold increase in flow rate from 5 to 20 m3/day and a gas-oil ratio of 50 m3/m3, period of pressure increase in the annular space decreases from 100 to 25 h. Similarly, for a gas-oil ratio of 100 m3/m3, the period of the annular pressure increase decreases from 28 to 7 h.
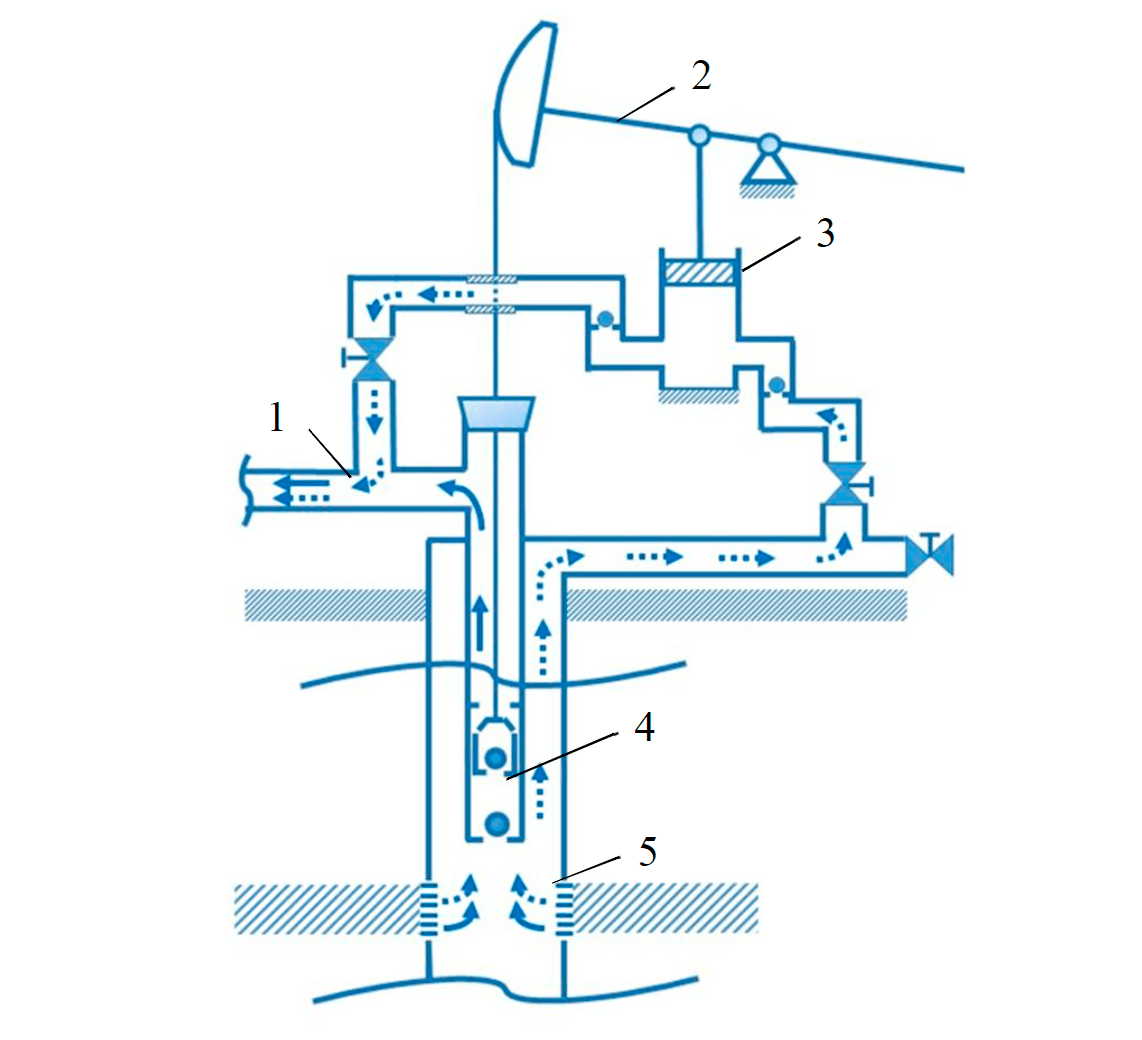
Fig.5. Rod unit with a reciprocating suspended compressor (arrows schematically show the direction of flow movement) 1 – flow line; 2 – beam engine; 3 – piston of suspended compressor; 4 – rod pump; 5 – near-bottomhole zone
Thus, period of gas pressure increase in the annulus decreases non-linearly with an increase in the gas-oil ratio and a decrease in water cut, in particular. It can be seen in the Fig.3 that with a gas-oil ratio of more than 100 m3/m3 and a water cut of up to 80 %, duration of annular gas accumulation is less than a day and can reach several hours. In this case, when using compressors, it is feasible to implement the mode of continuous withdrawal of annular gas. When operating wells with rod pumping units, one of the promising options is the use of suspended reciprocating compressor built into the kinematics of the beam engine (Fig.5). Suspended compressor is used to withdraw and redirect gas from the annulus to the flow line of the well, and the number of double strokes per minute of the compressor piston coincides with the number of strokes in the beam engine. Withdrawal parameters of the annular gas by a reciprocating compressor are calculated from the condition of the balance for the volume of gas. This condition considers the gas separated at the pump intake, which then enters the annulus, and the compressor flow rate (taking into account the gas volumetric ratio):
where Qk – compressor flow rate, m3/day; Fp – compressor plunger cross-sectional area, m2; S – stroke length of the compressor piston, m; n – number of double strokes, min–1; Kп – flow rate coefficient; Bgz – volumetric gas expansion coefficient in annular conditions.
Calculated graphs (Fig.6) show the effect of the gas-oil ratio on the compressor piston diameter required to maintain a given annular pressure. In the calculations, it is assumed that the stroke length of the compressor piston S = 1 m, number of double strokes per minute n = 6, compressor flow rate coefficient Kп = 0.5.
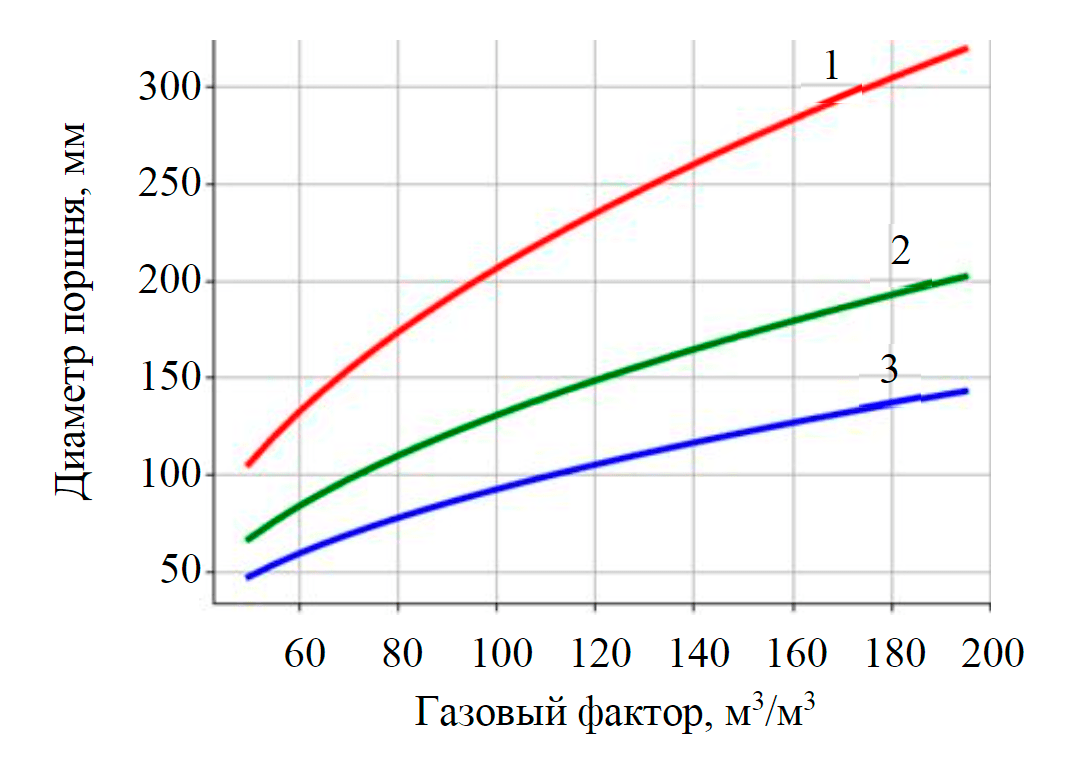
Fig.6. Influence of the gas-oil ratio on the piston diameter of the compressor at different annular gas pressures (water cut 70 %) 1 – 0.2; 2 – 0.5; 3 – 1 MPa
As the gas-oil ratio increases, the required compressor flow rate increases, and the piston diameter required to maintain the required flow rate also increases. For example, for an annular gas pressure of 1 MPa, with an increase in the gas-oil ratio from 50 to 200 m3/m3, the piston diameter increases from 47 to 144 mm (required compressor flow rate increases from 7 to 70 m3/day). With a decrease in the value of the maintained annular gas pressure from 1 to 0.2 MPa, the piston diameter increases from 90 to 205 mm, required compressor flow rate increases, respectively, from 30 to 142 m3/day (with a gas-oil ratio of 100 m3/m3), since as the pressure decreases, the gas expands and its density decreases, as a result of which the mass flow rate of the gas through the compressor decreases. In this regard, in order to increase the efficiency of gas pumping by the compressor, it is rational to maintain the maximum permissible annular gas pressure, at which the necessary submersion of the pump under the dynamic level is ensured: Pz.opt = Pin – ρоgΔHopt; Pz.opt – optimum annular gas pressure, MPa; ΔHopt – optimal submersion of the pump under the dynamic level, m (taken equal to 200 m). In the calculation example, at a gas pressure in the annulus of 0.2, 0.5 and 1 MPa, the dynamic level was 700, 740 and 800 m, respectively, the submersion of the pump under the level is 300, 260 and 200 m, thus the annular gas pressure of 1 MPa is optimal, providing the minimum flow rate of the compressor and the diameter of its piston.
Analysis of application boundaries for suspended compressors driven by a beam engine
Analysis of the technological regime for the well number equipped with rod pumps at the fields of the Ural-Volga region showed that more than 15 % of wells have an annular pressure of more than 1 MPa and about 2-3 % of wells more than 2 MPa. In this case, the gas-oil ratio can reach 400 m3/ton. Under such conditions, efficiency of the rod pump operation is significantly reduced, and in some cases, there is a cease of the flow rate.
It is necessary to reduce the annular pressure to a level of 0.4-0.6 MPa to reduce the gas influence. This is due to intensive foaming at lower pressures, which complicates the possibility of determining the dynamic level of the liquid in the well and establishing the optimal operating regime.
According to the calculations, for more than 95 % of all wells complicated by high gas-oil ratio, the maximum gas flow into the annulus is 1400 m3/day (initial data for the calculation: pressure in the annulus 0.5 MPa, gas-oil ratio 200 m3/ton, oil flow rate 8 ton/day, gas separation coefficient at the rod pump 0.5). Moreover, for more than 95 % of cases, the maximum line pressure is 3 MPa. Thus, when forming the nomenclature range, the compressor with the maximum flow rate should provide a flow rate of 1400 m3/day (under normal conditions) and an operating pressure of up to 3 MPa.
To find the minimum required compressor flow rate, a similar series of calculations was carried out, which showed that the compressor of the minimum flow rate should provide 20 m3/day.
Design features of the compressor considered allow changing the flow rate only by piston diameter, number of cylinders (main parameter), or by changing the stroke length (auxiliary parameter). In this case, size of the piston diameter is limited by the possibilities of providing the axial load. Therefore, at a linear pressure (pumping pressure of the compressor into the flow line) equal to 2 MPa, with a piston diameter of 100 mm, the axial load should be more than 1.5 ton (15 700 N).
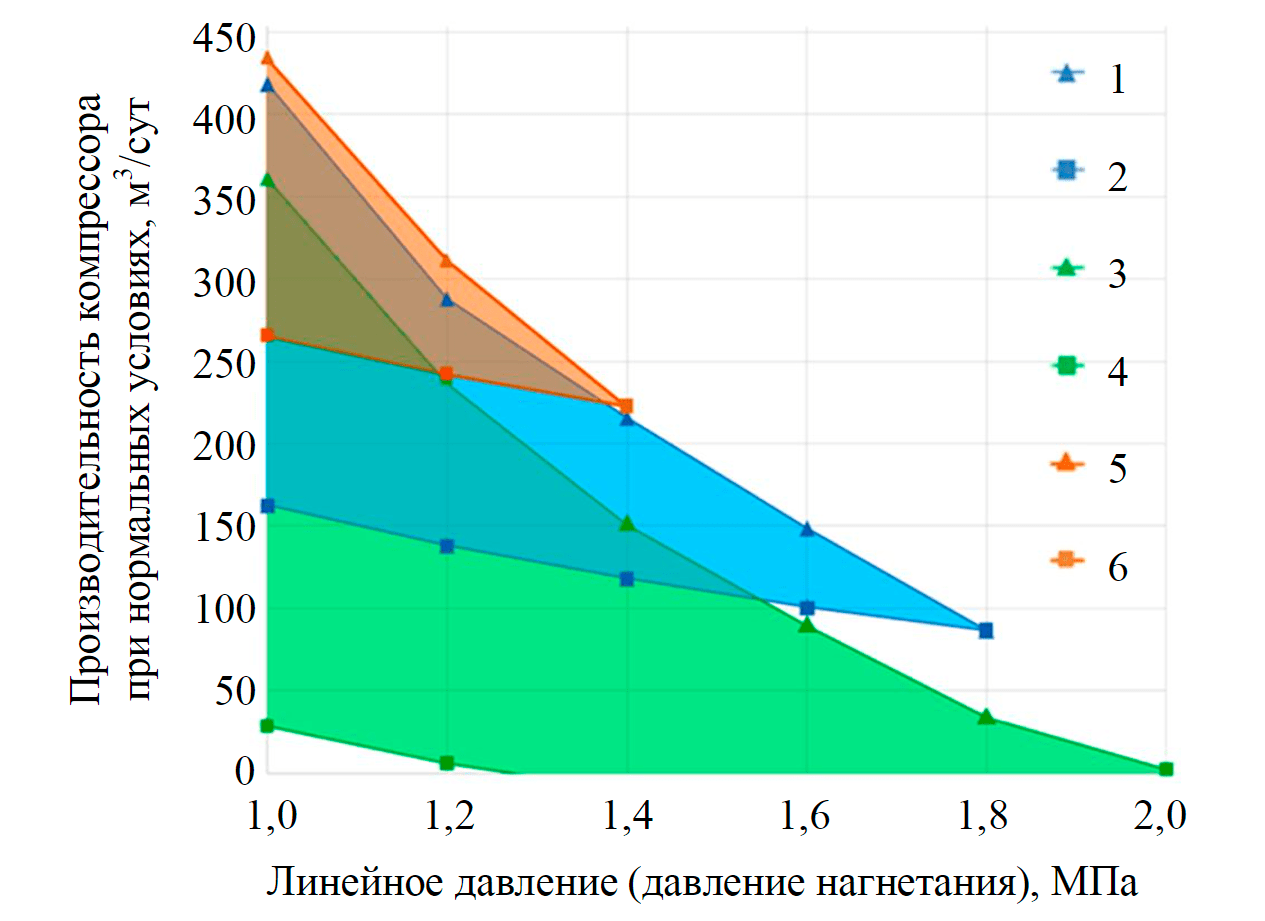
Fig.7. Estimated performance of various compressor diameters at maximum and minimum piston stroke lengths Diameter of the maximum piston stroke length: 1 – 125; 3 – 95; 5 – 144 mm. Diameter of the minimum stroke length of the piston: 2 – 125; 4 – 95; 6 – 144 mm
To determine the control ranges for compressors of various diameters, a limitation on the load of 500 kg, relative to the suspension point of the rods, is set. Calculations have established that when using a rod pump cylinder with a diameter of 95 mm as a compressor cylinder, the maximum regulation area is covered, highlighted in green in Fig.7. At pumping pressures up to 2 MPa, it is possible to establish minimum compressor flow rate values. Increasing the piston diameter to 125 mm (blue area in Fig.7) increases the load required to operate the compressor at a given pressure. Therefore, the maximum pressure provided by the compressor is limited to 1.8 MPa. At the same time, the minimum compressor flow rate is more than 100 m3/day at almost all pressures. A further increase in the diameter of the compressor piston does not significantly increase its flow rate (due to the indicated limitations).
Recommended cylinder diameter is 95 mm. This allows ensuring its manufacture from blanks of rod pump cylinders. If it is necessary to install a compressor with a higher flow rate, it is recommended to install multistage compressors, for example, the structures presented in [3]. In general, the application field of the considered compressor design for pumping gas under various operating conditions should be determined by calculations.
Conclusion
- High annular gas pressure has a negative effect on the efficiency of artificial lift of oil due to the increased risk of lowering the dynamic level up to pump intake, which reduces the performance of well pumps and flow rate.
- A method and an algorithm for calculating the rate of increase in gas pressure in the annulus, depending on the operating parameters and rheological properties of the pumped fluid, have been developed. An analytical dependence is obtained for calculating the gas accumulation period in the annulus, during which the dynamic level decreases down to the pump intake. Model calculations have been performed showing the relationship between the gas-oil ratio, well flow rate, water cut and the duration of the pressure increase period.
- It was found that the intensity of the annular gas pressure increase in time rises non-linearly with an increase in the gas-oil ratio and a decrease in water cut, and also rises linearly with an increase in the liquid flow rate. Duration of the gas accumulation period varies from several hours to several hundred hours, depending on the values of the gas-oil ratio, water cut and liquid flow rate.
- Influence of the operating (gas-oil ratio) and technological (value of the gas pressure maintained in the annulus) parameters on the flow rate of a suspended reciprocating compressor driven by a pumping unit, designed for forced withdrawal and redirection of annular gas into the flow line of he well, is analyzed. It was established that with an increase in the gas-oil ratio, required compressor flow rate increases, and the piston diameter required to maintain a given flow rate is also increased.
- It was found that with a decrease in the value of the annular gas pressure maintained, required compressor flow rate increases. Mass flow rate of the gas pumped out by the compressor decreases due to the expansion of the gas.
References
- Baimukhametov M.K., Gulishov D.S., Mikhaylov V.G. et al. Analysis of causes of gaszoil ratio growth at late stages of oil fields exploration. Izvestiya Tomskogo politekhnicheskogo universiteta. Inzhiniring georesursov. 2018. Vol. 329. N 8, p. 104-111 (in Russian).
- Gultyaeva N.A., Toshev E.N. Mass exchange in the oil-gas-water and its effect on the production of associated gas. Neftyanoe khozyaistvo. 2013. N 10, p. 100-103 (in Russian).
- Imashev R.N., Fedorov V.N., Zaripov A.M. On the gas factor change in the process of Arlanskoye field development. Neftyanoe khozyaistvo. 2016. N 8, p. 122-125 (in Russian).
- 4. Sevastyanov A.V., Mingulov Sh.G., Nigay Yu.V. Research and optimization of gas extraction from the annular space of oil wells. Problemy sbora, podgotovki i transporta nefti i nefteproduktov. 2016. N 2 (104), p. 42-48. DOI: 10.17122/ntj-oil-2016-2-42-48 (in Russian).
- Lekomtsev A.V., Mordvinov V.A., Poplygin V.V., Ponomareva I.N. Efficiency of electric submersible pumps at extraction of the gas-liquid blends from wells. Neftyanoe khozyaistvo. 2012. N 10, p. 132-133 (in Russian).
- Lekomtsev A.V., Martyshev D.A. Comparative analysis of methods for determining BHP during well test. Neftyanoe khozyaistvo. 2014. N 6, p. 37-39 (in Russian).
- Malyshev V.L., Moiseeva E.F., Kalinovsky Yu.V. Calculation of compressibility factor of main natural gas components by means of molecular dynamics simulations. Izvestiya Tomskogo politekhnicheskogo universiteta. Inzhiniring georesursov. 2019. Vol. 330. N 11, p. 121-129. DOI: 10.18799/24131830/2019/11/2356
- Mikhaylov V.G., Ponomarev A.I., Topolnikov A.S. Prediction of gas factor taking into account gas dissolved in the water at late stages development of oil fields. SOCAR Proceedings. 2017. Vol. 3, p. 41-48. DOI: 10.5510/OGP2017030032 (in Russian).
- Molchanova V.A., Topolnikov A.S. Study of the efficiency of the device for pumping gas from the annular space. Neftepromyslovoe delo. 2007. N 10, p. 34-40 (in Russian).
- Mordvinov V.A., Lekomtsev A.V., Martyushev D.A. Estimation of input pump pressure during low foaming gas-content oil pumping. Neftyanoe khozyaistvo. 2014. N 6, p. 61-63 (in Russian).
- Kordik K.E., Shkandratov V.V., Bortnikov A.E., Leontyev S.A. About trends in the oil-gas ratio change in the process of exploitation of Lukoil-West Siberia LLC fields. Neftyanoe khozyaistvo. 2016. N 8, p. 54-57 (in Russian).
- Isaev A.A., Takhautdinov R.Sh., Malykhin V.I., Sharifullin A.A. Development of the automated system for gas extraction from wells. Neft. Gaz. Novatsii. 2017. N 12 (205), p. 65-72 (in Russian).
- Urazakov К.R., Muhin I.А., Vahitova R.I. Modeling the characteristics of jet pump. Elektrotekhnicheskie i informatsionnye kompleksy i sistemy. 2015. Vol. 11. N 4, p. 41-50 (in Russian).
- Isaev A.A., Takhautdinov R.Sh., Malykhin V.I., Sharifullin A.A. Gas removal efficiency from a well. Georesursy. 2018. Vol. 20. N 4. Part 1, p. 359-364. DOI: 10.18599/grs.2018.4.359-364
- Allison A.P., Leal C.F., Boland M.R. Solving gas interference issues with sucker rod pumps in the Permian Basin. SPE Artificial Lift Conference and Exhibition – Americas, 28-30 August, 2018, The Woodlands, Texas, USA. Society of Petroleum Engineers, 2018. SPE-190936-MS. DOI: 10.2118/190936-MS
- Almukhametova E.M., Mikhaylov M.O., Stepanova R.R. Determination of the gas factor of oil at Western Siberia fields. International Symposium “Earth sciences: history, contemporary issues and prospects”, 10 March, 2020, Moscow, Russian Federation. IOP Conference Series: Earth and Environmental Science. 2020. Vol. 579. N 012018. DOI: 10.1088/1755-1315/579/1/012018
- Wang Weizhang, Zuo Xiangzuo, Zhao Hui. Analysis on tubing-casing annular gas production. Oil Drilling & Production Technology. 2008. Vol. 30. Iss. 3, p. 52-55.
- Cheng LI, Zhichuan Guan, Bo Zhang et al. Analysis of Tubing-Casing Annular Pressure in Early Production Stage in High Temperature High Pressure Wells. Offshore Technology Conference Asia, 2 November – 19 August, 2020, Kuala Lumpur, Malaysia. OnePetro. 2020. OTC-30059-MS. DOI: 10.4043/30059-MS
- Yonghui Liu, Yingchuan Li, Xiaoqi Li, Guilin Tang. An Approach to Apply the Gas in the Annulus to Assist Pumping Oil in High GOR Wells. Production and Operations Symposium, 31 March – 3 April, 2007, Oklahoma City, Oklahoma, USA. Society of Petroleum Engineers. 2007. SPE-107041-MS. DOI: 10.2118/107041-MS
- Minzheng Jiang, Tiancai Cheng, Kangxing Dong et al. An Efficient Downhole Oil/Water-Separation System with Sucker-Rod Pump. SPE Production & Operations. 2020. Vol. 35. Iss. 3, p. 522-536. DOI: 10.2118/201234-PA
- Behrouz M., Aghajani M. Solubility of methane, ethane and propane in pure water using new binary interaction parameters. IranianJournalofOil & GasScienceandTechnology. 2015. Vol. 4. N 3, p. 51-59.
- Ferreira M.V.D. New Challenges for Predicting Annulus Pressure Variation During Transient Operations in the Pre-Salt Wells. SPE Annual Technical Conference and Exhibition, 26-29 October, 2020, Virtual. OnePetro. 2020. SPE-201498-MS. DOI: 10.2118/201498-MS
- Gala R.D., Priyamvada K. Preventing gas locking in sucker rod pumps using an actuated traveling solenoid valve. SPE Artificial Lift Conference – Americas, 21-22 May, 2013, Cartagena, Colombia. OnePetro. 2013. SPE-165039-MS. DOI: 10.2118/165039-MS
- Haiwen Wang, Sixu Zheng, Daoyong Yang. Design and Application of Multiphase Sucker-Rod Pumps in Wells with High Gas-Oil Ratios. SPE Artificial Lift Conference – Latin America and Caribbean, 27-28 May, 2015, Salvador, Bahia, Brazil. OnePetro. 2015. SPE-173963-MS. DOI: 10.2118/173963-MS
- Yongfeng Kang, Zhengchun Liu, Adolfo Gonzales, Robello Samuel. Investigating the Influence of ESP on Wellbore Temperature, Pressure, Annular Pressure Buildup, and Wellbore Integrity. SPE Deepwater Drilling and Completions Conference, 14-15 September, 2016, Galveston, Texas, USA. Society of Petroleum Engineers. 2016. SPE-180299-MS. DOI: 10.2118/180299-MS
- Kulkarni S.D., Vyas A., Gupta H. Evaluating Precision of Fluid/Casing Design for Annular Pressure Build-Up APB Application Using Machine-Learning Tools. SPE Annual Technical Conference and Exhibition held in Calgary, 30 September – 2 October, 2019, Alberta, Canada. OnePetro. 2019. SPE-196179-MS. DOI: 10.2118/196179-MS
- Mingulov S.G., Mingulov I.S. Techniques for optimization of gas extraction from production wells annulus. International Conference: Actual Issues of Mechanical Engineering (AIME 2020), 27-29 October, 2020, Saint Petersburg, Russian Federation. IOP Conference Series: Materials Science and Engineering. 2021. Vol. 1111. N 012037. DOI: 10.1088/1757-899X/1111/1/012037
- Isaev A.A., Takhautdinov R.S., Malykhin V.I., Sharifullin A.A. Oil Production Stimulation by Creating a Vacuum in the Annular Space of the Well. SPE Annual Caspian Technical Conference, 16-18 October, 2019, Baku, Azerbaijan. Society of Petroleum Engineers. 2019. SPE-198401-MS. DOI: 10.2118/198401-MS
- Strider L.K., Wyatt B.K., Hayworth Jr.D.C. Patent N 8297362 USA. Natural gas supply apparatus and method. 2012.
- Mccarthy D. Patent N 10087924 USA. Gas compressor and system and method for gas compressing. 2018.
- Mccarthy D. Patent N 10167857 USA. Gas compressor and system and method for gas compressing. 2019.
- Guoqing Han, Xiaodong Wu, He Zhang et al. The optimization approach of casing gas assisted rod pumping system. International Petroleum Technology Conference, 19-22 January, 2014, Doha, Qatar. OnePetro. 2014. IPTC-17331-MS. DOI: 10.2523/IPTC-17331-MS
- Bo Zhang, Zhichuan Guan, Nu Lu et al. Trapped annular pressure caused by thermal expansion in oil and gas wells: A review of prediction approaches, risk assessment and mitigation strategies. Journal of Petroleum Science and Engineering. 2019. Vol. 172, p. 70-82. DOI: 10.1016/j.petrol.2018.09.041
- Pai R., Gupta A., Sathuvalli U.B. et al. Validation of Transient Annular Pressure Build-Up APB Model Predictions with Field Measurements in an Offshore Well and Characterization of Uncertainty Bounds. IADC/SPE International Drilling Conference and Exhibition, 3-5 March, 2020, Galveston, Texas, USA. OnePetro. 2020. SPE-199561-MS. DOI: 10.2118/199561-MS
- Zhao Hui, Yang Feng, Wang Hai-wen Mechanism of Enhanced Production by Enforcing Gas Evacuation of Multiphase Pump. Oil Field Equipment. 2013. Iss. 2, p. 4-8.