Justification on the safe exploitation of closed coal warehouse by gas factor
- 1 — Ph.D., Dr.Sci. Head of Department Empress Catherine II Saint Petersburg Mining University ▪ Orcid ▪ Elibrary ▪ Scopus ▪ ResearcherID
- 2 — Postgraduate Student Empress Catherine II Saint Petersburg Mining University ▪ Orcid ▪ Elibrary ▪ Scopus
- 3 — Ph.D. Associate Professor Empress Catherine II Saint Petersburg Mining University ▪ Orcid ▪ Elibrary
Abstract
The annual increase of coal production and its demand lead to the necessity in temporary storage places (warehouses) organization to accommodate raw coal materials before the shipment. It is noted that at the open method of coal storing the dust emission from loading/unloading operations and from the pile surface effects negatively the health of the warehouse workers and adjacent territories. An alternative solution is closed-type warehouses . One of the main hazards of such coal storage can be the release of residual methane from coal segregates into the air after degassing processes during mining and extraction to the surface, as well as transportation to the place of temporary storage. The study carries the analysis of methane content change in coal during the processes of extraction, transportation and storage. Physical and chemical bases of mass transfer during the interaction between gas-saturated coal mass and air are studied. It is determined that the intensity of methane emission depends on: the coal seam natural gas content, parameters of mass transfer between coal, and air and the ambient temperature. The dynamics of coal mass gas exchange with atmospheric air is evaluated by approximate approach, which is based on two interrelated iterations. The first one considers the formation of methane concentration fields in the air space of the bulk volume and the second accounts the methane emission from the pile surface to the outside air. It is determined that safety of closed coal warehouses exploitation by gas factor can be ensured by means of artificial ventilation providing volumetric methane concentration in the air less than 1 %. The flow rate sufficient to achieve this methane concentration was obtained as a result of computer modeling of methane concentration fields formation in the air medium at theoretically calculated methane emission from the pile surface.
Introduction
The coal industry is one of the key sectors of Russian industry, including mining, mining, production, transportation and marketing of coal. The Russian Federation ranks 2nd place in the world in terms of coal reserves, most of which are located in Siberia and the Far East [1-3]. Coal production in 2023 amounted to 438 million t, including 213 million t of coal exported to China, BRICS countries and others [4, 5].
The growth of coal production and increased commercial interest in its acquisition lead to the need for coal’s temporary storages in port terminals and storage areas to be shipped to the consumer in future [6-8].
Nowadays, the most common method of temporary coal storage is its placement in open area [9-11]. However, data analysis shows, this method, firstly, reduces the raw materials quality, and secondly, there is an aerotechnogenic load on the environment and workforce due to the dust emissions [12-14]. The cause of coal’s piles high dusting is wind flow, the value of which reaches 20 m/s in some regions. Calculation of coal dust emissions into the atmosphere from the open warehouses operations was carried out according to the Industry methodology. Dependence of atmospheric air dustiness (points at different distances from the piles) on the wind speed is presented in Fig.1.
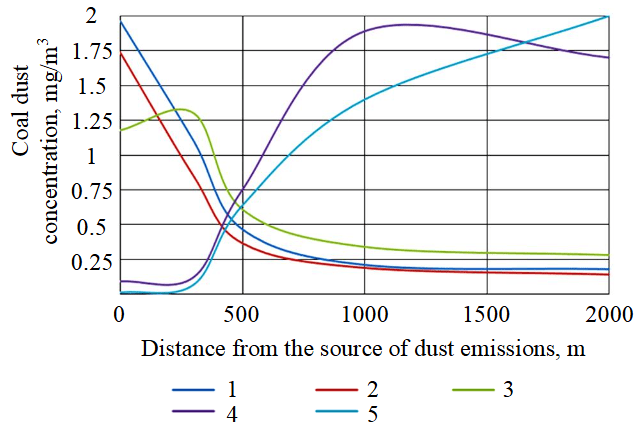
Fig.1. Dependence of coal dust dispersion on the distance to the dust source at different air velocities
1 – 1 m/s; 2 – 3 m/s; 3 – 5 m/s; 4 – 10 m/s; 5 – 20 m/s
The obtained analysis indicate that at low wind speed (1-5 m/s) removal of coal dust from the pile surface and its dispersion occurs mainly in the storage area and the adjacent territory of the enterprise, exposing the negative impact of the workforce. Due to wind flow increasing, dusting from the pile intensifies, but at the same time, dispersion and settling of coal dust will occur mainly outside the industrial territory, inducing aerotechnogenic impact on the environment.
An alternative solution for the environmental problem and prevention of coal quality reduction is the exploitation of temporary closed warehouses [15-17]. Following this method, the air velocity is determined only by the parameters of natural or forced ventilation. In comparison with the wind flow velocity, the air inside closed warehouse moves less faster which contributes to minimal dust disruption from the surface of coal pile. Meanwhile, the high residual methane content in coal segregates can lead to the formation of explosive concentrations in the warehouse air environment [18]. In order to avoid such cases, the maximum methane content in the closed warehouse air is limited by the current regulations (1 % of the volume) [19].
The obtained analysis indicate that many Russian coal deposits are characterized by high natural gas content. This information is presented in the Table, which contains the values of minimum and maximum natural methane content of some Kuzbass coal seams [20-22].
Methane content of some Kuzbass coal seams
Coal deposit |
Minimum natural methane content, m3/t |
Maximum natural methane content, m3/t |
Alardinskoye |
0,10 |
44,7 |
Baidayevskoye |
0 |
30,3 |
Berezovo-Biryulinskoye |
2,0 |
36,7 |
Egozovo-Krasnoyarskoye |
0,3 |
13,5 |
Kedrovsko-Krokhalevskoye |
0,01 |
17,6 |
Kiselevskoye |
0 |
35 |
Leninskoye |
0 |
25 |
Olzherasskoye |
1 |
31 |
Raspadskoye |
1 |
22 |
Sokolovskoye |
2 |
17,1 |
Shelkanskoye |
1 |
23,5 |
Chertinskoye |
0 |
35 |
Reduction of gas content (methane content) relative to its initial value takes place in several stages: coal extraction to the surface, loading into railcars, transportation by rail, unloading to the warehouse. The coal mass degassing processes at each stage depend on such parameters as petrographic coal composition, ash content, average size of a coal piece, ambient temperature, etc. [23-25].
Hence, the purpose of the work is to study the influence of residual gas content of coal on closed coal warehouse’s safe operating conditions and to choose the ventilation mode for gas regime normalization when required.
Methods
Methane in coal seams is in a bound sorbed state. During the coal massif distructure, a part of methane, located in seam pores and cracks, escapes into the mine space instantly, and the other part of gas – continue to desorb, but at a slower rate. The intensity of gas emissions from coal depends on several factors: ambient temperature, mass transfer parameters, initial gas coal content, etc. [26-28].
After mining and extraction to the surface and during transportation to the customer, coal is stored in various shapes of piles, which are bulk volumes containing coal pieces surrounded by air space. Piles may include coal mounds before loading into railcars, coal volumes filling in railway, and coal mounds formed in temporary storage places (port terminals, storage facilities at thermal power plants, chemical and metallurgical plants, etc.) [19, 29, 30].
If during the coal transportation from the extraction place to the final destination, degassing of initially contained in the coal methane occur only partially, the coal mass have some residual gas content.
The degassing process of coal stored in piles is carried out as follows. In the initial period, immediately after the pile formation, the methane concentration in the air, that filing intergranular voids is 0. Over time, the air voids begin to be filled with methane coming from the surface of each coal unit, and the methane concentration in the air voids continuously increases, reaching a certain asymptotic value. If the a coal pile surface is washed by air, then simultaneously with the increasing of methane concentration in the depths of the pile, an outflow of methane from the surface occurs, and in the adjacent to the pile surface zones, zones are formed with a reduced concentration of methane relative to the areas to which the influence of gas exchange from the surface has not yet spread.
It may appear that firstly the methane flow from the pile surface increases to a certain value, but then it decreases monotonously.
To estimate the dynamics of coal mass gas exchange with atmospheric air at all stages of its storage in piles or railcars, an approximate approach based on the use of two interrelated iterations determining both the formation of methane concentration fields in the air space of the bulk volume and the values of methane emission from the pile surface to the outside air is proposed.
The first iteration is performed under the assumption that methane emission per unit volume of the coal mass, where the coal particles surface area is Fvol, occurs under the condition of isolation of this volume, in which the coal particles and voids filled with air occupy volumes 1 – m and m, respectively, from the outside air surrounding its surface. The methane flow j from the each coal particle surface is calculated under the assumption that it can be represented in the form of a ball with radius r0, and the methane flow from its surface is determined by the effective diffusion coefficient Deff, the value of which was established on the basis of experimental studies (Fig.2) for Kuzbass coals ranks gas and fat (bituminous) coal [31].
The graph in the Fig.2 shows the increasing of effective diffusion coefficient with ambient temperature raising.
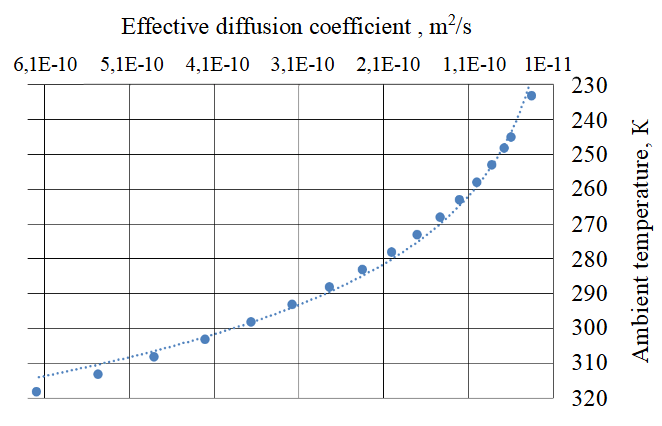
Fig 2. Graph of the change in effective diffusion coefficient from ambient temperature.
Correlation ratio √0.993
The differential equation of methane diffusion in each coal particle can be represented in the form:
where С – methane concentration in coal particles, kg/m3; Focoal – Fourier diffusion number for coal, m2/s, Focoal=Deffτ/r02; τ – time, s; C0 – initial methane concentration in coal mass, kg/m3; R – dimensionless radius, R = r/r0; r0 – average radius of coal particles of bulk coal volume, m; Cτ – methane concentration in the air, kg/m3.
The solution of equation (1), presented in a general form, at Cτ = const, has a complex form, which complicates its further use for calculating the methane concentration dynamics in air voids. Therefore, the asymptotic representation of the general solution at actual values of the Fourier diffusion number for the considered conditions was used to determine C(R, Focoal):
Then the diffusion flow for these Fourier number with high accuracy can be represented as:
In case Cτ varies according to an arbitrary law, the methane flow is established on the Duhamel's theorem:
Methane concentration changes in per unit volume of bulk airspace:
Initial conditions
The solution of equation (5), using the Laplace transform, with respect to the methane concentration in the air space can be represented in the form:
where f(Z) – tabulated function,
– probability integral at ; Fvol – total surface of coal particles in unit of coal mass bulk volume, m2/m3; m – the relative volume occupied by air per unit bulk volume of coal mass, unit fraction.
Initial methane concentration in coal mass is related to gas content (methane content) by the following:
where ρmet – methane density, kg/m3; ρcoal – coal density, m3/t; Xmet – initial methane concentration, m3/t.
One way to calculate the average methane concentration in the bulk volume airspace over the storage or transportation time Focoal is to integrate expression (6) with subsequent averaging over time Focoal. However, in this case, the expression turns out to be rather cumbersome, that is not effective for further estimates.
Another way to calculate is to use the balance equation, based on total methane flow calculation, that enters to the bulk volume airspace from the coal particles during the time . This is possible under the condition of the average methane concentration constancy for this time and with the subsequent calculation of the increment of the average methane concentration in the air space relative to the initial value equal to zero. The dependence can be presented as:
Calculations of for various diffusion coefficients are shown in Fig.3.
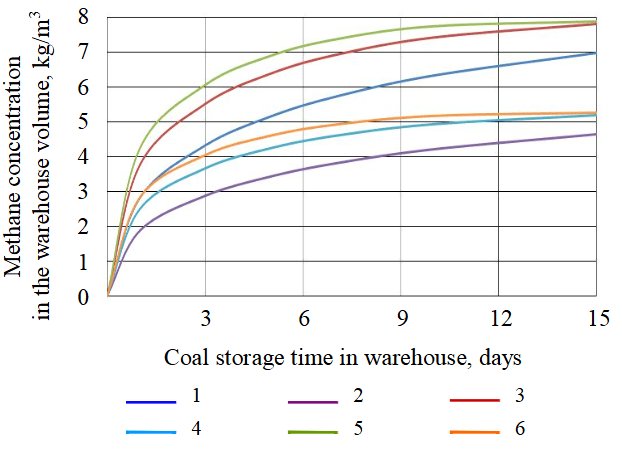
Fig.3. Graph of methane concentration changes in the airspace of the bulk volume over time
1 – residual methane content after transportation 15 m3/t (–20 °С); 2 – 15 m3/t (0 °С); 3 – 15 m3/t (+10 °С); 4 – 10 m3/t (–20 °С); 5 – 10 m3/t (0 °С); 6 – 10 m3/t (+10 °С)
According to calculations, the average methane concentration sharply increases after 1 day of storage. This raising of concentration is especially noticeable for high ambient temperatures, as the value of the effective diffusion coefficient increases with increasing temperature [32, 33]. For example, at residual methane content after transportation of 10 m3/t and duration of coal storage in the warehouse for 15 days, the methane concentration in the warehouse volume will tentatively be 8 kg/m3 in summer and 7 kg/m3 in winter period.
The second iteration allows to establish the methane emissions from the surface of the bulk volume into the air surrounded this surface at each time instant τ. To determine methane emissions from the bulk volume surface, it is necessary to choose its geometric shape. For the bulk volume of the railcars, it is proposed to use the semi-constrained space model, and for the bulk volume in the form of a pile, placed in a closed warehouse, the geometric model of an unconstrained cylinder with an equivalent radius Rsurf.
In this case, it is assumed that the methane flow from the surface of the bulk volume for the time τ is determined by the resulting effective diffusion coefficient Dreseff calculated according to the methodology similar to this work [34]. This methodology is based on the assumption that the coal mass can be considered as two binary mixtures, one of which consists of coal particles surrounded by voids containing air Deff1, and the other, on the contrary, of voids with air, which are surrounded by coal particles Deff2 The effective diffusion coefficient is calculated using the formulas:
where – effective diffusion coefficient of methane in coal according to the graph in Fig. 2, m2/s; – effective diffusion coefficient of methane in the air, = 2 · 10–5 m2/s [35-37]; vcoal – relative volume of coal particles, vcoal = 1 – m; vair – relative air space volume corresponding to the porosity of the bulk volume, vair = m.
Based on formulas (9) and (10) the resulting effective diffusion coefficient of methane is:
The methane emissions (methane flow) from the railcar’s surface and from the pile surface are:
where Fopile – Fourier diffusion number for coal pile; Cair – methane concentration in the air, kg/m3.
The total methane amount entering the air from the railcar bulk volume of coal is based on dependence (12). Furthermore, the total methane amount entering the air surrounding the open coal surface is determined firstly, and then – the residual gas content (methane content) of coal mass for the transportation time τtransp by next formula:
where Frailsurf – railcar open surface area, m2; Рrail – railcar load capacity, t.
Based on the results, the residual methane content of coal mass, loaded into the stockpile, can be from 14 to 7 m3/t depending on the ambient temperature and transportation time.
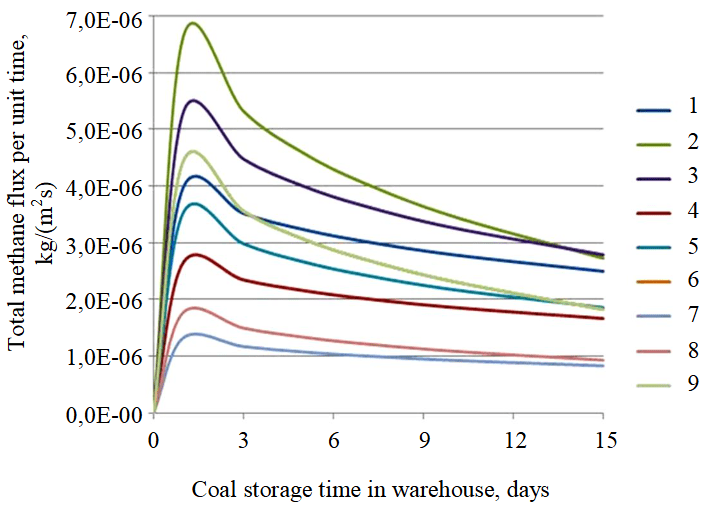
Fig. 4. Dependence of methane flow from the open coal pile surface on its storage time
1 – 15 m3/t (–20 °С); 2 – 15 m3/t (+10 °С); 3 – 15 m3/t (0 °С); 4 – 10 m3/t (–20 °С); 5 – 10 m3/t (0 °С); 6 – 10 m3/t (+10 °С); 7 – 5 m3/t (–20 °С); 8 – 5 m3/t (+10 °С); 9 – 5 m3/t (0 °С)
According to the formula (9), a dependence graph of methane flow from the open coal pile surface during its storage time was plotted (Fig.4). The initial methane concentration in the coal pile C0 was calculated with the residual methane content calculated by formula (14).
The methane concentration’s change in the closed warehouse, assuming a plane-parallel character of air movement along the pile surface, is determined by the following expression:
where Сtl – threshold level value, Сtl = 1 %; Р – pile lateral side perimeter, m2; L – pile length, m;
Based on formulas (13) and (15) the air flow rate vary from 5 to 27 m3/s in winter (average ambient temperature –20 °C) and from 11 to 45 m3/s in summer (average ambient temperature +20 °C) for different residual coal methane content before loading into the stockpile. The maximum air flow rate corresponds to the maximum value of methane flow.
Mathematical modelling of methane flow aerodynamics in the volume of a closed warehouse
The mathematical modelling of the gas transfer was carried out, taking into account the air flow aerodynamics where methane enters from the pile surface. It was accomplished to verify the validity of using a plane-parallel scheme of air movement in the coal warehouse and to establish the distribution of methane concentrations along the length of the warehouse, The Flow Vision software package was used for this purpose. The real geometry of the warehouse and the coal pile (height 23 m, width on the base 65 m and length 600 m) were taken as initial data. In addition, the mathematical model considered the influence of the loading and unloading equipment inside the warehouse (portal reclaimer) on the airflow aerodynamics.
Calculations is carried out for the residual methane content before loading to the stockpile, equal to 15 m3/t and at ambient temperature +20 °C. For these conditions, the average methane flow from the coal pile surface is 6-10–6 kg/(m2∙s).
The warehouse ventilation is carried out according to the fresh air supply scheme through openings located on the lateral side at the end of warehouse, and exhaust of polluted air through a centre pipe (Fig.5).
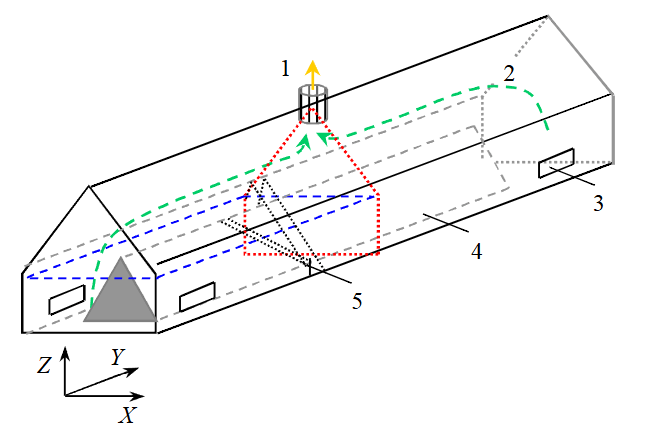
Fig.5. Scheme of a closed coal warehouse
1 – air exhaust pipe; 2 – airflow; 3 – open for air supply; 4 – coal pile; 5 – portal reclaimer
Due to the symmetry of the closed warehouse with respect to vertical planes passing perpendicular (in the central section of the warehouse) and parallel (along the length of the warehouse perpendicular to its base) to the horizontal axis, the calculation area was divided into 4 equal parts (Fig.5). All calculations were performed for one of these parts.
Gas admixture transfer process modelling was carried out for 16 h until the methane concentration stabilized. To establish the regularities of methane concentration distribution over the warehouse volume, the points 0-10 were chosen, located every 30 m from the air exhaust pipe towards the air supply openings (Fig.6).
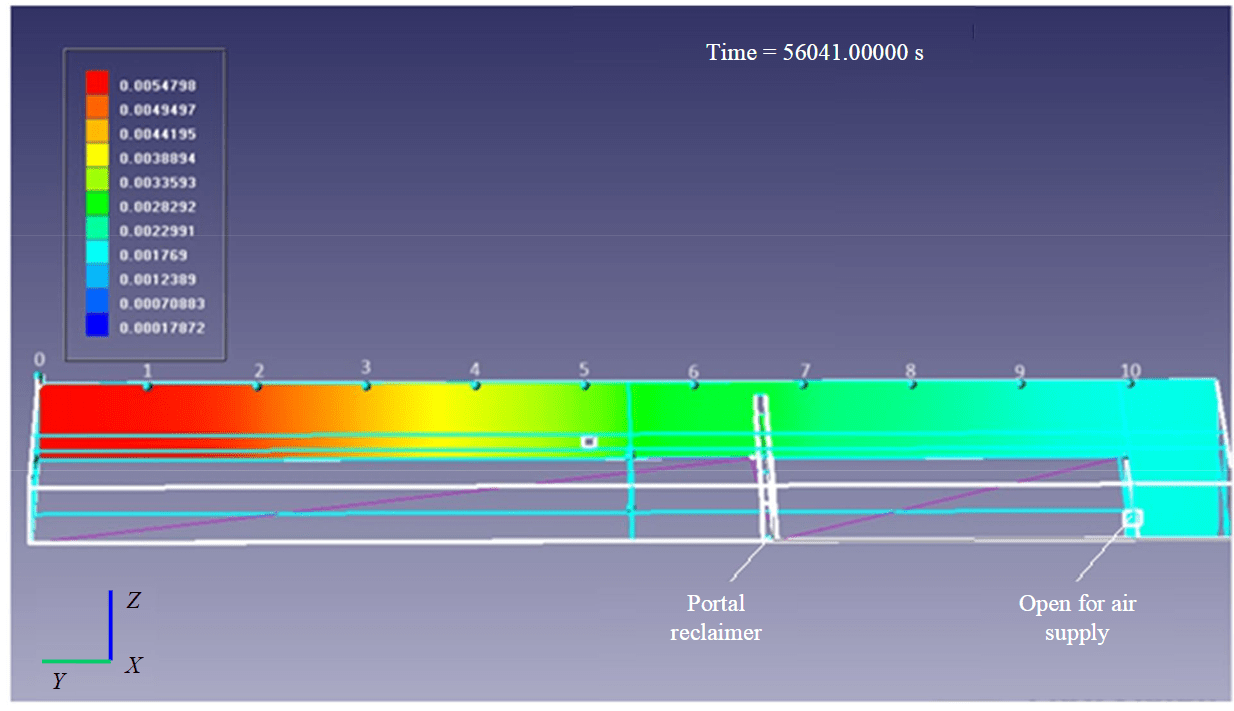
Fig.6. Methane mass fractions and location of measurement points
Resulting from the turbulent nature of air mass flow and the influence of loading and unloading equipment on the trajectory of their movement, the methane concentrations distribution over the volume of the closed warehouse will look as follows. The methane concentration takes the maximum value of 0.00547-0.99 % by volume (red colour) near the air exhaust pipe (Fig.6). This is explained by the post-penetration accumulation of methane during the rotational movement of air mass over the coal surface, emitting methane.
The methane concentration changes with time at different space points of a closed warehouse is presented in Fig.7. According to this figure, the growth of methane concentration up to 0.99 % occurs before it reaches quasi-stationary values within ~45000 s, which corresponds to 12.5 h of coal storage in the warehouse.
Thus, according the influence of complex flow aerodynamics, the air flow rate providing dilution of methane concentration up to threshold values by calculations is 48 m3/s. Satisfactory coincidence of the analytical calculations with the results of mathematical modelling (the discrepancy does not exceed 6 %), confirms the validity of the assumptions used in the mathematical model development.
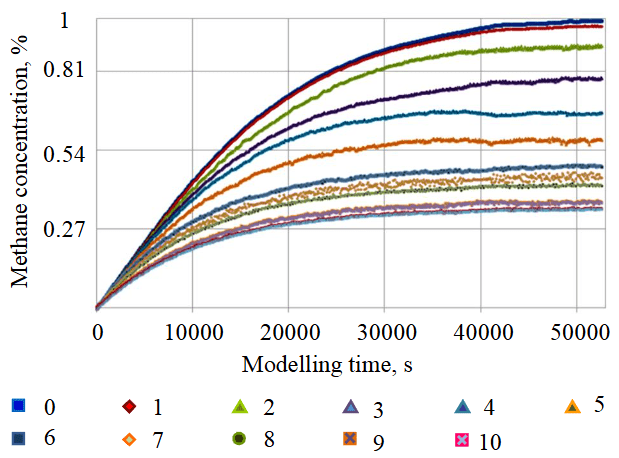
Fig.7. The graph of methane concentration changes with time at different space points of a closed warehouse
Conclusion
Coal placement in closed warehouses is an actual method of mineral temporary storage with the possibility to limit the climatic conditions impact on it, as well as to reduce the aerotechnogenic impact on the environment.
The high natural gas content of some coal seams can lead to explosive situations during mining, transport and temporary storage of raw materials due to the limited gas volume (mine, railcars, warehouse). In this regard, the ensuring safe operation problems of closed warehouses will be related to the released methane gas.
The information about the residual methane content of coal is important to prevent explosive situations at each stage:
- after coal mining, its extraction to the surface and temporary storage before loading into railcars;
- during transportation before coal placement in closed warehouses;
- during direct coal storage.
Despite reduction of residual gas coal content after each stage of handling and transportation, it can reaches values at which the operation of closed coal storage becomes unsafe, due to the possibility of exceeding the maximum permissible value of methane concentration in the air, taken as 1 % by volume.
The safety of closed coal warehouse operation in terms of methane factor will depend not only on residual methane content, but also on the ambient temperature, which directly affects the rate of methane release from coal particles: then higher the temperature, the more intense the methane release process is.
The reduction of methane content in summer (average ambient temperature +20 °C) is higher than in winter (average ambient temperature –20 °C) by almost 2 times. Thus, the accumulation of methane concentration in the closed warehouse volume in summer period will be faster, which requires more intensive ventilation for this period.
The analytical calculations results of the required air flow rate do not take into account the complex aero-dynamics of the air flow in contrast to mathematical modelling. Satisfactory coincidence of the obtained data confirms the validity of the assumptions used in the development of the mathematical model.
References
- Kornev A.V., Spitsyn A.A., Zaimentseva L.A., Zubko M.V. Research of the physico-chemical properties of hydrogel as a means of dust-explosion protection and dust reduction in coal mines. Mining Informational and Analytical Bulletin. N 9-1, p. 180-198 (in Russian). DOI: 10.25018/0236_1493_2023_91_0_180
- Overland I., Loginova J. The Russian coal industry in an uncertain world: Finally pivoting to Asia? Energy Research & Social Science. 2023. Vol. 102. N 103150. DOI: 10.1016/j.erss.2023.103150
- Korshunov G.I., Safina A.M., Karimov A.M. Research and Analysis of the Sources of Emission of Respirable Fraction of Dust at the Coal Mines. Occupational Safety in Industry. N 10, p. 65-70 (in Russian). DOI: 10.24000/0409-2961-2021-10-65-70
- Novak A. Fuel and energy Complex of Russia today and tomorrow: results and objectives. Energeticheskaya politika. 2024. N 1 (192), p. 6-13 (in Russian). DOI: 10.46920/2409 5516_2024_1192_6
- Magomet R.D., Rodionov V.A., Soloviov V.B. Methodological Approach to Issue of Researching Dust-Explosion Protection of Mine Workings of Coal Mines. International Journal of Civil Engineering and Technology. 2019. Vol. 10. Iss. 2, p. 1154-1161.
- Rush E.A., Vlasova N.V. Promising measures aimed at environmental protection when working with coal at the production sites of the terminal and storage complex. Modern Technologies. System Analysis. Modeling. N 2 (78), p. 20-32 (in Russian). DOI: 10.26731/1813-9108.2023.2(78).20-32
- Golovina E.I., Grebneva А.V. Management of groundwater resources in transboundary territories (on the example of the Russian Federation and the Republic of Estonia). JournalofMiningInstitute. Vol. 252, p. 788-800. DOI:10.31897/PMI.2021.6.2
- May Chan Myae Zaw, Alpatova P.N. Ambiguity in the Russian terminology of the energy industry. XLinguae. 2023. Vol. 16. Iss. 1, p. 60-69. DOI: 10.18355/XL.2023.16.01.05
- Mazurenko O., Rusinov I. Analysis of the current state and development of Russian marine coal terminals. TransportbusinessinRussia. N 4, p. 154-159 (in Russian).
- Korshunov G.I., Karimov A.M., Magamedov G.S., Tyulkin S.A. Reduction of respirable dust-induced impact on open pit mine personnel in large-scale blasting. Mining Informational and Analytical Bulletin. N 7, p. 132-144 (in Russian). DOI: 10.25018/0236_1493_2023_7_0_132
- Smirnyakov V.V., Rodionov V.A., Smirnyakova V.V., Orlov F.A. The influence of the shape and size of dust fractions on their distribution and accumulation in mine workings when changing the structure of air flow. Journal of Mining Institute. Vol. 253, p. 71-81. DOI: 10.31897/PMI.2022.12
- Vasina D., Rapoport I., Teslenko I. Solving environmental problems of open coal terminals in Vladivostok. International Journal of Professional Science. N 6, p. 27-36 (in Russian).
- Khokhlov S., Abiev Z., Makkoev V. The Choice of Optical Flame Detectors for Automatic Explosion Containment Systems Based on the Results of Explosion Radiation Analysis of Methane- and Dust-Air Mixtures. Applied Science. 2022. Vol. 12. Iss. 3. N 1515. DOI: 10.3390/app12031515
- Krivopishina M.E., Kostenko N.I. Rational equipment of the seaport terminal for export coal. Nauchno-tekhnicheskoe i ehkonomicheskoe sotrudnichestvo stran ATR v XXI veke. 2021. Vol. 1, p. 86-90 (in Russian).
- Qing-Wei Li, Yang Xiao, Cai-Ping Wang et al. Thermokinetic characteristics of coal spontaneous combustion based on thermogravimetric analysis. Fuel. 2019. Vol. 250, p. 235-244. DOI: 10.1016/j.fuel.2019.04.003
- Afanasev P.I., Makhmudov K.F. Assessment of the Parameters of a Shock Wave on the Wall of an Explosion Cavity with the Refraction of a Detonation Wave of Emulsion Explosives. Applied Sciences. 2021. Vol. 11. Iss. 9. N 3976. DOI: 10.3390/app11093976
- Jinling Guo, Junlian Gao, Kejia Yan, Bo Zhang. Unintended mitigation benefits of China’s coal de-capacity policies on methane emissions. Energy Policy. 2023. Vol. 181. N 113718. DOI: 10.1016/j.enpol.2023.113718
- Gridina E.B., Kovshov S.V., Antonenko T.I., Miroshnichenco A.K. Development of fire safety measures aimed at preventing and responding to spontaneous combustion in brown coal mines. Naukovyi Visnyk Natsionalnoho Hirnychoho Universytetu. N 6, p. 96-101. DOI: 10.33271/NVNGU/2020-6/096
- Gendler S.G., Stepantsova A.Yu., Mozzhanov D.B. Change of coal’s gas content during transportation to the temporary storage. JournalofCivilProtection. Vol. 8. N 2, р. 188-198. DOI: 10.33408/2519-237X.2024.8-2.188
- Vasilenko T., Kirillov A., Islamov A. et al. Permeability of a coal seam with respect to fractal features of pore space of fossil coals. Fuel. 2022. Vol. 329. N 125113. DOI: 10.1016/j.fuel.2022.125113
- Li Kh.U., Mashchenko I.D., Belaventsev L.P., Shlapakov P.A. On petrographic method of coal sorption properties definition. IndustrialSafety. N 1, p.31-44 (in Russian).
- Smirnyakov V.V., Smirnyakova V.V., Pekarchuk D.S., Orlov F.A. Analysis of methane and dust explosions in modern coal mines in Russia. International Journal of Civil Engineering and Technology. 2019. Vol. 10. Iss. 2, p. 1917-1929.
- Kongar-Syuryun C., Klyuev R., Golik V. et al. Principles of Sustainable Development of Georesources as a Way to Reduce Urban Vulnerability. Urban Science. 2024. Vol. 8. Iss. 2. N 44. DOI: 10.3390/urbansci8020044
- Dwivedi K.K., Chatterjee P.K., Karmakar M.K., Pramanick A.K. Pyrolysis characteristics and kinetics of Indian low rank coal using thermogravimetric analysis. International Journal of Coal Science & Technology. 2019. Vol. 6. Iss. 1, p. 102-112. DOI: 10.1007/s40789-019-0236-7
- Litvinova Т.Е., Tsareva А.А., Poltoratskaya М.Е., Rudko V.А. The mechanism and thermodynamics of ethyl alcohol sorption process on activated petroleum coke. Journal of Mining Institute. Vol. 268, p. 615-636.
- Alpatova P.N., May Chan Myae Zaw The componential semantic reflection of Russian energy industry terms in scientific articles. Research Journal in Advanced Humanities. 2024. Vol. 5. Iss. 2, p. 29-40. DOI: 58256/d498dh32
- Mustafin M.G. Modeling of rock massif geomechanical state at gas extraction from coal seams. JournalofMiningInstitute. Vol. 216, p. 57-61 (in Russian).
- Sikarev S.N., Adamov E.I., Smirnov S.G. Development of a mathematical model of behavior of air flows in the territory of open port coal warehouses. Russian Journal of Water Transport. N 62, p. 20-26 (in Russian). DOI: 10.37890/jwt.vi62.37
- Goncharov E.V., Tsyrel S.V. Geodynamic methods for assessing methane distribution in bituminous coal deposits and measures to intensify methane fluxes during mine gas drainage. Journal of Mining Institute. Vol. 222, p. 803-808. DOI: 10.18454/PMI.2016.6.803
- Safiullin R., Arias Z.P. Comprehensive Assessment of the Effectiveness of Passenger Transportation Processes using Intelligent Technologies. Open Transportation Journal. 2024. Vol. 18. N E26671212320514. DOI: 10.2174/0126671212320514240611100437
- Gendler S.G., Vasilenko T.A., Stepantsova A.Yu. Investigation of mass transfer of hard coal during its transportation to the place of temporary storage. Mining Informational and Analytical Bulletin. N 9-1, p. 135-148 (in Russian). DOI: 10.25018/0236_1493_2023_91_0_135
- Smirnov V.G., Manakov A.Y., Dirdin V.V. Activation energy for decomposition and formation of methane hydrates in pores of natural coal. Bulletin of the Kuzbass State Technical University. N 3 (103), p. 24-28 (in Russian).
- Gaidarov B.A. Review of the key characteristics of experimental methods for coalbed methane diffusion coefficient Trudy Instituta geologii Dagestanskogo nauchnogo tsentra RAN. 2022. N 4 (91), p. 24-31 (in Russian). DOI: 10.33580/2541-9684-2022-91-4-24-31
- Misnar A. Thermal conductivity of solids, liquids, gases and their compositions. Мoscow: Mir, 1968, p. 459 (in Russian).
- Starikov G.P., Yurchenko V.M., Mel’nik T.N. Activation of methane diffusion in coal under varied mechanical and thermodynamical parameters of a coal seam. Physics and High Pressure Technology. N 3, p. 122-130 (in Russian).
- Haoran Song, Baiquan Lin, Zheng Zhong, Ting Liu. Experimental study on methane diffusion kinetics in three typical metamorphic coals. Fuel. 2022. Vol. 311. N 122601. DOI: 10.1016/j.fuel.2021.122601
- Shaposhnik V.A. Activation energies of ion exchange processes. Sorption and Chromatography Processes. Vol. 22. N 5, p. 622-629 (in Russian). DOI: 10.17308/sorpchrom.2022.22/10683