Organotin pollutants in emerging coastal-marine sediments of the Kaliningrad shelf, Baltic Sea
- 1 — Ph.D. Leading Researcher Saint Petersburg Federal Research Center of the RAS ▪ Orcid ▪ Scopus
- 2 — Researcher Saint Petersburg Federal Research Center of the RAS ▪ Orcid ▪ Scopus
- 3 — Junior Researcher Saint Petersburg Federal Research Center of the RAS ▪ Orcid
- 4 — Ph.D. Head of Department A.P.Karpinsky Russian Geological Institute ▪ Orcid ▪ Scopus
- 5 — Ph.D. Senior Researcher A.P.Karpinsky Russian Geological Institute ▪ Orcid ▪ Scopus
Abstract
Based on two years of monitoring of modern bottom sediments of two sections of the Kaliningrad shelf of the Baltic Sea – “Curonian Spit” and “Northern Sambian” – an assessment of the sources of pollution with organotin compounds (OTs) and heavy metals was carried out. The content of individual organotin compounds and OTCs spectra obtained by gas chromatography with mass spectrometry of relatively coarse-grained bottom sediments indicate the presence of organotins in significant quantities – the total OTs content (ΣOTs) is from 0.6 to 8.3 ng/g. However, the content of tributyltin (TBT), the main component of anti-fouling systems for marine vessels and the most dangerous endocrine-disrupting compound among the hazardous substances for marine ecosystems, is at a low level (0-2.3 ng/g) in all studied samples and has not increased over the two-year observation period (biodegradation index 1.7-12.4). At the same time, the presence of abnormally high concentrations of mono-, triphenyl- and tricyclohexyltin in the sediments of the ”Northern Sambian” site (up to 30, 7 and 6.4 ng/g, respectively) indicates an additional source of pollution of coastal waters and shelf sediments (for example, plastic litter and agricultural runoff). The absence of significant shipping in the study areas ensures a consistently low level of pollution with tributyltin and its derivatives (less than 0.3 and 2.3 ng/g of TBT in 2017 and less than 0.1 and 1.3 ng/g in 2018 for the sites “Curonian Spit” and “Northern Sambian”, respectively), which indicates the activity of the processes of TBT transformation and self-cleaning of sandy sediments. However, the identified trends and their predictive accuracy require long-term observation and monitoring of the sediment environment using data on the deep-water part of the shelf, enriched in clay and humus components.
Funding Bottom sediment sampling was carried out during field work under the project “State monitoring of subsoil of the coastal-shelf zone of the Baltic, White and Barents Seas”. The work was carried out within the framework of the state assignment of the Ministry of Science and Higher Education of the Russian Federation (N 122041100086-5).
Introduction
Organotin compounds (OTs) are substances highly in demand by modern industry and are used throughout the world as stabilizers for PVC and in the production of polyurethanes, silicones, paints and pesticides [1-3]. Until recently, tributyltin compounds (TBT) were widely used as biocide components of anti-fouling systems in shipbuilding [4]. However, since the 70s of the last century, the harmful effects of these components, which are endocrine-disrupting compounds [5], on non-target marine biota have become obvious: the appearance of male sexual characteristics in female marine snails and mollusks, gastropods and bivalves; disorders of reproduction and growth in mussels; abnormalities of calcification of oyster and crustacean shells; immunological dysfunctions in fish [6]. At the same time, TBT has high toxicity (chronic) even in extremely low concentrations (1 ng/l), and its presence in the marine ecosystem has a serious impact at all levels of the food chain – from phytoplankton [7] to marine mammals [8], as well as potentially dangerous for humans – natural steroid/receptor binding and steroid signaling are disrupted, leading to excess obesity [5].
Currently, the problem of pollution of marine and freshwater areas with organotin compounds is relevant for the whole world – from Europe [3, 9, 10], Asia [8, 11], Africa, North and South America [5, 12] to the Arctic and Antarctic [13]. Some countries have introduced strict standards regarding the content of TBT in water, sediments and biota. In 2012, the Russian Federation acceded to the International Convention for the Control of Noxious Anti-fouling Systems on Ships (AFS Convention), which prohibits or restricts the use of any anti-fouling systems1. Earlier, in 1998, the Russian Federation, having become a member of the Helsinki Commission (HELCOM), ratified the Convention for the Protection of the Marine Environment of the Baltic Sea Area. The Convention established a list of priority hazardous marine pollutants, which included TBT and its derivatives2. However, despite the introduction of international restrictions on use, the level of OTs content in modern sediments remains high over the past decades, including in the Baltic Sea region [14, 15]. Recent studies have confirmed the high content of TBT in bottom sediments along the fairway and port areas of the Gulf of Finland [16].
The accumulation of OTs in bottom sediments is an acute problem for the marine environment and is determined by the influx of technogenic pollution, its stability and the rate of degradation in bottom sediments. If in the aquatic environment the half-life of OTs is several days, then in bottom sediments this process can last several years [14, 17]. Understanding which environmental factors and how they influence the distribution and accumulation of organotin compounds in marine sediments is necessary to predict the situation with these types of pollutants in specific regions.
Previously, the contamination of bottom sediments with organotins and heavy metals in the area of the islands of Gogland and Moshchny in the eastern part of the Gulf of Finland was studied, and the influence of environmental conditions was assessed – the nature of sediments, particle size distribution, the presence of certain chemical elements and inorganic compounds, gas saturation, the presence of anoxic zones – on the distribution and mobility of OTs in the sediments of this region [18].
An increase in the OTs content in gas-saturated zones of sediments and a decrease in their content with increasing alkalinity of sediments were discovered. At the same time, in the anoxia zone, a twofold increase in the content of OTs was recorded, and a tenfold increase in tetrabutyltin compared to the aerobic zone. This indicates the stimulating role of oxygen in the biotic degradation of OTs and the negative impact of the constantly expanding anoxic zones of the Baltic Sea on the processes of degradation of accumulated organotins.
A direct correlation has been established between the content of OTs and heavy metals and metalloids in fine-grained sediments. Analysis of the profiles of organotin compounds in samples taken over a two-year period in the eastern part of the Gulf of Finland showed the greatest contribution of tributyltin, the high level of which and low values of the degradation coefficient indicate the presence of a permanent source of pollution [18].
The purpose of the work is to study the state of contamination with organotins and heavy metals in modern sediments of the shelf areas of the Baltic Sea in the Kaliningrad region under anthropogenic loads that differ significantly from the Gulf of Finland region. Particular attention is paid to studying the influence of the granulometric characteristics of the sediment on the accumulation of OTs, the processes of sorption and binding of pollutants in the sediment, as well as the degradation of TBT and the possible self-purification of the sediment over time.

Fig.1. Sampling points on the lithological map (according to [19]) of the Curonian Spit region
1 – coarse сlastic sediments; 2 – medium-grained sand; 3 – fine-grained sand; 4 – silty sand; 5 – pelitic alevrite; 6 – outcrops of Quaternary sediments; 7 – sand of various granulometric compositions; 8 – sampling point and number
Methods
The samples were taken by specialists from the A.P.Karpinsky Russian Geological Institute during the 2017-2018 field seasons on the “Nord-3” vessel using a box corer within the Kaliningrad shelf in areas north of the Curonian Spit and near the northern coast of the Sambian Peninsula. All samples taken (depth 0-5 cm) are sands of various granulometric compositions from coarse-grained to fine-grained. On lithological maps, sampling points fall within the sand development fields (Fig.1 and 2). A total of 23 samples were selected for analysis.
Geological settings. The Kaliningrad shelf belongs to the region of the platform cover, represented by sedimentary rocks of the East European Platform (carbonate and clastic), the age of the rocks is from Devonian to Paleogene. The bedrock is overlain by Quaternary sediments (clays, sands, boulders) no more than 30 m thick [19]. The studied areas (Curonian Spit and the northern coast of the Sambian Peninsula) are located in shallow coastal waters with alternating denudation and accumulation surfaces – in places of wave erosion moraine deposits are exposed (boulder loams, sands with gravel and pebbles, bedrock outcrops), in places of wave accumulation – sands are predominantly widespread. Most of the studied samples were taken in places of wave accumulation, which is visually confirmed by the presence of a typical wave relief on the surface of sediments.

Fig.2. Sampling points on the lithologic map (by [19]) of the northern coast of the Sambian Peninsula
1 – coarse сlastic sediments; 2 – pebble-gravel sand; 3 – coarse – to very coarse-grained sand; 4 – medium-grained sand; 5 – fine-grained sand; 6 –outcrops of Quaternarysediments;7 – sampling point and its number
Quantitative assessment of the content of cation-organic tin compounds in bottom sediments – monobutyltin (MBT), dibutyltin (DBT), tributyltin (TBT), tetrabutyltin (TTBT), triphenyltin (TPhT) and tricyclohexyltin (TCyT) – was carried out using gas chromatography/mass-spectrometry (GC/MS) in accordance with the analytical procedure developed by the authors [20] based on the ISO 23161:20183 standard. Samples of bottom sediments with natural moisture were thoroughly mixed, homogenized, after which a sample weighing 1-3 g was taken, depending on the type of sediment. A 1:1 mixture of methanol and glacial acetic acid was then added to the sample, along with 50 ng of internal standard (tripropyltin) and ultrasonically extracted for 60 min. After centrifugation, acetate buffer (pH 4.5) was used and a derivatizing agent (sodium tetraethylborate) and hexane were added to the extract. The resulting mixture was stirred with a magnetic stirrer for 30 min. The hexane layer was then separated, dried over anhydrous sodium sulfate, and concentrated under a stream of nitrogen to a volume of 50 μL.
The analysis of the main derivatives was carried out in SIM (Selection ion monitoring) mode on a QP 2010 gas chromato-mass-spectrometer (Shimadzu, Japan). Parameters for recording spectra using the GC/MS method: ion source temperature – 200 °C, interface – 300 °C, injector– 300 °C; mode with constant gas carrier flow (helium) 1 ml/min; ionization energy 70 eV. The analysis was carried out on a capillary column of medium polarity TR-5 MS (60 mm ´ 0.25 mm ´ 0.25 μm, Thermo Electron, USA) according to the following temperature program: from 60 °C (2 min); heating 20 °C/min – up to 130 °C; 10 °C/min – up to 210 °C; 5 °C/min – up to 260 °C; 7 °C/min – up to 300 °C (holding time 10 min). The volume of the injected sample was 1 μl, the total analysis time was about 40 min.
Analytes were identified by two characteristic ions taking into account precise retention times. The mass fraction of each compound was calculated using the internal standard method using the organic tin cation conversion factors given in the ISO 23161:2018 method. The quantitative detection limit at the specified GC/MS parameters was 0.5 ng/g (recalculate to the dry weight). The suitability of the applied analytical technique was assessed before each series of analyses by adding control samples. Solvent control was carried out under the same concentration and chromatography conditions as for the samples under study.
In the Central Laboratory of the A.P.Karpinsky Russian Geological Institute the researchers measured the concentrations of Sr, Pb, As, Zn, Cu, Ni, Co, V, Cr, MnO, Fe2O3, TiO2 in 12 samples from the 2017 collection using the ICP-MS method (Agilent-7700 and Elan-DR-e) with preliminary acid decomposition of the sample (Co, Ni, Cu, Zn, Pb in a mixture of HNO3 + HF + HClO4 or HNO3 + HCl for As) or fusion with lithium metaborate (MnO, Fe2O3, TiO2, Sr, V, Cr), according to the approved method N10/2010 “Determination of macro- and microelements by mass spectrometry with inductively coupled plasma in rocks, ores, soils and soft sediments”.
The assessment of the granulometric characteristics of the sediment was carried out using a laser particle analyzer “Microsizer 201A” (VA Instal, Saint Petersburg, Russian Federation, range 1-300 µm). Granulometric analysis of samples was carried out in an aqueous suspension according to the standard method for a sample of 100 g, after stirring up of which weight measurements were carried out according to 21 size classes with registration of particles in the range of > 2.5 mm till < 0.01 mm in diameter. The results, presented by the characteristic particle size distribution curves for each sample (Fig.3), allow us to classify them according to sediment types – from very-coarse-grained (Svc) to very-fine-to-fine-grained (Svff) sands.

Fig.3. Particle distribution profiles by size class (granulometry) for sandy sediments of the studied samples:а – typical unimodal distribution (sample 17-KK-2), characteristic of most samples from the “Curonian Spit” site (2017, 2018);
b – typical bimodal distribution of particles (sample 17-CC-2), characteristic of the studied sediment samples from the “Northern Sambian” site (2017, 2018); c – polymodal distribution (anomalous) for sample 18-CC-1 (“Northern Sambian”, 2018)
The significance of the relationship between all studied parameters (OTs, granulometry and elemental composition) of the samples was assessed based on correlation analysis using the Spearman method (calculation and construction of a matrix of paired correlations, p < 0.05 (probability > 95 %) was set to identify a significant relationship) using the library Scipy, implemented in Python.
To assess the ecological state of the environment of the emerging coastal sandy sediment of the Kaliningrad shelf, several complex indicators were used. The butyltin degradation index (BDI) is often used to assess the novelty of TBT pollution sources [2, 12, 15]. The calculation is based on the ratio of the concentrations of the two main decomposition products (DBT and MBT) and the parent compound (TBT): BDI = (DBT + MBT)/TBT. BDI values below 1 indicate recent input of organotins into the accumulation area or low levels of biodegradation in the body [3, 17]. BDI values greater than one suggest progressive degradation of TBT deposited in the past. However, in coastal regions adjacent to river mouths and estuaries, BDI should be used with caution because DBT and MBT may come directly from other sources (e.g., domestic and industrial wastewater discharges) [17].
To assess natural or anthropogenic sources of heavy metals in sediments, various enrichment factors (EF) are often used, which are calculated relative to conservative elements such as Al, Fe, Sc, and Ti as reference elements, as well as a geoaccumulation index which allows assessing the accumulation of metals in bottom sediments by comparing current and pre-industrial concentrations, where Сx – are the measured concentrations of metals in the sediment fraction; Сb – geochemical background (concentration) of metals in global shale.
A coefficient of 1.5 is introduced to take into account possible changes in the background value due to natural fluctuations (effect of lithology). In this case, an estimated classification of the geoaccumulation index is used according to the level of potential environmental pollution: Igeo ≤ 0 – absence of pollution; 0 < Igeo ≤ 1 – minor to moderate contamination; 1 < Igeo ≤ 2 – moderate pollution; 2 < Igeo ≤ 3 – from moderate to heavy pollution; 3 < Igeo ≤ 4 – heavy pollution; 4 < Igeo ≤ 5 – from strong (heavy) to extreme and Igeo > 5 – extreme pollution.
Results
Granulometry of sediments. All studied samples (23 samples) are represented by medium-to-coarse-grained sands with a minimal proportion of clay component (less than 1%), which are characterized by a predominantly unimodal distribution of particle grain size – from 0.05 to 1.65 mm (Tables 1 and 2). There are samples with bimodal and more complex distributions (Fig.3). Obviously, this distribution pattern depends on the conditions of sediment formation at the sampling point, the presence of certain complicating relief forms, as well as local along-shore currents, which can be an additional source of sandy material. However, the main process that forms the sediment is wave surf, as well as tidal currents, which lead to the formation of fairly well washed and sorted sandy sediments.
Since the area and depth varied slightly, the particle size distribution of the 2017 and 2018 samples from each site is similar. Thus, for the “Curonian Spit” site in 2017, out of six studied samples, five were represented by fine-medium-grained sands with grain sizes of 0.25-0.125 mm, and one was coarse-grained sand with grains of 0.8-0.63 mm, and in 2018, out of five samples, four are represented by the same fine-medium-grained sand (0.25-0.125 mm), and one is very-coarse to coarse-grained sand with a particle size of 1.0-0.8 mm (Fig. 3, a).
At the “Northern Sambian” sampling site, samples in 2017 are represented by very-coarse to coarse-grained sands (four samples, 1.6-0.8 mm) and fine-medium-grained sands (three samples, 0.4-0.1 mm), and in 2018 – out of five samples, two are represented by very-coarse to coarse-grained sands (1.6-0.4 mm) and two fine-medium-grained sands (0.4-0.1 mm) (Fig. 3, b).
Another sample (Fig.3, c) has an anomalous particle size distribution with a wide range of 0.6-0.04 mm. It is obvious that the sampled deposits of the “Northern Sambian” site are distinguished by a larger proportion (representativeness) of coarse-grained sands compared to samples from the “Curonian Spit” site.
Samples from the “Northern Sambian” site are characterized by the presence of more complex types of particle grain-size distribution (bimodal, anomalous) compared to the sands of the site “Curonian Spit”, which are characterized by different, but exclusively unimodal distributions. This difference may be due not only to different conditions of sediment formation, but also, in part, to the low representativeness of the samples. However, the sampling sites are characterized by a comparable number of samples (11 and 12), and the granulometric characteristics of the sediments are consistently reproduced for the samples of 2017 and 2018, which may indicate the real differences between the sandy sediments of the two studied sites.
Organotin compounds. The results of a study of bottom sediments of the Kaliningrad shelf (11 samples from the “Curonian Spit” site and 12 samples from the “Northern Sambian” site) demonstrated the presence of detectable amounts of organotin compounds in all but one samples, 18-KK-5, from 0.6 to 32 ng/g (dry weight – d.w.).
Table 1
Granulometric characteristics (distribution by size class, mm) of bottom sediments of the 2017 sampling sites (in % per 100 g of the initial sample weight)
Sample |
Water depth, m |
Sediment type |
>2.5 |
2.5-2.0 |
2.0-1.6 |
1.6-1.25 |
1.25-1.0 |
1.0-0.8 |
0.8-0.63 |
0.63-0.5 |
0.5-0.4 |
0.4-0.315 |
0.315-0.25 |
0.25-0.2 |
0.2-0.16 |
0.16-0.125 |
0.125-0.1 |
0.1-0.08 |
0.08-0.063 |
0.063-0.05 |
0.05-0.04 |
0.04-0.01 |
<0.01 |
|
17-КК-1 |
19.5 |
Sf |
0 |
0 |
0 |
0 |
0.02 |
0.14 |
0.30 |
0.12 |
0.14 |
0.64 |
2.98 |
6.02 |
11.71 |
26.84 |
27.35 |
18.7 |
2.74 |
0.58 |
0.08 |
0.14 |
0 |
|
17-КК-2 |
19.9 |
Sc |
5.6 |
1.98 |
2.14 |
5.10 |
7.07 |
16.9 |
37.6 |
16.2 |
2.18 |
2.00 |
1.61 |
0.79 |
0.42 |
0.22 |
0.02 |
0 |
0 |
0 |
0 |
0 |
0 |
|
17-КК-3 |
13.7 |
Sf |
0 |
0 |
0 |
0.02 |
0.14 |
0.66 |
1.03 |
2.91 |
1.89 |
4.32 |
11.0 |
21.6 |
23.6 |
18.6 |
8.60 |
3.78 |
1.07 |
0.22 |
0.08 |
0.06 |
0.44 |
|
17-КК-5 |
17.2 |
Sf |
0 |
0 |
0 |
0 |
0.04 |
0.10 |
0.24 |
0.52 |
1.22 |
5.73 |
19.8 |
32.1 |
23.0 |
12.0 |
2.77 |
1.30 |
0.36 |
0.08 |
0.02 |
0.02 |
0.68 |
|
17-КК-6 |
16.2 |
Smf |
0 |
4.53 |
0.71 |
0.73 |
0.79 |
0.77 |
0.63 |
1.29 |
1.98 |
5.78 |
10.6 |
13.3 |
13.1 |
19.6 |
14.2 |
7.66 |
1.90 |
0.59 |
0.06 |
0.36 |
1.46 |
|
17-КК-7 |
14.5 |
Smf |
0 |
0.06 |
0.02 |
0.02 |
0.06 |
0.18 |
0.36 |
1.19 |
2.59 |
7.31 |
11.7 |
17.6 |
21.2 |
18.2 |
8.97 |
6.87 |
2.24 |
0.46 |
0.02 |
0.14 |
0.8 |
|
17-СС-1 |
25.6 |
Scvc |
0 |
1.62 |
3.55 |
22.6 |
39.0 |
10.6 |
1.72 |
1.68 |
2.12 |
5.09 |
6.34 |
3.78 |
1.07 |
0.24 |
0.08 |
0.12 |
0.04 |
0.02 |
0.02 |
0.02 |
0.28 |
|
17-СС-2 |
25.4 |
Scvc |
0 |
0.16 |
0.36 |
3.88 |
34.2 |
22.0 |
4.06 |
7.79 |
8.57 |
8.87 |
5.47 |
2.78 |
0.89 |
0.26 |
0.08 |
0.02 |
0.04 |
0.02 |
0.02 |
0.06 |
0.44 |
|
17-СС-3 |
25.0 |
Scvc |
0 |
0.58 |
2.47 |
5.95 |
38.9 |
22.2 |
4.10 |
7.24 |
4.73 |
5.55 |
4.81 |
2.31 |
0.58 |
0.04 |
0.02 |
0.02 |
0 |
0 |
0 |
0 |
0.52 |
|
17-СС-5 |
19.0 |
Svcc |
0 |
0.79 |
0.44 |
2.05 |
35.3 |
46.7 |
4.89 |
2.92 |
1.81 |
1.93 |
0.50 |
0.50 |
0.50 |
0.50 |
0.04 |
0.02 |
0 |
0 |
0 |
0 |
1.12 |
|
17-СС-7 |
21.4 |
Svff |
0 |
0.64 |
0.48 |
0.74 |
2.23 |
1.89 |
0.92 |
1.29 |
1.81 |
2.75 |
5.66 |
9.10 |
7.49 |
7.85 |
7.35 |
24.1 |
12.2 |
4.96 |
0.80 |
3.48 |
4.18 |
|
17-СС-9 |
6.4 |
Sm |
0 |
0.04 |
0.10 |
0.12 |
0.28 |
0.74 |
1.67 |
9.70 |
33.1 |
45.3 |
6.92 |
0.87 |
0.18 |
0.12 |
0.24 |
0.26 |
0.14 |
0.02 |
0.02 |
0.02 |
0.18 |
|
17-СС-10 |
17.9 |
Smc |
0 |
0.16 |
0.24 |
0.50 |
1.75 |
12.7 |
19.9 |
22.0 |
13.6 |
13.6 |
9.72 |
3.81 |
1.15 |
0.24 |
0.16 |
0.02 |
0.02 |
0.02 |
0.02 |
0 |
0.22 |
Note. КК – Curonian Spit; СС – Northen coast of Sambian Peninsula. Sediment types: Suf – unsorted, mostly fine-grained sand; Svc – very coarse-grained sand; Scvc – coarse to very coarse-grained sand; Svcc – sand very coarse to coarse-grained sand; Sc – coarse-grained sand; Smc – medium to coarse-grained sand; Scm – coarse to medium-grained sand; Sm – medium-grained sand; Sfm – fine to medium-grained sand; Smf – medium to fine-grained sand; Sf – fine-grained sand; Svff – very fine to fine-grained sand.
Table 2
Granulometric characteristics (distribution by size class, mm) of bottom sediments of the 2018 sampling sites (in % per 100 g of the initial sample weight)
Sample |
Water depth, m |
Sediment type |
>2.5 |
2.5-2.0 |
2.0-1.6 |
1.6-1.25 |
1.25-1.0 |
1.0-0.8 |
0.8-0.63 |
0.63-0.5 |
0.5-0.4 |
0.4-0.315 |
0.315-0.25 |
0.25-0.2 |
0.2-0.16 |
0.16-0.125 |
0.125-0.1 |
0.1-0.08 |
0.08-0.063 |
0.063-0.05 |
0.05-0.04 |
0.04-0.01 |
<0.01 |
18-КК-2 |
17.3 |
Smf |
0 |
0 |
0 |
0 |
0.02 |
0.1 |
0.44 |
3.42 |
3.64 |
8.4 |
18.1 |
24.3 |
18.92 |
14.38 |
5.18 |
1.86 |
0.54 |
0.24 |
0.1 |
0.04 |
0.28 |
18-КК-3 |
19.5 |
Svff |
0.02 |
0 |
0 |
0.04 |
0.02 |
0.3 |
0.74 |
0.38 |
0.44 |
1.72 |
5.17 |
8.74 |
13.7 |
29.0 |
22.4 |
12.1 |
3.09 |
0.96 |
0.28 |
0.14 |
0 |
18-КК-4 |
20.0 |
Sc |
0.02 |
0.02 |
0.04 |
0.30 |
3.24 |
35.8 |
29.2 |
4.62 |
0.58 |
1.16 |
2.24 |
1.82 |
1.50 |
3.54 |
6.91 |
6.59 |
1.36 |
0.44 |
0.14 |
0.08 |
0.38 |
18-КК-5 |
13.9 |
Sf |
0 |
0.02 |
0 |
0.02 |
0.02 |
0.14 |
0.26 |
0.66 |
1.28 |
4.60 |
11.5 |
18.5 |
23.4 |
19.0 |
8.20 |
7.24 |
3.30 |
1.02 |
0.32 |
0.16 |
0.36 |
18-КК-7 |
15.4 |
Sf |
0 |
0.04 |
0.04 |
0.04 |
0.14 |
0.3 |
0.42 |
0.94 |
1.16 |
3.42 |
10.7 |
24.1 |
25.9 |
20.8 |
6.87 |
3.20 |
1.22 |
0.42 |
0.14 |
0.01 |
0.08 |
18-СС-1 |
8.5 |
Suf |
0 |
0 |
0.02 |
0.02 |
0.04 |
0.25 |
0.99 |
3.09 |
5.55 |
11.5 |
9.93 |
5.49 |
4.59 |
12.2 |
10.75 |
22.3 |
4.95 |
1.13 |
6.59 |
0.17 |
0.44 |
18-СС-2 |
17.5 |
Sfm |
0 |
0 |
0.02 |
0.10 |
0.42 |
2.26 |
3.48 |
5.22 |
9.49 |
25.2 |
28.1 |
15.4 |
5.20 |
1.62 |
0.72 |
1.20 |
0.78 |
0.44 |
0.14 |
0.06 |
0.16 |
18-СС-7 |
19.2 |
Scvc |
0.98 |
0.62 |
0.96 |
10.6 |
35.6 |
15.2 |
6.87 |
8.29 |
7.03 |
7.35 |
3.34 |
1.42 |
0.50 |
0.18 |
0.10 |
0.24 |
0.24 |
0.2 |
0.1 |
0.1 |
0.06 |
18-СС-9 |
24.2 |
Scvc |
0.98 |
0.62 |
0.96 |
10.6 |
35.6 |
15.2 |
6.87 |
8.29 |
7.03 |
7.35 |
3.34 |
1.42 |
0.50 |
0.18 |
0.10 |
0.24 |
0.24 |
0.2 |
0.1 |
0.1 |
0.06 |
The total content of six OTs (ΣOTs = MBT + DBT + TBT + TTBT + TPhT + TCyT) varies over a wide range of values both for samples from one sampling site and between sites (Tables 3, 4). Thus, for samples taken in 2017 at the “Curonian Spit” site, ΣOTs varies from 0.6 to 5.0 ng/g d.w. with an average value of 1.83±1.71 (1σ). In this case, the main contribution is made by MBT (av. 0.93±0.59 ng/g d.w.), TPhT (av. 0.61±1.27 ng/g d.w.) and TTBT (av. 0.23±0.38 ng/g d.w.), while the TCyT in all samples from the site is not higher than the detection limit.
For samples from the “Northern Sambian” site from the 2017 collection, the level of ΣOTCs is significantly higher and varies from 1.3 to 32.4 ng/g d.w. with an average of 4.25±2.7 ng/g d.w., with the dominant components of TBT (av. 1.04 ± 0.84 ng/g d.w.), TCyT (av. 1.06±1.4 ng/g d.w.), MBT (av. 0.85±0.41 ng/g d.w.) and subordinate but significant TTBT (av. 0.67±0.37 ng/g d.w.) and DBT (av. 0.53±0.52 ng/g d.w.). Sample 17-CC-8 is anomalous both in terms of the total OTs content (32.4 ng/g d.w.) and the OTs spectrum: MBT – 30.0, DBT – 0.4, TBT – 1.8 ng/g d.w.
Table 3
Organotin compounds (ng/g d.w.) in the sands of the sampling sites in 2017
Sample |
MBT |
DBT |
TBT |
ТТBT |
ТPhT |
ТCyT |
∑OTs |
17-КК-1 |
0.60 |
<0.1 |
< 0.1 |
< 0.1 |
< 0.1 |
< 0.1 |
0.60 |
17-КК-2 |
0.40 |
0.10 |
0.30 |
< 0.1 |
0.20 |
< 0.1 |
1.00 |
17-КК-3 |
0.40 |
0.10 |
< 0.1 |
0.20 |
< 0.1 |
< 0.1 |
0.70 |
17-КК-5 |
0.90 |
0.20 |
< 0.1 |
< 0.1 |
< 0.1 |
< 0.1 |
1.10 |
17-КК-6 |
1.50 |
< 0.1 |
< 0.1 |
1.00 |
0.10 |
< 0.1 |
2.60 |
17-КК-7 |
1.80 |
< 0.1 |
< 0.1 |
< 0.1 |
3.20 |
< 0.1 |
5.00 |
17-СС-1 |
0.60 |
0.10 |
< 0.1 |
0.90 |
< 0.1 |
< 0.1 |
1.60 |
17-СС-2 |
0.30 |
< 0.1 |
< 0.1 |
1.00 |
< 0.1 |
< 0.1 |
1.30 |
17-СС-3 |
1.50 |
0.20 |
1.30 |
0.30 |
0.10 |
0.60 |
4.00 |
17-СС-7 |
1.10 |
1.50 |
2.30 |
0.60 |
< 0.1 |
3.00 |
8.50 |
17-СС-8 |
30.00 |
0.40 |
1.80 |
0.20 |
< 0.1 |
< 0.1 |
32.4 |
17-СС-9 |
0.80 |
0.20 |
0.90 |
0.50 |
0.30 |
3.20 |
5.90 |
17-СС-10 |
0.80 |
0.80 |
0.90 |
1.20 |
< 0.1 |
0.50 |
4.20 |
Table 4
Organotin compounds (ng/g d.w.) in the sands of the sampling sites in 2018
Sample |
MBT |
DBT |
TBT |
ТТBT |
ТPhT |
ТCyT |
∑OTs |
18-КК-2 |
1.30 |
< 0.1 |
< 0.1 |
0.20 |
< 0.1 |
< 0.1 |
1.50 |
18-КК-3 |
1.30 |
< 0.1 |
< 0.1 |
< 0.1 |
< 0.1 |
< 0.1 |
1.30 |
18-КК-4 |
2.00 |
0.40 |
< 0.1 |
< 0.1 |
< 0.1 |
< 0.1 |
2.40 |
18-КК-5 |
< 0.1 |
< 0.1 |
< 0.1 |
< 0.1 |
< 0.1 |
< 0.1 |
< 0.1 |
18-КК-7 |
2.90 |
0.90 |
< 0.1 |
< 0.1 |
0.10 |
< 0.1 |
3.80 |
18-СС-1 |
2.80 |
< 0.1 |
< 0.1 |
< 0.1 |
< 0.1 |
< 0.1 |
2.80 |
18-СС-2 |
4.90 |
0.70 |
< 0.1 |
< 0.1 |
< 0.1 |
< 0.1 |
5.60 |
18-СС-5 |
3.60 |
2.30 |
0.70 |
< 0.1 |
< 0.1 |
< 0.1 |
6.60 |
18-СС-7 |
4.90 |
3.40 |
< 0.1 |
< 0.1 |
< 0.1 |
< 0.1 |
8.30 |
18-СС-9 |
1.70 |
0.50 |
1.30 |
1.30 |
7.00 |
6.40 |
18.2 |
The composition and content of organotin compounds of the 2018 samples is significantly different. For the “Curonian Spit” site the level of ΣOTs is higher than in the samples of 2017 (1.3-8.3 ng/g d.w.), but the average value does not change (1.81±1.4 ng/g d.w.). The spectrum of compounds is limited to only two components – with the largest contribution of MBT (av. 1.51±1.0 ng/g d.w.) and a detectable amount of DBT (av. 0.29±0.37 ng/g d.w.), the other four components (TBT, TTBT, TPhT, TCyT) turned out to be below the sensitivity threshold of the analysis.
For the “Northern Sambian” sampling site, the total OTs content of the 2018 samples ranged from 2.8 to 18.2 ng/g dry weight (av. 5.83±2.3 ng/g d.w.) with the highest contribution from MBT (av. 3.58±1.38 ng/g d.w.), DBT (av. 1.39±1.4 ng/g d.w.) and TBT (av. 0.43±0.56 ng/g d.w.). The other components of OTs (TCyT, TPhT, TTBT) are below the detection limit. However, in the composition of the OTs of sample 18-CC-9 these components were in the highest concentration: TCyT – 6.4 ng/g d.w., TPhT – 7.0 ng/g d.w., and TTBT up to 1.3 ng/g d.w., and the total OTCs content was maximum – 18.2 ng/g d.w. (with TBT – 1.3, DBT – 0.5, MBT – 1.7 ng/g d.w.).
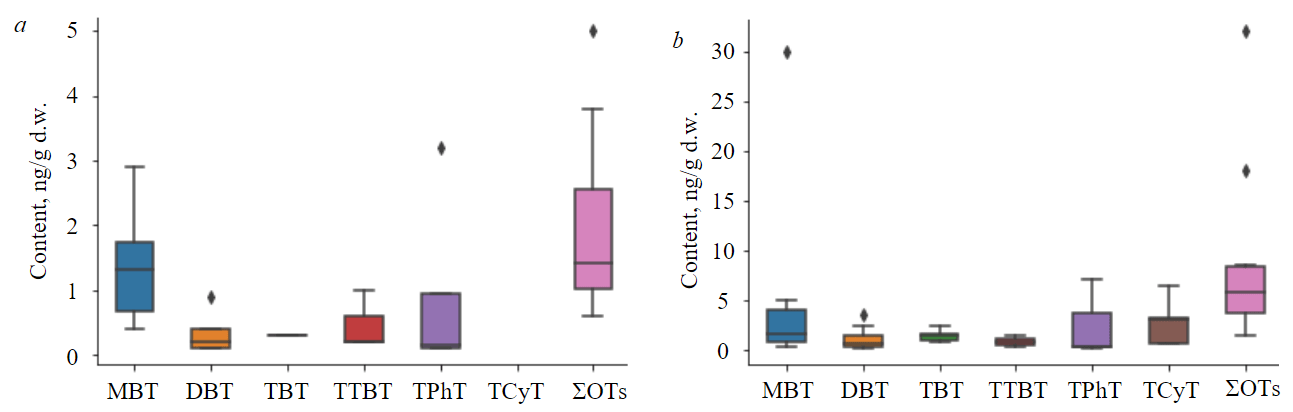
Fig.4. Distribution of the content of organotin compounds in sediments of the “Curonian Spit” (a) and “Northern Sambian” (b) sampling sites: monobutyltin, dibutyltin, tributyltin, tetrabutyltin, triphenyltin, tricyclohexyltin, and total
The range diagrams (Fig.4) show the distribution of OTCs contents in sediments. It is obvious that the content of ΣOTs in samples from the “Northern Sambian” site is statistically higher than in samples from the “Curonian Spit” site.
Analysis of the values of international regulations4 shows that the permissible level of TBT content in bottom sediments ranges from 0.01 to < 10 ng/g d.w. [11, 15], so none of the studied samples exceeded the recommended maximum value (10 ng/g d.w.). TPhT, TTBT and TCyT were detected in significant amounts only in the bottom sediments of the “Northern Sambian” site: 0.2-1.3, 0.1-7.0, and 0.1-6.4 ng/g d.w., respectively. The most probable source of their input into the aquatic ecosystems is water carriage, while MBT and DBT are formed to a large extent as a result of degradation of initial compounds – TBT and TTBT.
To assess the degree of TBT transformation in bottom sediments, the BDI coefficient is used – the ratio [MBT + DBT]/[TBT] [17]. If the value is greater than one, this indicates active processes of TBT transformation and self-purification of the aquatic ecosystem. For all the studied samples, the value of this coefficient was greater than one, indicating the efficiency of the deposit purification due to the TBT destruction. The change of this characteristic over the two-year observation period is also remarkable: BDI in the samples of the site “Curonian Spit” 2017 is characterized by the value of 1.7, while for the samples of 2018 this indicator reaches already 14-38, and in the samples of the site “Northern Sambian” it changes from 1.3 in 2017 to 12.4 in 2018. However, for the anomalous sample 18-СС-9, this index (BDI = 1.7) does not differ from the average for 2017 (av. 1.33), which, together with the anomalous structure of the OTs distribution for this sample, may indicate the input of organotins from an additional source, such as urban (Svetlogorsk) and/or agricultural runoff enriched with plastic waste and pesticide derivatives.

Fig.5. Average content of organotin compounds (Сav) depending on the granulometric type of sediment:total content of ΣOTs (а); MBT (b); ТТBT (c); DBT (d); ТCyT (e); TBT (f)
The total OTs content is the highest in the sands of the smallest grain size – very-fine to fine-grained (Fig.5, a). The greatest contribution to this result was the anomalously high content of MBT in the very-fine to fine-grained sand sampled at point 17-CC-8. Also in very-fine to fine-grained sands the increased content of TBT was recorded. At the same time, the maximum content of TCyT, TPhT and DBT is observed in very-coarse-grained and coarse- to very-coarse-grained sands, while the distribution of TTBT is more complex, but coarse-grained sands are characterized by relative enrichment with this component (Fig.5, b-f).
In order to reveal the relationship between the OTs content and sediment granulometry, Spearman’s pair correlation coefficients were calculated, since the samples are small (10-12 samples, see Tables 1, 2) and the normal law of distribution of values cannot be expected. The analysis of the calculated relationships was performed using the Scipy library, implemented in Python. The level of significant correlation was set at p < 0.05.
Visualization of the matrix of pair correlation coefficients is presented in Fig. 6 in the form of a heat map [21, 22]. High positive correlation characterizes the relationship of TBT with alevrite (r = 0.92) and pelitic fractions (r = 0.95). For TCyT and very coarse-grained sand r = 1, but only three pairs of values were used for the calculation, i.e. the result is unreliable. A high negative correlation was established for TBT and coarse-grained sand (r = –0.85). The results of the correlation analysis show that the concentration of TBT in sediments increases with increasing proportion of the fine-grained fraction (pelites and silts) and decreases with increasing proportion of coarse-grained sand. The positive relationship between TCyT content and the proportion of the very coarse-grained sand in the samples requires further investigation with an increase in the number of analyses and sample representativeness.

Fig.6. Spearman's correlation coefficient r. Circles indicate significant
(strong) correlation values, probability level p ≤ 0.05.

Fig.7. Scatter diagrams: content of TBT and coarse sand fraction (a), content of TBT and silt fraction (b).The red line shows the calculated regression line
Scatter plots of the most significant correlations between TBT and the proportion of coarse-grained sand and alevrite component are presented in Fig.7; there is a significant correlation (negative r = –0.926 (Fig.7, a) and positive r = 0.928 (Fig.7, b)). However, the correlation covers only a limited number of samples: six and four out of eight samples with TBT content above the detection limit. The results obtained are consistent with literature data indicating that sediments with a higher proportion of fine fractions adsorb TBT better [3, 10].
Element composition. The results of the analysis of the content of metals and oxides of 12 bottom samples from the 2017 collection are presented in Table 5. Samples from the “Curonian Spit” (KK) site are characterized by an increased metal contents compared to samples from the “Northern Sambian” site (CC): Ni – 2.56±0.4 μg/g (CC – 1.99±0.4), Cu – 1.91±0.4 (CC – 1.16±0.2), Zn – 25.1±6.4 (CC – 13.6±7.3), Pb – 7.34±1.1 (CC – 47±1.3), V – 24.8±4.1 (CC – 10.9±2.2), Sr – 58.3±8.6 (CC – 33.6±10.1). This can be compared with a larger share of fine-grained fraction in the sand deposits and a higher content of oxides: Fe2O3 – 1.78±0.3% (CC – 1.2±0.2 %) and TiO2 – 0.76±0.1 % (CC – 0.2±0.1 %). At the same time, the increased As – 6.65±5.3 µg/g (KK – 2.18±0.46 µg/g) correlates not only with the relative coarse-grained of the sediment, but also with an increased content of ΣOTs in samples from the “Northern Sambian” site. A similar pattern was previously noted when studying bottom sediments of the Gulf of Finland [18].
Table 5
Microelement and oxide contents in the sands of the sampling sites in 2017: “Curonian Spit” and “Northern Sambian”
Sample |
Co, μg/g |
Ni, μg/g |
Cu, μg/g |
Zn, μg/g |
Pb, μg/g |
TiO2, % |
V, μg/g |
Cr, μg/g |
MnO, % |
Fe2O3tot, % |
Sr, μg/g |
As, μg/g |
17-КК-1 |
2.00 |
2.98 |
1.77 |
25.0 |
9.03 |
0.98 |
31.2 |
61.0 |
0.03 |
2.22 |
70.60 |
2.99 |
17-КК-2 |
1.15 |
1.68 |
1.65 |
19.3 |
4.52 |
0.14 |
5.95 |
7.24 |
0.02 |
0.53 |
60.20 |
2.42 |
17-КК-3 |
1.55 |
2.25 |
1.39 |
16.7 |
6.45 |
0.70 |
22.0 |
60.9 |
0.03 |
1.55 |
53.70 |
1.85 |
17-КК-5 |
1.52 |
2.03 |
2.21 |
26.9 |
6.29 |
0.62 |
21.2 |
46.1 |
0.02 |
1.47 |
44.80 |
2.12 |
17-КК-6 |
1.85 |
3.02 |
2.50 |
34.4 |
7.52 |
0.82 |
26.5 |
66.7 |
0.03 |
1.92 |
61.10 |
1.90 |
17-КК-7 |
1.71 |
2.50 |
1.94 |
28.4 |
7.41 |
0.68 |
23.0 |
66.7 |
0.03 |
1.76 |
59.30 |
1.79 |
17-СС-2 |
2.17 |
2.22 |
1.09 |
22.2 |
5.37 |
0.16 |
11.2 |
11.5 |
0.04 |
1.73 |
36.70 |
10.70 |
17-СС-3 |
2.31 |
2.64 |
1.39 |
20.4 |
6.74 |
0.13 |
11.8 |
10.7 |
0.04 |
1.81 |
33.40 |
13.50 |
17-CC-5 |
1.30 |
1.83 |
1.01 |
10.3 |
3.90 |
0.11 |
8.63 |
9.37 |
0.02 |
0.80 |
29.50 |
5.24 |
17-СС-7 |
2.62 |
6.13 |
4.32 |
31.6 |
11.7 |
0.73 |
39.3 |
69.8 |
0.03 |
2.57 |
78.00 |
2.78 |
17-СС-9 |
1.22 |
1.76 |
<1 |
5.60 |
3.93 |
0.16 |
8.80 |
11.6 |
0.01 |
0.77 |
48.00 |
1.49 |
17-СС-10 |
1.07 |
1.50 |
<1 |
9.51 |
3.52 |
0.42 |
13.8 |
19.4 |
0.02 |
0.75 |
20.50 |
2.30 |
Detection limit |
0.5 |
1 |
1 |
1 |
1 |
0.001 |
2.5 |
1 |
0.0002 |
0.01 |
1 |
0.50 |
Discussion of the results
Environmental factors affecting the distribution and accumulation of organotin compounds in bottom sediments. The ability of bottom sediments to accumulate substances depends on the physicochemical characteristics of both the compounds themselves and the sediments (composition, particle size, organic matter). Nature and model studies have shown that the granulometric characteristics of sediments play a significant role in the sorption of OTs [10, 12, 22]. As the size of sediment granules decreases, their surface area increases, that leads to increased efficiency of adsorption of chemical compounds, including organotin compounds [23-25]. Fine-grained fractions of sediments adsorb more organic (humic) matter, which, in turn, accumulate OTs [24, 25]. The contribution of organic substances to the overall process of OTs sorption by bottom sediments can range from 55 to 85 % [24].
OTs belong to the group of organometallic compounds and therefore are characterized by many behavior features common to heavy metals in bottom sediments; they exhibit high sorption abilities for both the mineral and organic components of sediments. In this case, it is assumed that there is a competitive relationship for negatively charged sorption points (sites) on the surface of minerals between the TBT cation and divalent cations (Ba2+, Ca2+ and Mg2+). In addition, the competing influence of cations depends on their radii: larger cations (for example, Cs+) significantly reduce the sorption of TBT than smaller ones, for example Na+ [24]. The determining factors are the complexation of the corresponding cations with negatively charged ligands (with carboxyl and phenolic groups) and hydrophobic interactions [26, 27].
Positive or negative correlations between OTs and metal contents are determined by synergetic or competitive relationships in the process of sorption by bottom sediments; the toxic effect of metals on microorganisms involved in the biotic degradation of OTs; possible catalytic activity of metals in reactions of abiotic degradation of the OTs.
A factor regulating many processes occurring in water basins is also the pH value of the environment, which determines the form of the state of OTs, the rate of degradation, bioavailability, and sorption. Depending on the pH of the aquatic environment, OTs can be in different forms: ion – in the form of cations (if pH < pKa, constant of the acid dissociation) or neutral – in the form of hydroxyl complexes like TBTOH (if pH > pKa). Moreover, the value for TBT is pKa = 6.3 [28], and for TPhT pKa = 5.2 [26]. At a pH of 8, zooplankton concentrates OTs to the maximum, since 98 % of TBT and TPhT in this case are represented by neutral complexes (TBTOH and TPhTOH), which is preferable to the cationic form for penetration into living tissues [29]. The pH of the aquatic environment has a significant influence on the state of the sorbent surface. According to studies of the OTs sorption by various sorbents (mineral, organic, natural bottom sediments, etc.), the adsorption of TBT and DBT increases with increasing pH from 4 to 7 and decreases with a further increase in pH to alkaline conditions. While, the sorption of DBT on iron hydroxide decreases in the pH range from 6 to 8, and does not change on charcoal at pH 4-7. In contrast to TBT and DBT, the MBT sorption in bottom sediments onto iron hydroxide decreases when the pH changes from 6 to 8 [24].
The main OTs transformation occurs biotically [24, 26, 30] with the participation of various organisms, including bacteria [31], fungi [32] and algae [33]. It is known that biotic degradation occurs much faster under aerobic conditions than under anaerobic [31, 32, 34]. At the same time, the processes of OTs transformation occur predominantly in the pore water of bottom sediments, and the level of degradation of OTs in marine sediments is limited by desorption into pore waters, where their degradation occurs quite quickly – for TBT in aerobic conditions the half-life is 9.2 days, and for DBT in aerobic and anaerobic conditions – 2.9 and 9.1 days, respectively [24, 35].
Features of the accumulation of organotin compounds in bottom sediments of the Kaliningrad shelf. The main feature of the bottom sediments of the Kaliningrad shelf in the “Northern Sambian” and “Curonian Spit” areas is their relative coarse grain size (particle diameter 0.4-0.16 mm) with the presence of a small share of pelite-silty (less than 15%) and humus components, which explained by the formation conditions – coastal-marine deposits of the zone of surf-wave accumulation (depth 10-20 m below the sea surface). The constant wave action on the sand sediment ensures the formation of a monotonous sand fraction and prevents the sorption of both chemogenic and biogenic adsorbents on the surface of solid particles.
Despite the fact that the sampled sedimentary layer is in the aerobic zone, the conditions of its formation are not favorable for the accumulation of pollutants such as organotins and heavy metals. However, in all but one samples organotin compounds were found in significant quantities, the total content of which varied from 0.6 to 8.5 ng/g d.w., with maximum values for samples from the “Northern Sambian” site (32.4 and 18.2 ng/g d.w.). Moreover, the well-known [9] positive correlation between the content of TBT and the proportion of the silt-pelite fraction of the sand matrix (r = 0.95) is also well demonstrated. At the same time, for both sampling sites over a two-year observation period, the positive dynamics of tributyltin degradation is obvious: the BDI coefficient increases from 1.7 to 14-38 for the “Curonian Spit” and from 1.3 to 12.4 for the “Northern Sambian" sites. In addition, the content of TBT for the majority of the studied samples (15 out of 23) is below the detection limit and significantly lower than in adjacent areas of the Baltic Sea coast, as in the west (cf. for the Northern Sambian site 0.1-2.3 ng/g d.w., and for the area of the Szczecin Lagoon – from 2 to 3200 ng/g d.w., in the area of the National Nature Reserve of the Wřozovsky Bay, Oder River estuary – up to 80 ng/g d.w., 2017-2018 [21]), and in the east (Klaipeda port area: 0.5-333 ng/g d.w., Curonian Lagoon: 1-5 ng/g d.w., in the Baltic Sea, at a distance of 10 km from the coast – up to 20 ng/g d.w., 2019-2020 [22]). This indicates the efficiency of sediment self-purification in the studied areas and the absence of a significant tributyltin input as a component of anti-fouling paints.
Since the sampling sites are not an area of transit shipping, ship repair and construction, offshore sand mining and other industrial activities, this observation is expected and reflects a general decrease in organotin pollution of the Baltic Sea coastal waters with the introduction of a complete ban on the use of anti-fouling paints based on organotin complexes [4, 14, 15]. However, the presence of single sediment samples with anomalously high levels of organotin derivatives (MBT, TPhT, TCyT) at the “Northern Sambian” site, as well as a constant level of total OTs content over the two-year observation period for both sampling sites (mean ΣOTs content of 1.82±1.5 for KK, and 4.88±2.5 ng/g d.w. for CC) indicates an additional source of input of these contaminants into the coastal environment. The most likely source may be industrial wastewater enriched with plastic debris or pesticides. At the same time, it is obvious that at the “Northern Sambian” site, which is close to the Svetlogorsk urban runoff, the intake and levels of OTs pollution are higher than at the “Curonian Spit” site, the coast of which is a nature reserve.
Heavy metal contamination, which is often correlated with elevated levels of organotins (such as zinc, lead and copper from antifouling paints), was found to be below international limits [36-38] at the sampling sites and significantly lower than that recorded in bottom sediments of other regions of the Baltic Sea [39, 40]. Moreover, the content of metals and oxides in these sediments turned out to be lower than the average crustal values [41], and the Igeo factor for all studied samples (sampling in 2017) turned out to be negative, indicating the absence of any significant anthropogenic factor in the accumulation of these elements [39].
Differences in the level of content of the studied components for sandy sediments of two sites (relative enrichment of 2-3 times in KK samples compared to СС samples) are due to both the granulometric parameters of the sediments themselves (sandy sediments in the KK site are finer-grained and with a higher share of silt-pelite component) , as well as local features of the eroded rocks and the mineral composition of the sediment (for example, the sediment of the KK site is on average five times enriched in chromium compared to the sediment of the CC site). At the same time, it is necessary to note the relative enrichment in arsenic (As) of coarser-grained sandy sediments of the “Northern Sambian” site, which is associated with increased contents of organotin compounds in a number of samples, which requires increased attention and annual monitoring, since it may be associated with uncontrolled urban runoff in the Svetlogorsk area [37].
Conclusion
Analysis of bottom sediment samples collected in 2017-2018 on the Kaliningrad shelf of the Baltic Sea, showed the presence of detectable amounts of organotin compounds, although at least 10 years have passed since the complete ban on the use of anti-fouling paints for marine vessels based on TBT. However, the low contents of tributyltin in the studied samples (maximum 2.3 ng/g d.w.) and the high level of its degradation (BDI from 1.3-12.4 to 14-38) indicate the high efficiency of tributyltin transformation processes and self-purification of sandy sediments of two tested areas.
Differences in the local conditions of sediment formation in the studied sites of the “Curonian Spit” and “Northern Sambian” are manifested not only in granulometric features, but also in the OTs spectra and the content of heavy metals. Well-sorted sediment with a minor amount of pelite-silty material due to wave impact and the absence of significant anthropogenic impact, including sea traffic, in areas adjacent to the coast, determine the minimum level of contamination of the studied marine sediments. But the presence of single anomalous samples in terms of content and spectrum of OTs in a limited number of samples from the “Northern Sambian” site indicates additional sources of pollution, the control of which must be ensured by annual environmental monitoring of the state of bottom sediments, since the negative impact of such pollutants can lead to an irreversible change in the environmental situation for the Baltic region.
The content of organotin compounds and heavy metals must also be taken into account when assessing the environmental risks of engineering work on the seabed due to the identified correlation of harmful substances and fine-grained fractions.
References
- Duaa G., Zahraa R., Emad Y. A Review of Organotin Compounds: Chemistry and Applications. Archives of Organic and Inorganic Chemical Sciences. 2018. Vol. 3. Iss. 3, p. 344-352. DOI: 10.32474/AOICS.2018.03.000161
- de Oliviera D.D., Rojas E.G., dos Santos Fernandez M.A. Should TBT continue to be considered an issue in dredging port areas? A brief review of the global evidence. Ocean & Coastal Management. 2020. Vol. 197. N 105303. DOI: 10.1016/j.ocecoaman.2020.105303
- Jokšas K., Stakėnienė R., Raudonytė-Svirbutavičienė E. On the effectiveness of tributyltin ban: Distribution and changes in butyltin concentrations over a 9-year period in Klaipėda Port, Lithuania. Ecotoxicology and Environmental Safety. 2019. Vol. 183. N 109515. Doi: 10.1016/j.ecoenv.2019.109515
- Uc-Peraza R.G., Castro Í.B., Fillmann G. An absurd scenario in 2021: Banned TBT-based antifouling products still available on the market. Science of the Total Environment. 2022. Vol. 805. N 150377. DOI: 10.1016/j.scitotenv.2021.150377
- Beyer J., Song Y., Tollefsen K.E. et al. The ecotoxicology of marine tributyltin (TBT) hotspots: A review. Marine Environmental Research. 2022. Vol. 179. N 105689. Doi: 10.1016/j.marenvres.2022.105689
- Lagadic L., Katsiadaki I., Biever R. et al. Tributyltin: Advancing the Science on Assessing Endocrine Disruption with an Unconventional Endocrine-Disrupting Compound. Reviews of Environmental Contamination and Toxicology. Cham: Springer, 2018. Vol. 245, p. 65-127. DOI: 10.1007/398_2017_8
- Khanam M.R.M., Shimasaki Y., Hosain M.Z. et al. Effects of the antifouling agent tributyltin on the sinking behavior, photosynthetic rate and biochemical composition of the marine planktonic diatom Thalassiosira pseudonana. Ecotoxicology. 2022. Vol. 31. Iss. 7, p. 1158-1168. DOI: 10.1007/s10646-022-02577-9
- Sheng-Nan Shu, Rui-Tong Jiang, Jie Yin et al. Characteristics, sources and health risks of organotin compounds in marine organisms from the seas adjacent to the eastern ports of China. Regional Studies in Marine Science. 2023. Vol. 61. N 102929. DOI: 10.1016/j.rsma.2023.102929
- Suzdalev S., Gulbinskas S., Blažauskas N. Distribution of tributyltin in surface sediments from transitional marine-lagoon system of the south-eastern Baltic Sea, Lithuania. Environmental Science and Pollution Research. 2015. Vol. 22. Iss. 4, p. 2634-2642. DOI: 10.1007/s11356-014-3521-4
- Warford L., Mason C., Lonsdale J. et al. A reassessment of TBT action levels for determining the fate of dredged sediments in the United Kingdom. Marine Pollution Bulletin. 2022. Vol. 176. N 113439. DOI: 10.1016/j.marpolbul.2022.113439
- Bandara K.R.V., Chinthaka S.D.M., Yasawardene S.G., Manage P.M. Modified, optimized method of determination of Tributyltin (TBT) contamination in coastal water, sediment and biota in Sri Lanka. Marine Pollution Bulletin. 2021. Vol. 166. N 112202. DOI: 10.1016/j.marpolbul.2021.112202
- Quintas P.Y., Alvarez M.B., Arias A.H. et al. Spatiotemporal distribution of organotin compounds in the coastal water of the Bahía Blanca estuary (Argentina). Environmental Science and Pollution Research. 2019. Vol. 26. Iss. 8, p. 7601-7613. DOI: 10.1007/s11356-019-04181-7
- Kucklick J.R., Ellisor M.D. A review of organotin contamination in arctic and subarctic regions. Emerging Contaminants. 2019. Vol. 5, p. 150-156. DOI: 10.1016/j.emcon.2019.04.003
- Abraham M., Westphal L., Hand I. et al. TBT and its metabolites in sediments: Survey at a German coastal site and the central Baltic Sea. Marine Pollution Bulletin. 2017. Vol. 121. Iss. 1-2, p. 404-410. DOI: 10.1016/j.marpolbul.2017.06.020
- Filipkowska A., Kowalewska G. Butyltins in sediments from the Southern Baltic coastal zone: Is it still a matter of concern, 10 years after implementation of the total ban? Marine Pollution Bulletin. 2019. Vol. 146, p. 343-348. DOI: 10.1016/j.marpolbul.2019.06.050
- Metelkova L.O., Zhakovskaya Z.A., Mamontova V.N., Kukhareva G.I. Organotin compounds (OTS) in water and sediments from the Neva River and the Eastern Gulf of Finland. Water: Chemistry and Ecology. 2017. N 3, p. 70-82 (in Russian).
- Mil-Homens M., Almeida C.M.R., Dias S. et al. Spatial distribution and temporal trends of butyltin compounds (TBT, DBT & MBT) in short sediment cores of the SW Portuguese Shelf (western Iberian Margin, NE Atlantic). Science of the Total Environment. 2023. Vol. 900. N 165872. DOI: 10.1016/j.scitotenv.2023.165872
- Zhakovskaya Z., Metelkova L., Kukhareva G. et al. Mobility of metal-organic pollutants in the emerging coastal-marine sediment of the Baltic Sea: The case-example of organotin compounds in sediments of the Gulf of Finland. Journal of Sea Research. 2022. Vol. 190. N 102307. DOI: 10.1016/j.seares.2022.102307
- Atlas of geological and environmental geological maps of the Russian area of the Baltic sea. St. Petersburg: VSEGEI, 2010, p. 77 (in Russian).
- Metelkova L., Zhakovskaya Z., Kukhareva G. et al. Organotin compounds (OTs) in surface sediments, bivalves and algae from the Russian coast of the Barents Sea (Kola Peninsula) and the Fram Strait (Svalbard Archipelago). Environmental Science and Pollution Research. 2022. Vol. 29. Iss. 23, p. 34659-34669. DOI: 10.1007/s11356-021-18091-0
- Kucharski D., Giebułtowicz J., Drobniewska A. et al. The study on contamination of bottom sediments from the Odra River estuary (SW Baltic Sea) by tributyltin using environmetric methods. Chemosphere. 2022. Vol. 308. Part 1. N 136133. DOI: 10.1016/j.chemosphere.2022.136133
- Raudonytė-Svirbutavičienė E., Jokšas K., Stakėnienė R. On the effectiveness of tributyltin ban part II: Temporal and spatial trends of organotin pollution in intense sediment accumulation areas and dumping sites of the Baltic Sea. Journal of Hazardous Materials Advances. 2023. Vol. 10. N 100294. DOI: 10.1016/j.hazadv.2023.100294
- Wenjun Gui, Chunxia Tian, Qianqian Sun et al. Simultaneous determination of organotin pesticides by HPLC-ICP-MS and their sorption, desorption, and transformation in freshwater sediments. Water Research. 2016. Vol. 95, p. 185-194. DOI: 10.1016/j.watres.2016.02.056
- Liping Fang, Cuihong Xu, Ji Li et al. The importance of environmental factors and matrices in the adsorption, desorption, and toxicity of butyltins: a review. Environmental Science and Pollution Research. 2017. Vol. 24. Iss. 10, p. 9159-9173. DOI: 10.1007/s11356-017-8449-z
- Quintas P.Y., Fernández E.M., Spetter C.V. et al. Preliminary studies about the role of physicochemical parameters on the organotin compound dynamic in a South American estuary (Bahía Blanca, Argentina). Environmental Monitoring and Assessment. 2019. Vol. 191. Iss. 3. N 127. DOI: 10.1007/s10661-019-7260-3
- Cruz A., Anselmo A.M., Suzuki S., Mendo S. Tributyltin (TBT): A Review on Microbial Resistance and Degradation. Critical Reviews in Environmental Science and Technology. 2015. Vol. 45. Iss. 9, p. 970-1006. DOI: 10.1080/10643389.2014.924181
- Shkapenko V.V. Geochemical features of biotransformation of non-polar hydrocarbons and heavy metal compounds in bottom sediments: Avtoref. dis. … kand. geol. nauk. Kyiv: М.P.Sеmenenko IGMO, 2016, p. 20 (in Ukrainian).
- Martina Furdek Turk, Ivanić M., Dautović J. et al. Simultaneous analysis of butyltins and total tin in sediments as a tool for the assessment of tributyltin behaviour, long-term persistence and historical contamination in the coastal environment. Chemosphere. 2020. Vol. 258. N 127307. DOI: 10.1016/j.chemosphere.2020.127307
- Ronia Chung-tin Sham, Lily Shi Ru Tao, Yanny King Yan Mak et al. Occurrence and trophic magnification profile of triphenyltin compounds in marine mammals and their corresponding food webs. Environmental International. 2020. Vol. 137. N 105567. DOI: 10.1016/j.envint.2020.105567
- Rajendran K., Dey R., Ghosh A., Das D. In search of biocatalytic remedy for organotin compounds- the recalcitrant eco-toxicants. Biophysical Chemistry. 2022. Vol. 290. N 106888. DOI: 10.1016/j.bpc.2022.106888
- Kuzikova I., Andronov E., Zaytseva T. et al. A microcosm approach for evaluating the microbial nonylphenol and butyltin biodegradation and bacterial community shifts in co-contaminated bottom sediments from the Gulf of Finland, the Baltic Sea. Environmental Science and Pollution Research. 2022. Vol. 29. Iss. 46, p. 69849-69860. DOI: 10.1007/s11356-022-20751-8
- Soboń A., Szewczyk R., Długoński J. Tributyltin (TBT) biodegradation induces oxidative stress of Cunninghamella echinulate. International Biodeterioration & Biodegradation. 2016. Vol. 107. P. 92-101. DOI: 10.1016/j.ibiod.2015.11.013
- Finnegan C., Ryan D., Enright A.-M., Garcia-Cabellos G. A review of strategies for the detection and remediation of organotin pollution. Critical Reviews in Environmental Science and Technology. 2018. Vol. 48. Iss. 1, p. 77-118. DOI: 10.1080/10643389.2018.1443669
- Borrego B.B., Gracioso L.H., Karolski B. et al. Tributyltin degrading microbial enzymes: A promising remediation approach.Marine Pollution Bulletin. 2023. Vol. 189. N 114725. DOI: 10.1016/j.marpolbul.2023.114725
- Furdek M., Mikac N., Bueno M. et al. Organotin persistence in contaminated marine sediments and porewaters: In situ degradation study using species-specific stable isotopic tracers. Journal of Hazardous Materials. 2016. Vol. 307, p. 263-273. DOI: 10.1016/j.jhazmat.2015.12.037
- Norén A., Fedje K.K., Strömvall A.-M. et al. Low impact leaching agents as remediation media for organotin and metal contaminated sediments. Journal of Environmental Management. 2021. Vol. 282. N 111906. DOI: 10.1016/j.jenvman.2020.111906
- Tarrés M., Cerdà-Domènech M., Pedrosa-Pàmies R. et al. Transport and distributions of naturally and anthropogenically sourced trace metals and arsenic in submarine canyons. Progress in Oceanography. 2023. Vol. 218. N 103122. DOI: 10.1016/j.pocean.2023.103122
- Ugwu I.M., Igbokwe O.A. Sorption of Heavy Metals on Clay Minerals and Oxides: A Review. Advanced Sorption Process Applications. London: IntechOpen, 2019, p. 23. DOI: 10.5772/intechopen.80989
- Remeikaitė-Nikienė N., Garnaga-Budrė G., Lujanienė G. et al. Distribution of metals and extent of contamination in sediments from the south-eastern Baltic Sea (Lithuanian zone). Oceanologia. 2018. Vol. 60. Iss. 2, p. 193-206. DOI: 10.1016/j.oceano.2017.11.001
- Zaborska A., Siedlewicz G., Szymczycha B. et al. Legacy and emerging pollutants in the Gulf of Gdańsk (southern Baltic Sea) – loads and distribution revisited. Marine Pollution Bulletin. 2019. Vol. 139. p. 238-255. DOI: 10.1016/j.marpolbul.2018.11.060
- Rudnick R.L., Gao S. 4.1 – Composition of the Continental Crust // Treatise of Geochemistry. Elsevier, 2014. Vol. 4. p. 1-51. DOI: 10.1016/B978-0-08-095975-7.00301-6
1. International Convention on the Control of Harmful Anti-fouling Systems on Ships. URL (accessed 30.05.2024).
2. Hazardous substances of specific concern to the Baltic Sea. Final report of the HAZARDOUS project. Helsinki: HELCOM, 2009. Baltic Sea Environment Proceedings N 119, p. 96. URL (accessed 30.05.2024).
Baltic Sea Action Plan. 2021 update. Helsinki: HELCOM, 2021, p. 60. URL (accessed 30.05.2024).
3. ISO 23161:2018. Soil Quality – Soil quality – Determination of selected organotin compounds – Gas-chromatographic method. URL (accessed 30.05.2024).
4. HELCOM (2018). Tributyltin (TBT) and imposex. HELCOM core indicator report. URL (accessed 30.05.2024).
Background document on Tributlytin (TBT) in sediment, Swedish Quality Standard.London: OSPAR Commission, 2020, p. 38. URL (accessed 30.05.2024).
Australian and New Zealand Guidelines for Fresh and Marine Water Quality. Australian and New Zealand Environment and Conservation Council, Agriculture and Resource Management Council of Australia and New Zealand, 2000. Vol. 1. The Guidelines, p. 314. URL (accessed 30.05.2024).
Canadian Sediment Quality Guidelines for the Protection of Aquatic Life. Summary Tables. Update. Winnipeg: Canadian Council of Ministers of the Environment, 2002, p. 7. URL (accessed 30.05.2024).