Natural gas methane number and its influence on the gas engine working process efficiency
- 1 — Ph.D., Dr.Sci. Academician of the Russian Academy of Sciences Russian State Agrarian University named after K.A.Timiryazev ▪ Orcid
- 2 — Ph.D. Head of the Department Saint Petersburg Mining University ▪ Orcid ▪ Elibrary ▪ Scopus ▪ ResearcherID
- 3 — Ph.D., Dr.Sci. associate professor Saint Petersburg State Agrarian University ▪ Orcid
Abstract
The natural gas usage as a vehicle fuel in the mining industry is one of the priority tasks of the state. The article pays special attention to the component composition of natural gas from the point of view of its thermal efficiency during combustion in the combustion chamber of a power plant on a heavy-duty vehicle in difficult quarry conditions. For this, domestic and foreign methods for determining the main indicator characterizing the knock resistance of fuel in the combustion process – the methane number – are considered. Improvement of technical and economic indicators will be carried out by changing the composition of the gas mixture based on methane to fit the design features of the gas power plant, the methane number will be the determining indicator. A theoretical analysis of the influence of the methane number on such engine parameters as the compression ratio and the maximum speed of the flame front propagation in the second phase of combustion in the engine cylinder, expressed through the angle of rotation of the crankshaft, is presented. Based on the results of theoretical and experimental studies, the dependences of the influence of the methane number on the efficiency of the working process of the engine and its external speed characteristic were obtained.
Introduction
Thermal processes occurring in power plants of mining equipment using compressed or liquefied natural gas as a motor fuel are constantly being studied and improved. The most difficult task remains the influence of the composition of the gas-air mixture on the combustion process, which determines the efficiency, environmental friendliness and profitability of a vehicle intended for operation in difficult quarry conditions and transportation of large masses of various natural materials (stone, ore, coal, etc.) [5, 12, 13].
When studying the working process of an engine using a gas mixture based on methane, the physicochemical properties of these gases and their detonation resistance are considered. To assess fuel quality indicators, various methods are used, including those designed to determine the octane number (RON). I.I. Vibe studied the component composition of various petroleum fuels in his scientific works back in 1962, he considered the regularities of the change in the combustion rate inside the engine cylinder on the basis of the general equations of chemical reactions [9].
Research methodology
In practice, it is customary to consider the octane number of petroleum fuels according to the equation
where n – number of components in the fuel mixture; βi – the presence of the i-th component number in the fuel mixture; xi – octane number of the i-th number of components in the fuel.
The octane number of petroleum fuels shows resistance to detonation during the combustion of the working fluid in the engine cylinder. Analysis of various sources indicates that the RON for natural gas is in the range of 110-120 units, which characterizes the high quality of the fuel, expressed in terms of the lowest calorific value. It is not entirely correct to calculate the energy efficiency of natural gas by the method of oil fuels [1, 3, 6, 8]. In this case, it would be reasonable to use a method capable of determining the energy efficiency of a gaseous fuel based on its natural component origin, taking into account the theory of intermolecular interaction [7, 14].
In domestic science, the phase transformations of various gases, taking into account external temperature-dynamic effects, have been studied in depth [4, 19]. Many nomograms have been developed based on systems of gas mixtures characterizing the phase equilibria of one-, two- and multicomponent gases. Such studies made it possible to understand in detail the picture of changes in the intermolecular interaction of individual gases (methane, propane, ethane, butane, hydrogen, carbon dioxide, etc.) with each other in order to ultimately achieve the maximum thermal effect during the reaction of an oxidizer and natural gas (gas mixtures). All these scientific achievements were aimed at the development of oil and gas enterprises, but the research on the properties of gaseous fuels for transport heat engines in Russia is not enough.
Since 1960, AVL (Anstalt für Verbrennungskraftmaschinen List) has carried out experimental studies on the properties of natural gas, which is 92-98 % methane. According to experimental studies, the quality boundaries of both compressed and liquefied natural gas are determined by its heat of combustion and the value of detonation resistance during combustion. The methane number (MN) shows how many volume percent of methane (MN = 100) is contained in a mixture of methane and hydrogen (MN = 0), which begins to detonate at the same compression ratio as the test gas [10]. According to the AVL method, the optimal quality of natural gas is determined by the lower threshold of the knock resistance value (MN = 75).
Currently, there is no unified generally accepted methodology for determining MN, all existing methods are the development of individual companies. Therefore, when using different methods for determining MN, a discrepancy arises from 5 to 12 units. At present, a project GOST is being developed in Russia, which provides for a method for determining the methane number, which is based on the expression:
where RONm – motor method octane number.
The AVL method provides for the definition of RON as follows:
where х – molar fraction of components included in the gas СН4, С2Н6, С3Н8, С4Н10, СО2, N2.
As you know, MN can affect the main performance indicators of the mining equipment power plant. Let us briefly consider the known methods for determining the MN of natural gas and gas mixtures from various fields.
According to joint experimental studies of AVL and CARB (California air resources board) companies, the MN of natural gases from various fields changes due to the content of other gases in the methane composition, including inert gases, hence the relative density of such gas, due to the difference in the molar fraction of other gases (СН4, С2Н6, С3Н8, С4Н10, СО2 и N2), changes and contributes to a decrease or increase in MN. It is recommended to enter the Wobbe number, which characterizes the state of natural gas, taking into account the inert and other gases included in it.
where
In an article [18] the equation for determining the MN by the CARB method is as follows:
где Н/С – отношение количества атомов водорода к атомам углерода в углеводородных компонентах топлива.
При определении MN по методу CARB в качестве примера автор [18] использовал шахтный газ, MN шахтного газа по данному методу составило 108, по методу AVL MN = 100, расхождение в 7-8 ед. говорит о нетRONности определения MN при использовании различных методик. Метод CARB дает завышенные результаты MN, в то время как метод AVL – заниженные результаты. В связи с этим в [18] вводится уравнение, полученное методом наименьших квадратов,
where Н/С – the ratio of the hydrogen atoms number to carbon atoms in the hydrocarbon components of the fuel.
When determining the MN by the CARB method, as an example, the author [18] used mine gas, the MN of mine gas by this method was 108, by the AVL method the MN = 100, the discrepancy was 7-8 units. speaks of the inaccuracy of determining the MN when using different methods. The CARB method gives overestimated results for MN, while the AVL method gives underestimated results. In this regard, in [18] the equation obtained by the least squares method is introduced,
where MON – RON, determined by motor method.
Several methods for determining the methane number of natural gas and gas mixtures based on it are known in the world: AVL, CARB, GRI, MWM, Waukesha Knock Index, PKI MN, Cummins, Wartsila. All methods were developed to achieve efficient operation of a gas power plant or a convertible standard engine for a methane working process.
The methodology developed by CARB and KTH presents the following equation for determining the MN of natural gas:
Using the methods for determining the MN of natural gas for use as a motor fuel, it is necessary to separate the gases by MN (MN = 90, MN = 95, etc.) like gasoline fuel, which has different RONs recommended for use in the corresponding types of internal combustion engines. As a rule, in Russia, natural gas is used in power plants of trucks without studying its component composition, since it is already provided for by GOST, hence the problems of optimizing the working process of a converted gas engine when using natural gas in accordance with GOST 27577-2000 and GOST R 56021- 2014.
According to Gruzomobil (Saint Petersburg), a Cummins gas engine fueled by European natural gas delivers more effective power than a Cummins engine fueled by Russian natural gas. This indicates that in Russia there are more studies aimed at improving the design of an engine for operation on natural gas and less works on studying the physical properties of natural gas for reciprocating internal combustion engines, taking into account their design features.
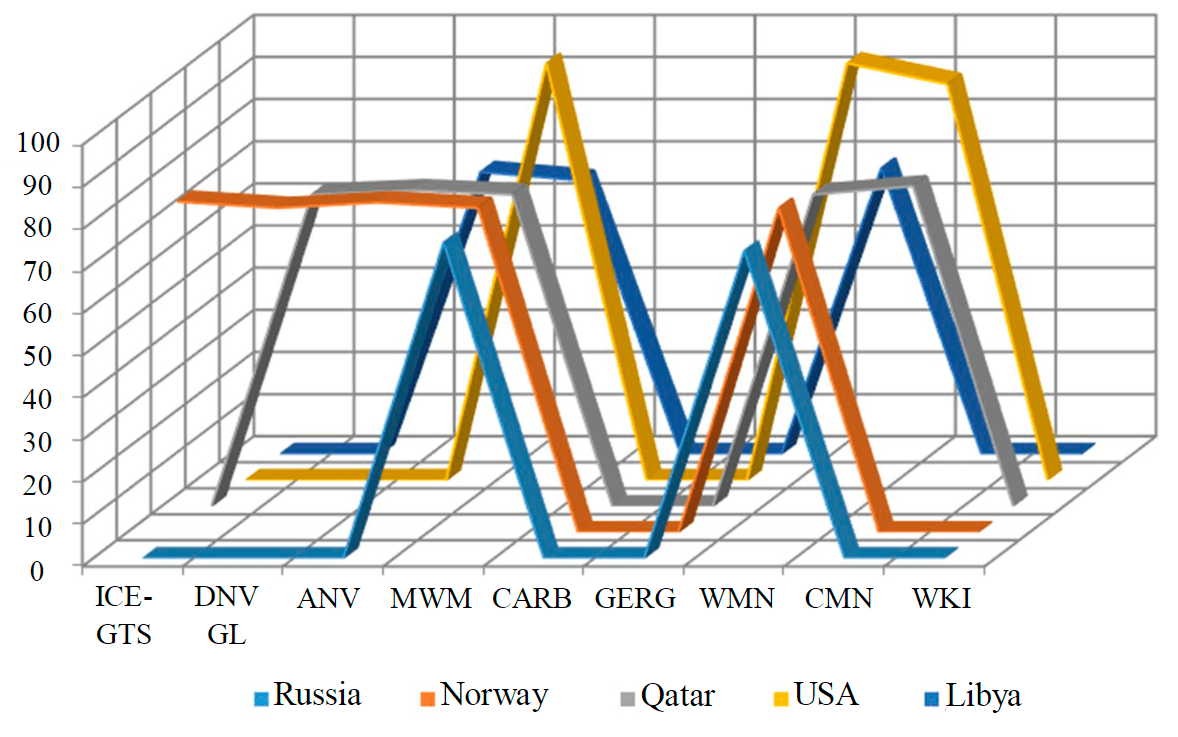
Fig.1. Comparison of methods for calculating the methane number
To reduce the imbalance between works aimed at improving the design of the engine and studying the physical properties of various gases, it is necessary to develop an algorithm for the selection and use of gases according to the MN in the corresponding piston engines. In this case, it is necessary to create artificial gas mixtures based on methane, which will increase the heat release energy due to the optimal selection of MN, while ensuring the detonation resistance of the operation of the gas power plant in a wide range of loads.
Manufacturers of gas power plants, focused mainly on freight auto transport, at the design stage, lay down certain design features, taking into account the component composition of the natural gas used [2, 21, 24, 28]. The European Union does not have large reserves of natural gas, with the exception of Norway, therefore, is supplied to Europe with natural gas from various countries (Russia, Norway, Qatar, USA, Libya, Egypt, Algeria, etc.).
According to the well-known methods for determining the MN of natural gas, studies of all natural gases supplied to Europe from different fields were carried out. The conducted studies have shown ambiguous results (Fig.1), it is known that the optimal MN > 70. Note in advance that not all the methods accurately determine MN, the most accurate is the AVL method.
From the data obtained by the AVL method, it follows that in most countries, including Russian natural gas, MN ≥ 70. However, even a high MN index is not considered a favorable gas for transport engines, which is caused by the presence of large quantities of the inert gases in the composition of natural gas (H2, N2, CO2, etc.), which reduce the efficiency of heat release during the combustion of fuel in the combustion chamber of the engine. Thus, not all natural gases with high MN can be used as the main motor fuel for gas engines, since they can cause detonation during combustion, and this additionally complicates the regulation of the air-fuel mixture in the intake system.
The use of natural gas as a motor fuel in heavy-duty vehicles operating in mining and quarrying conditions, taking into account the unfavorable environmental situation, will reduce the risk of the release of toxic components by 3-4 times [22, 26-28]. The efficiency of the task is achieved if we consider not the direct combustion of the produced gas in heat engines, but the combustion of a mixture of gases selected for the qualitative composition based on methane, which has a high heat release and is resistant to detonation. The composition of various methane gases is well illustrated by the distribution scale of MN in Fig.2.
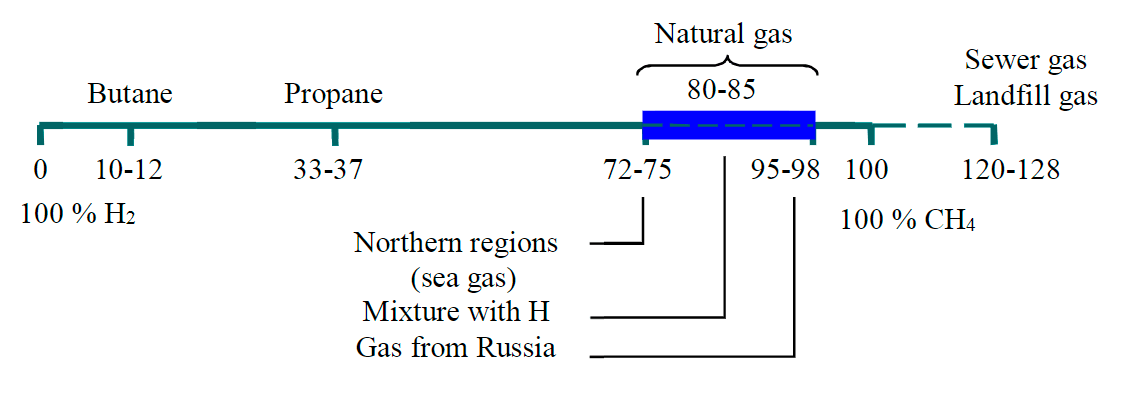
Fig.2. Scale of distribution of methane number of gases
Using the multicomponent composition of artificial gas mixtures based on methane, an algorithm of actions was developed to select gas mixtures, determine their physical properties, classify gases by methane number and work out recommendations for use in the corresponding types of engines (Fig.3).
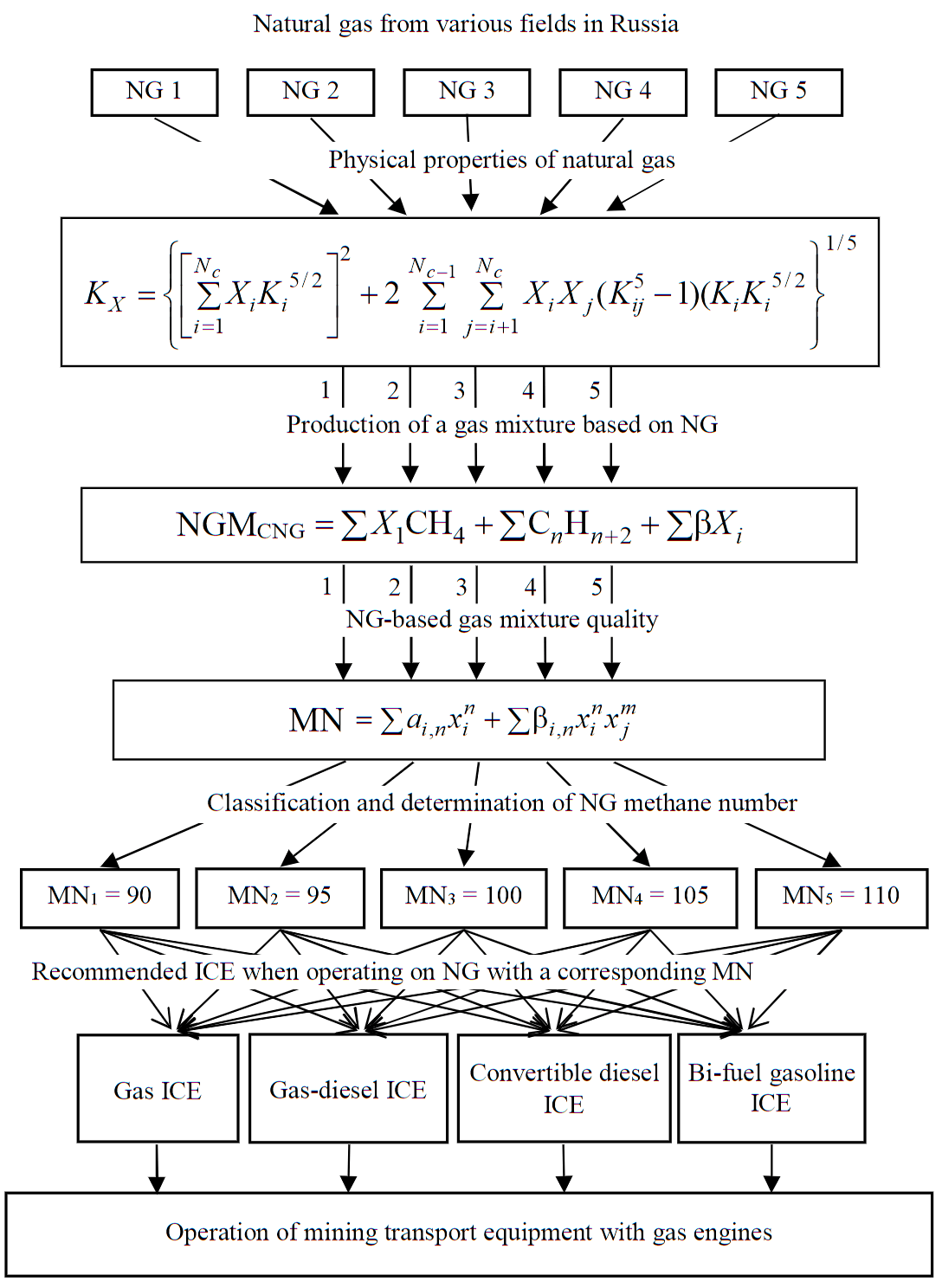
Fig.3. Algorithm for the selection and use of gas by MN in piston engines
To accomplish the set task, experimental studies of the effect of MN on the efficiency of a gas power plant designed to operate in difficult mining and quarry conditions were carried out, three gas mixtures based on natural gas were compiled component-wise (see table) [16].
Based on the gas composition given in the table, it is possible to select the qualitative composition of the gas mixture based on methane for any gas power plant of a heavy-duty vehicle without modifications and design changes [15, 16]. To confirm the reliability, conclusions and recommendations, a criterial mathematical model was developed and a multivariate regression analysis of the effect of MN on the efficiency of the working process of a gas internal combustion engine was performed.
Qualitative composition of the studied gas mixtures based on methane
Gas 1 (MN-75) |
Gas 2 (MN-98) |
Gas 3 (MN-104) |
CH4 – 83,2 |
CH4 – 89 |
CH4 – 99,7 |
C2H6 – 8 |
C2H6 – 5,2 |
C2H6 – 0,3 |
C3H8 – 3,6 |
C3H8 – 2,8 |
C3H8 – 0 |
C4H10 – 1,5 |
C4H10 – 1,1 |
C4H10 – 0 |
CO – 3 |
СО2 – 1,5 |
Н2 – 0 |
N2 – 0,7 |
N2 – 0,4 |
N2 – 0 |
Multivariate regression analysis was performed according to the technique presented in the work [25]. In sequential planning of the experiment, it is assumed that the general equation of the model [25]:
where Y – experimental observation response value; b0 – estimation of the regression coefficient; Хij – natural i-th level of j-th factor; k – number of factors to be controlled.
The most rational are the plans according to the number of experiments carried out, the main advantage of which is the proximity to D-optimal plans and the principled approach to compositional construction. Like all central compositional plans, they include the core, points and experiences in the center of the plane. All this makes it possible to obtain a relationship between the three main indicators – MN, compression ratio ε and crankshaft rotation angle φ2 [17].
The influence of the MN on the compression ratio is obvious, since the speed of propagation of the flame front changes, which can both improve and worsen the dynamics of the engine operation. In this case, the most attractive is the selection of a gas mixture based on natural gas with MN = 90-105 and a compression ratio ε = 11-14, while the optimal compression ratio is determined based on the corresponding type of engine.
To determine the reliability of theoretical studies in laboratory conditions, an experiment was carried out using a multicomponent gas mixture and natural gas as a motor fuel for a gas power plant. The tests were carried out using three investigated gas mixtures and two existing gas engine fuels used in Russia, each with a corresponding methane number.
A detailed methodology for calculating the effect of MN on the performance of a gas engine working process is presented in the work [16], based on the results of the calculation, the values from the confidence interval were compared with the corresponding values of the model parameters. Having transformed all the values according to the obtained confidence interval, the final model with the numerical values of the estimated indicators of the regression coefficients is presented
where х1 – angle of rotation of the crankshaft in the 2nd phase of combustion φ2; х2 – compression ratio ε; х3 – methane number MN.
Equation (10) made it possible to construct the dependence of the main indicators (MN, ε, φ2) influence on the power of a gas power plant when operating on the studied gas mixtures with different MN. Expression (10) determines the relationship between the MN and the engine performance, the compression ratio ε and the angle of rotation of the crankshaft φ2. In this case, additional calculations of the engine operating process are not required, since they have already been experimentally confirmed. [16]. Based on experimental data [6] the limits of the optimal values of the compression ratio ε = 12-14 and the angle of rotation of the crankshaft θ = 12-15° were determined. The resulting dependence (Fig.4) well illustrates the relationship between the MN, the compression ratio and the control characteristics of the quality of the air-gas mixture at the inlet to the engine cylinder.
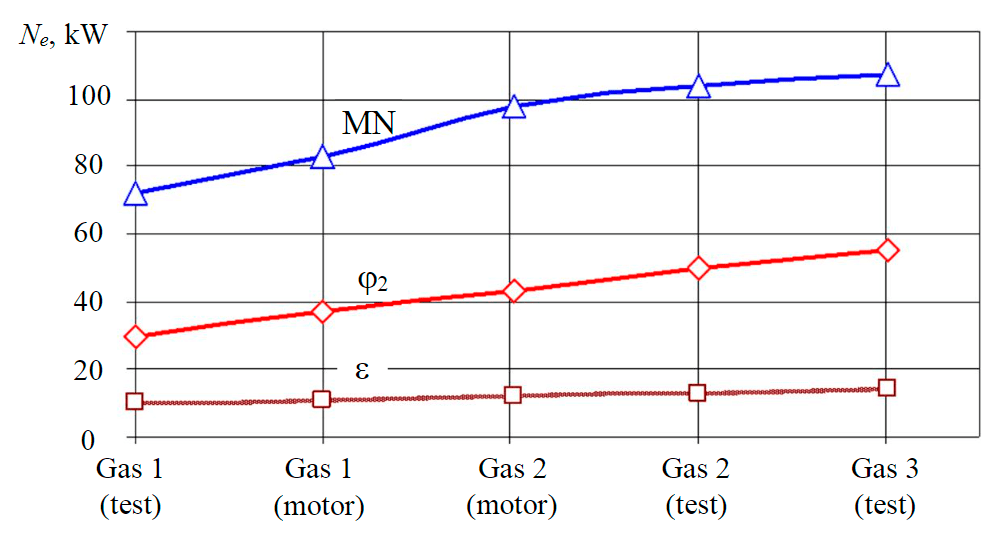
Fig.4. Dependence of the influence of the methane number of the effective working process of a gas power plant (PP); gas 1, 2, 3 (test) – test gas based on methane for PP (see table); gas 1 and 2 (motor) – natural gases used in Russia as motor gases for PP
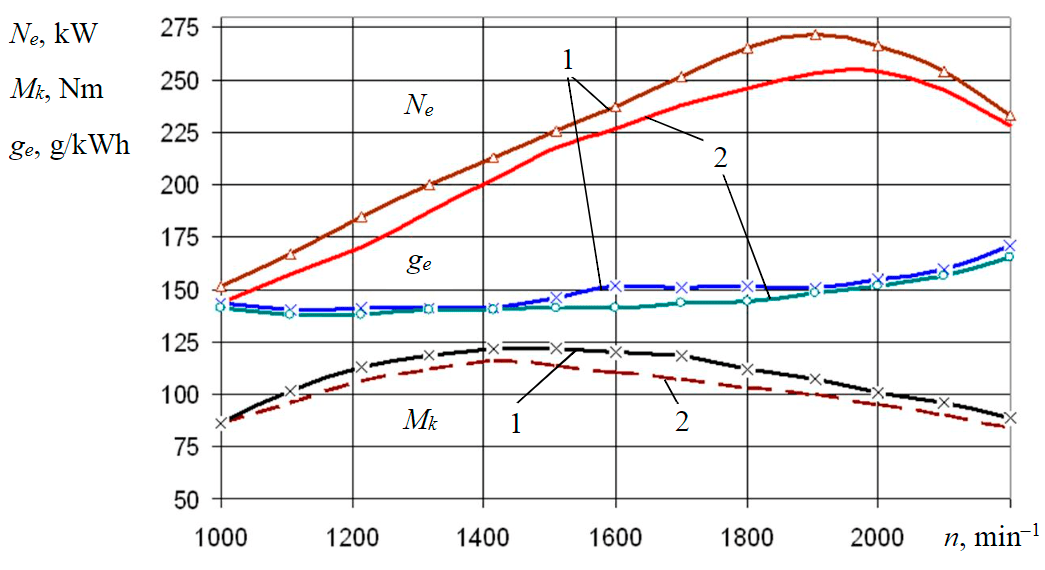
Fig.5. External speed characteristic of the gas power plant GChN12/13 of a mining dump truck 1 – investigated PP based on a mixed NG; 2 – prototyped PP based on NG
Research results
To confirm the adequacy of the developed model of the planned experiment and the influence of the methane number on the efficiency of the working process of the gas power plant of the mining heavy-duty vehicle, a numerical calculation was used, the element-by-element processing of the results obtained was carried out, and the dependence of the influence of the MN on the power indicators of the gas engine was obtained.
To confirm the theoretical study of the influence of the methane number on the engine parameters ε, φ2, which determine its energy efficiency, an experiment was carried out, an external speed characteristic was built (Fig.5) of the investigated power plant of a mining gas engine dump truck, where an increase in effective power by 10-15 % is observed.
The experimental results of the 8GChN12/13 gas PP confirmed the reliability of the theoretical studies obtained. The dependence of the relationship between the MN, the degree of compression and the angle of rotation of the crankshaft φ2 is obtained. The obtained dependence of the influence of the interconnection between the MN, the compression ratio and the maximum flame propagation speed in the engine cylinder, expressed through the angle φ2, made it possible to experimentally confirm the increase in the effective power within 10-15 %. A change in the methane number of natural gas MN = 75-97 with a compression ratio ε = 12 and an ignition advance angle θ = 12-15°, an increase in power, compression ratio up to ε = 14 while maintaining MN = 75-97 reduce the detonation resistance. It is recommended to increase the MN to 98-104 at θ = 12°.
The developed algorithm for adaptation and use of multicomponent gas mixtures made it possible, on the basis of experimental and calculated data, to qualify the gas according to MN = 75-104. The most suitable composition of natural gas as a motor gas in 8GChN12/13 PP is gas 2 with MN = 98 (composition: CH4 – 89; C2H6 – 5.2; C3H8 – 2.8; C4H10 – 1.1; СО2 – 1.5; N2 – 0.4 mol.%) and gas 3 with MN = 104 (CH4 – 99.7; C2H6 – 0.2; Н2 – 0.1 mol. %), at the same time, there is an increase in the effective power by 10-15 %.
Conclusion
The most optimal methane number of gas mixtures is MN = 97 with a compression ratio of ε = 12 and an angle ε2 = 18°, while an increase in the effective power by 10-15 % is observed. With a compression ratio of e = 13 the power of the PP increases by 17-22 % when using a gas mixture and natural gas with MN = 104. The developed algorithm allows selecting gas mixtures for the corresponding types of PP for mining equipment without complex design changes. The experiment showed that the most suitable gas mixtures for a 8GChN12/13 gas PP of a mining dump truck are gas 2 with MN = 98 and gas 3 with MN = 104.
References
- Afanasyev A.S., Khakimov R.T., Zagorski S.M. Effect of smoke on the use of diesel exhaust gases. Tekhniko-tekhnologicheskie problemy servisa. 2014. N 2 (28), p. 56-58 (in Russian). URL
- Barsuk N.E., Khaidina M.P., Khan S.A. "Green" gas in the gas transmission system of Europe. Gazovaya promyshlennos. 2018. N 10, p. 104-109 (in Russian). URL
- Abramchuk F., Kabanov O., Maamri R. et al. Choosing of performance parameters of light-duty engine running on natural gas and hydrogen mixture. Avtomobilnyi transport. 2011. N 29, p. 152-159 (in Russian). URL
- Gnedova L.A., Gritsenko K.A., Lapushkin N.A. et al. Gas engine fuels based on methane. Analysis of requirements for quality and raw materials. Vesti gazovoi nauki. Sovremennye tekhnologii pererabotki i ispolzovaniya gaza. 2015. N 1 (21), p. 86-97 (in Russian). URL
- Gilyaziev M.G. Taking into account the combined effect of pressure losses in the inlet and exhaust ducts of gas-pumping units on the power and fuel gas consumption of a gas turbine unit. Novye napravleniya innovatsionnoi deyatelnosti na predpriyatiyakh gazovoi promyshlennosti. Мoscow: Konvert, 2018, p. 175-184 (in Russian).
- Didmanidze O.N., Afanasev A.S., Khakimov R.T. Research of heat generation indicators of gas engines. Journal of Mining Institute. 2018. Vol. 229, p. 50-55. DOI: 10.25515/PMI.2018.1.50
- Zhou D., Kasas-Baskes Kh., Lebon Dzh. Extended irreversible thermodynamics. Izhevsk: Nauchno-issledovatelskii tsentr “Regulyarnaya i khaoticheskaya dinamika”, Institut kompyuternykh issledovanii, 2006, p. 528 (in Russian).
- Zaichenko V.M., Maikov I.LPyrolysis of hydrocarbons on carbon matrices. Мoscow: Nedra, 2014 , p. 235 (in Russian).
- Ivanov S.S., Tarasov M.Yu. Requirements for the associated gas treatment for gas-cylinder engines supply. Oil Industry. 2011. N 1, p. 102-105 (in Russian). URL
- Ilina M.N. Requirements for the preparation of associated petroleum gas for small-scale power generation. Izvestiya Tomskogo politekhnicheskogo universiteta. Energetika. 2007. Vol. 310. N 2 , p. 167-171 (in Russian). URL
- Gnedova L.A., Gritsenko K.A., Lapushkin N.A. et al. Evaluation of CNG knock resistance. Alternative Fuel Transport. 2011. N 5 (23), p. 53-56 (in Russian). URL
- Kantyukov R.A., Tameev I.M., Zimnyakov S.A. et al. Development of technology for deep pumping of gas from the repaired sections of main gas pipelines using a mobile compressor station. Nauka i tekhnika v gazovoi promyshlennosti. 2011. N 1 (45), p. 49-52 (in Russian). URL
- Tarasov M.Yu., Ivanov S.S. Preparation of petroleum gas to power gas piston power plants. Neftyanoe khozyaistvo. 2009.Kantyukov R.R., Tahaviev M.S., Gilyaziev M.G. et al. The development of mathematical model of gaz-transport system area. Transport and storage of oil products and hydrocarbons. 2015. N 2, p. 3-7 (in Russian). URL
- N 2, p. 46-49 (in Russian). URL
- Gumerov A.G., Bazhaikin S.G., Ilyasova E.Z., Avdeeva L.A. Utilization of associated petroleum gas in the fields. Ufa: Institut problem transporta energoresursov Respubliki Bashkortostan, 2010, p. 111 (in Russian).
- Khakimov R.T., Didmanidze O.N., Sheremeteva M.I. Methods for determining the methane number of the component composition of natural gas Sbornik trudov III Vserossiiskoi (s mezhdunarodnym uchastiem) nauchno-prakticheskoi konferentsii nauchnykh, nauchno-pedagogicheskikh rabotnikov, aspirantov i studentov “Sovremennye transportnye tekhnologii: zadachi, problemy, resheniya”, 22 March2019, Chelyabinsk, Rossiya. Yuzhno-Uralskii institut upravleniya i ekonomiki, 2019, p. 74-84 (in Russian).
- Khakimov P.T. Influence of burnout characteristics on the performance of a gas engine operating cycle when using an electronic control system. Gruzovik. 2008. N 4, p. 27-29 (in Russian). URL
- Pylev V.A., Prohorenko A.A., Kravchenko S.A. et al. Mine gas – motor fuel for explosion engines. Internal Combustion Engines. 2007. N 1, p. 10-15 (in Russian).http://mystukr.mari.kiev.ua/index.php/0419-8719/article/view/78879
- Anthropogenic and natural radiative forcing. Climate Change 2013: The Physical Science Basis. Contribution of Working Group I to the Fifth Assessment Report of the Intergovernmental Panel on Climate Change. Cambridge University Press, 2013, p. 659-740. DOI: 10.1017/CBO9781107415324
- Backman M., Rogulska M. Biomethane use in Sweden. The Archives of Automotive Engineering. 2016. Vol. 71. N1, p. 7-19. DOI: 10.14669/AM.VOL71.ART1
- Billig E., Thraen D. Renewable methane – A technology evaluation by multi-criteria decision making from a European perspective. Energy. 2017. Vol. 139, p. 468-484. DOI: 10.1016/j.energy.2017.07.164
- Nehrkorn T., Eluszkiewicz J., Wofsy S.C. et al. Coupled weather research and forecasting-stochastic timeinverted lagrangian transport (WRF-STILT) model. Meteorol Atmos Phys. 2010. Vol. 107, p. 51-64. DOI: 10.1007/s00703-010-0068-x
- Kagan L., Valiev D., Liberman M. et al. Effects of hydraulic resistance and heat losses on deflagration-to-detonation transition. Pulsed and continuous detonation. 2010, p. 108-112.
- Fallde M., Eklund M. Towards a sustainable socio-technical system of biogas for transport: the case of the city of Linköping in Sweden. Journal of Cleaner Production. 2015. Vol. 98, p. 17-28. DOI: 10.1016/j.jclepro.2014.05.089
- Malenshek M., Olsen D.B. Methane number testing of alternative gaseous fuels. Fuel. 2009. Vol. 88, p. 650-656. DOI: 10.1016/j.fuel.2008.08.020
- Artemov V.I., Borovskih O.V., Lazarev D.O. et al. Mathematical Model and 3D Numerical Simulation of Heat and Mass Transfer in Metal-hydride Reactors. 17th World Hydrogen Energy Conference (WHEC 2008), 15-19 June 2008. Brisbane, Australia. WHEC, 2008, p. 628-631.
- Peischl J., Ryerson T.B., Brioude J. et al. Quantifying sources of methane using light alkanes in the Los Angeles basin, California. Journal of Geophysical Research: Atmospheres. 2013. Vol. 118. Iss. 10, p. 4974-4990. DOI: 10.1002/jgrd.50413
- Lee S.W., Baik Doo-Sung, Rogers T., Petersen P. Study on Performance and Exhaust Gas Characteristics of Directly Injected CNG Engine. International Journal of Bio-Science and Bio-Technology. 2014. Vol. 6. N 2, p. 179-186. DOI: 10.14257/ijbsbt.2014.6.2.18
- Schart J., Kovacs A. ERGaR: Tool for Cross Border Transfer and Mass Balancing Biomethane within the European Natural Gas Network. Workshop: Biomethane in the Natural Gas Network. Brussels, 2017. URL (дата обращения: 17.11.2021).