Development and validation of an approach to the environmental and economic assessment of decarbonization projects in the oil and gas sector
- Ph.D. Researcher Luzin Institute for Economic Studies of the Kola Science Centre of the RAS ▪ Orcid
Abstract
This article addresses the problem of selecting a priority decarbonization project for an oil and gas company aiming to reduce greenhouse gas emissions. The wide range of decarbonization options and assessment methods prompted the development of a comprehensive ranking system for project selection. This system incorporates both internal and external factors of project implementation, a two-stage algorithm that filters out unsuitable projects taking into account sustainable development goals, and a quantitative evaluation approach using absolute and relative indicators. The proposed system evaluates decarbonization projects by considering not only the reduction of emissions in both absolute and relative terms, but also the broader environmental, social, and economic aspects relevant to the oil and gas company and the national economy. It includes a ranking mechanism for identifying priority projects and integrates carbon regulation incentives and green taxonomy tools into the economic assessment for more precise comparative analysis. The quantitative assessment in absolute terms involves a specialized net present value calculation, which accounts for revenue from both carbon credit sales and the potential sale of new low-carbon products, if applicable. The proposed assessment provides for targeted analysis of specific performance indicators, such as the cost per unit of emissions reduced, tax and social security contributions per unit of emissions reduced, energy efficiency improvements, and other indicators used for additional assessments of projects under otherwise equal conditions.
Funding This study was supported by a grant from the Russian Science Foundation (Project N 22-78-10181 “Decarbonization of the Russian Oil and Gas Sector: Concept, New Interfaces, Challenges, Technological, Organizational, and Managerial Transformations”).
Introduction
The sustainable development of oil and gas companies can be achieved through the adoption of low-carbon strategies that aim to minimize their impact on the climate and environment while maintaining economic and business operations. Many companies have set ambitious long-term goals, targeting reductions in specific greenhouse gas (GHG) emissions and product carbon footprints by 2050 [1], alongside intermediate goals for 2030 [2]. These carbon neutrality goals involve implementing measures to reduce both absolute and specific GHG emissions within a defined time frame, spanning from a baseline year to a target year. The most common approaches to achieving both long-term and intermediate carbon neutrality targets include pursuing various decarbonization strategies, such as climate action projects.
Since the term “climate action project” can have multiple interpretations and is associated with different criteria, this study adopts a broader definition, namely that of a decarbonization project. Such a project is defined as one or more activities designed to reduce or prevent GHG emissions and/or increase carbon capture within the operational boundaries of an oil and gas company. Given the diversity of decarbonization pathways [3-5], a key challenge for oil and gas companies committed to low-carbon development is determining the most optimal decarbonization projects. Companies often set additional global goals and face constraints when striving for carbon neutrality, such as ensuring positive net present value for decarbonization projects, maximizing carbon credits upon project completion, and leveraging green financing to support sustainable development initiatives. This means that they need to assess not only the emissions reduction potential of decarbonization projects but also their broader environmental, economic, and social effects.
Previous studies addressing project assessments in the oil and gas sector incorporate elements of multi-factor assessment models considering environmental, social, and economic aspects in the implementation of investment projects [6]. Some studies have also proposed synchronizing these models with the project life cycle in the course of their practical application [7, 8]. Additionally, environmental and economic assessments of corporate operations and projects have been proposed, using indicator groups related to production, environmental protection, and environmental economic activities [9]. In other studies, terms such as “strategic environmental assessment” are used to refer to evaluating the potential environmental and socio-environmental impacts of strategic decisions, such as regional or sectoral development plans [10]. Similarly, “environmental and economic assessment” is intended to support technical and economic decision-making by incorporating environmental considerations alongside technological and economic factors [11, 12]. However, comprehensive environmental and economic assessments specific to decarbonization projects have received limited attention in the academic literature.
The purpose of this study is to develop a methodology for the environmental and economic assessment of decarbonization projects within oil and gas companies. The main objectives are to address the following research questions: What indicators should shape the foundation of the assessment system for decarbonization projects in the oil and gas sector? How should these projects be ranked to identify those with the highest priority?
An algorithm has been developed for assessing decarbonization projects in the oil and gas sector, accounting for both internal and external factors. The algorithm follows a deductive approach, where the conditions for implementing the decarbonization project are progressively clarified, and additional parameters with indirect impacts are evaluated as the process unfolds. The comparative assessment of these projects, used for ranking, takes into consideration their environmental, social, and economic benefits not only for the company itself but also for the region and national economy.
The concept of climate efficiency is introduced to measure the effectiveness of actions aimed at reducing and/or preventing greenhouse gas emissions or increasing their capture, expressed in specific quantitative terms. This concept is enhanced by incorporating sustainable development factors, including economic efficiency and the minimization of negative environmental impacts.
The research acknowledges the role of feasibility studies as key tools for investment decision-making. Since their results have a major influence on the ranking of decarbonization projects, they are considered along with the project’s contribution to achieving carbon neutrality targets.
The approach to the quantitative assessment of the economic efficiency of decarbonization projects is based on net present value and its components. However, it is refined by accounting for the specific aspects of decarbonization project implementation. These include the use of green taxonomy incentives and carbon trading mechanisms [13-16], as well as penalties for exceeding GHG emissions quotas [17]. Additional environmental impacts and other factors affecting the project’s cash flow, both positive and negative, are also considered.
The development of the algorithm and indicators is based on the following assumptions:
- The decision-making process for selecting a decarbonization project follows the principle of maximizing emission reduction while ensuring that negative social, environmental, and economic impacts remain within acceptable limits. A decarbonization project could potentially lead to environmental degradation and/or an increase in negative effects, which should be considered during project selection.
- To prevent the deterioration of environmental indicators beyond the scope of the project, additional sustainable development criteria are introduced to safeguard against the decline in indirect parameters.
- Since the social impact is challenging to quantify, this study uses financial indicators such as contributions to insurance and pension funds, healthcare costs, and employee wages associated with project implementation. The analysis of the social significance of a project focuses solely on parameters that can be measured through financial data. Consequently, indicators such as the Human Development Index, changes in quality of life, and health-related impacts (e.g., morbidity), which can also be affected by decarbonization projects, are excluded due to the difficulty in applying a universal and objective comparative assessment. However, these factors are expressed in monetary terms to reflect the project’s contribution to social development.
- The quantitative absolute indicators used for assessing decarbonization projects are based on traditional approaches to conducting feasibility studies aimed at analyzing investment projects, with the added consideration of environmental and economic aspects.
- The methodology does not impose restrictions on investment volumes or project timelines.
This study proposes a five-stage process for the selection, assessment, and ranking of potential decarbonization projects:
Stage 1 involves the preliminary selection of decarbonization projects. This includes eliminating those that cannot be implemented due to non-compliance with strategic planning documents or conflicts with stated objectives. At this stage, the feasibility of the decarbonization project is assessed.
Stage 2 assesses the remaining projects for economic, environmental, and climate efficiency. In this study, environmental efficiency encompasses aspects of the natural environment, such as air, water, and soil pollution, while climate-related issues are treated as a separate category. At this stage, the project’s contribution to GHG emissions reduction is evaluated, ensuring alignment with sustainable development criteria.
Stage 3 deals with a qualitative assessment of the project’s contribution to decarbonization and sustainable development goals. The project is evaluated based on its adherence to priority criteria, with the accumulated total used for comparison.
Stage 4 involves a quantitative assessment of the project’s environmental, climate, and investment efficiency for the company implementing the project. Additionally, the socio-economic impact of the project on national and regional economies is considered.
Stage 5 is dedicated to the comparative analysis of the priority project’s specific indicators against those of other decarbonization projects, including projects undertaken by other companies.
The primary research methods employed in this study are decomposition, classification, and strategic analysis, drawing on current practical materials related to the topic.
Discussion
Decarbonization efforts encompass a broad array of methods and tools [3-5, 15]. The climate efficiency of each decarbonization strategy can be evaluated within the context of a specific industrial process, company, or segment of the oil and gas industry. For instance, targeted measures such as reducing methane leaks or curbing the flaring of associated petroleum gas can be implemented, alongside broader strategies that include improving energy efficiency, increasing the share of renewable energy in energy consumption, or carbon sequestration [18, 19].
However, the outcomes of a decarbonization project can vary significantly depending on factors such as production scale, regional, geographical, and climatic conditions, and the characteristics of the oil and gas segment in which it is applied. The same project may yield different results depending on the internal and external environments of the company. The external environment includes factors beyond the company’s control, while internal factors consist of those within the company’s direct management and control. Identifying and qualitatively assessing these factors helps categorize decarbonization strategies suitable for a company (Fig.1).
External factors can set the direction for decarbonization, while internal factors serve as the mechanisms through which it is implemented. For example, in response to rising demand for low-carbon raw materials, a company might increase the share of low-carbon components in its products to meet consumer needs and expectations. By managing internal factors, a company can reduce emissions across different scopes. To lower the carbon footprint of products, a company might select materials, services, equipment, and suppliers that produce fewer GHG emissions, thereby reducing scope 3 emissions [20]. To cut indirect energy emissions in scope 2, the company might explore local climatic advantages for using low-carbon, renewable energy sources. Reducing other indirect emissions in Scope 3 could involve optimizing supply chain logistics to favor local consumers and minimize transportation emissions. By combining various internal and external factors, a company can develop a tailored decarbonization strategy and prioritize its projects accordingly.
Ranking decarbonization projects and determining their priority in the oil and gas industry is a complex problem, which is solved based on economic efficiency assessments [21]. Decarbonization projects, which focus on reducing, preventing, or capturing GHG emissions, can have varying degrees of impact on the natural environment, social well-being, and the economy [22]. Thus, a comprehensive assessment of these projects is essential [23, 24]. The ranking process is further complicated by the fact that it extends beyond mere compliance with legal regulations and does not solely focus on technical and economic indicators such as projected carbon pricing. Strategic decision-making must consider a broad range of factors, including principles of sustainable development that integrate environmental, financial, climatic, and social aspects [25-28].
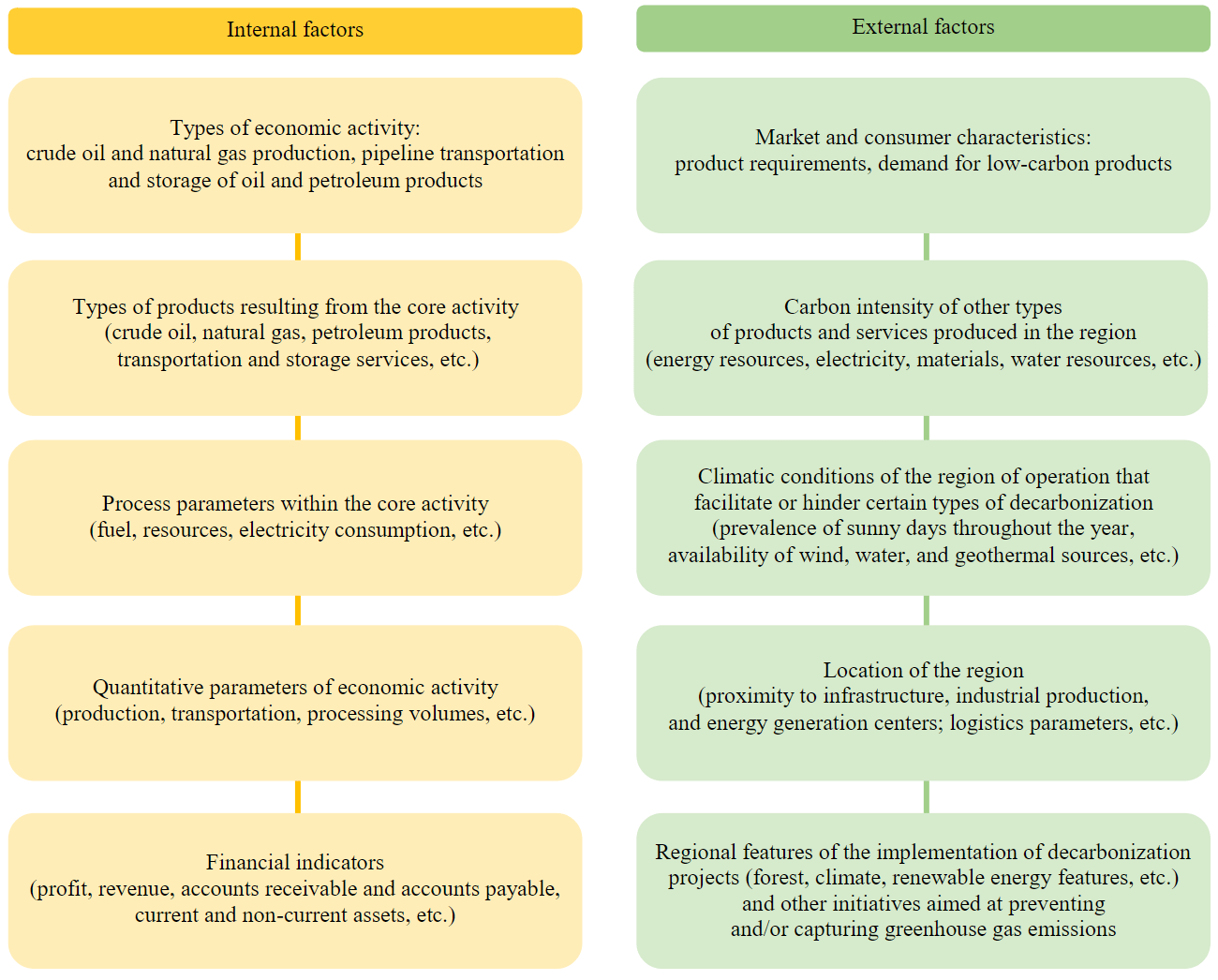
Fig.1. Factors influencing the choice of a decarbonization path RES – renewable energy sources
In line with the concept of sustainable development, which evaluates projects not only in terms of achieving the UN’s Sustainable Development Goal N 13 (Climate Action) but also with respect to other goals such as resource efficiency, air and water quality preservation, employment, and innovation, it is essential to conduct a step-by-step, multi-criteria assessment of decarbonization projects. This approach helps identify projects that meet the maximum number of sustainable development goals while remaining economically viable for the oil and gas company [29].
The key indicator for determining high-priority projects is the simultaneous fulfillment of the conditions outlined in Fig.2. Projects that meet only one or two conditions are considered lower priority.
From a sustainable development perspective, the fundamental criteria for ranking decarbonization projects include the reduction and/or prevention of greenhouse gas emissions and/or an increase in carbon capture, the minimization of negative environmental impacts [30], and the maximization of resource, heat, material, and energy savings. Additionally, projects that reduce operational costs and maximize positive net present value hold higher priority [31].
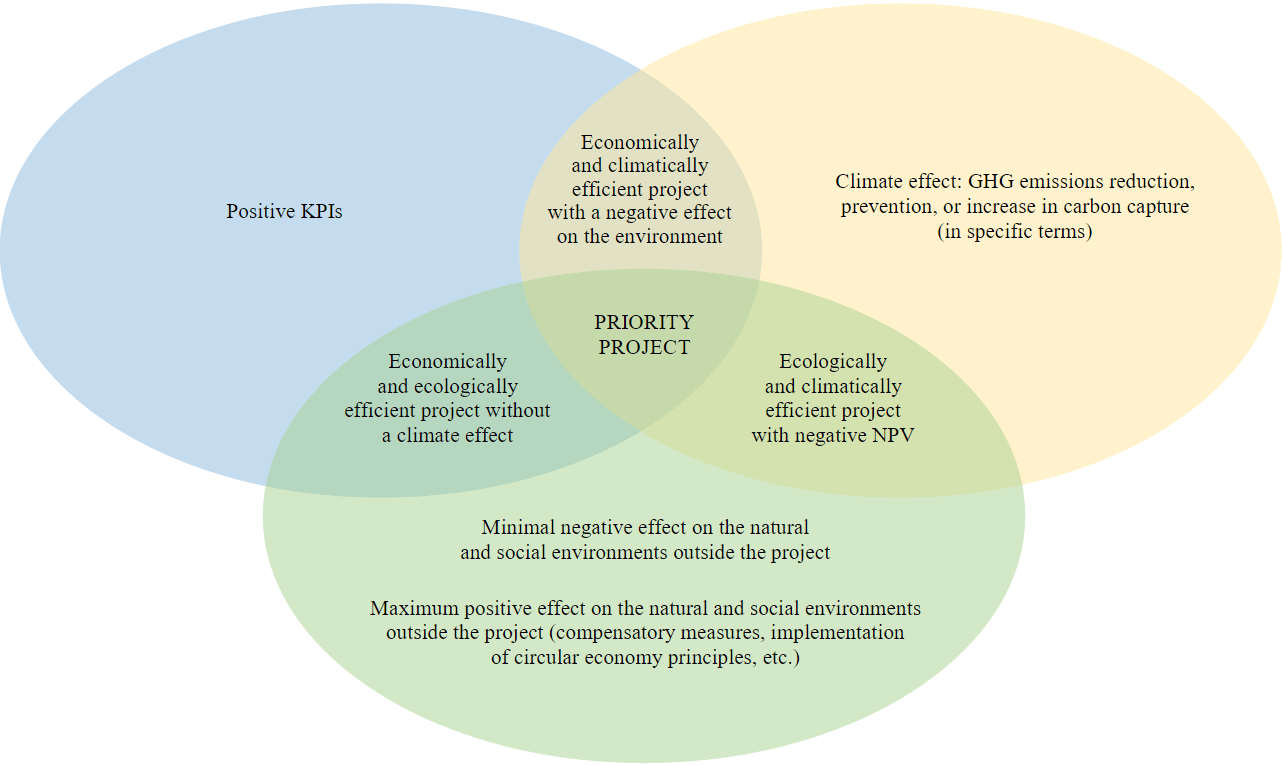
Fig.2. Conditions for prioritizing a decarbonization project NPV – net present value
Oil and gas companies’ strategic planning documents may include various targets that limit the feasibility of certain decarbonization methods:
- increasing hydrocarbon production or introducing high-carbon products (e.g., bitumen);
- expanding operations in regions or countries where low-carbon development principles have not been integrated into local production activities.
Due to this, stage 1 of the decarbonization project assessment process involves comparing the project’s decarbonization approach with the company’s general policy, goals, and strategy. This step also checks the project’s potential effectiveness (Fig.3).
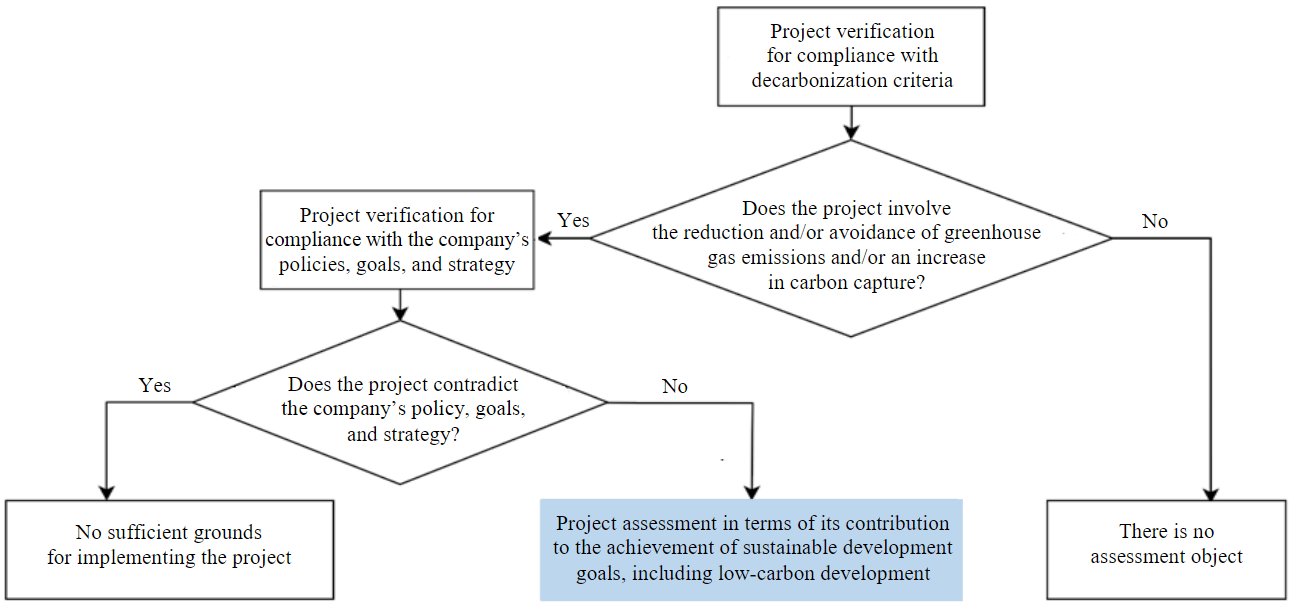
Fig.3. Stage 1 – Preliminary selection of decarbonization projects, feasibility assessment
Guided by stage 1 of the process of selecting, assessing, and ranking decarbonization projects (Fig.3), after excluding projects that fail to reduce greenhouse gas emissions, either in absolute or specific terms, and that conflict with the company’s strategic planning documents, the process moves to stage 2, which includes a qualitative assessment of the project’s alignment with environmental, social, climate, and economic efficiency criteria [32, 33], as well as its adherence to the principles of sustainable development (Fig.4).
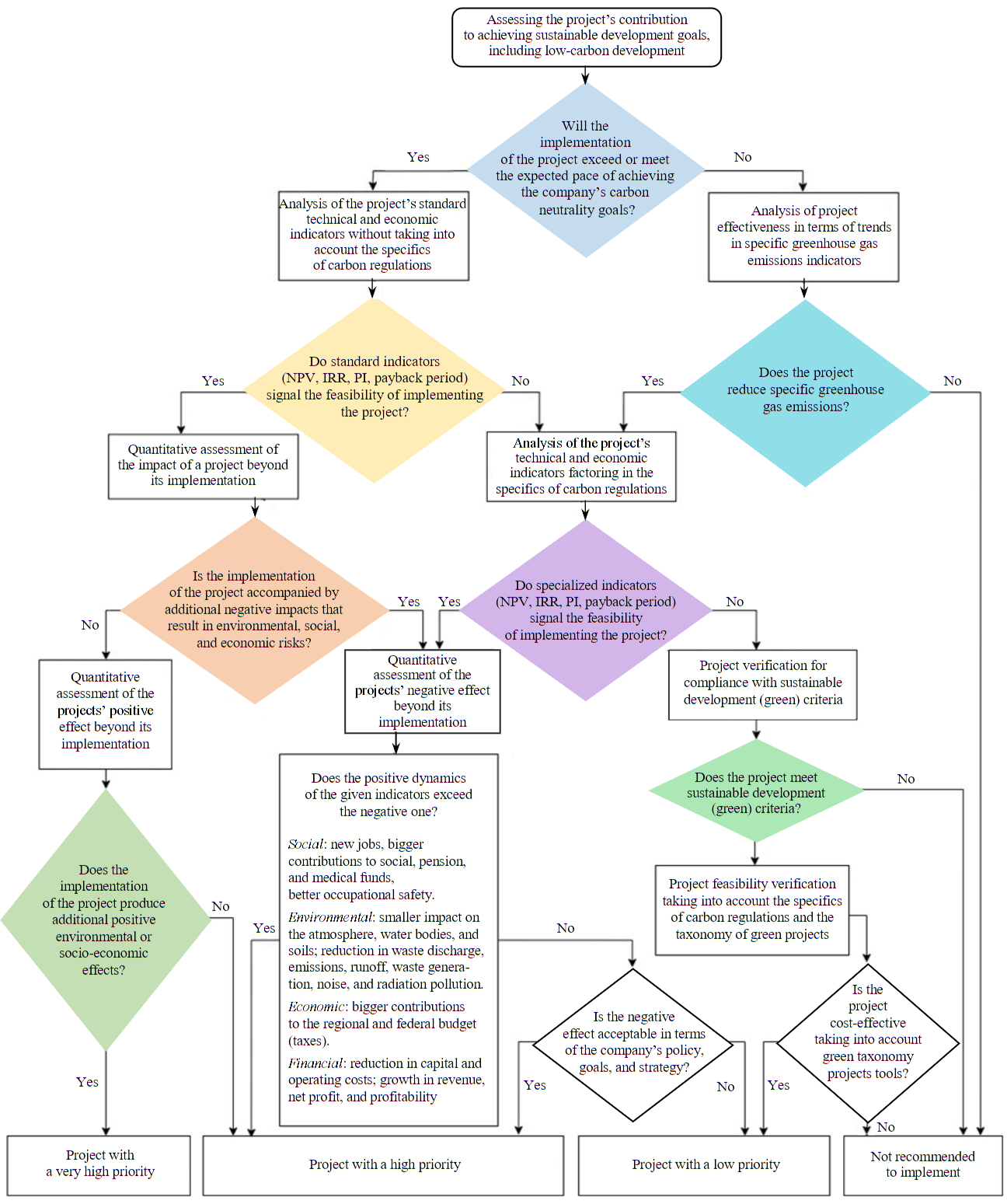
Fig. 4. Stage 2 – Project assessment based on the criteria of economic, environmental, and social efficiency, and sustainable development principles IRR – internal rate of return; PI – profitability index
At stage 2, several projects may pass the criteria, making it necessary to rank and compare them. Stage 3 involves a quantitative assessment of how well each project meets these criteria. Figure 5 illustrates the principle of incrementally adding value to projects based on how well they align with the priority criteria. Each subsequent criterion, ranked higher than the previous one, is evaluated on the condition that the lower criterion has been met. The priority of a project increases with the difficulty of meeting these criteria: point 1 represents the most challenging but highest-priority condition, while point 8 is the easiest to fulfill, though it does not guarantee high priority.
It is important to distinguish between points 2 and 6 of the quantitative assessment (Fig.5). This study proposes two approaches to conducting a feasibility study: the standard approach (point 2) and a specialized approach (point 6). The standard approach follows traditional methods of financial analysis, using tools such as NPV, PI, IRR, and payback period. In this case, the project’s cash flow is calculated without factoring in low-carbon regulation mechanisms, answering the question of whether the decarbonization project is economically viable on its own.
Projects with positive results from the standard assessment – without considering carbon regulation tools—are generally more attractive (point 2) than those relying on specialized tools (point 6). This is because these projects reduce operational costs, increase production efficiency with the same or lower GHG emissions, and thus show a lower carbon footprint. Examples include energy efficiency improvements [34], higher durability of equipment, better utilization of raw materials, reduction in hydrocarbon leaks, and prevention of emergencies resulting in major damage and hydrocarbon losses. However, decarbonization projects often do not exhibit high economic efficiency by traditional measures, which is why carbon management tools (e.g., carbon credits or penalties for exceeding GHG quotas) were introduced to boost their attractiveness.
The order of criteria for assessing decarbonization projects is as follows. Since reducing production volumes does not qualify as either a climate project or a decarbonization project, the first criterion focuses on reducing specific rather than absolute emissions [35]. The second criterion assesses absolute emissions, but instead of comparing emissions before and after project implementation, which would limit the increase in the scale of production activities, the comparison is based on “without the project” versus “with the project” scenarios [36], aligning with climate standards such as ISO 14064-2, Gold Standard, Global Carbon Council, and Verra, etc.
Next, a specialized economic assessment is conducted to encourage companies to undertake decarbonization projects. If the project shows positive economic indicators, compliance with national sustainable development regulations is added as a broader criterion. At this stage, the project is evaluated against the best available technologies (BAT) to ensure that it does not exceed legal limits, though these are less strict than the subsequent criterion, namely minimal negative impact on other social and environmental spheres (water bodies, soil, air, biodiversity) or the absence of such impact.
A more complex condition follows: the project must positively impact operational indicators, such as reducing operating costs. Finally, technical and economic indicators are considered without the use of support measures for GHG reduction. If the project is economically viable without such incentives, it is highly prioritized (point 2). If a project fulfills all of these criteria and significantly reduces emissions, it achieves the highest priority (point 1). The threshold for significance is determined by the company and may be based on meeting or exceeding average annual GHG reduction targets, or achieving/exceeding the expected target by a specific year.
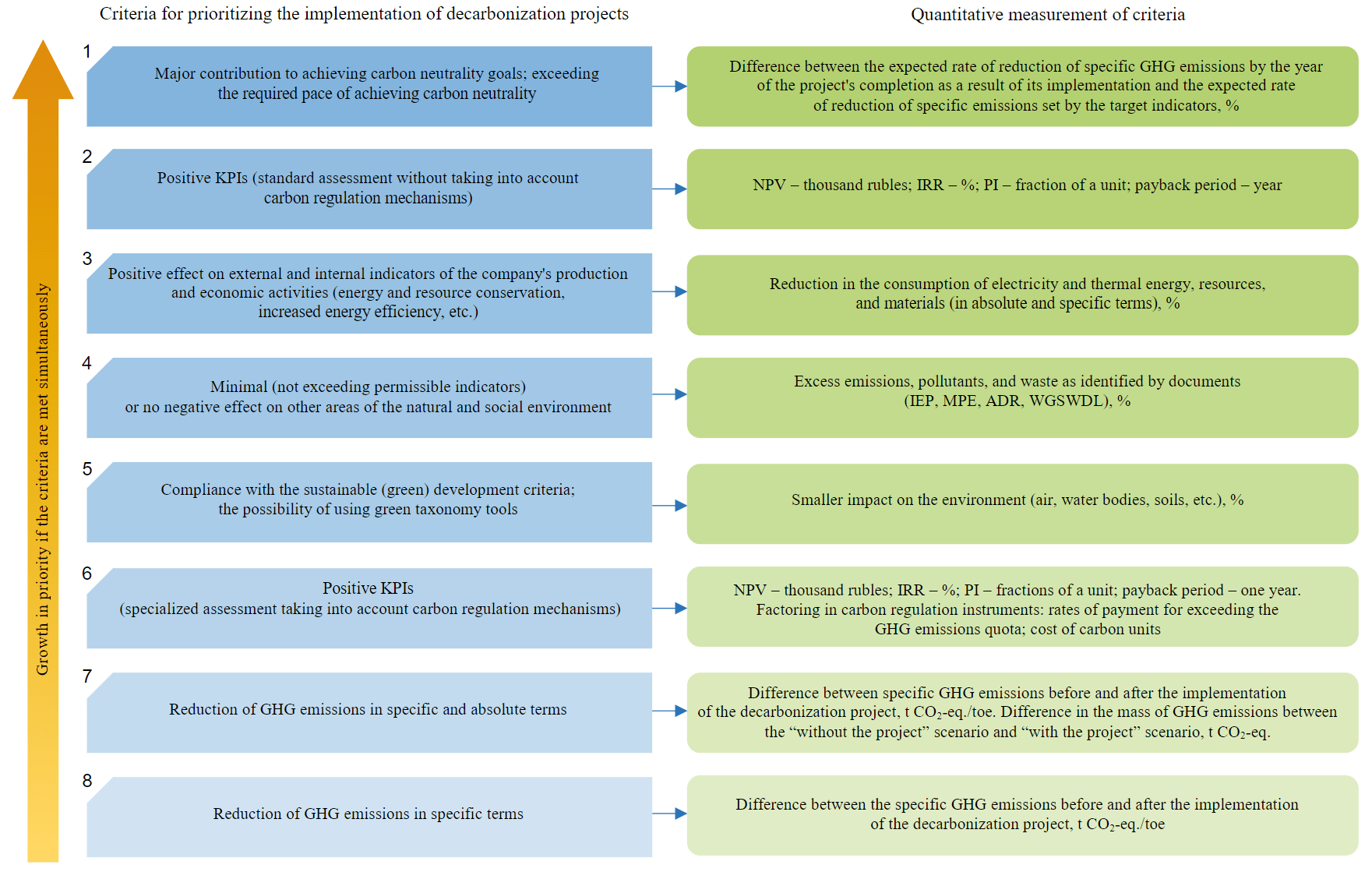
Fig.5. Stage 3 – Quantitative assessment of the project's compliance with the criteria using the principle of incremental priority IEP – integrated environmental permit; MPE – maximum permissible emissions; ADR – allowable discharge rates; WGSWDL – waste generation standards and waste disposal limits
For instance, if a project reduces both absolute and specific emissions but fails to show positive results in the economic assessment, even with carbon regulation mechanisms, it would be assigned priority level 7. Alternatively, if the project aligns with sustainable development criteria (point 5) but fails to minimize negative environmental impacts (e.g., due to generating a large volume of new waste during equipment maintenance), it would not qualify for priority level 4.
The possibility of advancing through priority levels is determined by the measurability of assessment results based on the indicators outlined in the diagram section covering the quantitative measurement of criteria. At this stage, it becomes clear to what extent the decarbonization project will help the company meet or exceed its carbon neutrality targets, or whether only a marginal reduction in specific GHG emissions can be expected. If a project focuses solely on reducing absolute GHG emissions, it is assigned a lower priority because its effectiveness is dependent on production volumes and asset sales. This approach, according to national legislation, does not qualify as a climate project or a valid emissions reduction method.
In conducting the environmental, economic, and climate assessments, a comprehensive system of indicators is proposed. These indicators reflect the project’s impact on various environmental elements (such as pollutant emissions, wastewater discharge, and water resource usage), the social environment (including changes in staff numbers, pension contributions, medical and insurance payments), and contributions to regional and federal tax revenues.
There are numerous approaches to defining the stages of investment projects. Depending on the research objectives, stages can be categorized as pre-investment, investment, operational, or decommissioning. For the purposes of this study, the first two stages are combined due to the absence of a detailed investment analysis. The decarbonization projects are assessed through three stages:
- pre-operational (design, equipment procurement and installation, construction, dismantling, reconstruction, and upgrading);
- operational (the use of equipment and technologies aimed at reducing GHG emissions);
- post-operational (equipment dismantling and land reclamation if necessary due to the end of the lifecycle).
This study focuses exclusively on the operational stage, as it directly relates to the project’s impact on scope 1 and scope 2 emissions. The pre-operational and post-operational stages, which are associated with scope 3 emissions, are not included in the analysis because no national-level metho-dological guidelines are available for these stages. However, for net present value calculations, the capital expenditures of the pre-operational stage are included, as they are necessary for such assessments. The absolute indicators related to the operational stage of decarbonization projects are presented in Table 1.
Decision-making regarding the implementation of investment projects primarily relies on their technical and economic indicators [37, 38]. Consequently, this assessment is conducted at the next stage.
At stage 4 of the selection, assessment, and ranking of decarbonization projects, it is proposed to identify absolute indicators that reflect the impact of the decarbonization project both within the company and at the national level. These indicators are expressed in physical and monetary terms (see Table 1). Lines 1-7 present indicators related to the impact of the decarbonization project on the company, with Lines 1-5 focusing on the project’s cost aspects and Lines 6-7 addressing revenue aspects. Lines 8-9 reflect the project’s influence on the national economy and the social sphere.
Table 1
Stage 4 – A system for quantitative climate, environmental, and economic assessment of decarbonization projects using absolute indicators
N |
Decarbonization project parameter |
Physical aspect |
Value, thousand rubles |
1 |
Energy consumption, MWh, Gcal |
Volume of electricity and thermal energy consumption |
Electricity and thermal energy costs |
2 |
Resource consumption, m3, t |
Volume of resource consumption within the main activity (water, sand, steam, proppant, cement, foam insulation, chemical reagents, additives, metal structures, fuel, other materials) |
Resource costs |
3 |
Impact on other components of the environment, t |
Volume of waste generation, incineration, and disposal*; volume/mass of pollutant discharges into water bodies; volume/mass of pollutant emissions into the atmosphere |
Payment for negative environmental impact |
4 |
Climate damage caused by failure to comply with legal requirements, thousand tons of CO2-eq. |
Amount of greenhouse gas emissions exceeding the established quota (if applicable) |
Payment for exceeding the established GHG emissions quotas |
5 |
Capital expenditures of the decarbonization project, including those aimed at the production of new products (if applicable), units |
The number of units of purchased equipment and materials categorized as capital expenditures; services rendered by third-party organizations (design, research and development, etc.); work performed by third-party organizations (construction, installation, etc.) |
Equipment, work, and services costs |
6 |
Climate efficiency, thousand tons of CO2-eq. |
Change scope 1, 2, and 3 emissions; change in capturing GHG emissions; change in the prevention of GHG emissions |
Value of carbon units (emission reduction units) |
7 |
Additional financial effect, toe; TJ, MW |
Production of standard products; production of new products |
Revenue from sales of additional volumes of standard products and/or new products produced as a result of the decarbonization project (if applicable) |
8 |
State of the economy (budget) of the region or country, thousand rubles |
Taxes (value-added tax (VAT), income tax, property tax) |
Tax contributions to regional and federal budgets as a result of the implementation of the decarbonization project and related projects, including taxes covering social, insurance, pension, and medical needs |
9 |
Social efficiency, number of people |
The difference between the number of new jobs created by the project and the number of previously existing jobs (taking into account the suspension of other projects or works, if applicable) |
* Condition: If the waste was previously buried but is subjected to incineration within the framework of the decarbonization project, the positive effect is not taken into account.
Given the potential for divergent trends among these parameters, it is recommended to identify quantitative indicators of potential changes. This requires determining absolute indicators that can estimate the project’s revenue, operating costs, and the economic effects beyond the project itself. Utilizing absolute indicators allows for a comparative assessment of decarbonization projects, making it possible to analyze one project in isolation or to compare multiple projects against each other. This approach helps identify the feasibility of implementing a specific project in relation to others executed by the same company.
Based on the information in Table 1, a method can be developed for quantitatively assessing the decarbonization project in absolute monetary terms (over the entire implementation period) by comparing cash inflows and outflows:
- for the company, the internal impact of a decarbonization project can be expressed as
where ERi is the total amount of emission reduction resulting from the implementation of decarbonization project i (thousand t CO2-eq); PCUis the cost of one unit of GHG emission reduction (carbon unit) (thousand rub./thousand t CO2-eq); CAPEXis capital expenditures for the decarbonization project (thousand rub.); rgreenium is the correction coefficient accounting for preferential financing terms (if applicable), assuming the decarbonization project qualifies as a sustainable project, including green projects; ProdNEW is the number of new products resulting from the decarbonization project (units); PProdNEW is costs per unit of new products (thousand rub./unit); tis the year; r is the discount rate, %; DOPEXis the change in operating costs due to the implementation of the decarbonization project, assuming there are GHG emission quotas which may be exceeded,
Cenergyi is energy consumption costs to implement the decarbonization project (thousand rub.); CRenergyD is reduction in energy consumption costs from the decarbonization project (thousand rub.); Cmaterialsi is resource and material consumption costs for the decarbonization project (thousand rub.); CRmaterialsD is reduction in resource and material consumption costs due to the project (thousand rub.); Cimpacti is payment for the negative environmental impact of the decarbonization project (thousand rub.); CRimpactD is reduction in the payment for negative environmental impact as a result of the project (thousand rub.); E is baseline corporate GHG emissions without the decarbonization project (thousand tons of CO2-eq); Eq isthe quota for GHG emissions (if any) (thousand t of CO2-eq).; Te ispayment for exceeding the established emission quota (thousand rub.).
If no quotas are set or there is no excess, the formula simplifies accordingly:
- for the national economy, the external economic efficiency of the decarbonization project can be represented as:
where ΔTax ischange in tax payments, factoring in possible reductions from core activities and increases resulting from project implementation (thousand rub.); ΔPayments is change in social security contributions, accounting for potential reductions from core activities and increases resulting from the decarbonization project (e.g., the net increase in the number of employees) (thousand rub.).
By employing these absolute indicators, we can derive specific indicators that enable further comparison of decarbonization projects based on a unified parameter, both in physical and monetary terms (see Table 2).
Table 2
Stage 5 – A system for quantitative climate, environmental, and economic assessment of decarbonization projects using specific indicators
Indicator |
Description |
Units of measurement |
Climate impact |
Change in the carbon footprint of products sold throughout the supply chain |
t CO2-eq./toe; thousand t CO2-eq./TJ |
Energy consumption |
Change in electricity consumption per product unit produced or sold |
MWh/toe; MWh/boe; MWh/thousand rub. |
Resource consumption |
Consumption of individual types of resources and materials used within the framework of core activities (water, sand, proppant, cement, foam insulation, chemical reagents, additives, fuel, other materials) per unit of manufactured output |
t/toe; m3/toe; t/TJ; m3/TJ |
Environmental impact |
Mass of waste produced, incinerated, or disposed of; mass of pollutant discharges into water bodies; mass of pollutant emissions into the atmosphere per unit of output |
t of waste by hazard class/toe, t of pollutants by type/toe, t of waste by hazard class/TJ, t of pollutants by type/TJ |
Costs |
Capital and operating costs per mass of GHG emissions reduced |
thousand rub./t CO2-eq. |
Economy/climate ratio |
Capital and operating costs per revenue from GHG emission reduction units, carbon unit price |
thousand rub./thousand rubles |
Tax/climate ratio |
Taxes resulting from project implementation (VAT, pro-perty tax, income tax) per mass of GHG emissions reduction |
thousand rub./t CO2-eq. |
Society/climate ratio |
Insurance, pension, and medical taxes paid for the staff involved in project implementation for the mass of GHG emissions reduction; salaries |
thousand rub./t CO2-eq. |
In assessing specific indicators, some analyses use the volume of manufactured products, while others rely on the volume of sold products. This distinction arises because sold products account for the processes of transportation and delivery to the end consumer, aligning more closely with comprehensive approaches that evaluate all stages along the product’s value chain. In this context, the volume of products is adjusted to account for technological losses and inventory that remains unsold due to economic or logistical reasons. Considering the volume of manufactured products is useful for analyzing and optimizing production processes and operational activities.
Specific indicators are employed to gauge the effectiveness of project implementation by comparing the specific indicators from the base year with those from the reporting year if the project is ongoing, or with the completion year if the project has been completed. These specific indicators for the reporting year can also help identify the highest priority project among several possibilities, focusing on the most significant key specific indicator for the company.
An example of a project that demonstrates the results of Stage 5 in the proposed algorithm –utilizing a quantitative climate, environmental, and economic assessment system for a decarbonization project – is the initiative to increase the utilization of associated petroleum gas (APG) at the A field in the Khanty-Mansi Autonomous Okrug – Yugra, which has been underway since 2018. Over the 15-year period under consideration, the volume of APG production has reached 1,424.85 million m3. The decarbonization project aims to reduce greenhouse gas emissions from APG flaring by generating electricity with the help of a natural gas-fired reciprocating engine (RE). This initiative increases the level of APG utilization from 95 to 99 % while ensuring compliance with existing legislative requirements[6]. In this case, the reduction in emissions is not a result of external factors, nor does it stem from a decrease in economic activity or cause an increase in emissions outside the project. Thus, it can be concluded that the project meets the established criteria[7]. The initial data for the project, detailed in Table 3, include parameters that are considered in the context of either the decarbonization project (items 4, 6, 9, 10, and 11) or the entire company (items 5, 7, 8, 12, and 13). This approach assesses the impact of the decarbonization project on the main production activities of the company. The revenue from the sale of carbon units (item 11) is calculated by multiplying the number of verified emission reduction units by the selling price of the carbon units.
Table 3
Case study: Initial data without and with the decarbonization project
N |
Parameter |
No project |
With project |
1 |
APG production, million m3 |
1424.85 |
1424.85 |
2 |
APG utilization, % of production |
95 |
99 |
3 |
APG utilization, million m3 |
1353.61 |
1410.60 |
4 |
APG flaring, million m3 |
71.24 |
14.25 |
5 |
Capital investments (project), thousand rub. |
0.00 |
102900.00 |
6 |
Operating expenses, thousand rub. |
561000.00 |
400943.60 |
7 |
Waste generated, t |
582.00 |
593.64 |
8 |
Pollutant emissions generated, t |
1781.06 |
413.21 |
9 |
Greenhouse gas emissions generated, including |
249448.49 |
1172.93 |
|
flaring, t CO2-eq. |
249448.49 |
1164.15 |
|
natural gas-fired reciprocating engine, t CO2-eq. |
0.00 |
8.79 |
10 |
Electricity generated, MWh |
0.00 |
30.90 |
11 |
Revenue from carbon units, thousand rub. |
0.00 |
496568.68 |
12 |
Payment for waste generation, thousand rub. |
552.90 |
563.96 |
13 |
Payment for pollutant emissions, thousand rub. |
534.32 |
123.96 |
Note. The table is based on decarbonization project data.
Additional positive outcomes of the project include a major reduction in pollutant emissions released into the atmosphere, as well as a decrease in operating costs for the energy supply of the field infrastructure. This reduction is primarily due to the generated electricity being utilized on-site rather than being sold as a new product. However, there are also some negative consequences associated with the project. These include a 2 % increase in waste generated as a result of RE servicing, as well as a 54 % rise in the payments for pollutant emissions (nitrogen dioxide, nitrogen oxide, etc.) associated with RE operation. Despite these challenges, revenue generated from the sale of carbon units offsets the costs associated with waste generation and pollutant emissions, as well as the capital costs of the project. The net discounted cash flow for the decarbonization project is estimated at 53,624.00 thousand rub., calculated using a discount rate of 15 %. The resulting specific indicators are presented in Table 4.
Table 4
Quantitative assessment of the decarbonization project: Results
The system of quantitative assessment indicators for decarbonization projects |
No project |
With project |
Climate impact, t СО2-eq./toe |
16.41 |
0.08 |
Environmental impact (t/toe), including |
0.79 |
0.59 |
waste |
0.05 |
0.05 |
pollutant emissions |
1.52 |
1.13 |
Costs, thousand rub./t CO2-eq. |
n/a |
0.46 |
Economy/climate ratio, thousand rub./thousand rub. |
n/a |
0.23 |
Tax/climate ratio, thousand rub./t CO2-eq. |
n/a |
0.42 |
Society/climate ratio, thousand rub./t CO2-eq. |
n/a |
0.05 |
Note. Oil production for the period under review amounted to 10,626.90 thousand t. To convert it to toe, a coefficient of 1.43 was used.
The project’s impact on the climate is assessed within the defined boundaries of variable industrial processes, specifically comparing the handling of associated petroleum gas (APG) within the “without the project” and “with the project” scenarios. This approach excludes other greenhouse gas emissions to eliminate the influence of external variable factors. As a result of the project implementation, the economic and climatic coefficient (Table 4), which is evaluated within the context of the APG utilization decarbonization project, has decreased. To achieve a reduction of one ton of CO2-equivalent emissions, the project incurred total costs of 460 rub. per ton of CO2-equivalent, as indicated by the project cost metrics. The economic and climatic coefficient, which is less than 1, stands at 0.23, indicating that revenue from the sale of carbon units exceeds the capital and operating costs of the project. Furthermore, for each ton of GHG emissions reduced, there are additional fiscal implications: 420 rub. in taxes and 50 rub. in social security contributions, which are reflected in the fiscal and socio-climatic coefficients.
Discussion
Oil and gas companies that have established targets for reducing GHG emissions face the challenge of selecting priority decarbonization projects or groups of projects [2, 31]. These initiatives are not only aimed at achieving the specified goals but also at acquiring additional benefits, such as maximizing NPV and minimizing negative impacts on the surrounding social and natural environments, as well as the climate, both within and outside the projects [11]. Furthermore, these projects must comply with the criteria set by climate project standards and regulatory requirements. The wide array of decarbonization opportunities [3, 5] allows companies to identify several viable projects, considering their geographical, infrastructural, and technological parameters [7]. Typically, companies evaluate the potential economic efficiency of design solutions.
However, each project will exhibit different emission reduction metrics, capital and operating costs, environmental impact indicators, and associated payments for negative impacts. Consequently, this variability necessitates the involvement of different managers and workers for each project. All differences can be assessed in monetary terms using formula (1), which provides a universal financial representation of each project. Standard feasibility study tools often lack a detailed evaluation of environmental impact payments and fail to incorporate carbon regulation instruments or preferential financing terms aligned with green taxonomy. As a result, such assessments tend to be less accurate. By refining existing tools, it becomes possible to account for the nuances of climate regulation and quantitatively assess the environmental impacts of these projects.
For instance, a company can evaluate the potential revenue from selling carbon units over the entire project lifecycle, compare the result with capital and operating costs, assess the reduction in tax payments, and analyze how environmental impact payments will change due to the decarbonization project.
Moreover, for a comprehensive analysis and decomposition of quantitative assessment components, it is crucial not to overlook the qualitative stage of evaluation. This stage identifies whether the project affects the surrounding social and natural environments and whether new types of products emerge from its implementation, facilitating better synchronization of the decarbonization project with other aspects of corporate economic activities.
Another important aspect involves assessing specific parameters – such as energy consumption and coefficients of economic and climate efficiency (see Table 2) which will support informed decision-making when comparing indicators obtained in previous assessment stages under otherwise equal conditions. Future research could focus on adapting budgeting tools for both decarbonization projects and broader applications.
Conclusion
In developing a methodology for the environmental and economic assessment of decarbonization projects in the oil and gas sector, we identified internal and external factors influencing project selection, determined the economic, climatic, and environmental conditions necessary for ranking these projects, and proposed a five-stage process for selecting, assessing, and ranking potential decarbonization initiatives. This process includes evaluating project feasibility and compliance with established criteria, which are structured according to increasing priority. Additionally, we introduced tools for quantitative assessment and comparative analysis of decarbonization projects using both absolute and specific indicators.
The selection of optimal decarbonization projects for an oil and gas company can be achieved through qualitative and quantitative analyses, encompassing a multi-criteria assessment of projects based on climatic, environmental, economic, and social factors. The diverse nature of decarbonization projects necessitates a universal mechanism for comparative analysis, as proposed in this study. Clarifying priorities and establishing additional criteria set by companies will improve management decision-making methods aimed at achieving GHG emission reduction goals and facilitate the automation of greenhouse gas emission management processes.
References
- Zhoujie Wang, Songyan Li, Zhijun Jin et al. Oil and gas pathway to net-zero: Review and outlook. Energy Strategy Reviews. 2023. Vol. 45. N 101048. DOI: 10.1016/j.esr.2022.101048
- Cherepovitsyna A., Sheveleva N., Riadinskaia A., Danilin K. Decarbonization Measures: A Real Effect or Just a Declaration? An Assessment of Oil and Gas Companies’ Progress towards Carbon Neutrality. Energies. 2023. Vol. 16. Iss. 8. N 3575. DOI: 10.3390/en16083575
- Grushevenko E., Kapitonov S., Melnikov Yu. et al. Decarbonization of the oil and gas industry: International experience and Russia’s priorities. Moscow: Moskovskaya shkola upravleniya SKOLKOVO, 2021, p. 158 (in Russian).
- Romasheva N., Cherepovitsyna A. Renewable Energy Sources in Decarbonization: The Case of Foreign and Russian Oil and Gas Companies. Sustainability. 2023. Vol. 15. Iss. 9. N 7416. DOI: 10.3390/su15097416
- Sheveleva N.A. Decarbonization strategies and methods for oil and gas sector. Environmental protection in oil and gas complex. N 2 (311), p. 25-31 (in Russian). DOI: 10.33285/2411-7013-2023-2(311)-25-31
- Cherepovitsyn A.E., Tsvetkov P.S., Evseeva O.O. Critical analysis of methodological approaches to assessing sustainability of arctic oil and gas projects. Journal of Mining Institute. Vol. 249, p. 463-478. DOI: 10.31897/PMI.2021.3.15
- Kirillov I.S. An assessment of investment projects in the Russian oil and gas industry: Economic, social, and environmental aspects: dis. … kand. ekon. nauk. Moscow: Finansovyi universitet pri Pravitelstve Rossiiskoi Federatsii, 2013, p. 24 (in Russian).
- Vurdova N.G., Lishchuk A.N. The impact of environmental-economic risks on the effectiveness of the investment-construction project for the reconstruction of wastewater treatment facilities of oil refineries. Environmental protection in oil and gas complex. N 3 (312), p. 23-31 (in Russian). DOI: 10.33285/2411-7013-2023-3(312)-23-31
- Yarygin G.A., Vilchek G.E. Utilizing strategic environmental assessment to optimize offshore oil and gas projects. Neftegaz.RU. 2013. N 11-12, p. 42-45 (in Russian).
- Dyakov M.Yu., Mikhailova E.G., Sharakhmatova V.N. Strategic environmental assessment in regional planning. Problems of Territory’s Development. N 2 (100), p. 80-94 (in Russian). DOI: 10.15838/ptd.2019.2.100.5
- Krainova E.A., Mushba B.V. Ecological and economic balance of decision-making during the energy transition to decarbonization of the oil and gas industry. Equipment and technologies for oil and gas complex. 2022. N 5 (209), p. 37-44 (in Russian). DOI: 10.33285/1999-6942-2022-5(209)-37-44
- Pinaev V.E. Modeling of assessment system for oil and gas projects industry case study YaNAO. Naukovedenie. 2016. Vol. 8. N 4, p. 13 (in Russian).
- Novoselova I.Yu., Novoselov A.L. Environmental multiplier of federal and regional budgets’ participation in mining companies’ projects. Economics, taxes & law. 2022. Vol. 15. N 3, p. 99-109 (in Russian). DOI: 10.26794/1999-849X-2022-15-3-99-109
- Novoselova I.Yu., Novoselov A.L. Modeling of regional trade in environmental pollution quotas. Economics, taxes & law. Vol. 15. N 4, p. 96-106 (in Russian). DOI: 10.26794/1999-849X-2022-15-4-96-106
- Vetrova M.A., Bogdanova A.A., Yarullina I.E. Decarbonization of oil industry in the context of circular development of economy. Problems of Modern Economics. 2021. N 3 (79), p. 196-199 (in Russian).
- Rekord S.I., Kulikov D.V. International aspects in formation of technological model of decarbonization of natural gas. Problems of Modern Economics. 2019. N 3 (71), p. 176-180 (in Russian).
- Kozenyasheva M.M., Savinova A.A. Decarbonization in the field of international shipping. Equipment and technologies for oil and gas complex. N 1 (217), p. 60-65 (in Russian). DOI: 10.33285/1999-6942-2023-1(217)-60-65
- Zaedinov A.V. Modernization of the business-model of climate projects in the context of formation of the Russian sequestration industry. Problems of Modern Economics. 2023. N 2 (86), p. 202-206 (in Russian).
- Jenvey N. Technology Focus: Decarbonization. Journal of Petroleum Technology. 2021. Vol. 73. Iss. 7, p. 64. DOI: 10.2118/0721-0064-JPT
- Danilin K.P., Cherepovitsyna A.A., Beloshitskiy A.V. Oil and gas companies’ greenhouse gases emissions reporting: Scope 3. Oil Industry Journal. 2023. N 5, p. 139-144 (in Russian). DOI: 10.24887/0028-2448-2023-5-139-144
- Kurka T. Application of the analytic hierarchy process to evaluate the regional sustainability of bioenergy developments. Energy. 2013. Vol. 62, p. 393-402. DOI: 10.1016/j.energy.2013.09.053
- Ilinova А.А., Romasheva N.V., Stroykov G.A. Prospects and social effects of carbon dioxide sequestration and utilization projects. Journal of Mining Institute. Vol. 244, p. 493-502. DOI: 10.31897/PMI.2020.4.12
- Singh H., Chengxi Li, Peng Cheng et al. Real-Time Optimization and Decarbonization of Oil and Gas Production Value Chain Enabled by Industry 4.0 Technologies: A Critical Review. SPE Production & Operation. 2023. Vol. 38. Iss. 3, p. 433-451. DOI: 10.2118/214301-PA
- Ponkratov V.V., Kuznetsov A.S., Muda I. et al. Investigating the Index of Sustainable Development and Reduction in Greenhouse Gases of Renewable Energies. Sustainability. 2022. Vol. 14. Iss. 22. № 14829. DOI: 10.3390/su142214829
- Agbaji A.L., Morrison R., Lakshmanan S. ESG, Sustainability and Decarbonization: An Analysis of Strategies and Solutions for the Energy Industry. SPE EuropEC – Europe Energy Conference featured at the 84th EAGE Annual Conference & Exhibition, 5-8 June 2023, Vienna, Austria. OnePetro, 2023. N SPE-214346-MS. DOI: 10.2118/214346-MS
- Cortez M.C., Andrade N., Silva F. The environmental and financial performance of green energy investments: European evidence. Ecological Economics. 2022. Vol. 197. N 107427. DOI: 10.1016/j.ecolecon.2022.107427
- Boguslavsky D.V., Sharov K.S., Sharova N.P. Using Alternative Sources of Energy for Decarbonization: A Piece of Cake, but How to Cook This Cake? Environmental Research and Public Health. 2022. Vol. 19. Iss. 23. N 16286. DOI: 10.3390/ijerph192316286
- Tsai W.-H. Carbon Emission Reduction – Carbon Tax, Carbon Trading, and Carbon Offset. Energies. 2020. Vol. 13. Iss. 22. N 6128. DOI: 10.3390/en13226128
- Al Kindi O.M., Al Hinai S., Ghefeili H. et al. Decarbonization Will Not Come for Free: Asset-M Marginal Abatement Cost Curve. SPE EuropEC – Europe Energy Conference featured at the 84th EAGE Annual Conference & Exhibition, 5-8 June 2023, Vienna, Austria. OnePetro, 2023. N SPE-214414-MS. DOI: 10.2118/214414-MS
- Yasemi S., Khalili Y., Sanati A., Bagheri M. Carbon Capture and Storage: Application in the Oil and Gas Industry. Sustainability. 2023. Vol. 15. Iss. 19. N 14486. DOI: 10.3390/su151914486
- Telegina E.A., Chapaikin D.A. Directions of the Energy Transition in the Policy of Global Oil and Gas Companies. Studies on Russian Economic Development. Vol. 33. N 5, p. 555-560. DOI: 10.1134/S1075700722050148
- Gorbacheva N.V. Comparative analysis of decarbonising economy in Siberia and Scandinavia megaregions: Price, value and values of energy. Voprosy Ekonomiki. N 10, p. 124-148 (in Russian). DOI: 10.32609/0042-8736-2023-10-124-148
- Porfiriev B.N., Shirov A.A., Kolpakov A.Y., Edinak E.A. Opportunities and risks of the climate policy in Russia. Voprosy Ekonomiki. N 1, p. 72-89 (in Russian). DOI: 10.32609/0042-8736-2022-1-72-89
- Kolpakov A.Yu. Energy Efficiency: Its Role in Inhibiting Carbon Dioxide Emissions and Defining Factors. Studies on Russian Economic Development. 31. N 6, p. 691-699. DOI: 10.1134/S1075700720060076
- Yuh-Ming Tsai, Cherng-Yuan Lin. Effects of the Carbon Intensity Index Rating System on the Development of the Northeast Passage. Journal of Marine Science and Engineering. 2023. Vol. 11. Iss. 7. N 1341. DOI: 10.3390/jmse11071341
- Glebov L.S., Glebova E.V. CO2 emission in the production and use of diesel fuel and biodiesel. Environmental protection in oil and gas complex. N 2 (299), p. 23-27 (in Russian). DOI: 10.33285/2411-7013-2021-2(299)-23-27
- Karaeva A., Magaril E., Al-Kayiem H. et al. Approaches to the assessment of ecological and economic efficiency of investment projects: Brief review and recommendations for improvements. WIT Transactions on Ecology and the Environment. 2021. Vol. 253, p. 515-525. DOI: 10.2495/SC210421
- Makepa D.C., Chihobo H.C., Musademba D. Techno-economic analysis and environmental impact assessment of biodiesel production from bio-oil derived from microwave-assisted pyrolysis of pine sawdust. Heliyon. 2023. Vol. 9. Iss. 11. N e22261. DOI: 10.1016/j.heliyon.2023.e22261