Assessment of ancylite ore dressability by flotation method
- 1 — Ph.D. Leading Researcher Mining Institute, KSC RAS ▪ Orcid ▪ Scopus
- 2 — Ph.D. Leading Researcher Geological Institute, KSC RAS ▪ Orcid ▪ Scopus ▪ ResearcherID
- 3 — Ph.D. Leading Researcher Geological Institute, KSC RAS ▪ Orcid ▪ Scopus ▪ ResearcherID
- 4 — Researcher Mining Institute, KSC RAS ▪ Orcid ▪ Scopus ▪ ResearcherID
- 5 — Junior Researcher Geological Institute, KSC RAS ▪ Orcid ▪ Scopus
- 6 — Technologist Mining Institute, KSC RAS ▪ Orcid ▪ Scopus ▪ ResearcherID
Abstract
For more than 50 years, most rare earth elements were extracted from carbonatite deposits, which can contain different rare earth phases, but the main extracted minerals are bastnaesite, monazite and xenotime. Many studies focused on the improvement and development of dressing circuits for ores of these minerals. However, in some carbonatite complexes, rare earth deposits are composed partly or mainly of ancylite ores. This type of rare earth ores was very poorly studied in terms of dressability – previous experiments with ancylite ores are rare and not productive enough. Ancylite is the main concentrator of rare earth elements in most carbonatite complexes of the Devonian Kola Alkaline Province (northwest Russia). Dressability of ancylite ore from the Petyayan-Vara carbonatite field in the Vuorijärvi alkaline-ultramafic complex was assessed using the flotation method. The complex is one of the most potential rare earth deposits associated with carbonatites in the Kola Region. Petrographic and mineralogical studies demonstrated the occurrence of abundant iron and barite oxide inclusions in ancylite, which imposes restrictions on physical separation of these three minerals. The study of petrogeochemical and mineralogical composition of fractions formed during mechanical grinding of ores to a size less than 2.0 mm showed that even at this stage of sample preparation, the finest-grained fractions (less than 0.071 mm) were enriched in ancylite (to 19 vol.% or more with a content of 15 vol.% in ore). Three classes of reagents were considered as collectors in flotation experiments: fatty acids, alkyl hydroxamic acids, and amino acid derivatives. The reagent from the amino acid derivative class was highly efficient. The use of such a collector in combination with sodium hexametaphosphate depressant made it possible to obtain a flotation concentrate in an open circuit with total rare earth oxides content 33.4 wt.% at 64.7 % extraction.
Funding
Sampling of ancylite ores, their petrographic, mineralogical and geochemical studies were accomplished with financial support of the Russian Science Foundation Project N 19-77-10039-P. Studies of ore dressability were conducted under the State assignment FMEZ-2022-0003 GoI KSC RAS (Apatity). Geological study of carbonatites of the Vuorijärvi massif was accomplished under the State assignment FMEZ-2024-0004 GoI KSC RAS (Apatity).
Introduction
Rare earth elements (REE) are widely used in modern technologies (including “green” ones) [1, 2]. Approximately 51.4 % of the world REE resources are concentrated in carbonatite deposits, and bastnaesite-(Ce) (Ce,La)CO3F, monazite-(Ce) (Ce,La)PO4 and xenotime-(Y) YPO4 are the most significant REE minerals and are of the greatest commercial interest [3, 4]. Ores of carbonatite deposits are difficult to beneficiate, since in most cases both rare earth minerals and the rock component are represented by carbonates. The similarity of their physical properties requires a consistent use of different dressing methods. For this reason, the technologies applied and proposed for the extraction of rare earth metal concentrates are generally multi-stage and combine gravity, magnetic and flotation dressing methods [5]. A high density of REE minerals determines the advisability of gravity separation [6, 7] as pre-concentration [5, 8] and in finishing operations [9, 10]. Magnetic separation is relevant when there is a difference in magnetic properties of the separated minerals [11, 12]. The same applies to minerals with low magnetic susceptibility [13, 14]. Magnetic separation in a weak field is used to separate iron-containing minerals also after reducing roasting. However, the most demanded method of REE ore dressing is flotation [15]. Most studies aimed at development and improvement of the flotation process focus on the surface characteristics of the most common rare earth minerals – bastnaesite and monazite, on their interaction with flotation reagents [16, 17]. Since minerals containing REE oxides and gangue minerals often have similar physicochemical properties, the correct selection of the reagent mode and preliminary preparation are of great importance for their flotation separation [18, 19]. Oxyhydryl collectors are used for dressing of REE ores [20] including traditional fatty acids [21, 22], with participation of activators [23] and depressants [24]. Most demanded for REE ore flotation are the compounds with a hydroxamate group [25, 26]. On the surface of minerals, these reagents form mono- or bidentate complexes [27]. The presence of two hydroxamate groups in the reagent based on alkyl malonic acids ensures a high selectivity with respect to bastnaesite [28]. Both aliphatic [29] and aromatic reagents are considered as collectors: benzene hydroxamic [30], naphthyl hydroxamic [31], salicylic hydroxamic [32] acids. The problem largely lies in the fact that the rocks that are REE ores in certain carbonatite deposits have a specific mineral composition. This requires the selection of specific reagents and their combinations that are most efficient in dressing of ores from each deposit.
It should be noted that in recent years bastnaesite took the leading position as a source of REE, which is primarily due to the development of large deposits in China (for example, Bayan-Obo) and the USA (Mountain Pass) [33]. Therefore, most of the research focuses on the improvement and development of dressing circuits for bastnaesite ores. However, there are geological complexes in the world with ores associated with alternative rare earth carbonates. An example of non-bastnaesite mine-ralization in carbonatites are ores of the second largest US deposit Bear Lodge [34], where a significant volume of REE is contained in ancylite-(Ce) CeSr(CO3)2(OH)H2O. Unfortunately, there are only a few works devoted to dressing of such ores [34]. As studies of the Bear Lodge ancylite ores showed, a direct transfer of dressing experience accumulated for bastnaesite ores to ancylite ores is not successful. The use of physical dressing methods alone (gravity and magnetic separation) makes it possible to obtain a concentrate containing 6.64 wt.% REE at an extraction rate 86.4 % from the Bear Lodge deposit ore (4.5 wt.% REE). Studies of the surface properties of ancylite, strontianite and calcite under the action of octane hydroxamic acid showed that the properties of these minerals are similar [34], which makes it necessary to investigate other classes of reagents for the flotation extraction of ancylite. A technology [35, 36] including high-intensity magnetic separation followed by flotation of the non-magnetic fraction was proposed for the ore of this deposit. According to the proposed technology, a concentrate containing 11.2 % REE at extraction rate 61.2 % is expected to be obtained from ore containing 4.5 wt.% REE. In this paper, the object of the study is one of the varieties of rare earth ores of the Petyayan-Vara carbonatite field in the Vuorijärvi alkaline-ultramafic complex (Kola Region, northwest Russia) in which the main concentrator of REE is also ancylite. REE resources in these carbonatites were not calculated, but this object is potentially one of the most promising REE deposits in the Kola Region associated with carbonatites [37]. In the process of developing new deposits, the analysis of geometallurgical parameters and potential methods of mineral extraction is of crucial importance [38]. The objective of this work is to assess the dressability of Petyayan-Vara ancylite ore by the flotation method. The results obtained can be used in the development of both rare earth deposits in the Kola Alkaline Province in particular, and ancylite ores of carbonatite deposits in general.
Geology of the study object
The Vuorijärvi massif, along with more than twenty other complexes, is part of the Paleozoic Kola Alkaline Province formed 380-360 million years ago. In a number of complexes in this Province (the Khibiny, Sebljavr, Sallanlatva, Sokli, Vuorijärvi massifs), the occurrence of REE mineralization was recorded [39] including that of economic interest. For example, according to the available data [40], barite-rich ankerite and siderite carbonatites of Sallanlatva (the so-called barite ores) traced to a depth of 500 m (C2+P1 reserves) contain about 1 million tons of REE oxides. It should be noted that the specific feature of all carbonatite complexes in the Kola Province, in which the presence of REE mineralization was ascertained, is a wide occurrence of ancylite with subordinate other REE minerals, such as bastnaesite, burbankite, monazite, etc. [39], which brings them closer to the Bear Lodge deposit [41, 42]. However, the resources of most alkaline-ultramafic complexes in the Province were not assessed due to insufficient study of REE rocks occurring there. One of such complexes is Vuorijärvi, in which a large-scale occurrence of REE carbonatites was established in the Petyayan-Vara area [43]. Rare earth elements-carbonatites of Petyayan-Vara were discovered in the 1950s during exploration for phlogopite [43], but until recently, no specialized work on their study was carried out. This article is part of a comprehensive study aimed at filling this gap. Among the carbonatites of the Petyayan-Vara field, two types of REE-rich rocks were described [43] – ancylite-dolomite and bastnaesite-dolomite carbonatites (ancylite and bastnaesite ores) with the former markedly predominating. Both ore varieties are for the most part breccias composed of fragments of early magmatic dolomite carbonatites and cement with a high REE content. In ancylite ores (Fig.1, a) the cement consists of ancylite-(Ce), barite, first-generation strontianite, and calcite (±quartz) (Fig.1, b); in bastnaesite ores, the predominant matrix minerals are bastnaesite-(Ce) and quartz. Total content of rare earth oxides (ΣTR2O3) in ancylite ores averages 10 wt.% or more, whereas bastnaesite ores contain 5 wt.% or less ΣTR2O3. The available data allow stating that lower REE contents in bastnaesite ores and a lower prevalence of such rocks are due to their nature – bastnaesite ores formed as a result of late local metasomatic action on ancylite ores which caused dissolution of the latter, remobilization and deposition of REE in bastnaesite (with accompanying dilution) as well as the release of Sr and its precipitation at a distance in second-generation strontianite [44, 45]. Detailed information on various aspects of geology of the Vuorijärvi carbonatites is presented in [40, 46].
Methods
For flotation experiments at the Petyayan-Vara area, about 1 t of ancylite ore was taken, which was pre-crushed before the experiment in laboratory jaw and roller crushers manufactured by JSC REC “Mekhanobr-tekhnika” to a size –2 mm. Samples were divided by the size grades into 10 fractions (Table 1) for each of which the chemical composition was determined, and artificial thin sections were made for mineralogical analysis. The content of total REE oxides was determined gravimetrically, the content of magnesium, calcium, barium and iron – by atomic absorption spectroscopy (atomic absorption spectrometer “Quantum 2MT”), silicon – by colorimetry (spectrophotometer UNICO 1201). Analytical errors were 1.5 % for concentrations >10 wt.% and 3.5 % for concentrations from 1 to 10 wt.%. The contents of individual REE were determined by the ICP-MS method (inductively coupled plasma mass spectrometer ELAN 9000 (PerkinElmer). Studies of phase and intraphase heterogeneity of individuals and the study of chemical composition of minerals were accomplished by optical methods on an Axioplan polarizing microscope (with concomitant registration of mineral bodies using a video camera) and by electron microscopy methods on a LEO-1450 scanning electron microscope with a Quantax energy-dispersive attachment. Quantitative analysis of the content of minerals in fractions was performed by calculation (based on chemical compositions of fractions and the determined composition of minerals) with verification of the obtained results using the ISA software application. This software is designed to calculate from backscattered electron (BSE) images the percentage of pixels of various shades of grey corresponding to certain minerals at fixed brightness and contrast parameters. An example of operation of such application is shown in Fig.1, c, d.
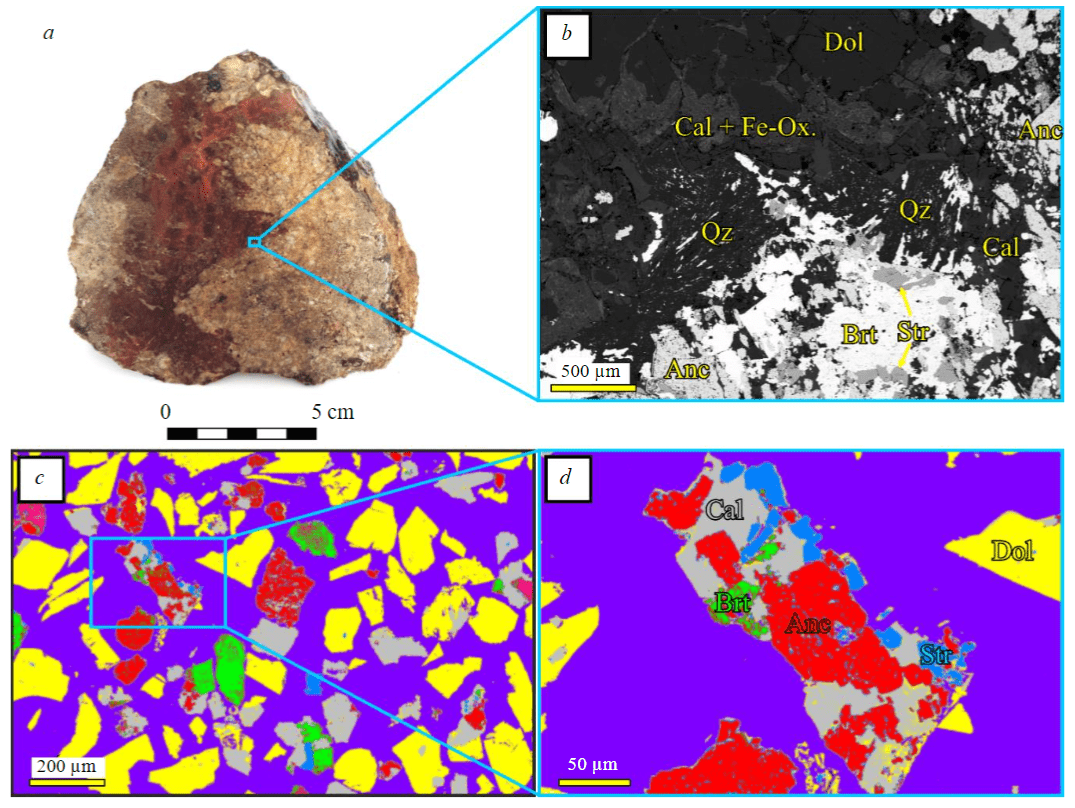
Fig.1. Ancylite ores of the Petyayan-Vara area, Vuorijärvi carbonatite massif: a – general view of rocks; b – backscattered electron (BSE) image of the contact between brecciated dolomite carbonatite and cement bearing REE mineralization; c, d – an example of processing a BSE image of an artificial thin section (for illustration, a fraction from –0.315 to +0.2 mm was taken) by ISA software visualizing the ratios of minerals in ore Anc – ancylite; Brt – barite; Cal – calcite; Dol – dolomite; Fe-Ox – iron oxides; Qz – quartz; Str – strontianite. Epoxy resin is shown in purple
A 400 g ore subsample was crushed to flotation size in a laboratory ball mill. The content of –0.071 mm grade in ground ore was 49.8 %. Before flotation, a desliming operation was performed, the yield of sludge was 6.2-6.5 %. Flotation was carried out in a 237FL-A laboratory mechanical flotation machine (manufactured by JSC REC “Mekhanobr-tekhnika”), the chamber volume in rougher flotation was 1 l, in recleaning operations 0.5 l. Air consumption was 1 l/min per 1 l of pulp volume. Flotation was carried out on tap water in an open circuit including rougher flotation and three recleaning operations of the froth product. The required pH value of 9.8-9.9 was created by adding Na2CO3. The time of rougher flotation and recleaning operations is 3 min, flotation temperature 18-20 °C. Dressing results were calculated on the basis of data on chemical analysis of dressing products.
Table 1
Content of the main components in an ancylite ore sample
Size grade, mm |
Yield, % |
Content, wt.% |
|||||
CaO |
MgO |
Fetot |
SiO2 |
BaO |
ΣTR2O3 |
||
+6 |
5.01 |
26.74 |
8.98 |
2.01 |
4.74 |
3.55 |
10.83 |
From –1.6 to +1.0 |
9.50 |
27.27 |
11.84 |
2.35 |
1.23 |
3.26 |
10.45 |
From –1.0 to +0.63 |
9.01 |
27.80 |
13.69 |
2.48 |
2.11 |
2.09 |
6.84 |
From –0.63 to +0.4 |
9.01 |
27.73 |
14.16 |
2.46 |
2.19 |
2.12 |
6.15 |
From –0.4 to +0.315 |
4.10 |
27.51 |
14.42 |
2.43 |
1.53 |
2.00 |
6.93 |
From –0.315 to +0.2 |
6.80 |
26.76 |
14.29 |
2.57 |
1.49 |
1.91 |
6.20 |
From –0.2 to +0.16 |
3.01 |
26.06 |
12.46 |
2.61 |
1.88 |
2.21 |
7.81 |
From –0.16 to +0.071 |
3.03 |
26.14 |
10.92 |
2.62 |
1.67 |
2.86 |
11.45 |
From –0.071 to +0.05 |
44.91 |
24.70 |
10.92 |
2.67 |
1.87 |
3.98 |
14.42 |
From –0.05 |
5.62 |
23.36 |
7.73 |
3.95 |
2.82 |
6.96 |
18.33 |
Total |
100.00 |
25.86 |
11.69 |
2.62 |
2.01 |
3.41 |
11.50 |
Experiments on non-froth flotation in a Halimond tube were performed on samples of ancylite and rock minerals separated from the ore fraction with a size from –0.16 to +0.1 mm. Separation was carried out in heavy liquid with a density 3.65 g/cm3. REE content in the fraction with density ρ > 3.65 g/cm3 was βTR2O3 = 45.8 %, the fraction of carbonate rock minerals (mainly calcite and dolomite) was characterized by a content of βCaO = 29.35 %; βMgO = 14.44 %. The required pH value of 9.6-9.8 was created by adding NaOH. A 0.5 g mineral subsample was agitated for 1 min with NaOH solution, 2 min with a collector solution, and then floated in a 100 ml chamber for 3 min. Air feed rate was 5.3 ml/min, flotation temperature 20 °C. Strength of reagent fixation on ancylite and rock minerals was assessed by flotation in a Halimond tube under desorption conditions. After agitation of the mineral with reagent, 50 % of the liquid phase was replaced with distilled water with pH 9.6-9.8, stirred for another 2 min, and then floated. The efficiency of reagents was assessed by the yields of separation products; in case of the rock mineral fraction, the resulting products were analysed for CaO and MgO content.
Discussion of results
According to the accomplished study, the main petrogeochemical components of the studied ancylite ores are (in descending order) CaO, MgO and ΣTR2O3, and the contents of the last two components are very close (Table 1). The fractions identified by size are similar in chemical composition, but there are a number of trends: CaO content is consistent and decreases slightly only in the finest-grained fractions; MgO content is at maximum in medium-grained fractions (from –0.63 to +0.2) and decreases both in the direction of coarsening and decreasing grain size; ΣTR2O3 content, on the contrary, is minimal in medium-grained fractions (6-7 wt.%), increases twofold (to 11 wt.%) in the direction of coarser-grained fractions and threefold (to 18 wt.%) in the direction of fine-grained fractions. It should be noted that, according to ICP-MS analysis, in the studied ore the REE are represented mainly (99.5 %) by lanthanides of the cerium group (from Ce to Eu), with (La + Ce) share accounting for 86.3 %.
Quantitative mineralogical analysis (Table 2) showed that the ore under study is a mixture of carbonate minerals, the separation of which by flotation is a very interesting and complicated task due to similarity of their surface properties. The main mineral of ancylite ores is dolomite, the content of which, like that of MgO, is determined in medium-grained fractions (to 83 vol.%). The content of ancylite (the second most common mineral of the studied ores) changes according to the same pattern as the content of ΣTR2O3 reaching its maximum in the finest-grained fractions (to 24 vol.%) with a minimum (8-9 vol.%) in medium-grained fractions. The content of barite changes in a way similar to ancylite. This is due to the fact that in ancylite ores of Petyayan-Vara a significant part of barite, like strontianite, occurs as inclusions in ancylite and close intergrowths with it (see Fig.1, d), which makes these minerals physically difficult to separate. At the geochemical level (see Table 1), this relationship shows up as a strong linear dependence between the contents of ΣTR2O3 (the main concentrator of REE – ancylite) and BaO (concentrator – barite) with a Pearson correlation coefficient 0.95. The third most common mineral in ancylite ores is calcite, the content of which turned out to be maximum in the coarsest-grained fraction (to 21 vol.%), sharply decreased (to 3-5 vol.%) in medium-grained fractions and again increased (to 18 vol.%) in the finest-grained fractions.
Table 2
Quantitative mineral composition of ancylite ore
Size grade, mm |
Mineral, vol.% |
||||||
Dol |
Cal |
Anc |
Str |
Brt |
Fe-Ox |
Qz |
|
+1.6 |
52.27 |
20.60 |
14.46 |
3.81 |
3.36 |
0.55 |
4.95 |
From –1.6 to +1.0 |
65.98 |
11.58 |
13.36 |
4.38 |
2.95 |
0.52 |
1.23 |
From –1.0 to +0.63 |
77.99 |
6.81 |
8.94 |
1.70 |
1.94 |
0.48 |
2.15 |
From –0.63 to +0.4 |
78.62 |
5.02 |
7.83 |
4.00 |
1.92 |
0.43 |
2.18 |
From –0.4 to +0.315 |
80.63 |
3.83 |
8.89 |
2.90 |
1.82 |
0.40 |
1.54 |
From –0.315 to +0.2 |
82.82 |
2.98 |
8.24 |
2.11 |
1.80 |
0.51 |
1.54 |
From –0.2 to +0.16 |
73.33 |
7.85 |
10.55 |
3.51 |
2.12 |
0.66 |
1.98 |
From –0.16 to +0.071 |
64.73 |
13.26 |
15.57 |
1.13 |
2.76 |
0.79 |
1.77 |
From –0.071 to +0.05 |
62.80 |
10.19 |
19.03 |
1.56 |
3.73 |
0.79 |
1.92 |
–0.05 |
44.98 |
18.38 |
24.46 |
0.88 |
6.59 |
1.77 |
2.94 |
Total |
66.84 |
9.81 |
15.13 |
2.27 |
3.18 |
0.71 |
2.06 |
In the present work, reagents of three classes of compounds are considered as collectors for flotation of ancylite. Fatty acids, including oleic acid, are traditional collectors for flotation of carbonate minerals and, as a rule, are considered as a reference compound for a comparative evaluation of the action of new reagents.
Hydroxamic acids are widely used in flotation of REE ores. The presence in the molecule of this collector of a hydroxamate group capable of forming complex compounds with transition metals, determines the selectivity of their action at flotation of bastnaesite and monazite ores [27, 34]. Using Raman spectroscopy, the preferential adsorption of octane hydroxamic acid on synthetic cerium carbonate was shown in comparison with calcite [47].
The effect of three classes of collectors on ancylite ore minerals was studied using the non-froth flotation method: fatty acids using oleic acid as an example; alkyl hydroxamic acids on the example of pelargonic hydroxamic acid C8H17CONHOH (the reagent was synthesized at the Laboratory of the Mining Institute of KSC RAS, acid number is 321 mg/g of substance, melting point 82 °C) and amino acid derivatives exemplified by Berol 2015 reagent (Nouryon). All the reagents were used as sodium salts pre-saponified with a calculated amount of NaOH.
Figure 2 shows the concentration dependences of floatability of collectors from three classes of compounds with respect to ancylite and rock minerals. From the data in Fig.2, a higher hydrophobizing effect with respect to all minerals is obvious for oleic acid and Berol 2015 reagent, which can be expected due to a greater length of the hydrocarbon radical. In case of pelargonic hydroxamic acid, an order of magnitude higher reagent concentrations are required to achieve the same effect, which is associated with a much shorter length of the hydrocarbon radical. It should be noted that the difference in the action of reagents with respect to ancylite and rock minerals is more pronounced for pelargonic hydroxamic acid. This indicates a higher selectivity of the action of such a collector.
Flotation under desorption conditions showed (Fig.3) that, in terms of their efficiency with respect to ancylite, the reagents are arranged in the following order: oleic acid > Berol 2015 > C8H17CONHOH. This sequence of reagents is determined to a greater extent by the length of the hydrocarbon radical in these collectors. The larger the hydrophobic radical, the lower concentration of reagent is sufficient to ensure hydrophobization of the mineral surface. In case of pelargonic hydroxamic acid, the hydrocarbon part of the molecule is quite small, and the remaining concentration of reagent on the surface after “washing” is insufficient for the required hydrophobization of the mineral. With increasing concentration of collector, the specificity of interaction of the functional group that ensures fixation of reagent on the mineral surface becomes more important. As for rock carbonate minerals, a high degree of reagent “washing” under desorption conditions is demonstrated for Berol 2015 reagent. Hydrophobic effect of oleic acid in relation to calcite and dolomite also shows up under desorption conditions, which indicates a low efficiency of recleaning operations when using this collector in flotation.
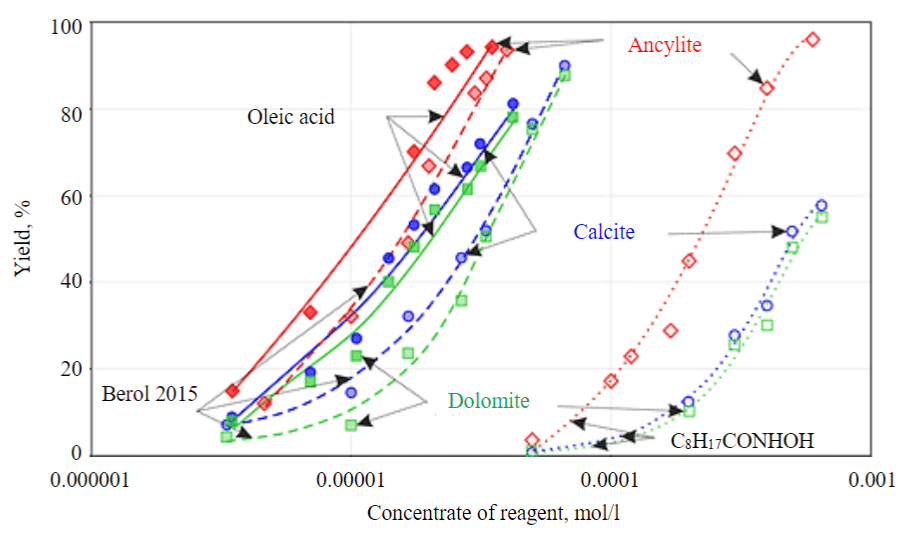
Fig.2. Results of flotation experiment in Halimond tube for ancylite (red), calcite (blue) and dolomite (green) with reagents: oleic acid (solid lines), Berol 2015 (dashed lines) and С8H17CONHOH (dotted lines)
Evaluation of the properties of reagents demonstrated in non-froth flotation of minerals suggests that the highest efficiency in flotation of ancylite ore should be expected for pelargonic hydroxamic acid due to its high selectivity with respect to ancylite and Berol 2015 reagent, the specificity of the action of which is combined with a low strength of fixation on rock minerals. The dependencies obtained in flotation of ancylite ore at pH = 9.6-9.8 differ slightly from those obtained in non-froth flotation of pure minerals (Fig.4). To obtain similar quality indicators of the concentrate and the extraction of REE into it when using pelargonic hydroxamic acid, approximately 2-2.5 times less reagent is required than with Berol 2015. However, a “cleaner” product with a lower content of rock minerals is obtained when using Berol 2015.
It is known that the use of depressants allows increasing the flotation efficiency. In some cases, depressant reagents at certain flow rates play the role of a peptizer of sludge particles which can affect the flotation of such easily crushable mineral as ancylite. Two well-known depressant reagents were tested at different flow rates on the ore under study: liquid glass (LGl) and sodium hexametaphosphate (HMPh) [48]. Flotation was carried out in an open circuit, on fresh water, with rougher flotation and three recleanings of the froth product. The best of the results obtained are given in Table 3.
Table 3
Open circuit flotation of ancylite ore with different depressants
Reagent mode, g/t |
Concentrate |
Content in tailings, wt.% |
|||||
Content, wt.% |
Extraction, % |
||||||
ΣTR2O3 |
CaO |
MgO |
ΣTR2O3 |
CaO |
MgO |
ΣTR2O3 |
|
350 g/t С8H17CONHOH + 150 g/t LGl |
18.8 |
18.49 |
7.32 |
55.2 |
15.2 |
12.7 |
3.47 |
350 g/t С8H17CONHOH + 100 g/t HMPh |
20.5 |
20.83 |
7.84 |
47.5 |
13.7 |
11.8 |
4.50 |
800 g/t Berol 2015 + 100 g/t LGl |
32.0 |
13.62 |
3.62 |
60.8 |
8.0 |
4.8 |
2.00 |
1,000 g/t Berol 2015 + 120 g/t HMPh |
33.4 |
13.45 |
3.8 |
64.7 |
7.7 |
5.2 |
2.10 |
The advantage of Berol 2015 reagent which belongs to the class of amino acid derivatives over hydroxamic acids is also retained when using depressants. At a higher quality of the concentrate in this case (33.4 % ΣTR2O3), a more complete REE extraction into the concentrate is achieved (64.7 % in the open circuit from the flotation feed). The obtained results have a clear advantage over the previously obtained dressing indicators of similar ancylite ore with carbonate rock minerals. As is known from literary sources, it is expected to obtain a concentrate with 11.2 % ΣTR2O3 content at 61.2 % extraction from the ore of the Bear Lodge deposit (4.5 % ΣTR2O3) as a result of combination of high-intensity magnetic separation and the flotation method [35]. The concentration degree of the valuable component (REE) in this case is only 2.5-fold. In our case, using only the flotation method, a concentrate was obtained with 4.5-fold REE concentration degree.
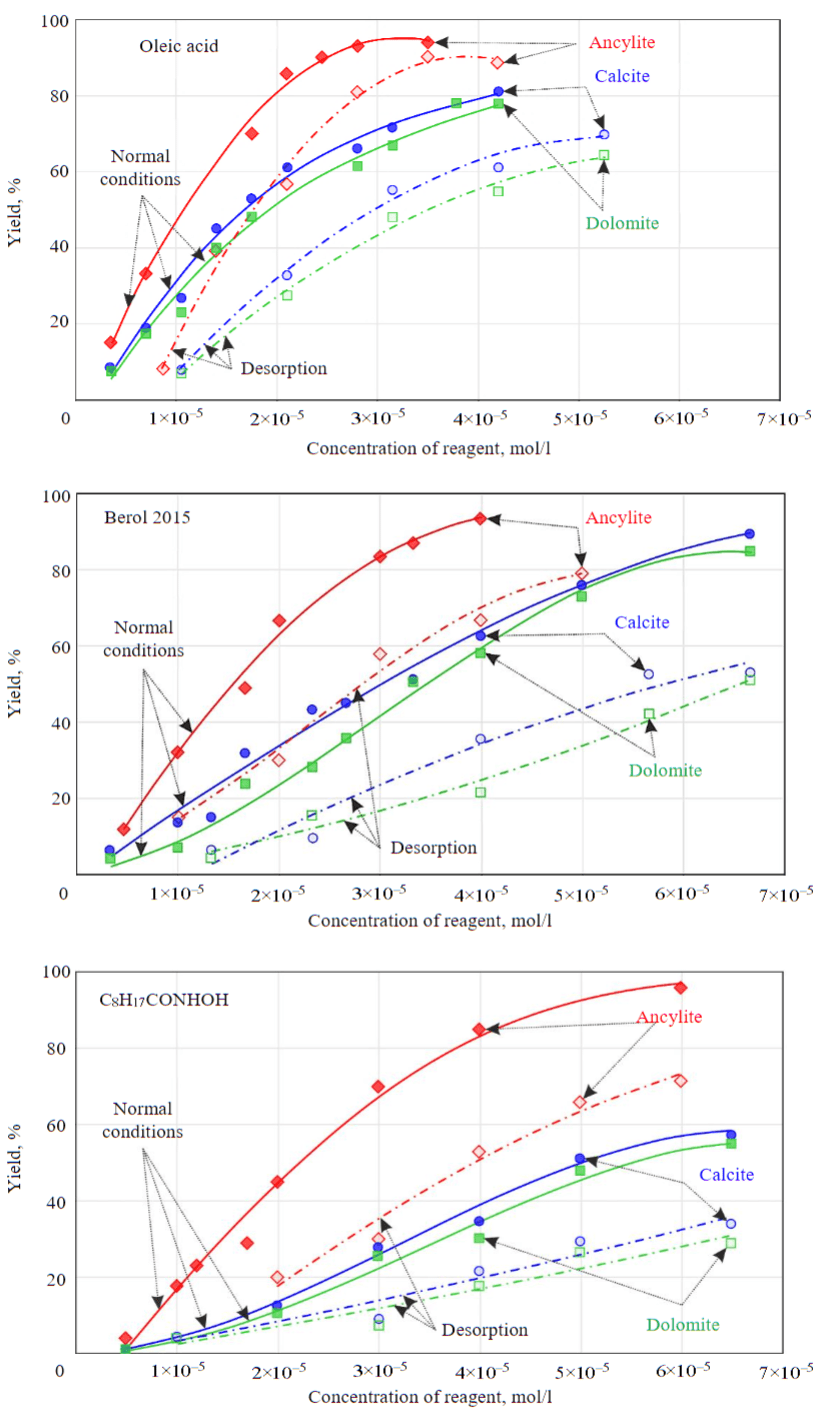
Fig.3. Flotation with sodium oleate (a), Berol 2015 (b) and C8H17CONHOH (c) of ancylite (red), calcite (blue) and dolomite (green) under normal conditions (solid lines) and under desorption conditions (dash-dotted lines)
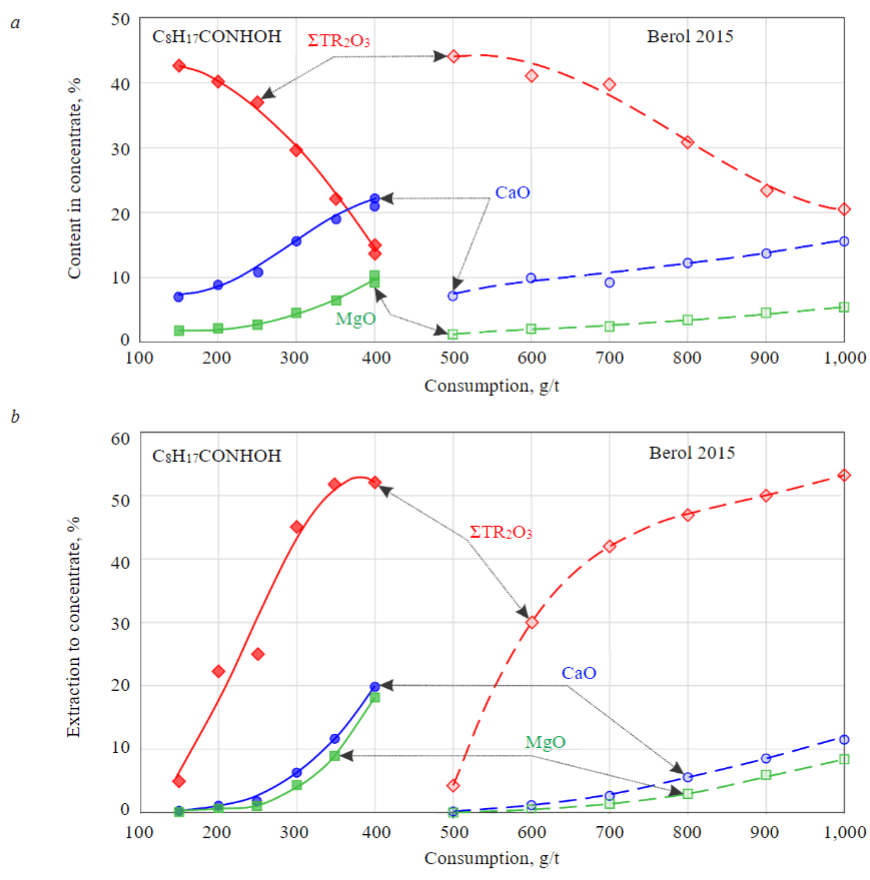
Fig.4. Indicators of ancylite ore flotation with Berol 2015 (solid lines) and С8H17CONHOH (dashed lines) reagents for ΣTR2O3 (red), CaO (blue) and MgO (green): a – content in concentrate; b – extraction to concentrate
The possibility of efficient separation of rare-earth carbonates from calcite and dolomite by flotation will undoubtedly be in demand in the combined dressing circuit. A high density of rare-earth carbonates determines the advisability of gravity dressing at the first stage. However, given the tendency of ancylite to overcrushing, flotation will be required to extract the valuable component from the sludge products of gravity.
Conclusion
Dressability of ancylite ore from the Petyayan-Vara area in the Vuorijärvi alkaline-ultramafic complex was studied. An evaluation of dressability of ancylite ore by the flotation method was accomplished. It was shown that reagents with nitrogen-containing groups in their structure – alkyl hydroxamic acid and amino acid derivatives – are characterized by a higher selectivity of action in relation to REE carbonates. The reagent from the class of amino acid derivatives (Berol 2015) is characterized by a less strong fixation on rock minerals, which ensures more efficient recleaner operations. When using the best reagent mode including Berol 2015 reagent (amino acid derivatives) in combination with the hexametaphosphate depressant, a flotation concentrate was obtained in an open circuit with ΣTR2O3 content 33.4 wt.% at 64.7 % extraction from flotation feed.
References
- Chakhmouradian A.R., Wall F. Rare Earth Elements: Minerals, Mines, Magnets (and More). Elements. 2012. Vol. 8. N 5, p. 333-340. DOI: 10.2113/gselements.8.5.333
- Jordens A., Ying Ping Cheng, Waters K.E. A review of the beneficiation of rare earth element bearing minerals. Minerals Engineering. 2013. Vol. 41, p. 97-114. DOI: 10.1016/j.mineng.2012.10.017
- Yushina T.I., Petrov I.M., Cherny S.A., Petrova A.I. Overview of processing technologies for the raw materials of rare-earth metals (REM) at existing enterprises. Obogashchenie Rud. N 2, p. 45-51 (in Russian). DOI: 10.17580/or.2020.02.08
- Das S.K., Angadi S.I., Kundu T., Basu S. Mineral Processing of Rare Earth Ores. Rare-Earth Metal Recovery for Green Technologies. Springer, 2020, p. 9-38. DOI: 10.1007/978-3-030-38106-6_2
- Pell R., Wall F., Xiaoyu Yan et al. Temporally explicit life cycle assessment as an environmental performance decision making tool in rare earth project development. Minerals Engineering. 2019. Vol. 135, p. 64-73. DOI: 10.1016/j.mineng.2019.02.043
- Wall F. Chapter 13 – Rare earth elements. Critical Metals Handbook. Hoboken: Wiley, 2014, p. 312-339. DOI: 10.1002/9781118755341.ch13
- Abaka-Wood G.B., Quast K., Zanin M. et al. A study of the feasibility of upgrading rare earth elements minerals from iron-oxide-silicate rich tailings using Knelson concentrator and Wilfley shaking table. Powder Technology. 2019. Vol. 344, p. 897-913. DOI: 10.1016/j.powtec.2018.12.005
- Norgen A., Anderson C. Recovery of Rare Earth Oxides from Flotation Concentrates of Bastnaesite Ore by Ultra-Fine Centrifugal Concentration. Metals. 2021. Vol. 11. Iss. 9. N 1498. DOI: 10.3390/met11091498
- Maksimova V.V., Krasavtseva E.A., Savchenko E.E. et al. Study of the composition and properties of the beneficiation tailings of currently produced loparite ores. Journal of Mining Institute. Vol. 256, p. 642-650. DOI: 10.31897/PMI.2022.88
- Abaka-Wood G.B., Zanin M., Addai-Mensah J., Skinner W. Recovery of rare earth elements minerals from iron oxide–silicate rich tailings – Part 1: Magnetic separation. Minerals Engineering. 2019. Vol. 136, p. 50-61. DOI: 10.1016/j.mineng.2019.02.026
- Rejith R.G., Sundararajan M. Combined magnetic, electrostatic, and gravity separation techniques for recovering strategic heavy minerals from beach sands. Marine Georesources & Geotechnology. 2018. Vol. 36. Iss. 8, p. 959-965. DOI:1080/1064119X.2017.1403523
- Andronov G.P., Zakharova I.B., Filimonova N.M. et al. Magnetic Separation of Eudialyte Ore under Pulp Pulsation. Journal of Mining Science. Vol. 52. N 6, p. 1190-1194. DOI: 10.1134/S1062739116061735
- Thi Yen Chau Nguyen, Lan-Huong Tran, Mueller K.K. et al. Pre-concentration of fluorite from a rare earth element carbonatite deposit through the combination of magnetic separation and leaching. Minerals Engineering. 2021. Vol. 174. N 106998. DOI: 10.1016/j.mineng.2021.106998
- Kim K., Jeong S. Separation of Monazite from Placer Deposit by Magnetic Separation. Minerals. 2019. Vol. 9. Iss. 3. N DOI: 10.3390/min9030149
- Weiping Liu, Xuming Wang, Miller J.D. Collector Chemistry for Bastnaesite Flotation – Recent Developments. Mineral Processing and Extractive Metallurgy Review. 2019. Vol. 40, p. 370-379. DOI: 10.1080/08827508.2019.1647840
- Marion C., Ronghao Li, Waters K.E. A review of reagents applied to rare-earth mineral flotation. Advances in Colloid and Interface Science. 2020. Vol. 279. N 102142. DOI: 10.1016/j.cis.2020.102142
- Wencai Zhang, Honaker R. A fundamental study of octanohydroxamic acid adsorption on monazite surfaces. International Journal of Mineral Processing. 2017. Vol. 164, p. 26-36. DOI: 10.1016/j.minpro.2017.05.006
- Bulatovic S.M. 24 – Flotation of REO Minerals. Handbook of Flotation Reagents: Chemistry, Theory and Practice. Elsevier, 2010, p. 151-173. DOI: 10.1016/B978-0-444-53082-0.00024-X
- Abaka-Wood G.B., Fosu S., Addai-Mensah J., Skinner W. Flotation recovery of rare earth oxides from hematite–quartz mixture using sodium oleate as a collector. Minerals Engineering. 2019. Vol. 141. N 105847. DOI: 10.1016/j.mineng.2019.105847
- Zhoujie Wang, Houqin Wu, Yanbo Xu et al. Effect of dissolved fluorite and barite species on the flotation and adsorption behavior of bastnaesite. Separation and Purification Technology. 2020. Vol. 237. N 116387. DOI: 10.1016/j.seppur.2019.116387
- Yushina T.I., Petrov I.M., Cherny S.A., Petrova A.I. Rare-earth metal ore processing technologies when developing new deposits. Obogashchenie rud. N 6, p. 47-53 (in Russian). DOI: 10.17580/or.2020.06.08
- Geneyton A., Filippov L.O., Heinig T. et al. Towards the efficient flotation of monazite from silicate-rich tailings with fatty acids collectors using a lanthanum salt as a selective phosphate activator. Minerals Engineering. 2021. Vol. 160. N 106704. DOI: 10.1016/j.mineng.2020.106704
- Zhoujie Wang, Houqin Wu, Jie Yang et al. Selective flotation separation of bastnaesite from calcite using xanthan gum as a depressant. Applied Surface Science. 2020. Vol. 512. N 145714. DOI: 10.1016/j.apsusc.2020.145714
- Yiming Lin, Cheng Chen, Weiqing Wang et al. Beneficial effects and mechanism of lead ions for bastnaesite flotation with octyl hydroxamic acid collector. Minerals Engineering. 2020. Vol. 148. № 106199. DOI: 10.1016/j.mineng.2020.106199
- Mei Li, Kai Gao, Dongliang Zhang et al. The influence of temperature on rare earth flotation with naphthyl hydroxamic acid. Journal of Rare Earths. 2018. Vol. 36. Iss. 1, p. 99-107. DOI: 10.1016/j.jre.2017.07.004
- Wanhala A.K., Doughty B., Bryantsev V.S. et al. Adsorption mechanism of alkyl hydroxamic acid onto bastnäsite: Fundamental steps toward rational collector design for rare earth elements. Journal of Colloid and Interface Science. 2019. Vol. 553, 210-219. DOI: 10.1016/j.jcis.2019.06.025
- Hao Duan, Wengang Liu, Xinyang Wang et al. Investigation on flotation separation of bastnaesite from calcite and barite with a novel surfactant: Octylamino-bis-(butanohydroxamic acid). Separation and Purification Technology. 2021. Vol. 256. N DOI: 10.1016/j.seppur.2020.117792
- Xinyang Yu, Ruirui Zhang, Siyuan Yang et al. A novel decanedioic hydroxamic acid collector for the flotation separation of bastnäsite from calcite. Minerals Engineering. 2020. Vol. 151. N 106306. DOI: 10.1016/j.mineng.2020.106306
- Espiritu E.R.L., Naseri S., Waters K.E. Surface chemistry and flotation behavior of dolomite, monazite and bastnäsite in the presence of benzohydroxamate, sodium oleate and phosphoric acid ester collectors. Colloids and Surfaces A: Physicochemical and Engineering Aspects. 2018. Vol. 546, p. 254-265. DOI: 10.1016/j.colsurfa.2018.03.030
- Xinyu Zhu, Yiming Lin, Yang Huang et al. Adsorption of ferric ions on the surface of bastnaesite and its significance in flotation. Minerals Engineering. 2020. Vol. 158. N 106588. DOI: 10.1016/j.mineng.2020.106588
- Shiming Cao, Yijun Cao, Yinfei Liao, Zilong Ma. Depression Mechanism of Strontium Ions in Bastnaesite Flotation with Salicylhydroxamic Acid as Collector. Minerals. 2018. Vol. 8. Iss. 2. N 66. DOI: 10.3390/min8020066
- Zhen-Yu Wang, Hong-Rui Fan, Lingli Zhou et al. Carbonatite-Related REE Deposits: An Overview. Minerals. 2020. Vol. Iss. 11. N 965. DOI: 10.3390/min10110965
- van Rythoven A.D., Pfaff K., Clark J.G. Use of QEMSCAN® to characterize oxidized REE ore from the Bear Lodge carbonatite, Wyoming, USA. Ore and Energy Resource Geology. 2020. Vol. 2-3. N 100005. DOI: 10.1016/j.oreoa.2020.100005
- Hao Cui, Anderson C.G. Fundamental Studies on the Surface Chemistry of Ancylite, Calcite, and Strontianite. Journal of Sustainable Metallurgy. 2017. Vol. 3. Iss. 1, p. 48-61. DOI: 10.1007/s40831-016-0097-x
- Hao Cui, Anderson C. Simulation of the Flotation of Bear Lodge Ore and a Preliminary Economic Analysis. Extraction 2018. Proceedings of the First Global Conference on Extractive Metallurgy. Springer, 2018, p. 2597-2608. DOI: 10.1007/978-3-319-95022-8_219
- Hao Cui, Anderson C.G. Alternative flowsheet for rare earth dressing of Bear Lodge ore. Minerals Engineering. 2017. Vol. 110, p. 166-178. DOI: 10.1016/j.mineng.2017.04.016
- Downes H., Balaganskaya E., Beard A. et al. Petrogenetic processes in the ultramafic, alkaline and carbonatitic magmatism in the Kola Alkaline Province: A review. Lithos. 2005. Vol. 85. Iss. 1-4, p. 48-75. DOI: 10.1016/j.lithos.2005.03.020
- Andersen A.K., Clark J.G., Larson P.B., Neill O.K. Mineral chemistry and petrogenesis of a HFSE (+HREE) occurrence, peripheral to carbonatites of the Bear Lodge alkaline complex, Wyoming. American Mineralogist. 2016. Vol. 101. Iss. 7, p. 1604-1623. DOI: 10.2138/am-2016-5532
- Wall F., Zaitsev A.N. Rare earth minerals in Kola carbonatites. Phoscorites and carbonatites from mantle to mine: the key example of the Kola alkaline province. London: Mineralogical Society of Great Britain and Ireland, 2004, p. 341-373. DOI: 10.1180/MSS.10.10
- Afanasev B.V. Mineral resources of alkaline-ultrabasic massifs in Kola Peninsula. St. Petersburg: Roza vetrov, 2011, p. 224 (in Russian).
- Andersen A.K., Clark J.G., Larson P.B., Donovan J.J. REE fractionation, mineral speciation, and supergene enrichment of the Bear Lodge carbonatites, Wyoming, USA. Ore Geology Reviews. 2017. Vol. 89, p. 780-807. DOI: 10.1016/j.oregeorev.2017.06.025
- Moore M., Chakhmouradian A.R., Mariano A.N., Sidhu R. Evolution of rare-earth mineralization in the Bear Lodge carbonatite, Wyoming: Mineralogical and isotopic evidence. Ore Geology Reviews. 2015. Vol. 64, p. 499-521. DOI: 10.1016/j.oregeorev.2014.03.015
- Kozlov E., Fomina E., Sidorov M. et al. The Petyayan-Vara Carbonatite-Hosted Rare Earth Deposit (Vuoriyarvi, NW Russia): Mineralogy and Geochemistry. Minerals. 2020. Vol. 10. Iss. 1. N 73. DOI: 10.3390/min10010073
- Kozlov E.N., Fomina E.N. Mass balance of complementary metasomatic processes using isocon analysis. MethodsX. 2022. Vol. 9. N 101609. DOI: 10.1016/j.mex.2021.101609
- Fomina E.N., Kozlov E.N. Stable (C, O) and radiogenic (Sr, Nd) isotopic evidence for REE-carbonatite formation processes in Petyayan-Vara (Vuoriyarvi massif, NW Russia). Lithos. 2021. Vol. 398-399. N 106282. DOI: 10.1016/j.lithos.2021.106282
- Karchevsky P.I., Moutte J. The phoscorite-carbonatite complex of Vuoriyarvi, northern Karelia. Phoscorites and carbonatites from mantle to mine: the key example of the Kola alkaline province. London: Mineralogical Society of Great Britain and Ireland, 2004, 163-199. DOI: 10.1180/MSS.10.06
- Sarvaramini A., Azizi D., Larachi F. Hydroxamic acid interactions with solvated cerium hydroxides in the flotation of monazite and bastnäsite – Experiments and DFT study. Applied Surface Science. 2016. Vol. 387, p. 986-995. DOI: 10.1016/j.apsusc.2016.07.044
- de Souza A.L., de Albuquerque R.O., Lameiras F.S. et al. Use of depressants in the direct flotation of a silicate-carbonate phosphate ore. Rem: Revista Escola de Minas. 2014. Vol. 67. N 2, p. 191-196. DOI: 10.1590/s0370-44672014000200010