Radiation characteristics of coals at different stages of metamorphism
- 1 — Ph.D. Leading Engineer Federal Research Center of Coal and Coal Chemistry, Siberian Branch of the RAS ▪ Orcid
- 2 — Ph.D., Dr.Sci. Chief Researcher Federal Research Center of Coal and Coal Chemistry, Siberian Branch of the RAS ▪ Orcid
- 3 — Ph.D., Dr.Sci. Leading Researcher Federal Research Center of Coal and Coal Chemistry, Siberian Branch of the RAS ▪ Orcid
Abstract
The formation of deposits and subsequent metamorphic processes that affect concentrations of radioactive elements in coal can indicate ongoing geological activities, therefore, analyzing trends in the radiation characteristics of coal throughout the metamorphic series is highly relevant. The aim of this work is to experimentally evaluate the radiation characteristics of different coal ranks (metamorphic stages) using thermoluminescent (TL) dosimetry and beta activity measurements, and to identify correlations between these radiation characteristics and data obtained from technical, elemental, and thermogravimetric analyses, as well as mass spectrometric and electron paramagnetic resonance spectroscopy (EPR) measurements. For dosimetric measurements that indirectly characterize the content of radionuclides in coal, a modified dosimetric complex and original soil-equivalent thermoluminescent detectors based on SiO2 were used. The analysis of the obtained results supports the use of TL studies to determine the ash content of coals at low and medium stages of metamorphism (coal rank B→G), while indicating that this method is not feasible for coals at higher stages of metamorphism. The correlation dependencies in the metamorphism series suggest abrupt change in the conditions of coal formation during the time range corresponding to transformation from high to low volatile bituminous coals (coal rank G→Zh→K). These abrupt changes in regional metamorphism conditions (time, temperature, pressure, oxidation-reduction conditions) are confined to the boundary of the Permian and Triassic periods (~250 million years ago), during which both the transformation of existing coal deposits and the formation of new deposits occurred.
Funding
This work was carried out as part of the state assignment of the Institute of Coal Chemistry and Material Science, Federal Research Center for Coal and Coal Chemistry, Siberian Branch of the RAS (project 121031500513-4) using the equipment of the Shared Research Facilities of the Federal Research Center for Coal and Coal Chemistry, Siberian Branch of the RAS.
Introduction
The radioactivity of coal, like that of other natural objects, is determined by the content of natural uranium and thorium in equilibrium with their decay products, as well as the content of a number of beta-emitting long-lived isotopes (40K, 50V, 87Rb, 115In, 123Te, 138La, 176Lu, 187Re), the main one being 40K due to its high content [1-3].
The content of 238U and the equilibrium amount of 226Ra in solid coal matrix generally corresponds to their content in soils and is at safe concentration levels. Due to the good solubility of 226Ra, equilibrium distribution can be disrupted. The decay of 226Ra leads to the appearance of a gaseous short-lived decay product (222Rn), whose potential release into the inter-pore space from the solid matrix usually disrupts the natural radioactive equilibrium in the 238U series [3-5]. In equilibrium with its nearest short-lived decay products, in the absence of a new source of 222Rn, it rapidly decays according to an exponential law, so emanation can only be detected at the location of a constant radon source [3]. The short-lived decay products of 222Rn produce α-, β- and γ- radiation.
Radioactive impurities are present in both the organic and ash fractions of coal. Thorium is mainly found in phosphate minerals (monazite, apatite), while uranium is present both in mineral inclusions – uranium-thorium-containing minerals (zirconium, pyrochlore, monazite, or their own minerals) – and in the organic fraction as organometallic compounds. The organic part usually contains an order of magnitude less uranium than the inorganic part (which determines the ash content); therefore, after coal combustion, most of the uranium, thorium and their decay products are retained in solid waste (the specific concentration of radioactive elements in ash is 5-7 times higher than in the original coal) [2, 6-8]. Elevated levels of uranium and thorium in coals are often accompanied by the accumulation of rare-earth elements, zirconium, hafnium, and other rare elements [6-8].
In general, the radionuclide content in the coals of the Kuznetsk Basin is not high and is comparable to that in soils [3, 9]. The worst radiation levels in Kuzbass are found in the brown (lignite and sub-bituminous) coals of the Kansk-Achinsk Basin, mined in the Kemerovo region at the Itatsky open-pit mine [3, 6-9]. The uranium content in coals and host rocks of the Itatsky deposit is several times higher than the values typical for Kuzbass (uranium, average – 56.9 g/t, variations – 6-139 g/t) [3, 7]. The distribution of uranium across the open-pit area is localized, with areas of elevated content occupying ~20 % of the area [3, 7]. In the ash and slag material formed during the combustion of such coal, the uranium content reaches up to 900 g/t, and this material is classified according to global standards as ordinary uranium ore (uranium content range of 0.05-0.1 %) [9-11].
Since radionuclides are concentrated in 10-20 % of the mineral fraction of coal, radioactivity can reflect the mineral content of coal and correlate with the ash content of samples [12-14].
This study investigates the radiation characteristics of coals from the Kuznetsk Basin across different stages of metamorphism and correlates the obtained data with the results of technical analysis [15-17] and other physicochemical studies (elemental and thermogravimetric analyses, mass spectrometric and EPR studies) [18-21], with the aim to determine the feasibility of using the radiation characteristics of coal to assess ash content and analyze changes in radiation characteristics across the metamorphic series.
Methods
Samples and research methodology
Coals (Table 1) from the metamorphic series with particle sizes ≤ 3 mm were studied [13-15, 19]. A representative sample of coals was transferred into a plastic container (1 liter), filling it to 2/3, with thermoluminescent detectors placed inside. Thus, the detectors were surrounded on all sides by a layer of coal and were kept in the sample for 20 days.
For all selected representative samples from the metamorphic series, comprehensive information was available regarding the coal mine of provenance, technical characteristics (Table 1), and the results of extended physicochemical analyses conducted at the Shared Research Facilities of the Federal Research Center for Coal and Coal Chemistry, Siberian Branch of the RAS [13-15].
Table 1
Characteristics of coal samples
Analytical sample description |
Coal rank |
Ad, % |
Vdaf, % |
Wa, % |
N 27, open-pit mine “Kaichaksky” |
B |
10 |
53.1 |
11.8 |
N 72, open-pit mine “Kamyshansky” |
D |
6.2 |
44.5 |
7.6 |
N 64, mine “V.D.Yalevsky”, seam 52 |
DG |
4.7 |
42.6 |
5.7 |
N 40, mine “S.M.Kirov”, seam Polenovskiy |
G |
3.3 |
40.4 |
1.2 |
N 15, mine “S.D.Tikhova”, seam 23 |
Zh |
7.8 |
33.3 |
0.8 |
N 10, OOO “Uchastok Koksovy”, seam II internal |
K |
4.9 |
21.3 |
0.6 |
N 34, open-pit mine “Tomusinsky” |
OS |
6.7 |
19.8 |
0.1 |
N 45, open-pit mine “Bachatsky” |
SS |
4.7 |
19.0 |
1.3 |
N 81, AO “Kuznetskinveststroy”, seam 19a |
Т |
6.2 |
14.4 |
0.5 |
Notes: Ad – ash content; Vdaf – volatile matter yield; Wa – analytical moisture content.
Equipment
The study of absorbed doses accumulated in coals due to the presence of radioactive elements was conducted using the thermoluminescence dosimetry method [3, 22, 23]. A modified dosimetric complex DTU-01M was used, providing signal registration in the temperature range up to 450 °C with the ability to change the heating rate from 1 to 20 °C/s. The DTU-01M dosimetric devise was calibrated together with TLD-K detectors based on SiO2, which were placed in the analyzed sample for a fixed exposure period, and the absorbed doses caused by the radionuclide content in the coal were calculated based on the detector readings [3, 24]. Because the effective atomic number, Zeff, of the detectors closely corresponds to that of quaternary deposits, the detectors correctly measure the absorbed dose [3, 23, 24].
After exposure, the detectors were placed on the heating element of the calibrated DTU-01M complex, and the absorbed dose was measured in centigrays (cGy). To register background radiation, the detectors were also placed in a container without coal. The background radiation was subtracted to quantify the annual dose due to the content of radionuclides in coal, in excess of the background dose.
Due to the lack of hygroscopicity, TLD-K detectors can be used for dosimetry of aqueous media, i.e. for studying the characteristics of mine waters, settling ponds, discharges, and drinking surface and borehole waters [3].
To determine the beta activity of coals (Bq/kg), a KRVP-3B radiometer with a large lead chamber was used. The activity of dried potassium chloride powder, which has a standard beta activity due its 40K content, was measured as a reference sample.
EPR measurements were carried out using a Bruker EMX 6/1 micro spectrometer in the X-band (9.5 GHz) with the following settings:
- magnetic field sweep range ΔH = 1300-5600 Gs;
- signal gain coefficient 2.24·102;
- microwave power attenuation 20 dB;
- signal conversion time 15 ms;
- signal sweep time 31 s.
Results
The results of dosimetry and determination of beta activity of coal samples are presented in Table 2. Each sample contained at least five detectors; Table 2 shows the average values and deviations for the samples. The average deviation did not exceed 3 % for any of the samples. The results indicate that specific beta activities and the absorbed doses in addition to the background are correlated, decreasing with increase in coal metamorphism from grade B to grade Zh; and highly metamorphosed coals are more radioactive than moderately metamorphosed coals.
Table 2
Results of dosimetry and determination of beta activity of coal samples
Coal rank |
Dose during monitoring D, cGy |
Average deviation ΔD, cGy |
Annual absorbed dose, cGy/year |
Annual absorbed dose minus background, cGy/year |
Specific beta activity A, Bq/kg |
B |
0.0225 |
0.0005 |
0.411 |
0.081 |
75 |
D |
0.0208 |
0.0004 |
0.380 |
0.050 |
46 |
DG |
0.0202 |
0.0004 |
0.369 |
0.039 |
37 |
G |
0.0196 |
0.0004 |
0.358 |
0.028 |
27 |
Zh |
0.0190 |
0.0004 |
0.346 |
0.016 |
16 |
K |
0.0221 |
0.0005 |
0.404 |
0.074 |
72 |
OS |
0.0235 |
0.0007 |
0.428 |
0.098 |
84 |
SS |
0.0225 |
0.0006 |
0.410 |
0.080 |
60 |
T |
0.0241 |
0.0007 |
0.440 |
0.110 |
62 |
Correlation analysis of radiation characteristics of coals in the metamorphism series with other physicochemical characteristics. Figure 1 shows the dependencies of absorbed doses on moisture content and volatile matter yield, along with the correlation coefficients when approximating the data with a linear relationship.
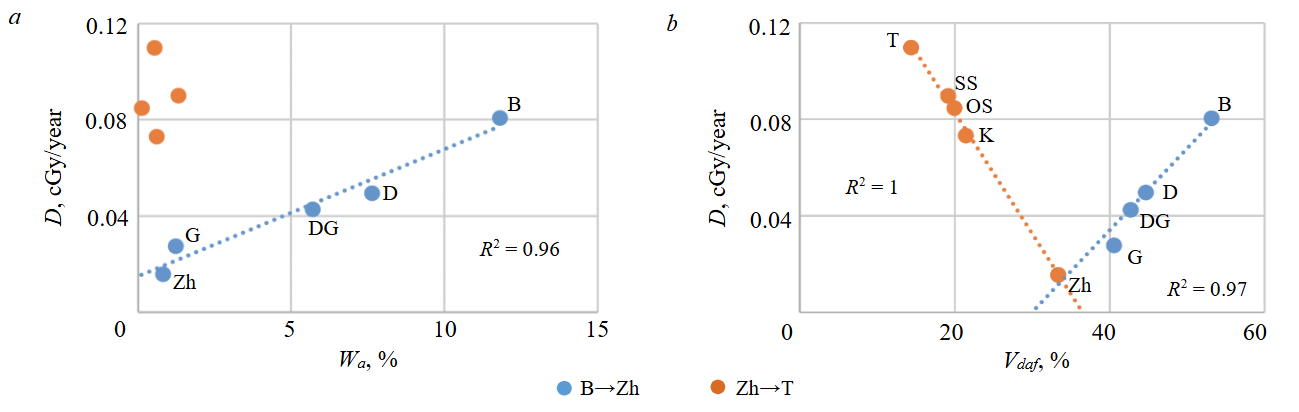
Fig.1. Dependence of the absorbed dose, formed in coals due to radioactive elements, on moisture content (a) and volatile matter yield (b)
Comparison of radiation characteristics with the results of technical analysis of coal samples in the B→T series shows:
- the dependence of the absorbed dose on the degree of coal metamorphism in the B→T series is complex. From B→Zh, radioactivity decreases to the minimum values in the Zh sample, and then, with increasing metamorphism, radioactivity increases from Zh→T (Fig.1).
- coals with higher moisture content in the Zh→B series (decreasing degree of metamorphism) are more radioactive, suggesting that some radionuclides are in water-soluble compounds.
- the radioactivity of coals increases with a volatile matter yield of more than 35 % (in the Zh→B series). For highly metamorphosed coals (in the T→Zh series), radioactivity decreases with a volatile matter yield in the range of 15 to 35 %. This trend suggests that some radionuclides are associated with the organic fraction of the coal.
Since radionuclides are mainly concentrated in the ash part of coal, let us consider the dependence of the absorbed dose D on the ash content Ad (Fig.2).
For coal samples in the G→B series, the ash content of the samples increases from 3 to 10 %, and in this series, a linear increase in absorbed doses is observed (Fig.2, a). When the ash content range is expanded to 25 % (including samples of G and D coals with high ash content from the “Zarechnaya” mine), samples with higher ash content fit well into the linear dependence of dose on ash content (Fig.2, b). This allows for a preliminary conclusion about the possibility of determining the ash content of coal samples of low and medium degrees of metamorphism based on their radiation characteristics. An additional argument confirming the possibility of using dosimetric studies of coals to determine ash content is the constancy of doses normalized to ash content in the B→G series. The slight change in absorbed doses per unit ash content in the B→G series of metamorphosed coals may contribute minimally to the error in determining ash content based on radioactivity.
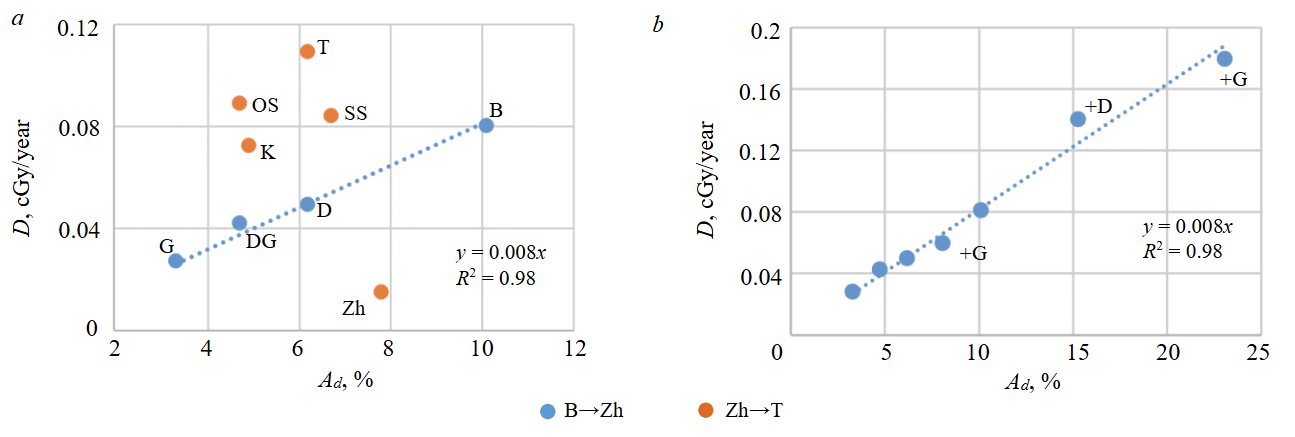
Fig.2. Dependence of the absorbed dose on the ash content of coal samples Ad = 3-10 % (a) and up to 25 % (b)
For samples in the Zh→T series, no dependence of the dose on the ash content is observed (Fig.2, a). It should be noted that the dose per unit ash content for K→T coals, with a wide range of values, is almost twice as high as for low- and medium-metamorphosed coals. This may indicate the presence of radionuclides in the organic matrix of highly metamorphosed coals. The Zh coal sample stands out with a low value of the dose normalized to ash content.
When comparing the radiation characteristics of coals in the metamorphism series with the results of elemental analysis of organic elements in coal samples in the B→Zh series, the following patterns are observed (Fig.3):
- decrease in the radioactivity of samples with an increase in the content of carbon, nitrogen, and hydrogen (increase in the degree of metamorphism);
- increase in absorbed doses with an increase in oxygen content (decrease in the degree of metamorphism);
- coals with higher degrees of metamorphism show a change in the trend and reveal different tendencies in the Zh→T series;
- with an increase in the degree of metamorphism in the Zh→T series, absorbed doses increase after passing the minimum for Zh coals.
The decrease in absorbed doses with an increase in nitrogen concentration in the B→Zh series (increase in the degree of metamorphism) changes to an increase in dose with a decrease in nitrogen content when transitioning to higher degrees of metamorphism in the Zh→T series. The turning point is the Zh coal sample.
A characteristic inflection of the characteristics when crossing the degree of metamorphism Zh is observed for oxygen content at a concentration of ~15 %, carbon at a concentration of ~78 % (Fig.3), and for volatile matter content at a concentration of ~35 % in coal samples (see Fig.1). Absorbed doses pass through a minimum at certain concentrations and then increase with the degree of metamorphism. The observed patterns likely result from sharply changing conditions of coal formation or transformation (time, temperature, pressure, etc.) during the formation of coal deposits of grades G→Zh→K.
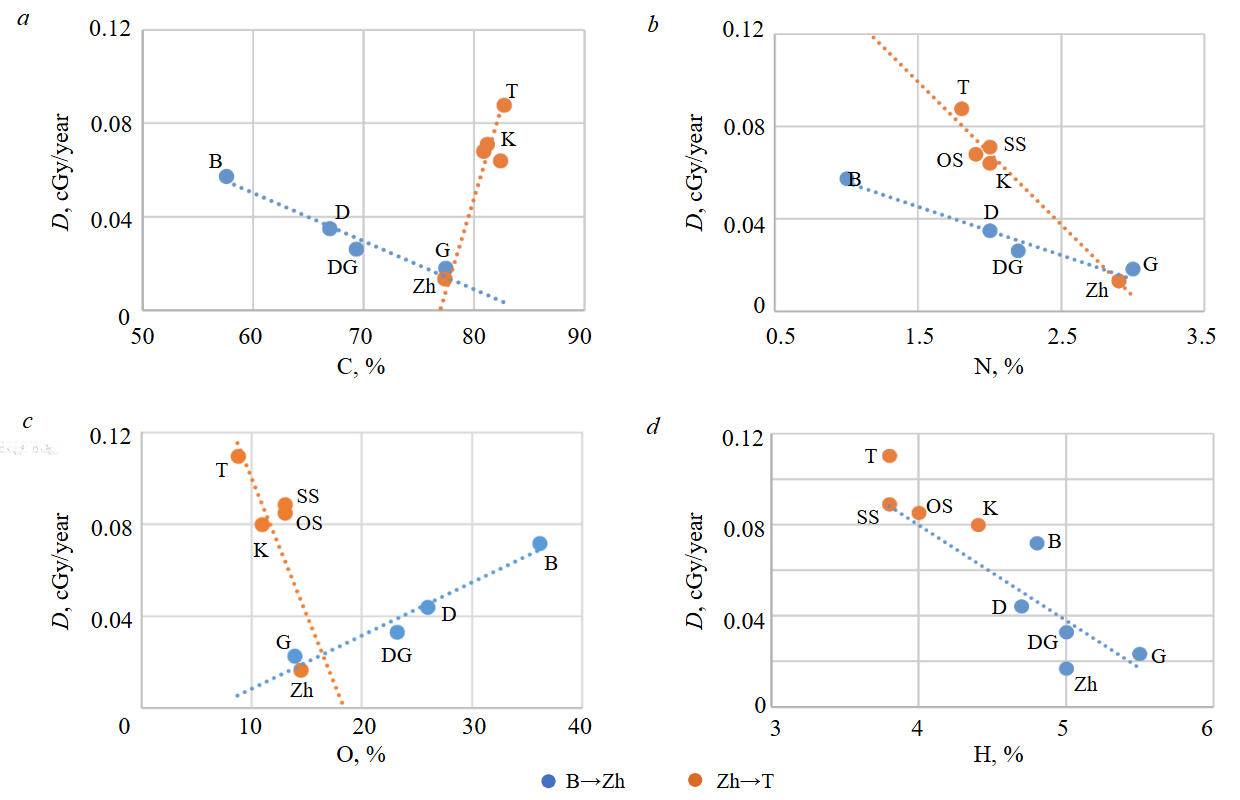
Fig.3. Dependence of the absorbed dose on the content of carbon (a), nitrogen (b), oxygen (c) and hydrogen (d) in the metamorphism series
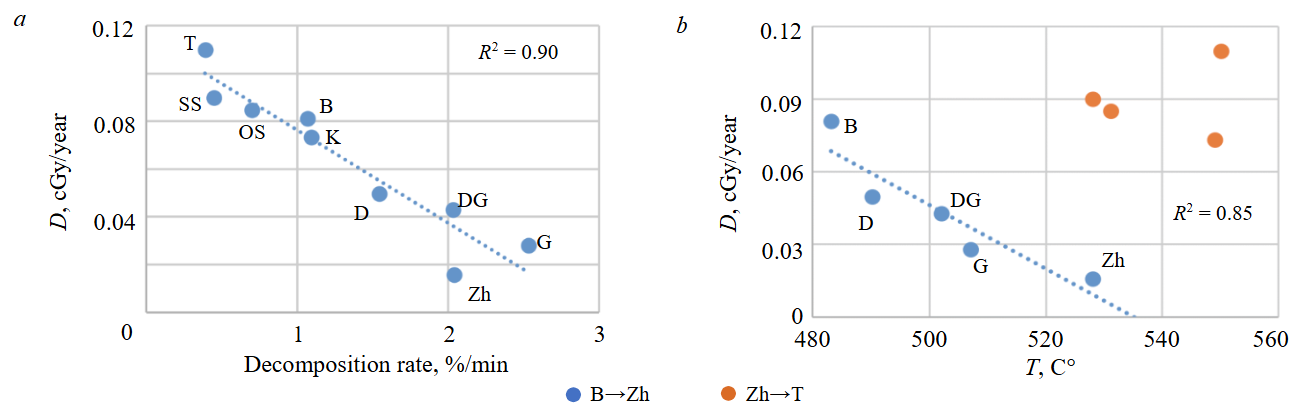
Fig.4. Correlation dependences of absorbed doses and degrees of metamorphism on the rates of coal decomposition (a) and the temperatures of maximum H2O release in an inert environment (b)
The dependence of absorbed doses and degrees of metamorphism on the rates of coal decomposition and the temperatures of maximum H2O release in an inert environment in Fig.4 is multidirectional. With an increase in the decomposition rate of coal samples in an inert environment, a decrease in absorbed dose is observed for T→K coals (decrease in the degree of metamorphism) and for B→Zh coals (increase in the degree of metamorphism), with minimum dose values for the metamorphism stages G and Zh (Fig.4). A similar trend is observed for the dependence of absorbed dose on hydrogen concentration (see Fig.3). The maximum temperatures of H2O release during decomposition in an inert environment in the B→Zh series increase with a decrease in absorbed doses.
The distribution of carbon across structural fragments – carbonyl (C=O); carboxyl (COOH); aromatic carbon atoms (Car); aromatic carbon atoms bonded to oxygen atoms (Car–O); protonated aromatic carbon atoms (Car–H); methoxyl fragments (OCH3); alkyl fragment carbon atoms (CH2) – modeled based on NMR studies in an inert environment, shows diverse behavior of fragments depending on absorbed doses (degrees of metamorphism of samples) (Fig.5). With the increase in the degree of metamorphism and the decrease in absorbed doses in the B→Zh series, there is an increase in the content of Car, as well as CH3 and OCH3 fragments, and a decrease in the amount of Car–H, Car–O, CH2 fragments, C–O–C, COOH, and C=O. The correlation dependencies of Car, CH2, C–O–C, and COOH on the absorbed dose for the entire studied metamorphism series are shown in Fig.5.
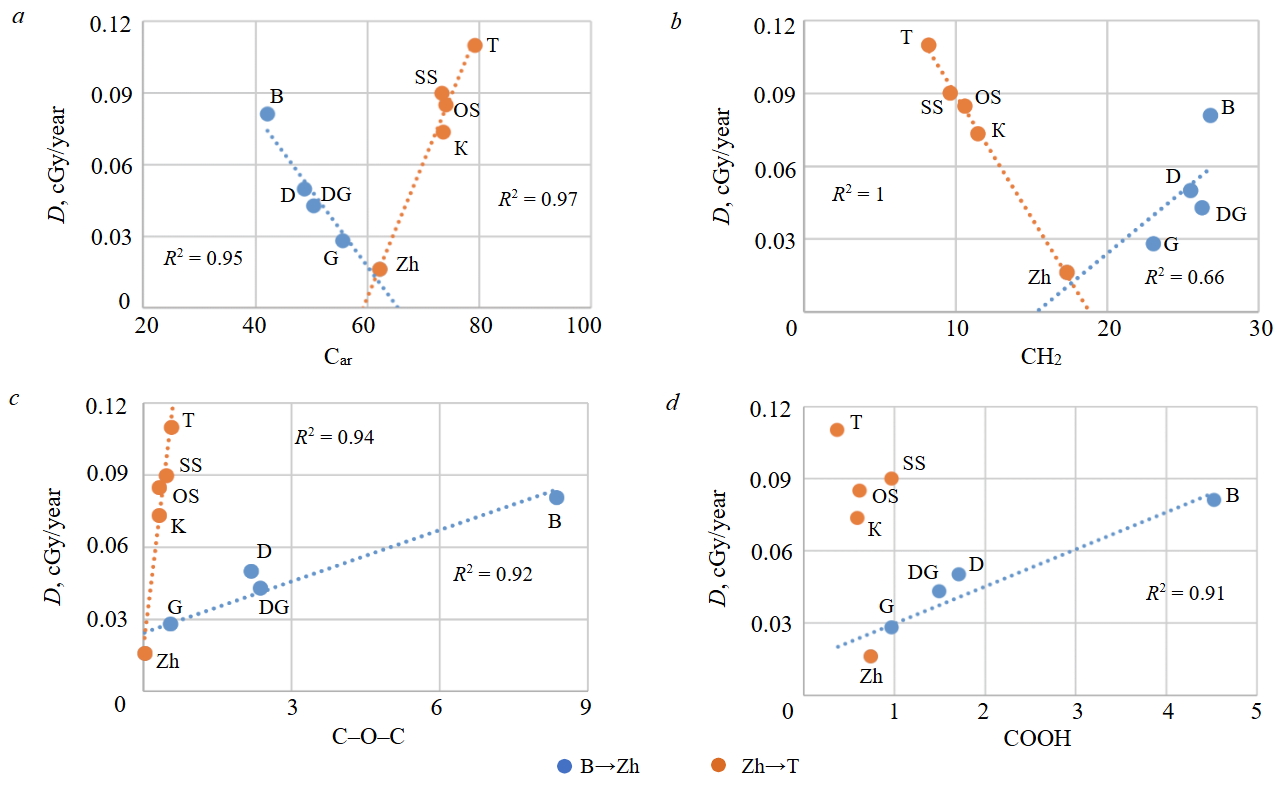
Fig.5. Correlation dependences of absorbed doses and degrees of metamorphism from structural fragments of carbon in the series B→T: Car (a); CH2 (b); C–O–C (c) and COOH (d)
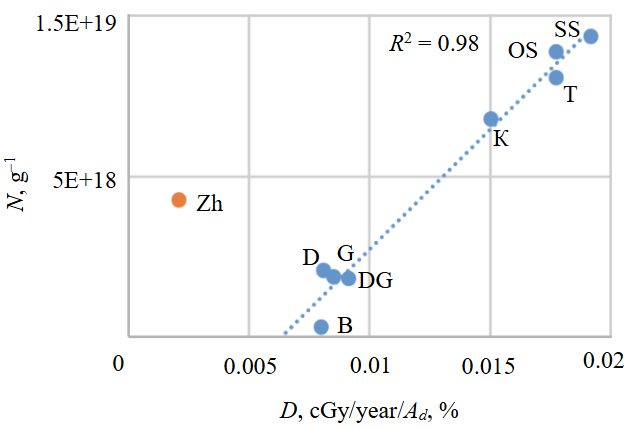
Fig.6. Dependence of the number of paramagnetic centers in a gram of coal on the absorbed dose normalized to ash content (for coal with unit ash content)
As in Figs.1 and 4, a characteristic inflection of the dependencies is observed when coals pass through the degrees of metamorphism G→Zh→K, with a turning point at the Zh stage. For coals of high metamorphism stages, an increase in dose with an increase in the degree of metamorphism is observed, along with an increase in Car and C–O–C content and a decrease in CH2 content.
Figure 6 shows the dependence of the number of paramagnetic centers on the dose normalized to ash content, i.e., the dose per unit ash content. Coals exhibit paramagnetism due to the presence of various types of paramagnetic centers (defects) [25-27]. Paramagnetic centers can accumulate in coal due to prolonged exposure to alpha-emitting radionuclides contained in them [28, 29].
With an increase in the degree of metamorphism, there is an increase in the normalized absorbed dose and the number of paramagnetic centers. Along with the radioactivity of coals, the time factor also plays a role in the observed dependence. The younger the coal, the less time it is exposed to radiation from radionuclides contained in the sample or the surrounding rocks, and the fewer paramagnetic centers it contains. The older the coal, the longer the exposure time and the higher the accumulated number of paramagnetic centers.
The Zh coal sample deviates from the dependence, as it has a high number of paramagnetic centers despite the minimum absorbed dose and average age. It can be assumed that, in addition to the considered radiation factor, more complex specific conditions of defect formation (temperature, pressure, etc.) are responsible for their creation.
Discussion of results
The changes in radioactivity in the coal metamorphism series at low and medium stages of metamorphism are generally predictable and are determined by the time frames of coal formation and the half-lives of the main present radionuclides [1-3]. The age of the oldest coals is estimated at 3.5·108 years, and the half-lives of the main radionuclides are comparable to this age (Table 3).
Table 3
Characteristics of decay and activity estimates of the main radionuclides contained in coal
Radionuclide |
Half-life, coal age (years) |
Specific activity, Bq/g |
Radionuclide content in natural element mixture, % |
Average radionuclide content in coal, g/t |
Average radionuclide activity in coal, Bq/kg |
40K |
1.25E+09 |
2.65E+05 |
0.0119 |
0.1 |
29.3 |
238U |
4.5E+09 |
1.2E+04 |
99.3 |
1.9 |
23.4 |
235U |
7.1E+08 |
7.9E+04 |
0.7 |
1.2E-02 |
0.96 |
234U |
2.45E+05 |
2.3E+08 |
0.0055 |
0.0 |
0.0 |
232Th |
1.4E+10 |
3.1E+03 |
100 |
6.9 |
21.4 |
Coal |
3.5E+08 |
0.075 |
Σ75 |
After the formation of the radionuclides in the coal, coal activity naturally decreases over time, which may explain the observed decrease in activity in the B→K series with comparable concentrations of radionuclides during formation. With an increase in the degree of metamorphism due to prolonged irradiation of the coal mass by radionuclides contained in the sample, the number of radiation defects should increase, which is observed experimentally in EPR studies. Thus, the time factor of metamorphism explains the observed dependence of changes in the radioactivity of samples in the series of weakly metamorphosed samples. For highly metamorphosed samples, it is necessary to assume the presence of radionuclides in both the mineral and organic components of coals, with higher radionuclide content in the biosphere during their formation.
A linear dependence of radioactivity on ash content is recorded at medium and low degrees of metamorphism (G→B) and can be used to determine the ash content of a sample by its radioactivity. When transitioning to higher degrees of metamorphism, the dependence becomes significantly more complex.
The dependencies of absorbed dose on volatile yield, carbon, oxygen, nitrogen concentrations, and carbon structural fragments shown in Figs.1, 3, and 5 demonstrate a sharp change in absorbed doses when transitioning from Zh coal to higher degrees of metamorphism. All dependencies undergo a sharp change in trend with a tendency for absorbed doses to increase in the K→T metamorphism series, contrary to what is observed at low degrees of metamorphism. The sharp change in the trend of dependencies can only be explained by invoking the hypothesis of significant changes in the conditions of formation or transformation of coal masses (temperature, depth, pressure, gas environment, mineralization, redox conditions) [26-29]. The change in radionuclide accumulation conditions in coals occurs during the stages of metamorphism G→Zh→K and is likely associated with global processes during their formation period, with more intense radionuclide input into biosphere objects during the formation of highly metamorphosed coals.
Changes in the trend of dependencies at the boundary of metamorphism G→Zh→K can be traced without involving radiation measurements if the volatile matter content in coal is taken as an indicator of the degree of metamorphism (Fig.7). A sharp change in the rates of carbon and oxygen accumulation, as well as the presence of maxima in hydrogen and nitrogen content, is observed when coals pass through the Zh metamorphism stage.
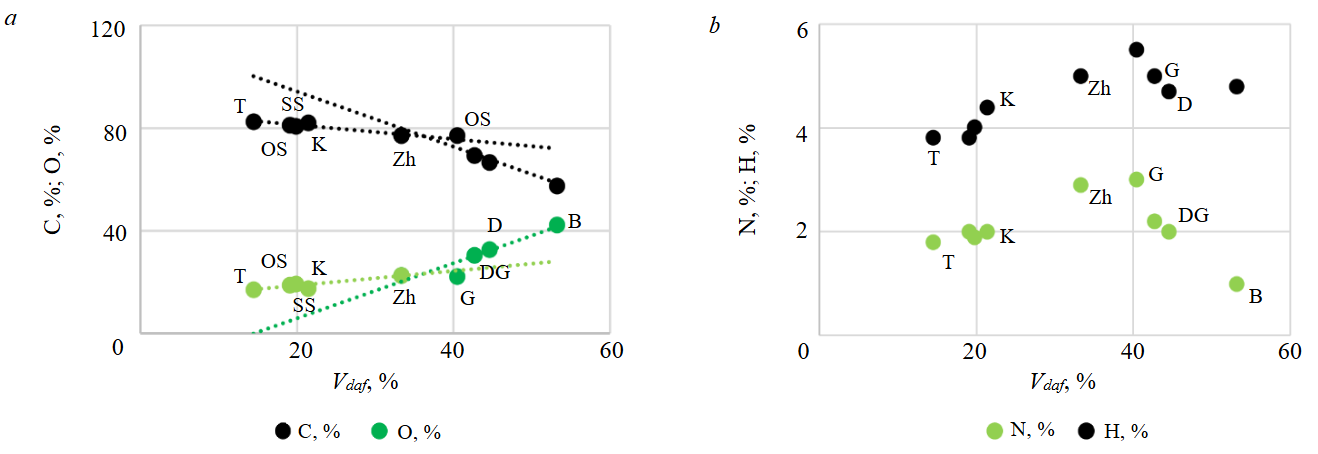
Fig.7. Dependence of concentrations of the main organic elements of coal on the content of volatile substances in coals of different stages of metamorphism: a – carbon, oxygen; b – nitrogen, hydrogen
The combination of identified factors may indicate changes in both radiation conditions and redox conditions in the biosphere during the formation period of Zh→G coals [30-32].
Conclusion
The analysis of the results supports the feasibility of using thermoluminescent analyses to determine the absorbed doses formed due to the coal radionuclide content, for determining the ash content of coal with low and medium degrees of metamorphism (B→G).
The observed correlations of absorbed doses with technical, elemental, and thermogravimetric analysis data, as well as EPR and mass spectrometric study results in the metamorphism series, indicate changes in coal formation (transformation) in the time range of the coals G→Zh→K, with abrupt change in conditions during this period.
Based on the analysis of the results, it can be assumed that the process of coal formation and metamorphism K→T spans the time range of the Carboniferous and Permian periods, while coals with lower degrees of metamorphism were formed after a major volcanic activity outbreak at the end of the Permian – early Triassic, which radically changed the structure of the Earth's biosphere. This period is characterized by the release of a large amount of “light” carbon into the atmosphere due to the combustion of surface coal deposits through which rising magma broke through [33-35].
The change in formation conditions is associated with a sharp change in regional metamorphism conditions (time, temperature, pressure, redox conditions) at the boundary of the Permian and Triassic periods (~250 million years ago). This time is characterized by volcanism, continental plate shifts, Siberian Traps eruptions, carbon release into the atmosphere, combustion processes with the deposition of a large amount of products, rapid sediment burial, and the catastrophic extinction of marine and terrestrial life forms on Earth [35-37].
References
- Rikhvanov L.P. Radioactivity and radioactive elements as the factor of the geological environment and his use in sciences about the earth. Prospect and protection of mineral resources. N 12, p. 55-61 (in Russian).
- Pak Y.N., Pak D.Y., Ponomaryova M.V. et al. Radioactivity of Coal and Its Combustion Wastes. Coke and Chemistry. Vol. 61. N 5, p. 188-192. DOI: 10.3103/S1068364X1805006X
- Aluker N.L., Artamonov A.S., Herrmann M. Radiation Monitoring as a Measure to Improve the Safety of Coal Mining. Instruments and Experimental Techniques. Vol. 65. N 4, p. 628-635. DOI: 10.1134/s0020441222040017
- Kochkin B.T. Reduced-Type Alterations at Exogenic Infiltration Uranium Deposits and Their Relation to Rising Groundwater. Geology of Ore Deposits. Vol. 62. N 1, p. 19-30. DOI: 10.1134/S1075701520010043
- Chekryzhov I.Yu., Popov N.Yu., Arbuzov S.I. Uranium and thorium in the coals of the Cainozoic basins of Primorye. Radioactivity and Radioactive Elements in Environment: Proceedings of VI International Conference, 20-24 September 2021, Tomsk, Russia. In 2 volumes. Tomsk: National Research Tomsk Polytechnic University, 2021. Vol. 1, p. 600-604 (in Russian).
- Sidorova G.P., Krylov D.A. Radiation hazard issues in the coal power generation industry. Mining Informational and Analytical 2017. N 11, p. 200-209 (in Russian). DOI: 10.25018/0236-1493-2017-11-0-200-209
- Fedorin V.A., Nifantov B.F., Anikin M.V., Borisov I.L. Valuable impurity elements in Kuzbass coals. Naukoemkie tekhnologii razrabotki i ispolzovaniya mineralnykh resursov. N 3, p. 85-88 (in Russian).
- Vyalov V.I., Nastavkin A.V. Concentration Levels of Industrially Valuable Trace Elements in Coals. Solid Fuel Chemistry. Vol. 53. N 5, p. 314-318. DOI: 10.3103/S0361521919050112
- Schastlivtsev E.L., Kovalev V.A., Potapov V.P. Monitoring the state of the natural environment in coal mining areas of Kuzbass. Novosibirsk: SO RAN, 2013. 312 с (in Russian).
- Artemev V.B., Galkin V.A., Makarov A.M. et al. Mechanism for preventing the occurrence of a hazardous production situation. Ugol. 2016. N 5, p. 73-77 (in Russian). DOI: 10.18796/0041-5790-2016-5-73-77
- Cheng-lin Miao, Meng-meng Duan, Xin-xiu Sun, Xin-yu Wu. Safety management efficiency of China’s coal enterprises and its influencing factors – Based on the DEA-Tobit two-stage model. Process Safety and Environmental Protection. Vol. 140, p. 79-85. DOI: 10.1016/j.psep.2020.04.020
- Legnaioli , Campanella B., Pagnotta S. et al. Determination of Ash Content of coal by Laser-Induced Breakdown Spectroscopy. Spectrochimica Acta Part B: Atomic Spectroscopy. 2019. Vol. 155, p.123-126. DOI: 10.1016/j.sab.2019.03.012
- Kamoza E.S., Mikhailova E.S., Ismagilov Z.R. Studying the effect the physicochemical properties of fine size raw coal organic and mineral composition have on beneficiation efficiency. News of the Higher Institutions. Mining Journal. N6, p. 65-75 (in Russian). DOI: 10.21440/0536-1028-2021-6-65-75
- Kraft Y.V., Gavrilyuk O.M., Ismagilov Z.R. Low-Temperature Pyrolysis of Kuznetsk Basin Coal: Composition of the Coke and Chemistry. 2023. Vol. 66. N 1, p. 17-22. DOI: 10.3103/S1068364X23700400
- Zhuravleva N.V., Khabibulina E.R., Ismagilov Z.R. et al. Studies of the interconnection of the structure of fossil coal and the concentrations of polycyclic aromatic hydrocarbons in it. Chemistry for Sustainable Development. Vol. 24. N 3, p. 355-361 (in Russian). DOI: 10.15372/KhUR20160310
- Jun Deng, Shuai-Jing Ren, Yang Xiao et al. Thermal properties of coals with different metamorphic levels in air atmosphere. Applied Thermal Engineering. 2018. Vol. 143, p. 542-549. DOI: 10.1016/j.applthermaleng.2018.07.117
- Vranjes-Wessely S., Misch D., Issa I. et al. Nanoscale pore structure of Carboniferous coals from the Ukrainian Donets Basin: A combined HRTEM and gas sorption study. International Journal of Coal Geology. 2020. Vol. 224. N 103484. DOI: 10.1016/j.coal.2020.103484
- Pengxiang Wang, Mingjun Liu, Tong Pei et al. Ultraviolet Raman spectra: The reasonable method of evaluating coal pyrolysis AIP Advances. 2020. Vol. 10. Iss. 11. N 115007. DOI: 10.1063/5.0018691
- Nelyubina N.V., Kraft Ya.V., Lyrshchikov S.Yu., Ismagilov Z.R. Investigation of the Correlation between the Energy Threshold of Laser Ignition of Coals and the Degree of Their Aromaticity. Chemistry for Sustainable Development. Vol. 29. N 5, p. 576-581. DOI: 10.15372/KhUR2021335
- Sheta S., Afgan S., Zongyu Hou et al. Coal analysis by laser-induced breakdown spectroscopy: a tutorial review. Journal of Analytical Atomic Spectrometry. 2019. Vol. 34. Iss. 6, p. 1047-1082. DOI: 10.1039/C9JA00016J
- Wenbing Li, Jidong Lu, Meirong Dong et al. Quantitative Analysis of Calorific Value of Coal Based on Spectral Preprocessing by Laser-Induced Breakdown Spectroscopy (LIBS). Energy & Fuels. 2018. Vol. 32. Iss. 24-32. DOI: 10.1021/acs.energyfuels.7b01718
- Sobolev I.S., Orekhov K.A., Orekhov A.N. Background and anomaly components analysis of the radioactive spatial patterns in gamma-spectrometric exploration for mineral resources. Radioactivity and Radioactive Elements in Environment: Proceedings of VI International Conference, 20-24 September 2021, Tomsk, Russia. In 2 volumes. Tomsk: National Research Tomsk Polytechnic University, 2021. Vol. 1, p. 536-541 (in Russian).
- Aluker N.L., Suzdaltseva J.M., Herrmann M., Dulepova A.C.High-efficiency thermoluminescent detectors for measuring the absorbed ionizing radiation dose in the environment. Instruments and Experimental Techniques. Vol. 59. N 5, p. 733-739. DOI: 10.1134/S002044121605002X
- Aluker N.L., Artamonov A.S., Gimadova T.I., Zverev A.S. Thermoluminescent Dosimeters Based on Aluminum Oxide and Aluminum Nitride Ceramics. Instruments and Experimental Techniques. Vol. 64. N 6, p. 860-868. DOI: 10.1134/S0020441221050158
- Nelyubina N.V., Nikitin A.P., Valnyukova A.S., Ismagilov Z.R. Study Of Fossil Coals In The Kuznetsk Basin By Epr Spectroscopy. Journal of Physics: Conference Series. 2021. Vol. 1749. N 012018. DOI: 10.1088/1742-6596/1749/1/012018
- Furega R.I., Khabibulina E.R., Ismagilov Z.R. et al. EPR study of hard coals reached in Kuzbass. Butlerov Communications. Vol. 51. N 9, p. 149-152 (in Russian).
- Soroka E.I., Galeev A.A., Petrova V.I. et al. Organic Matter in the Saf’yanovka Copper Massive Sulfide Deposit (Middle Urals). Proceedings of Kazan University: Natural Sciences Series. Vol. 161. N 2, p. 307-324 (in Russian). DOI: 10.26907/2542-064X.2019.2.307-324
- Krasnovyd S.V., Konchits A.A., Shanina B.D. et.al. Adsorption processes on a carbonaceous surface: Electron spin resonance study. Physica B: Condensed Matter. Vol. 651. N 414571. DOI: 10.1016/j.physb.2022.414571
- Konchits A.A., Shanina B.D., Valakh M.Ya. et al. Local structure, paramagnetic properties, and porosity of natural coals: Spectroscopic studies. Journal of Applied Physics. 2012. Vol. 112. Iss. 4. N 043504. DOI: 10.1063/1.4745015
- Burgess S.D., Bowring S.A. High-precision geochronology confirms voluminous magmatism before, during, and after Earth’s most severe extinction. Science Advances. 2015. Vol. 1. Iss. 7. N e1500470. DOI: 10.1126/sciadv.1500470
- Pavlov V.E., Fluteau F., Latyshev A.V. et al. Geomagnetic Secular Variations at the Permian-Triassic Boundary and Pulsed Magmatism During Eruption of the Siberian Traps. Geochemistry, Geophysics, Geosystems. 2019. Vol. 20. Iss. 2, p. 773-791. DOI: 1029/2018GC007950
- Daoliang Chu, Grasby S.E., Haijun Song et al. Ecological disturbance in tropical peatlands prior to marine Permian-Triassic mass extinction. Geology. Vol. 48. N 3, p. 288-292. DOI:10.1130/G46631.1
- Menghan Li, Grasby S.E., Shui-Jiong Wang et al. Nickel isotopes link Siberian Traps aerosol particles to the end-Permian mass extinction. Nature Communications. Vol. 12. N 2024. DOI: 10.1038/s41467-021-22066-7
- Payne J.L., Clapham M.E. End-Permian Mass Extinction in the Oceans: An Ancient Analog for the Twenty-First Century? Annual Review of Earth and Planetary Sciences. 2012. Vol. 40, p. 89-111. DOI: 10.1146/annurev-earth-042711-105329
- Elkins-Tanton L.T., Grasby S.E., Black B.A. et al. Field evidence for coal combustion links the 252 Ma Siberian Traps with global carbon disruption. Geology. 2020. 48. N 10, p. 986-991. DOI: 10.1130/G47365.1
- Valiulin S.V., Onischuk A.A., Zamashchikov V.V. et al. Influence of Organic Aerosol in Coal Mines on the Ignition Limit of Methane-Air Mixture. Russian Journal of Physical Chemistry B. Vol. 15. N 2, p. 291-298. DOI: 10.1134/S199079312102024X
- Ribeiro J., Ania C.O., Suárez-Ruiz I., Flores D. Chapter 7 – The Spontaneous Combustion of Coal-Mine Waste and Stream Effects in the El Bierzo Coalfield, Spain. Coal and Peat Fires: A Global Perspective. Elsevier, 2019. Vol. 5: Case Studies – Advances in Field and Laboratory Research, p. 97-124. DOI: 10.1016/B978-0-12-849885-9.00007-X