Geochemical studies of rocks of the Siberian igneous province and their role in the formation theory of unique platinum-copper-nickel deposits
- Ph.D., Dr.Sci. Leading Researcher V.I.Vernadsky Institute of Geochemistry and Analytical Chemistry of RAS ▪ Orcid
Abstract
The Norilsk deposits, unique both in their geological structure and reserves of nickel and platinum, have attracted the attention of researchers for several decades. Several hypotheses have been proposed to explain their formation. Two of them are the most widely accepted: the model of ore formation in an intermediate chamber from picritic melt enriched in sulphides and the formation of sulphides in situ through the assimilation of sulphate-bearing sediments by tholeiitic magma as it rises to the surface. The main questions regarding the genesis of these deposits are: the composition of the parental magmas that formed the ore-bearing massifs; the relationship between intrusions and effusive rocks; and the extent and role of assimilation of host rocks by magmas. These issues are discussed in the article using a large amount of analytical data obtained by the author during the study of the magmatic rocks and geological structures in the Norilsk area. Based on these data, it was concluded that none of the proposed models could fully explain all observed geological features of the deposits as well as the appearance of the unique sulphide ores. In order to solve the problem of the genesis of the Norilsk deposits, it is necessary to analyse the evolution of P3-T1 magmatism over time in the Siberian large igneous province, especially in its eastern part, and to determine its association with ore-forming processes; and its investigating it is a priority task for understanding sulphide ore formation. Solving this task should be based not only on geophysical data but also on studies of the geochemistry of igneous rocks within the province.
Introduction
D.I.Mendeleev was an outstanding encyclopaedist who, along with chemistry, also paid special attention to mineralogy and the phenomenon of isomorphism, which he discussed in his qualifying papers [1]. He was also interested in the behaviour of elements in coal and oil formation processes [2] related to geochemistry, one of the emerging trends in geology during the 1870s. His student at St. Petersburg University, V.I.Vernadsky, made a significant contribution to this field. Geochemistry plays a leading role in the Earth sciences and helps solve many geological problems in new ways. One such problem is the origin of platinum, copper, and nickel deposits [3-5] related to large igneous provinces (LIPs), the largest of them is the Siberian province, which has been studied by both foreign [6, 7], and Russian geologists [8-10]. The use of modern analytical methods in the study of rocks and ores helps to gain a better understanding of the formation process and potential for discovering new deposits.
The most important area of anomalous concentration of strategically important metals is the northern Eastern Siberia, i.e., the North Siberian nickel-bearing province [11], which comprises super-large PGE-Cu-Ni deposits at Norilsk [12-14], playing a leading role in the global nickel and platinum metal economy. The geological structure of these deposits is characterized by a unique combination of large sulphide orebodies (the first tens of meters) with thin (100 meters thick) mafic bodies belonging to the P3-T1 trap formation, attracting the ongoing interest of researchers from various countries. Other copper-nickel deposits worldwide are associated with large ancient plutons [15, 16]. The genesis of these unique sulphide bodies presents one of the most significant challenges in geology, linked to the formation of giant geochemical anomalies in the Earth's crust. The International Geological Correlation Program N 354 (1995-1999), the symposia held in St. Petersburg (1996), Adelaide (2009), Beijing (2017) and other cities were devoted to this problem. Numerous hypotheses for the formation of the Norilsk deposits were proposed, including magmatic, metasomatic, and hydrothermal origin [3, 17, 18]. The magmatic theory, supported by experimental works [19, 20] and the discovery of solid solutions in ores [21], is the most widely accepted. However, within this theory, there are two fundamentally different models within this theory: one assumes the formation of sulphides in a closed magmatic system from picritic magma enriched in metals and volatile components, which appeared in an intermediate chamber [3, 13, 22]; the other accounts for ore formation through the assimilation of anhydrite-bearing rocks by magma in an open magmatic system, where the intrusions are the horizontal parts of channels through which magma erupts onto the surface [23]. Thus, the most common models differ in sulphides origin: they can be brought into the crystallization chamber by silicate magma or formed in situ. The latter model has also become widespread due to the presumed accumulation of a large amount of sulphides [24-26]. Each of these models has its own advantages and disadvantages, but there are some issues that are controversial and require further study, such as the composition of the parental magmas in ore-bearing intrusions; relationship between ore-bearing intrusions and basalts, and the extent of assimilation of host rock by magmas.
At the first stage of research, the models were tested. The analysis revealed that none of them completely matched to geological data we had collected. Therefore, we decided to develop a new approach to modeling for the formation of deposits in the Arctic region, taking into account the evolution of magmatism and the location of deposits within it [27, 28].
Basic information about geological structure of the area
The problem of formation of large igneous provinces is one of the main challenges in in geology, because their appearance plays a significant role in the history of the Earth's evolution [29]. These provinces also include continental trap provinces, with the largest Siberian province [30]. Recently, all igneous rocks with similar ages (251 million years) [29] and geographical locations (Western Siberia, the Kuznetsk Basin, the Altai Mountains, and the Urals) have been assigned to this province without considering their geological characteristics [31, 32]. Whereas, the term “traps” refers only to basalts covering vast areas of ancient platforms (“flood basalts”), which only a few provinces are associated with: Deccan, Paraná-Etendeka, Emeishan, and Colombia. According to the accepted terminology [33], this term considers the geological structure of the flows (covers), their tectonic position and chemical composition. Typical traps have a consistent thickness and rock composition (mainly tholeiitic) over wide areas.
These volcanic formations are common in Eastern Siberia and the southern Taimyr that are parts of the ancient Siberian Platform, which has an Archean basement and PR-T platform cover. Typical traps include tholeiitic basalts from the Tuklonsky – Samoedsky formations in the Norilsk region, as well as their analogues in Putorana, such as the Ayansky and Khonnamakitsky formations, and in the Tunguska Syncline, such as the Kochechumsky, Nidymsky, Yambukansky formations, and the Betlingsky Formation in Taimyr. These formations have very similar geochemical characteristics: MgO 6-7 wt.%; TiO2 0.9-1.7 wt.% [30]; Gd/Yb = 1.48-1.58 and La/Sm = 2.14-2.81; 87Sr/86Sr = = 0.70466-70653; eNd from +0.14 to +1.90; 206Pb/204Pb = 18.231-18.844 [5-7]. They correspond to gabbro-dolerite sills with a normal alkalinity, which are mainly combined in the Katangsky and Norilsk ore-bearing complexes, among others [8, 9].
However, in the same area, on shoulders of the Yenisei-Khatanga Trough and feathering structures (such as in Western Siberia), the volcanic rocks are commonly confined only to linear structures (known as paleorift zones). These zones are characterized by a local distribution of basalts, significant variations in thickness and composition. The composition ranges from alkaline to ultramafic rocks. Some examples of these formations are: Syradasaisky in Taimyr, Ivakinsky – Nadezhdinsky in the Norilsk region, and Arydzhangsky – Maimechinsky in Maimecha-Kotui region. The MgO content in these rocks varies from 3-5 to 22-24 wt.%. The TiO2 content is also variable, ranging from 0.9 % to 5 wt.%. Other important ratios include La/Sm, which ranges from 2.4 to 5.72, and Gd/Yb, which varies between 1.6 and 7.9. 87Sr/86Sr = 0.70302-0.71114; eNd from –11.0 to + 6.6; 206Pb/204Pb = = 17.796-19.111 [13, 30]. Intrusive rocks of similar composition present in these zones and are mainly exposed in Taimyr (Dyumtaleysky, Bootankagsky massifs, etc.) [8, 9]. These fundamental differences between the two types of magmatism called rift and platform (also known as typical trap) [34] are crucial both for understanding the mechanisms of the LIP formation and the conditions under which magmatic deposits were formed.
It is worth highlighting the Siberian large igneous province comprising both types of igneous formations. This province can be divided into the West Siberian Igneous Province and the East Siberian Igneous province. The latter includes as its main component the Siberian Trap Province formed by platform basalts.
The East Siberian province (Fig.1) consists of volcanic, plutonic, and pyroclastic rocks that are predominantly of Early Triassic age [29]. On the surface, the province has a zonal structure, with lavas dominating in the central part, followed by tuffs, and intrusive rocks are exposed along the periphery. Within the province, there are several areas with different structures and composition of igneous rocks. The thickness of volcanic rocks is greatest in the northern regions (up to 3.5 km), in the Norilsk and Maimecha-Kotui areas, where rocks with high alkalinity and magnesium content are found. Most of the rocks in the province are represented by tholeiitic basalts of normal alkalinity ranging from 1 to 1.5 kilometers in thickness, which developed in the Tunguska Syneclise and have been partially eroded since then. The subdivision of volcanogenic strata into formations was carried out during geological surveys in the 1960s and 1970s, and continues during 1:1 million surveying (the third generation). A special stratigraphic chart has been created for different areas.
Intrusive rocks are divided into several complexes, the most common of which is the Katanga complex represented by gabbro-dolerites of normal alkalinity (6-7 wt.% MgO). Sills of this complex crop out at the rim of the Tunguska Syneclise. In the northern part of the province, there are also subalkaline and alkaline massifs, as well as ultramafic rocks, where copper-nickel mineralization has been recorded [13].
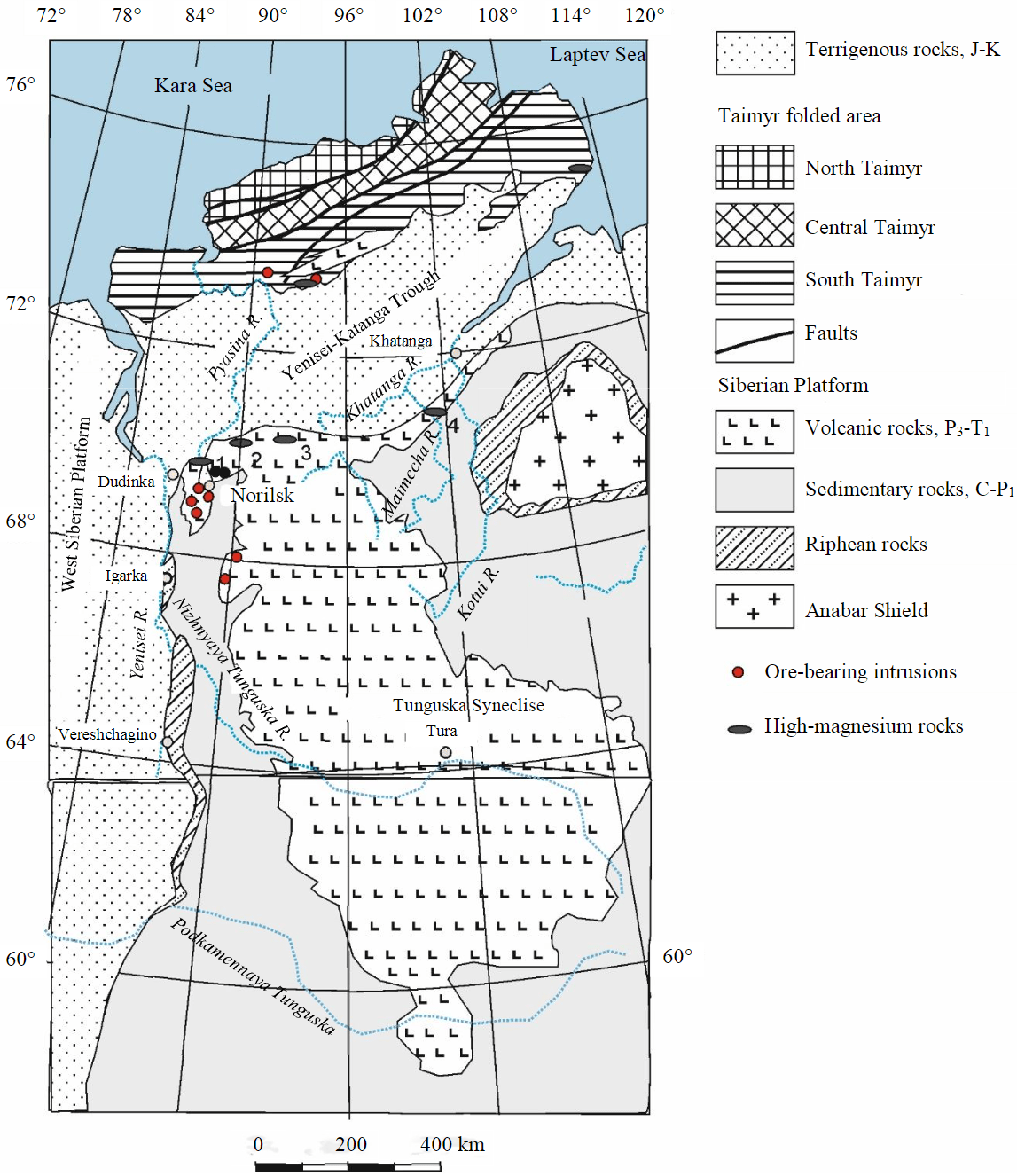
Fig.1. Scheme of tectonic structure of Eastern Siberia with location of intrusives with PGE-Cu-Ni mineralization
Sulphide PGE-Cu-Ni deposits are associated with high-magnesium intrusions in the Norilsk region [13]. The largest ones are concentrated within the Talnakh and Norilsk ore clusters, in periclinal parts of the Kharaelakh and Norilsk troughs (Fig.2). The ore-bearing massifs are ribbon-shaped bodies approximately 100 meters thick, 1-2 kilometers wide and 15-18 kilometers long. They typically occur subconformably with the Devonian-Permian terrigenous-carbonate deposits (Kharaelakh, Talnakh, Norilsk 1, Pyasino-Vologchansky), or less commonly break through tuffaceous-lava rocks as dyke- or pipe-shaped bodies (Norilsk 2, Mount Bol’shaya Bar’iernaya, Northern Maslovsky and Southern Maslovsky). Intrusions are composed of gabbro-dolerites (from bottom to top): contact, taxitic, picritic, olivine, olivine-containing, olivine-free [14]. The upper zone is composed of gabbro-diorites, leucogabbro, ferrogabbro (gabbro-dolerites enriched in titanomagnetite), igneous breccias; and some horizons may be absent. Ores are represented by the dissemination of sulphides in gabbro-dolerites of the lower horizons of intrusive bodies (picritic and taxitic gabbro-dolerites), as well as in their host rocks, and by continuous sulphides veins in lower contact with the underlying rocks. Low-sulphide platinum mineralization was discovered in the upper parts of the ore-bearing intrusions [35].
Objects and methods of studies
The main objects of our research were the intrusions that comprise the main deposits of the Norilsk region, i.e., Oktyabr’sky, Talnakh, Norilsk 1, Maslovsky, Chernogorsky and Vologochansky (Fig.2). These intrusions were studied during contractual work between the GEOKHI RAS and OOO Norilskgeologiya based on boreholes drilled in different years, such as OUG-2, TG-21, ZF-12, ZF-10, RG-2, MN-2, G-22, OV-29, OV-32, Ch-55, OM-4, OM-24, and LP-1, etc. Sills occurring along the rim of the Tunguska Syneclise and cropping out on the surface were also studied in bedrock exposures. This also applies to effusive rocks that occur both in the main tectonic structures of the Norilsk region, and in key areas of the East Siberian Province. Basalts were rarely studied in boreholes (KhS-51, KhS-59, OM-6, OM-25, LP-5). Abundant analytical data (major, trace elements and distribution of radiogenic isotopes; composition of ore and rock-forming minerals) were summarized in papers [36, 37].
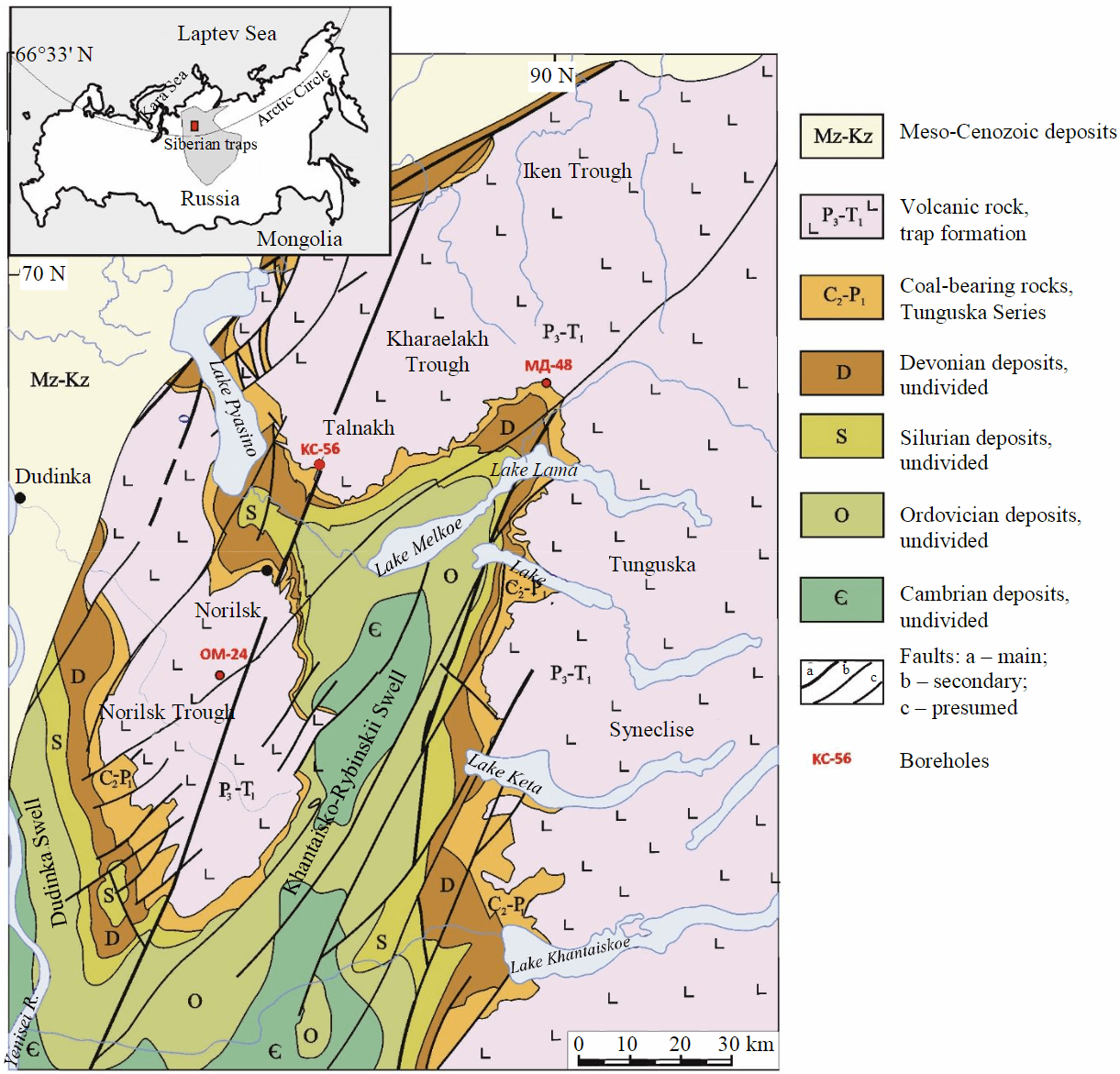
Fig.2. Geological base map of Norilsk region
Concentrations of major oxides in the rocks were determined using the X-ray fluorescence method (XRF; AxiosmAX model from PANalytical – Almelo, The Netherlands) at the Institute of Ore Geology, Petrography, Mineralogy and Geochemistry of Russian Academy of Sciences by A.I.Yakushev. Trace elements contents were analyzed by inductively coupled plasma mass spectrometry of (ICP-MS) at the Institute of Applied and Theoretical Mechanics (Chernogolovka city) by V.K.Karandashev. Additionally, the contents of both elements were determined by mass spectrometry with inductively coupled plasma with laser ablation of material (LA-ICP-MS) at the Max Planck Institute for Chemistry in Mainz, Germany, by N.A.Krivolutskaya and D.V.Kuzmin under the supervision of B.Stoll. A large amount of analytical data on the composition of rock-forming minerals was also obtained there using a GEOL JXA 8200 microprobe in laboratory of A.V.Sobolev. Sr, Nd and Pb isotopes were analysed in rocks at VSEGEI in St. Petersburg by B.V.Belyatsky. Isotopic analysis of sulphur in sulphides was done at the DVGI DVO RAS in Vladivostok by analyst T.A.Velivetskaya using the standard procedure. Experimental study of melt inclusions was conducted by N.A.Krivolutskaya at GEOKHI RAS. These research methods were earlier described in the monograph [36]. Computer modelling of the composition of the Talkhan magma was performed using the COMAGMAT software in collaboration with A.A.Ariskin [37].
Research results
Assessment of compositions of parental magmas of ore-bearing intrusions
For magmatic deposits in which sulphides are the components of intrusive rocks, the composition of magmas plays a significant role in the formation of ore, specifically the content of major oxides, trace elements, and especially, fluid components. Three methods were used to assess these:
- Calculation of weighted average composition of rocks in the ore-bearing intrusions. Initially, the composition of primary magmas was parallelized with composition of the most high-magnesium rocks, such as picritic gabbro-dolerites and troctolites. This led to the idea that ore-bearing intrusions formed from ultramafic magma similar to the Gudchikhinsky Formation [13, 38], and even to the conclusion that such magma was injected independently of gabbro magma [39]. To evaluate the parental melts, the compositions of contact zones of the intrusions were used. However, these zones can reflect the processes of assimilation of host rock by magma, and they are also subject to hydrothermal alteration. Additionally, during the emplacement of heterogeneous magmas phenocrysts can be “driven away” from the contact zone, so, the composition of the chilled zones most often does not correspond to the true composition of the parental magma.
The most widely used method for estimating the composition of magmas is based on the bulk composition of rocks, when they are slightly altered. M.N.Godlevsky [3] noted the specific features of ore-bearing intrusions in the Norilsk region obtained from calculating of their weighted average composition. These features include an namely their elevated magnesium content (MgO 10-12 wt.%), a chromium content of 0.4 wt.%, and a reduced titanium content (< 1 wt.% TiO2 ), indicating a deeper source of ore-beating magmas, approaching to picrite, compared to typical traps. A.P.Likhachev [14] proposed a formation mechanism for such melts as a result of sequential batch melting of the mantle. Calculations of weighted average compositions of ore-bearing intrusions were also used to estimate the composition of magmas. In particular, the weighted average composition of the Kharaelakh intrusion was determined for borehole KS-56 (Fig.2, Tables 1, 2) in the northeastern Oktyabr’sky deposit.
Table 1
Rock composition in borehole KS-56, wt.%
Depth, m |
Components |
|||||||||
SiO2 |
TiO2 |
Al2O3 |
Fe2O3 |
MnO |
MgO |
CaO |
Na2O |
K2O |
P2O5 |
|
1,380.4 |
51.25 |
1.12 |
26.77 |
12.75 |
0.04 |
2.74 |
0.53 |
0.40 |
4.04 |
0.19 |
1,382 |
57.06 |
1.13 |
24.75 |
9.27 |
0.04 |
2.73 |
0.34 |
0.85 |
4.05 |
0.07 |
1,382.9 |
56.46 |
1.15 |
23.43 |
9.98 |
0.09 |
2.87 |
1.05 |
2.30 |
2.87 |
0.22 |
1,385 |
48.38 |
1.08 |
17.74 |
10.96 |
0.20 |
7.25 |
10.60 |
2.77 |
0.83 |
0.14 |
1,387.6 |
49.57 |
1.10 |
16.92 |
10.57 |
0.17 |
7.17 |
11.75 |
2.44 |
0.61 |
0.14 |
1,441.9 |
42.44 |
0.69 |
11.43 |
17.69 |
0.18 |
14.50 |
8.02 |
1.21 |
0.28 |
0.10 |
1,516.2 |
49.04 |
0.91 |
17.61 |
9.86 |
0.18 |
8.20 |
11.23 |
2.29 |
0.53 |
0.14 |
1,530.7 |
49.07 |
0.91 |
16.74 |
9.90 |
0.20 |
8.20 |
11.92 |
2.33 |
0.54 |
0.07 |
1,536.6 |
42.83 |
0.50 |
6.94 |
14.16 |
0.20 |
29.51 |
4.92 |
0.61 |
0.16 |
0.07 |
1,537.4 |
40.47 |
0.61 |
8.02 |
19.59 |
0.23 |
22.45 |
5.13 |
0.70 |
0.17 |
0.06 |
1,540.4 |
42.73 |
0.72 |
11.03 |
16.01 |
0.21 |
17.54 |
7.30 |
1.16 |
0.36 |
0.12 |
1,541.6 |
42.31 |
0.72 |
11.82 |
16.26 |
0.11 |
14.32 |
8.19 |
1.39 |
0.29 |
0.08 |
1,551.3 |
37.22 |
0.46 |
16.16 |
20.46 |
0.14 |
7.41 |
16.18 |
0.24 |
0.03 |
0.06 |
1,552.6 |
47.49 |
0.49 |
21.88 |
9.12 |
0.08 |
7.13 |
11.40 |
1.97 |
0.47 |
0.07 |
1,554.85 |
44.93 |
0.48 |
17.26 |
16.06 |
0.15 |
7.46 |
6.84 |
1.63 |
0.95 |
0.07 |
1,556.4 |
53.65 |
0.63 |
23.32 |
7.08 |
0.13 |
4.25 |
5.85 |
3.48 |
1.98 |
0.13 |
1,558.7 |
51.40 |
1.36 |
26.94 |
11.08 |
0.06 |
3.92 |
1.37 |
0.78 |
3.00 |
0.57 |
1,559.2 |
58.68 |
1.02 |
23.74 |
8.91 |
0.08 |
2.74 |
0.78 |
0.67 |
3.33 |
0.07 |
1,561.4 |
50.95 |
1.70 |
28.17 |
6.88 |
0.05 |
3.90 |
1.81 |
3.10 |
3.34 |
0.27 |
The obtained results (Table 2) are in good agreement with representative weighted average compositions of the Norilsk intrusions calculated using a large number of analyses along different vertical sections, which makes it possible to take into account the complex morphology of the ore-bearing massifs, which differs significantly from the layered ones.
Table 2
Weighted average compositions of the intrusions of the Norilsk complex, wt.%
Components |
Norilsk 1 |
Talnakh |
NE Kharaelakh |
SW Kharaelakh |
Chernogorsky |
|
1 |
2 |
3 |
4 |
5 |
||
SiO2 |
48.3 |
46.1 |
46.15 |
50.26 |
47.2 |
|
TiO2 |
0.85 |
0.74 |
0.83 |
0.91 |
0.61 |
|
Al2О3 |
15.33 |
15.5 |
15.08 |
17.32 |
16.7 |
|
FeO |
12.3 |
13.4 |
13.56 |
10.89 |
9.91 |
|
MnO |
0.19 |
0.15 |
0.17 |
0.18 |
0.16 |
|
MgO |
10 |
11.3 |
10.81 |
7.93 |
11.64 |
|
CaO |
10.4 |
10.3 |
9.52 |
9.49 |
11.4 |
|
Na2O |
1.86 |
1.32 |
1.76 |
2.01 |
1.85 |
|
K2O |
0.57 |
0.69 |
0.56 |
0.84 |
0.46 |
|
P2O5 |
0.2 |
0.25 |
0.12 |
0.18 |
0.07 |
|
Cr |
1,000 |
1,900 |
– |
1,058 |
1,800 |
Notes. Data on borehole numbers: 1 – 54 boreholes [3]; 2 – 29 boreholes [40]; 3 – KS-56 (Table 1); 4 – TG-21; 5 – Ch-55 [36]. A dash – the element was not determined.
- Calculation methods. The geochemical thermometry method [41] allowed, using the COMAGMAT computer program, to estimate the composition of the melt, its crystallization temperature and the number of intratelluric phenocrysts for the Talnakh intrusion, when when it was introduced into a modern chamber [37]. It was calculated that the Talnakh magma contained 7 vol.% olivine and 4 vol.% plagioclase, which means that the proportion of crystals in the magma was 11 vol.%. The composition of the melt containing these minerals was close to that of basalts from the Morongovskaya – Mokulaevskaya formations, which are typical platform basalts. The composition of this melt was as follows: wt.%: 49.44 SiO2, 1.20 TiO2, 15.44 Al2O33, 11.43 FeO, 0.22 MnO, 8.06 MgO, 11.53 CaO, 1.82 Na2O, 0.69 K2O, and 0.19 P2O5.
- Composition of melt inclusions in rock-forming minerals is an important aspect of studying the composition of parental magmas. The first and the second methods help to assess the compositions of solid and liquid phases of parental magmas, but do not allow determining the content of volatile components in them, which many researchers believe play a crucial role in ore formation. These components, such as water and chlorine, can transfer sulphides through magma to the upper zones of massifs and even to the surface [42, 43]. The idea of elevated content levels of volatile components in magmas, especially water and chlorine, is based on the discovery of thick metasomatic aureoles around the ore-bearing intrusions within the Talnakh ore cluster [44]. However, recent research indicates that these aureoles have formed due to the circulation of meteoric water around the contacts of igneous rocks and host rocks, which are characterized by increased fracturing and permeability [45].
To study the contents of volatile components in magmas, we analysed melt and fluid inclusions in olivines and pyroxenes from several ore-bearing massifs, such as Talnakh, Norilsk 1, Northern Maslovsky and Southern Maslovsky (Fig. 3) as well as from barren intrusions, like the Low Talnakh and Zelenogrivsky [28, 31]. The composition of the melt was found to be similar to tholeiitic basalts with a slightly higher magnesium content (MgO 8-10 wt.%) (Table 3). The concentrations of volatile components are equally low in both ore-bearing and barren intrusions, with H2O ranging from 0.4 to 1.1 wt.% ; Cl from 0.02 to 0.2 wt.%, and F averaging 315 ppm, which corresponds to their formation in platform conditions near the surface [46].
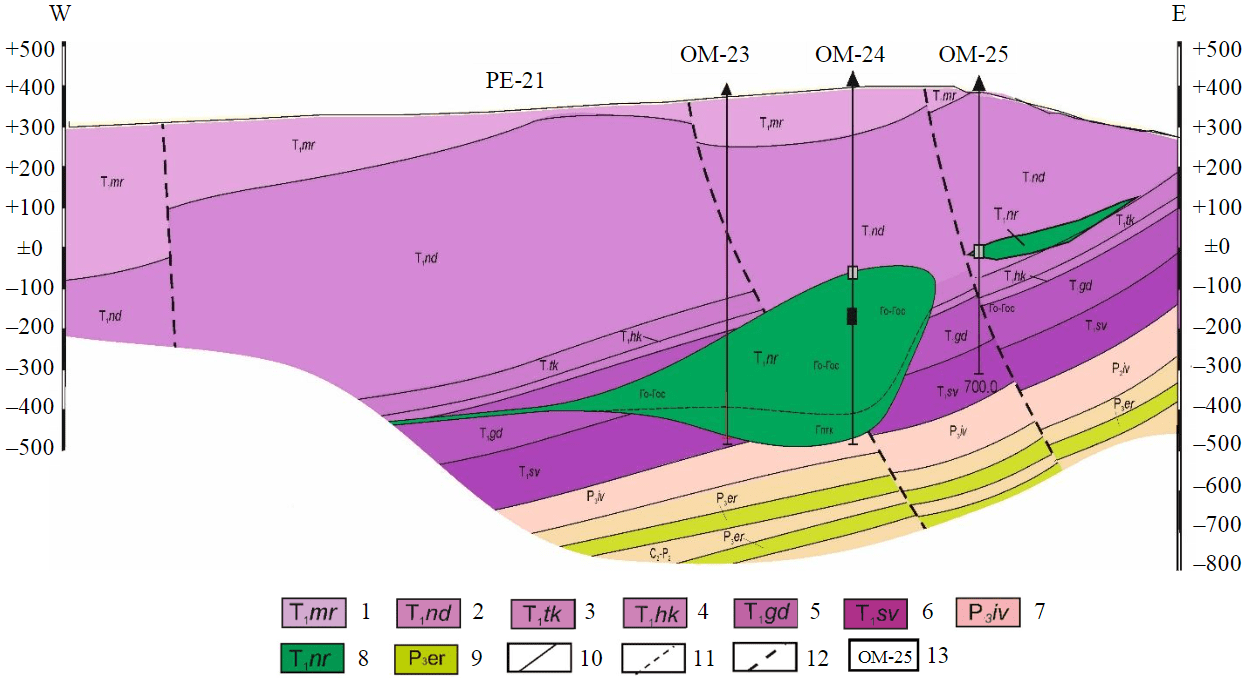
Fig.3. Geological section of the Southern Maslovsky intrusion (according to data of OOO Norilskgeologiya with modifications by the author). Location of the sample from borehole OM-24/589 is shown by a black rectangle 1-7 – volcanic rocks, formations (1 – Morongovsky; 2 – Nadezhdinsky; 3 – Tuklonsky; 4 – Khakanchansky; 5 – Gudchikhinsky; 6 – Syverminsky; 7 – Ivakinsky); 8, 9 – intrusive complexes (8 – Norilsk, 9 – Ergalakh); 10 – geological boundaries; 11 – boundaries between rock varieties; 12 – faults; 13 – boreholes and their numbers
Table 3
Composition of phases in crystallized inclusion and experimentally quenched melt, wt.%
Point number, phase |
SiО2 |
TiО2 |
Al2О3 |
FeО |
MnО |
MgO |
CaО |
Na2O |
K2О |
P2О5 |
V2О5 |
Cr2О3 |
NiО |
ZnО |
S |
Cl |
H2O |
Total |
|
1. Cpx2 |
51.69 |
0.9 |
3.17 |
12.68 |
0.33 |
13.45 |
19.39 |
0.3 |
– |
– |
0.08 |
– |
– |
– |
– |
– |
– |
101.99 |
|
2. Cpx1 |
52.53 |
0.52 |
2.24 |
9.46 |
0.23 |
15.75 |
20.47 |
0.26 |
– |
– |
0.14 |
– |
– |
– |
– |
– |
– |
101.6 |
|
3. USp |
0.33 |
20.8 |
10.89 |
67.49 |
0.5 |
0.39 |
0.15 |
– |
– |
– |
0.29 |
0.08 |
0.09 |
0.1 |
– |
– |
– |
101.11 |
|
6. Gl |
66.5 |
0.14 |
20.69 |
1.73 |
0.13 |
0 |
3.45 |
6.66 |
1.98 |
0.49 |
0 |
– |
– |
– |
– |
– |
– |
101.78 |
|
Melt |
49.2 |
1.73 |
10.82 |
14.9 |
0.26 |
7.15 |
11.51 |
2.68 |
0.54 |
0.17 |
– |
– |
– |
– |
0.11 |
0.15 |
0.44 |
99.87 |
Notes. Numbers of points and the area (shown by a square) on which glass composition was determined are shown in Fig.4, b. Melt – composition of a homogenized inclusion at T = 1,190 °C in clinopyroxene (Mg#75) from sample OM-24/676.8. A dash – the element was not determined.
Completely homogenized inclusions are represented by quenched melts. The concentrations of trace elements in them were determined using secondary ion mass spectrometry (SIMS) at the Valiev IPT RAS, Yaroslavl Branch by analysts S.G.Simakin and E.V.Potapov. The results are presented in Table 1, where the concentrations of trace elements are as follows, ppm: 33 Rb, 187 Sr, 135 Zr, 188 Ba, 25 Ce, 5.30 Sm, 5.91 Gd, 5.41 Er, 9.0 Yb, 0.37 Ta, 1.04 Th, 0.40 U, 3.96 Pb, 3.55 Hf, 0.66 Lu, 7.00 Dy, 3.3 Eu, 18 Nd, 3.5 Pr, 11 La, 0.55 Cs, 6.0 Nb, 42 Y, 4.4 Yb, 4.4 Er. The distribution spectrum of these trace elements in the inclusion normalized to the primitive mantle is shown in Fig.4, c (inclusion). This figure also demonstrates the distribution of the trace elements in a rock (sample OM-24/676.7) and in the basalts of the Mokulaevsky Formation with which the ore-bearing intrusions are compared [23]. The last two spectra are practically the same, while the inclusion spectrum is characterized by higher contents of all elements, which is due to their accumulation in the fractionated melt from which the host mineral, pyroxene, crystallized. This also accounts for a minor negative anomaly of strontium reflecting a slightly earlier crystallization of plagioclase.
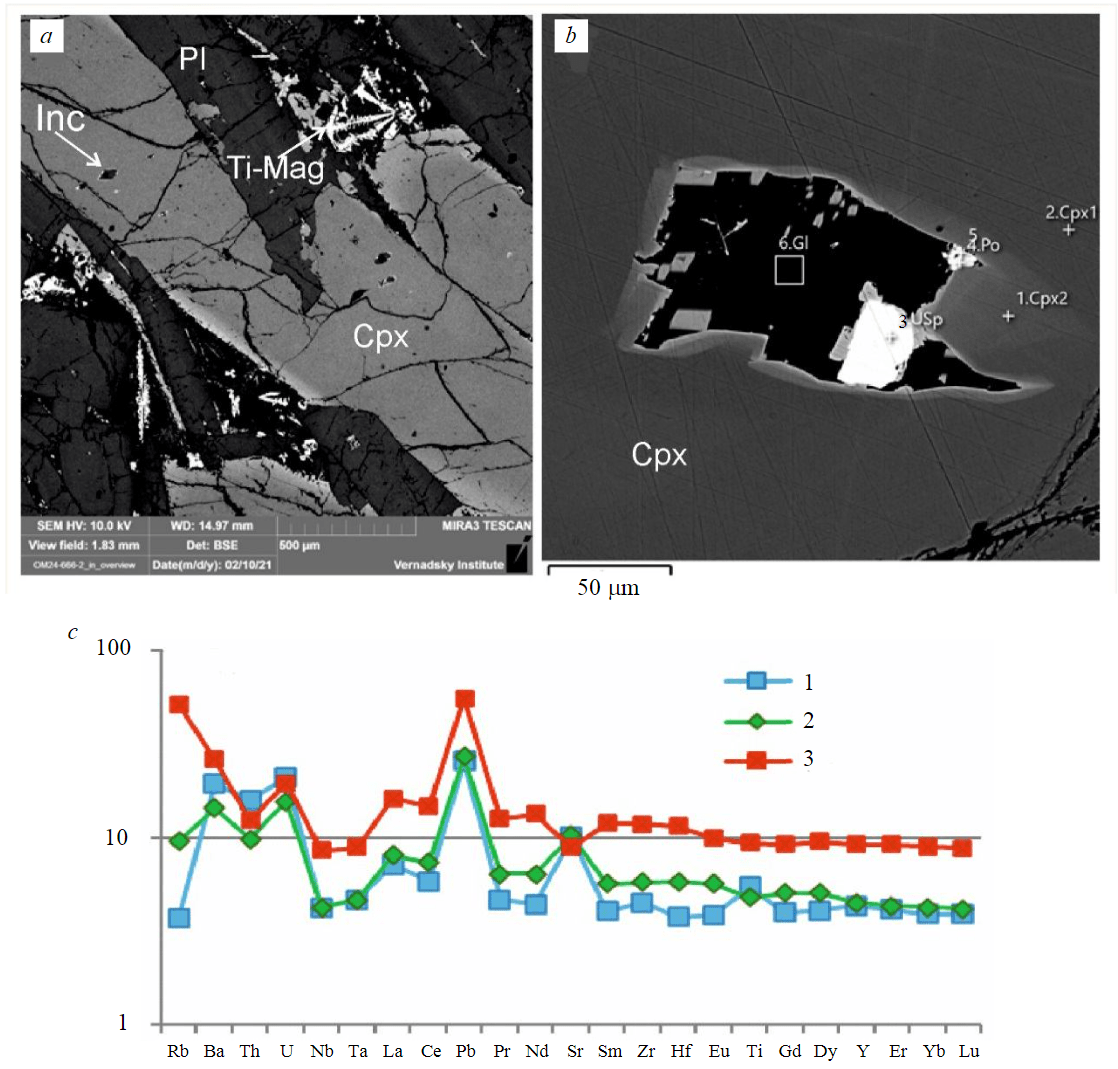
Fig.4. Morphology and composition of melt inclusions in сlinopyroxene of the Southern Maslovsky deposit (borehole OM-24, depth 589 m, see Fig.3): a – general view; b – inclusion with points of measurements (see Table 3); c – distribution spectra of trace elements: basalts of the Mokulaevskaya Formation (1), host rocks (2) and inclusions (3) (normalized to primitive mantle [47]); Cpx – clinopyroxene; Pl – plagioclase; Ti-Mag – titanomagnetite; Inc – inclusion; Gl – glass; USp – ulvospinel; Po – pyrrhotite
Relationship between ore-bearing intrusions and basalts
The question of relationship between ore-bearing massifs and lavas naturally arises when analyzing the geological structure of a region and has been actively discussed since the late 1950s [3, 13, 23]. The difficulty lies in the fact that ore-bearing intrusions stratigraphically occur beneath the tuff-lava sequence and rarely come into contact with basalts. An exception to this is the case in the Norilsk Trough, where several massifs cut through effusive rocks of the Ivakinsky – Gudchikhinsky formations (Norilsk 1, Norilsk 2) and the Ivakinsky – Nadezhdinsky formations (Southern Maslovsky intrusion) [36]. However, the Morongovsky and Mokulaevsky basalts [23], which occur much at a higher elevation than these formations, are believed to be comagmatiс with them, though there are no known contacts between them and ore-bearing massifs as well. The similarity in trace element and isotope compositions [6, 43] of these two groups of rocks (Fig.5) suggests that they may be related in some way, despite their apparent lack of contact. In recent years, a significant amount of geochemical data on ore-bearing intrusions has been collected [7-9]. This data indicates a similarity between many of the parameters of these intrusions and typical traps, including data on Sr and Nd isotopes. However, despite the similarity of isotopic characteristics, such as eNd (Fig.5, b), Yu.A.Kostitsyn and co-authors [10] found that ore-bearing intrusions have a very narrow range of variation in this parameter, compared to volcanic rocks. Even a complete identity in geochemical indicators, both isotopic and trace element, cannot serve as proof of a connection between intrusions and basalts, since they may crystallize from different portions of the same magma, but arriving either to the surface, or crystallizing at depth, even at the same time. This phenomenon is illustrated in Fig.6, a (left part). The connection between these formations (Fig.6, a, right part), which can occur either in the immediate vicinity of different intrusions or at a significant distance from them (as indicated by the vertical line separating these areas in the Fig.6), can only be confirmed through geological research.
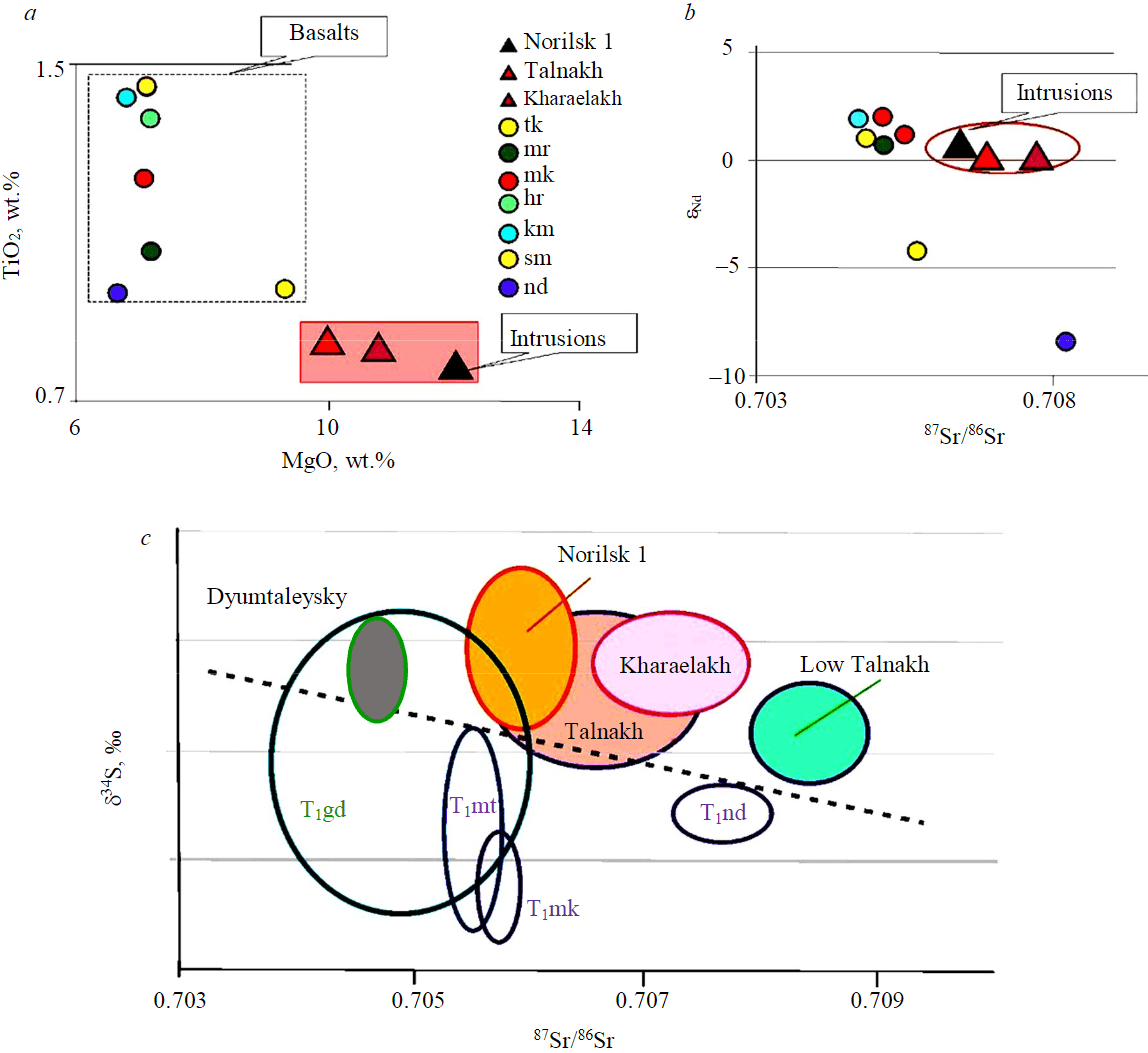
Fig.5. Diagrams of MgO –TiO2 (a); 87Sr/86Sr – eNd (b); 87Sr/86Sr – δ34S (c) for intrusive rocks and platform basalts [8, 36, 48-50]. Formations: tk – Tuklonskaya; mr – Morongovskaya; mk – Mokulaevskaya; hr – Kharaelakhskaya; km – Kumginskaya; sm – Samoedskaya; nd – Nadezhdinskaya; gd – Gudchikhinskaya
The lack of association between intrusions and lavas is primarily determined by their different contents of major oxides (see Fig.5, a), specifically, elevated concentrations of magnesium and chromium in the intrusive rocks, containing sulphide ore, and decreased concentrations of titanium, which is often ignored.
The association of the ore-bearing massifs with basalts of all formations is contradicted by the almost complete absence of sulphides (< 0.1 wt.%) in the latter. If the rocks are part of a single system, basalts should contain some sulfide minerals, as it is unlikely that sulfide melt would completely precipitate in only one feeder channel over a large area covered by basalt in Eastern Siberia. An important factor of the lack of direct association between basalts and intrusions is the difference in the isotopic composition of sulphur: all ore-bearing massifs have a heavier composition (Fig.5, c). The isotopic composition of the disseminated sulphide ores from the picritic gabbro-dolerites (sample numbers corresponds to borehole numbers and depth, m) in the Oktyabr’sky deposit is as follows: δ34S, ‰: +10.1 – RT-30/1,532.2; +9.4 – RT-30/1,540.5; +9.4 – RT-101/1,668.9; +10.1 – RT-101/1,709.2; +10.4 – RT-101/1,715.9; +10.1 – RT-7/1,473.8; +11.2 – RT-7/1,625.
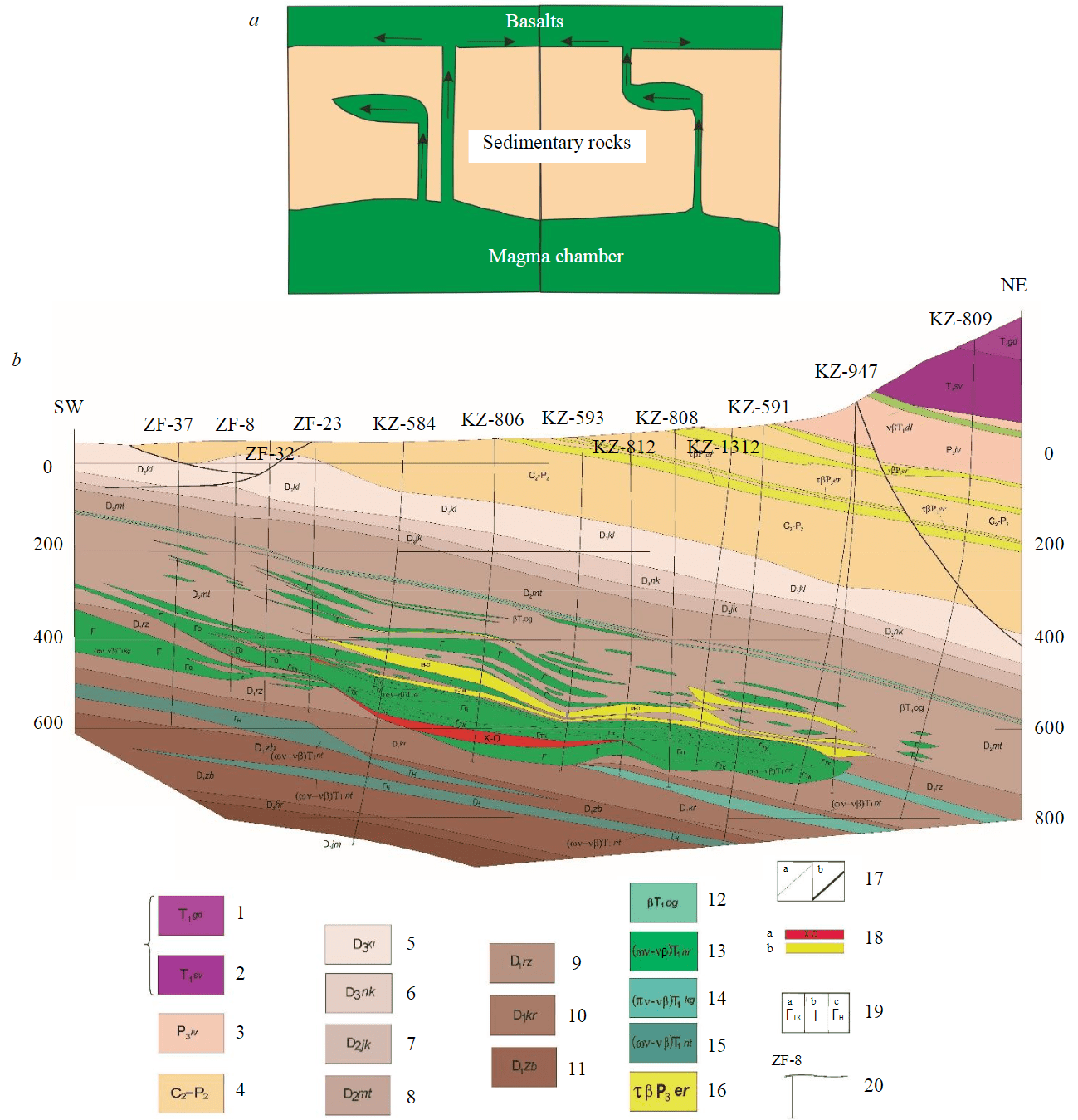
Fig.6. Scheme (a) of closed (left) and open (right) magmatic systems and structure (b) of the western part of Oktyabr’sky deposit (compiled by the author using the materials of OOO Norilskgeologiya). Arrows show the direction of magma movement 1-2 – Triassic system, formations (1 – Gudchikhinsky; 2 – Syverminsky); 3 – Permian system, undivided Ivakinsky Formation; 4 – Carboniferous system, undivided Tunguska Series; 5-11 – Devonian system, formations (5 – Kalargonsky; 6 – Nakokhozsky; 7 – Yuktinsky; 8 – Manturovsky; 9 – Razvedochninsky; 10 – Kureisky; 11 – Zubovsky); 12-16 – intrusive complexes (12 – Oganersky; 13 – Norilsk; 14 – Kruglogorsky; 15 – Low Talnakh; 16 – Ergalakh); 17 – geological boundaries (a) and faults (b); 18 – orebodies: a – massive ores (Х-O – main Kharaelak orerbody), b – cupriferous ores; 19 – intrusive rocks: a – picritic, troctolitic, taxitic and contact gabbro-dolerites; b – olivine-free, olivine-bearing, olivine gabbro-dolerites; c – olivine and picritic gabbro-dolerites of the Low Talnakh intrusion; 20 – boreholes and their numbers
Numerous attempts to use U-Pb data on zircons and baddeleyites [8, 9] to resolve the problem of the age relationships between different intrusions their selves and intrusions and lavas failed not only due to the insufficient accuracy of the method (which can have a small error of up to 10 million years and overlaps with the range of rock formation), but presence in rocks of a large number of these minerals that vary in composition. For example, in samples from the Kharaelakh massif, the age of zircons varies in the range from 347±16 to 235±6.1 Ma [39]. This variation is apparently not related to the accuracy of dating, but rather to the presence in rocks of really different-aged minerals that do not reflect the time of crystallization of the rocks.
A rapid development of geochemical methods, including local ones, over the past three decades has led to the situation where their results were are often not related to the geological basis. The only reliable criterion for the existence of an open magmatic system, in which the intrusions are part of the feeder channels for lavas to the surface, is geological data that confirm such a structure. Currently, there is no such evidence for the intrusions of the Norilsk complex. The independence of ore-bearing intrusions from basalts is most clearly demonstrated by the example of the Kharaelakh massif, which contains the largest deposit in the Norilsk region Oktyabr’sky deposit. An important fact about this is its complete preservation throughout the geological history, specifically, the absence of signs of erosion in any part of it. This allows us to reconstruct its relationships with host rocks as revealed during detailed prospecting and exploration (Fig.6, b). The structure of the western part of the deposit, which is crucial for understanding its origin, has been discussed in several papers [5, 51]. The section clearly shows that the intrusive rocks wedge out to the west and are not associated with basalts. If there were connecting channels between these formations, they would be exposed on the day surface above the intrusion, since the supposed effusives comagmatic rocks to the massifs are eroded in this area. The ribbon-like morphology of most of ore-bearing intrusions also, although indirectly, confirms the absence of a direct association with lavas, which can be seen in the southern Siberian Platform, where sills with a staircase structure are widespread.
Thus, the question of relationships between the intrusions and lavas (in any area) cannot be resolved only through geochemistry. While there is no doubt that the similarity of their geochemical characteristics indicates a genetic connection between rocks of different facies, it cannot be the evidence of a direct link between them, such as comagmatism, formation from the same portion of magma. Effusive and intrusive rocks that have the same composition (in the absence of additional processes, such as assimilation, mixing, etc.) can crystallize simultaneously or at different times. The difficulty in obtaining geological evidence of their connection and lack of direct observation due to poor rock exposure has led to the use of geochemical features as evidence for a single magma system.
Assimilation processes during intrusion of ore-bearing magmas
Occurrence of huge volumes of sulphide ores associated with thin bodies of silicate rocks led to the question about the source of sulphur in the deposits in the Norilsk region. M.N.Godlevsky [3] suggested that the required amount of sulphur in ores could be obtained by the assimilation of anhydrite from the host rocks. To test this hypothesis, he and L.I.Grinenko [49], began the studies of the isotopic composition of sulphur in sulphides. The first results showed its heavy composition (δ34S = +12 ‰ on average) compared to other copper-nickel deposits worldwide where δ34S was close to 0 ‰ and corresponded to its content in meteorites. These results have been reproduced for many years [9, 26, 52] and are considered as evidence for the assimilation of anhydrite-containing rocks by ore-bearing magmas. However, there are also arguments against this idea:
- Rapid cooling of the intrusions not only presumes the long-term assimilation of host rocks [15], but also prevents a large-scale interaction between magma and surrounding rocks. This is evidenced by the subvolcanic nature of the intrusive bodies, which are composed of gabbro-dolerites with characteristic ophitic structures, zoned rock-forming minerals and glassy inclusions characteristic of such bodies [22, 28].
- Geochemistry of contact zones. Geochemical features of the contact rocks also indicate rapid crystallization of magmas and cooling of massifs. We have previously considered this using the example of the Southern Maslovsky intrusion [36], which is located in effusive rocks. The lower contact lies in trachybasalts of the Ivakinsky Formation, while the upper contact occurs in the basalts of the Nadezhdinsky Formation (in its lower sub-formation). The host rocks differ from gabbro-dolerites in terms of concentrations and ratios of trace elements, particularly the increased La/Sm ratio, which indicates the assimilation of the host rocks by the magma. The value of (La/Sm)n either decreases in gabbro-dolerites at a distance of 1-2 m from the upper contact within the intrusion (borehole OM-25), or it does not change at all (borehole OM-24), indicating a complete absence of assimilation in the zone of contact zone. Similar studies were carried out for the Kharaelakh intrusion.
- Weighted average compositions of massifs occurring in rocks of different lithologies and genesis. Presumed large-scale assimilation of host rocks should be reflected in the weighted average composition of intrusions occurring in deposits with different compositions and the volume of ores contained therein. Often, as evidence of extensive assimilation processes, attention is drawn to the different deposits with varying volume of mineralization and associated with the main intrusive bodies, such as the Kharaelakh, Talnakh and Norilsk 1 massifs. They occur entirely within the Devonian rocks (partly containing anhydrite), the Devonian – terrigenous Tunguska Series (С2-Р2) and the Tunguska Series and the tuff-lava sequence, respectively. However, consideration of a larger number of arrays does not confirm this pattern. First and foremost, the author of this article studied the Pyasino-Vologochansky intrusion. Its geological location is similar to that of the Kharaelakh intrusion, but it does not contain large amounts of ores, and its metal reserves significantly lower than those in the Oktyabr’sky deposit. Although the Chernogorsky and Norilsk 1 massifs are located close to each other in terms of stratigraphy, their ore contents differ significantly.
- The problem of sulphur source. Anhydrite. Anhydrite, which is most often found in rocks surrounding ore-bearing massifs, is considered a potential source for obtaining sulphur during ore formation. However, its direct melting is impossible, as the melting point of the mineral is higher than the temperature of magma (1,450 °C compared to 1,200 °C of the melt). This can be seen from rare anhydrite xenoliths found in igneous rocks [14, 15]. It is often assumed that anhydrite decomposes (at temperature below 1,100 °C) releasing gases that could dissolve in the mafic magma. However, the volume of sulphur produced is not known. The solubility of sulphur oxides (SO3 and SO2) is low, with a maximum of 1 wt.%, and, thus, the amount of sulphur in a melt does not exceed 0.5 wt.%. The igneous origin of anhydrite found in the picritic gabbro-dolerites [53] has not been confirmed, as morphological characteristics alone are insufficient to determine magmatic genesis. In addition, the assessment of redox conditions during the crystallization of ore-bearing rocks made using the olivine-spinel equilibrium [36] indicates that reduction conditions exist in which anhydrite could not crystallize. Since hydrothermal and metasomatic anhydrite are often present in these rocks, it is necessary to establish clear criteria for classifying different types of anhydrite, with isotopic data playing the most important role in this process. However, the author has not conducted such studies, and therefore the magmatic origin of anhydrite described in the article remains uncertain.
An important characteristic of anhydrite as a source of sulphur in ores is the isotopic composition of the sulphur it contains. The isotopic composition of sulphur in sedimentary anhydrite which is considered as a contaminant differs significantly from that in sulphides ores from the Norilsk deposits. The δ34S value of anhydrite from the Devonian deposits ranges from +22 to + 24 ‰, while the most typical δ34S value for sulphides is +12, 8 ‰ [52]. Initially, in sulphide ores from the western part of the Oktyabr’sky deposit, the values δ34S in sulphides and anhydrites from enclosing rocks were similar (δ34S =12-13 ‰). This may be due the fact that the the analyzed anhydrites in this part of the deposit have a metasomatic origin, as metasomatic processes are common in this area [44]. The resulting variations in anhydrites are characteristic of rocks altered by the late hydrothermal processes. Away from intrusions, anhydrite from the Devonian rocks has a heavier sulphur composition, which that differs from the ores [52].
Difference in isotopic composition of host rocks and ores is often explained by the supply of sulphur into magma from two sources: the mantle and the crustal. Although there are no calculations of this mixing, it is assumed that they contribute equal portions in the formation of sulphides. However, in this case, the amount of mantle sulphur that should be present in the melt greatly exceeds its solubility in mafic magmas, so it cannot be obtained in situ.
Another possible mechanism for the enrichment of mafic magmas with sulphur is its supply from fluids circulating in the host rocks during the formation of the ore-bearing rocks [9]. Specific details of this process, such as the composition of the fluid (meteoric water?), the amount of sulphur dissolved in it, the modes of sulphur transfer, and isotope effects are not fully characterized in this work. It is important to note that sulphur in anhydrite exists in an oxide form (S6+), while in the melt it presences in a reduced form (S2–). Therefore, it is necessary to determine at what stage this transition occurs. Since the solubility of water in platform magmas under near-surface conditions does not exceed 1wt.%, and the amount of sulphur present in the magma is also limited (the author of research [9] does not give its possible content), even if some water does enter the melt, the amount of resulting sulphides will be negligible compared to the amount found in the deposits [23].
In addition, fluid must be supplied during the stage of magma formation when sulphur is converted into sulphides. The movement mechanism of how cold fluid moves into hot magma in a thermal gradient field is not considered in this work. It has been observed that when mafic magmas emplacement into sedimentary rocks, gases and fluid components from the host rocks “forced out” from the area where intrusion become localized [54]. The model under consideration does not address the composition of the rocks from which the anhydrite was removed and distribution of its aureole. Based on the estimated volume of removed sulphur, the rocks would be sterile with regard to anhydrite over a large area. However, anhydrite-bearing rocks are found in the immediate vicinity of the ore-bearing intrusions. In particular, it is well known that in the rocks of the Manturovskaya Formation on the western flank of the Kharaelakh massif, there are nodules of red sedimentary anhydrite with no signs of hydrothermal reworking. V.V.Reverdatto and colleagues [55] estimated the possible extent of thermal impact on the host rocks, which in near-surface conditions (2 km) does not exceed 500 m for gabbro. It is in this area closest to the contact where one should expect to find formations remaining after the dissolution and removal of anhydrite by fluids. However, the rocks occurring in this area do not show any signs of its dissolution or removal of sulphates. In addition to the Talnakh ore cluster, there are deposits associated with massifs that occur not in sedimentary rocks, but in basalts (Maslovsky). It is difficult to imagine the transfer of sulphur from the host rocks through fluids in these cases.
The problem of sulphur origin in the Norilsk ores turned out to to be much more complex than previously thought, due to the use of new geochemical research methods. In particular, the study of radiogenic isotopes has revealed some unexpected findings. Firstly, strontium isotopes not always correlate with sulphur isotopes in ore-bearing intrusions. For example, the ratios of strontium and sulphur in the Talnakh and Low Talnakh intrusions show opposite relationships compared to what would be expected based on the degree of assimilation. The Talnakh massif, which contains ores with high metal reserves (δ34S = +10.9), should have a more radiogenic strontium composition due to significant assimilation of the host rocks. However, the barren Low Talnakh massif (δ34S +6.6) [9, Table 22] has a different composition, with a higher ratio of 87Sr/86Sr (0.709-0.710) [9, Table 56] compared to the Talnakh (0.706). These findings suggest that further research is needed to better understand the complex interactions between sulphur and strontium during ore formation in the Norilsk region. Secondly, the author of the book [9] did not explain the appearance of heavy sulphur (δ34S = +10.9) [9, Table 22] in the rocks of mantle origin. For these rocks, 87Sr/86Sr ratios are typically between 0.704 and 0.705 [9, Table 43], and eNd values are +4.5 [9, Table 32]. Data on radiogenic isotopes in sedimentary anhydrite, particularly Pb [36], also indicate that they cannot be considered as a contaminant for magmas during ore formation.
Heterogeneity of isotopic composition of sulphides from different deposits in the Norilsk region is interpreted as evidence of the existence of an open magmatic system, in which host anhydrite rocks assimilated by magmas [26]. However, it is important to note that the duration of high-temperature exposure cannot explain the observed heterogeneity, as there is little or no fractionation under these conditions. Instead, the heterogeneity is likely due to the lack of equilibrium during the crystallization of sulphides. The fact that sulphides are heterogeneous in δ34S also cannot be evidence of a crustal source of sulphur rather than a mantle source, as explained in the article, since the nature of heavy sulphur in the mantle [56] had not yet found an adequate explanation. Radiogenic isotopes (Pb, Sr) in anhydrites indicate that their ratios are quite different from those in ore-bearing intrusions [36], which does not allow considering a specific mineral and the rocks containing it as a source of sulphur.
There are presumptions that the melt has been enriched with sulphides due to the interaction between magmas and host rocks in deeper conditions than those recorded in the Norilsk region [57]. However, within the Siberian Platform, all possible stratigraphic occurrence levels of mafic-ultramafic massifs are exposed from the Cambrian rocks to the tuff-lava sequence, especially in its southern part. Nevertheless, nowhere were sulphides formed at their contact with the host rocks. The water content in the host rocks is also important, as in dry conditions, high-temperature metamorphic rocks of the spurrite-merwinite facies are formed [58]. In case of water-bearing rocks, metasomatites of various composition are formed, such as within the Talnakh ore cluster. If any process, such as assimilation or fluid supply, had led to local enrichment of sulphur from anhydrite, it would have manifested in different regions of Eastern Siberia where evaporites and mafic intrusions are widespread, recording the formation of sulphide ores. However, the deposits are located only in certain tectonic zones.
Other sources of sulphur
The assumption about the supply of sulphur not from anhydrite (or not only from anhydrite), but from hydrogen sulphide deposits or bitumen in the Paleozoic strata of Eastern Siberia was made by L.I.Grinenko [49]. Even without considering the exact mechanism of how it might have been supplied into the magma, it is clear that this source cannot be the main factor in ore formation. This is because there are no known Paleozoic hydrocarbon deposits in the Norilsk area, and more importantly, all the oils and bitumen from Eastern Siberia are characterized by low levels of sulphur (< 1 wt.%). Therefore, it is unlikely that they could have supplied the required amount of sulphur needed for ore formation, taking into account their volume.
- Mass balance calculations of calcium. Mass balance calculations of calcium are most important for understanding the role of assimilation in ore formations. A significant amount of sulphur is concentrated in the Norilsk deposits [23], which, if it originates from anhydrite, requires the release of an almost equal amount of calcium that must be present in minerals from either the surrounding rocks or the gabbro-dolerite. Since there is no recorded calcium enrichment in the host rocks, some researchers [26, 52] assume its concentration in leucogabbro within ore-bearing bodies. Despite this, the weighted average composition of the ore-bearing intrusions with varying ore reserves is not different (see Table 2), which would be expected if the ore-bearing massifs were enriched with this element. Since such calculations were made for a large number of boreholes in the Norilsk 1 and Talnakh massifs, the expected enrichment would have been identified and could serve as one of the prospecting criteria for ore-bearing intrusions. The composition of the ore-bearing massifs corresponds to the typical gabbro with concentrations of CaO varying between 9 and 10 wt.%. Thick leucogabbro horizons are also found in many non-ore-bearing plutons around the world.
Thus, the data presented indicate that the deposits formed in a closed system; the composition of parental melts did not differ significantly from the compositions of melts that formed barren massifs and volcanics during the platform stage of the Siberian province. However, there were slight increases in magnesium and chromium contents. The processes of host rock in situ assimilation by magmas were localized and did not significantly affect ore formation. Therefore, sulphides were supplied to the crystallization chamber by the parental magma. The composition of the ores can help to understand only the evolution of the sulphide melt within the chamber (or approaching it) but it does not explain its origin. There remains a question about the mechanism of sulphide formation in magmas. This can be only solved by understanding the evolution of the Permo-Triassic magmatism in Eastern Siberia and its role in ore formation. Therefore, it is essential to consider the structural features and composition of igneous rocks in the Norilsk ore region compared to other areas.
A new approach to the genesis of deposits
The study of P-T-igneous rocks in Eastern Siberia continued for almost a century [29, 58]. The difficulty in constructing a general scheme of their evolution both in space and time, which is basis for creating an adequate model of formation, is due to the immense size of the region, in which these formations occur as well as the inaccessibility of many areas that have been investigated by various organizations. This has led to the emergence of numerous individual schemes for subdividing both effusive and intrusive rocks, which are reflected in the legends of maps on a 1:200,000 scale and on 1:1,000,000. Their correlation using modern geochemical methods is one of the most important tasks of modern geology.
A specific position of the Norilsk region within the structure of the Siberian igneous province has been noted in publications from the 1950s [3, 12]. This area has been identified as an unusual petrographic province [3] characterized by a greater diversity of igneous rocks compared to other parts of the platform. Based on our representative set of analytical data (XRF + ICP + Sr, Nd and Pb isotopes) from the key areas in Eastern Siberia (see Fig.1), we have also demonstrated that this area represents a specific geochemical province [27].
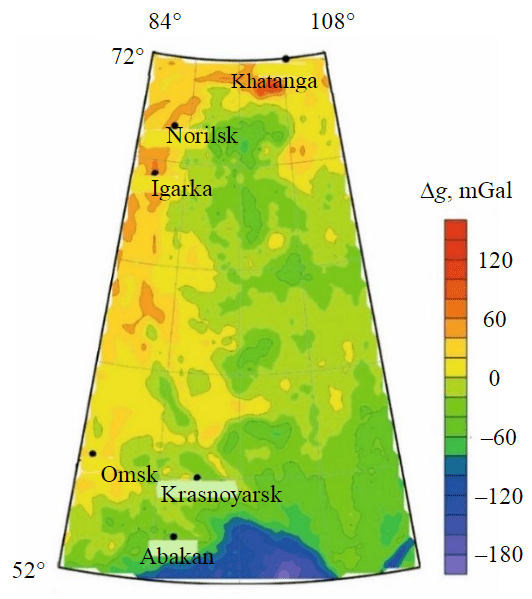
Fig.7. Map of gravity isoanomalies in Bouguer reduction for Eastern Siberia [59]
Analysis of the obtained analytical data made it possible to compare the igneous rocks of the key areas [27] and draw several conclusions. The most significant one is the determination of a diverse range of rocks in the Arctic zone of Eastern Siberia, including the Taimyr, Norilsk and Maimecha-Kotui regions, in comparison to the Tunguska Syneclise and its surrounding area. In the northern part of the region, rocks characterized by typical crustal features are common, they have negative Ta-Nb anomalies, positive Pb anomalies, high La/Sm ratios, low Gd/Yb ratios (87Sr/86Sr = 0.705-0.710), and eNd values ranging from –7 to +1. Simultaneously, here were found products of mantle magmas without Ta-Nb and Pb anomalies with a high Gd/Yb ratio (87Sr/86Sr = 0.703, eNd = 5) indicating the occurrence of garnet in the source and great depths of magma generation. This situation is explained by the complex tectonic structure of the northern part of the area, which is determined by the formation and development of the Yenisei-Khatanga Trough and smaller paleorift zones feathering it (including the Norilsk-Igarka rift). Along with typical traps (thick covers of tholeiitic basalts that overlay the ancient platform) rift rocks are also present and are confined only to narrow local grabens. These rocks have large variations in structure and composition. They are especially common in the north-west part of the area where major linear magnetic and gravity anomalies are located and the unique Norilsk deposits were found (Fig.7). Within these rift zones, both inside and at their edges, active long-term magmatic activity took place, manifesting itself in the intrusion of different types of magma at different depths within the lithosphere and Earth's crust. Therefore, it can be assumed that the specific geological conditions in this area played a role in the accumulation of sulphides, which led to the formation of the large deposits.
Conclusion
Formation history of ore deposits in Eastern Siberia can be divided into two stages: the formation of sulphide-bearing magmas and their intrusion into the upper crust and the crystallization of these magmas and evolution of sulphide matter in modern chambers. Most studies on the Norilsk ore deposits focus on the final stage of formation, which involves a detailed analysis of minerals using local methods [22, 43, 60]. These studies provide information about the processes of crystallization of silicate and sulphide melts, as well as their subsequent transformations in situ. This information can be used to improve mining techniques [61, 62]. Based on these works, conclusions are drawn about the whole ore formation process. However, less attention has been paid to the conditions of the origin and evolution of ore-bearing magmas. There is no doubt that the location of the unique deposits within the Norilsk-Igarka paleorift zone plays a crucial role in the genesis of the deposits. Magmas similar to those that formed the ore deposits in geochemistry have also intruded other areas of the region, but they do not contain large industrial accumulations of sulfides [30]. Further development of the theory of magmatic ore formation in northern Eastern Siberia should be based on an understanding of the evolution of tectonics and magmatic activity during the Late Permian and the Early Triassic periods on the Siberian Platform, in particular, it is important the evolution of the Yenisei-Khatanga Trough which plays a key role in the generation of magma and ores in the north of the province.
References
- Mendeleev D.I. Isomorphism in connection with other relationships of crystalline state to composition. Thesis presented at completion of the course at the Main Pedagogical Institute by student D.Mendeleev. St. Petersburg: Tipografiya I.I.Glazunova i kompanii, 1856, p. 234 (in Russian).
- Mendeleev D.I. Oil industry in the North American state of Pennsylvania and the Caucasus. St. Petersburg: Tipografiya tovarishchestva “Obshchestvennaya polza”, 1877, p. 304 (in Russian).
- Godlevsky M.N. Traps and ore-bearing intrusions of Norilsk region. Moscow: Gosgeoltekhizdat, 1959, p. 68 (in Russian).
- Nesterenko G.V., Almukhamedov A.I. Geochemistry of differentiated traps (Siberian Platform). Moscow: Nauka, 1973, p. 298 (in Russian).
- Proceedings of the Sudbury-Norilsk Symposium / Ed. by P.C.Lightfoot, A.J.Naldrett. Sudbury: Ontario Ministry of Northern Development and Mines, Ontario Geological Survey, 1994. Spec. vol. 5, p. 423.
- HawkesworthJ., Lightfoot P.C., Fedorenko V.A. et al. Magma differentiation and mineralisation in the Siberian continental flood basalts. Lithos. 1995. Vol. 34. Iss. 1-3, p. 61-81.
- Keays R.R., Lightfoot P.C. Crustal sulfur is required to form magmatic Ni-Cu sulfide deposits: evidence from chalcophile element signatures of Siberian and Deccan Trap basalts. Mineralium deposita. 2010. N 45. Iss. 3, p. 241-257. DOI: 10.1007/s00126-009-0271-1
- Malich K.N., Belousova E.A., Griffin W.L. et al. New insights on the origin of ultramafic-mafic intrusions and associated PGE-Cu-Ni sulphide deposits of the Norilsk and Taimyr provinces, Russia: evidence from radiogenic and stable isotopes. Processes and ore deposits of ultramafic-mafic magmas through space and time. Amsterdam: Elsevier, 2018, p. 197-238.
- Petrov O.V. Isotope Geology of the Norilsk Deposits. Berlin, Heidelberg: Springer, 2019, 306.
- Kostitsyn Y., Krivolutskaya N., Somsikova A.V. et al. Geochemical Features of Potentially Ore-Bearing Mafic Intrusions at the Eastern Norilsk Region and Their Relationships with Lavas (NW Siberian Traps Province). Minerals. Vol. 13. Iss. 2. N 213. DOI: 10.3390/min13020213
- Urvantsev N.N. North Siberian nickel-bearing province. Geologiya i geofizika. 1974. Vol. 15. N 3, p. 3-11 (in Russian).
- Kotulskii V.K. On the origin of magmatic copper-nickel deposits. Doklady AN SSSR. 1946. Vol. 51. N 5, p. 381-384 (in Russian).
- Geology and Metallogeny of Sulfide Deposits Norilsk Region U.S.S.R. / by O.A.Dyuzhikov, V.V.Distler. Littleton: Society of Economic Geologists, Inc., 1992. Special Publication, N 1, p. 242.
- Likhachev A.P. Platinum-nickel-copper and platinum deposits. Moscow: Eslan, 2006, p. 495 (in Russian).
- Naldrett J. Magmatic Sulfide Deposits. Geology, Geochemistry and Exploration. Berlin, Heidelberg: Springer, 2004, p. 728. DOI: 10.1007/978-3-662-08444-1
- Lightfoot P.C. Nickel Sulfide Ores and Impact Melts. Origin of the Sudbury Igneous Complex. Elsevier, 2016, p. 680.
- Zotov I.A. Transmagmatic fluids in magmatism and ore formation. Moscow: Nauka, 1989, p. 214 (in Russian).
- Zolotukhin V.V. Basic pegmatoids of the Noril’sk ore-bearing intrusives and the problem of genesis of the platinoid-copper-nickel mineralization of the Norilsk type. Novosibirsk, Siberian Branch RAS, SPC UIGGM, 1997. Iss. 834, p. 90 (in Russian).
- Kullerud G., Yund R.A., Moh G.H. Phase Relations in the Cu-Fe-S, Cu-Ni-S, and Fe-Ni-S systems. Magmatic Ore Deposits: A Symposium. Society of Economic Geologists. Vol. 4, p. 323-343. DOI: 10.5382/Mono.04.23
- Sinyakova E.F., Vasilyeva I.G., Oreshonkov A.S. et al. Formation of Noble Metal Phases (Pt, Pd, Rh, Ru, Ir, Au, Ag) in the Process of Fractional Crystallization of the CuFeS2 Minerals. 2022. Vol. 12. Iss. 9. N 1136. DOI: 10.3390/min12091136
- Brovchenko V.D., Sluzhenikin S.F., Kovalchuk E.V. et al. Platinum Group Element Enrichment of Natural Quenched Sulfide Solid Solutions, the Norilsk 1 Deposit, Russia. Economic Geology. 2020. Vol. N 6, p. 1343-1361. DOI: 10.5382/econgeo.4741
- Ryabov V.V., Shevko A.Ya., Gora M.P. Trap Magmatism and Ore Formation in the Siberian Norilsk Region. Dordrecht: Springer, 2014. Vol. 1. Trap Petrology, p. 390. DOI: 10.1007/978-94-007-5022-7
- Radko V.A. The facies of intrusive and effusive magmatism in the Norilsk region. Saint Petersburg: Cartographic factory of VSEGEI, 2016, p. 226 (in Russian).
- Naldrett A.J. Fundamentals of Magmatic Sulfide Deposits. Magmatic Ni-Cu and PGE Deposits: Geology, Geochemistry, and Genesis. Society of Economic Geologists. 2011. Vol. 17, p. 1-50. DOI: 10.5382/Rev.17
- Li C., Ripley E.M., Naldrett A.J. A new genetic model for the giant Ni-Cu-PGE sulfide deposits associated with the Siberian flood basalts. Economic Geology. 2009. Vol. 104. N 2, p. 291-301. DOI: 10.2113/gsecongeo.104.2.291
- Ketrov A.A., Yudovskaya M.A., Shelukhina Yu.S. et al. Sources and Evolution of Sulfur Isotopic Composition of Sulfides of the Kharaelakh and Pyasino-Vologochan Intrusions, Norilsk Ore Region. Geology of Ore Deposits. Vol. 64. N 6, p. 350-376. DOI: 10.1134/s1075701522050038
- Krivolutskaya N.A., Latyshev A.V., Dolgal A.S. et al. Unique PGE–Cu–Ni Noril’sk Deposits, Siberian Trap Province: Magmatic and Tectonic Factors in Their Origin. Minerals. Vol. 9. Iss. 1. N 66. DOI: 10.3390/min9010066
- Krivolutskaya N.A., Konyshev A.A., Kuzmin D.V. et al. Is the Permian–Triassic Mass Extinction Related to the Siberian Traps? Geochemistry International. 2022. Vol. 60. Iss. 13, p. 1321-1349. DOI: 10.1134/S0016702922130067
- Kamo S.L., Czamanske G.K., Amelin Y. et al. Rapid eruption of Siberian flood-volcanic rocks and evidence for coincidence with the Permian–Triassic boundary and mass extinction at 251 Ma. Earth and Planetary Science Letters. 2003. Vol. 214. Iss. 1-2, p. 75-91. DOI: 10.1016/S0012-821X(03)00347-9
- Zolotukhin V.V., Vilenskii A.M., Dyuzhikov O.A. Basalts of Siberian Platform. Specific features of geology, composition and genesis of Permo-Triassic effusives. Novosibirsk: Nauka, Sibirskoe otdelenie, 1986, p. 245 (in Russian).
- Reichow M.K., Saunders A.D., White R.V. et al. Geochemistry and petrogenesis of basalts from the West Siberian Basin: An extension of the Permo-Triassic Siberian Traps, Russia. Lithos. 2005. Vol. Iss. 3-4, p. 425-452. DOI: 10.1016/j.lithos.2004.09.011
- Reichow M.K., Pringle M.S., Al’Mukhamedov A.I. et al. The timing and extent of the eruption of the Siberian Traps large igneous province: Implications for the end-Permian environmental crisis. Earth and Planetary Science Letters. 2009. Vol. 277. 1-2. p. 9-20. DOI: 10.1016/j.epsl.2008.09.030
- Vasilev Yu.R., Prusskaya S.N., Mazurov M.P. A New Type of Large-Scale Manifestation of Within-Plate Intrusive Trap Magmatism (West Siberian Craton). Doklady Earth Sciences. 2007. Vol. 413. N 2, p. 187-191. DOI: 10.1134/S1028334X07020109
- Almukhamedov A.I., Medvedev A.Ya., Zolotukhin V.V. Chemical evolution of the Permian-Triassic basalts of the Siberian platform in space and time. 2004. Vol. 12. N 4, p. 297-311.
- Sluzhenikin S.F., Yudovskaya M.A., Barnes S.J. et al. Low-Sulfide Platinum Group Element Ores of the Norilsk-Talnakh Camp. Economic Geology. 2020. Vol. 115. N 6, p. 1267-1303. DOI: 10.5382/econgeo.4749
- Krivolutskaya N.A. Siberian Traps and Pt-Cu-Ni Deposits in the Norilsk Area. Cham: Springer, 2015, p. 364. DOI: 10.1007/978-3-319-17205-7
- 37. Krivolutskaya N.A., Ariskin A.A., Sluzhenikin S.F., Turovtsev D.M. Geochemical Thermometry of Rocks of the Talnakh Intrusion: Assessment of the Melt Composition and the Crystallinity of the Parental Magma. 2001. Vol. 9. N 5, p. 389-414.
- Dyuzhikov O.A., Sharkov E.V. Hypermafic-mafic volcanogenic-plutonogenic associations of the North Asian craton and the Baltic Shield. Geodinamika, magmatizm, sedimentognez i mineralgeniya Severo-Zapada Rossii: Materialy Vserossiiskoi konferentsii, 12-15 noyabrya 2007, Petrozavodsk, Rossiya. Petrozavodsk: Institut geologii KarNTs RAN, 2007, p. 126-129 (in Russian).
- Malitch K.N., Belousova E.A., Griffin W.L. et al. Magmatic evolution of the ultramafic–mafic Kharaelakh intrusion (Siberian Craton, Russia): insights from trace-element, U–Pb and Hf-isotope data on zircon. Contributions to Mineralogy and Petrology. 2010. Vol. 159. Iss. 6, p. 753-768. DOI: 10.1007/s00410-009-0452-z
- Dneprovskaya M.B., Frenkel M.Ya., Yaroshevskii A.A. Quantitative model of layering formation in the Talnakhskii intrusive. Construction of models of ore-forming systems. Novosibirsk: Nauka, Sibirskoe otdelenie, 1987, p. 96-106 (in Russian).
- Ariskin A.A., Barmina G.S. Modelling of phase equilibria during crystallization of basaltic magmas. Moscow: Nauka, MAIK “Nauka/Interperiodika”, 2000, p. 363 (in Russian).
- Chayka I.F., Kamenetsky V.S., Zhitova L.M. et al. Hybrid Nature of the Platinum Group Element Chromite-Rich Rocks of the Norilsk 1 Intrusion: Genetic Constraints from Cr Spinel and Spinel-Hosted Multiphase Inclusions. Economic Geology. 2020. Vol. 115. N 6, p. 1321-1342. DOI: 10.5382/econgeo.4745
- Barnes S.J., Yudovskaya M.A., Iacono-Marziano G. et al. Role of volatiles in intrusion emplacement and sulfide deposition in the supergiant Norilsk-Talnakh Ni-Cu-PGE ore deposits. Geology. 2023. Vol. 51. N 11, p. 1027-1032. DOI: 10.1130/G51359.1
- Turovtsev D.M. Contact metamorphism of Norilsk intrusions. Moscow: Nauchnyi mir, 2002, p. 318 (in Russian).
- Pokrovskii B.G., Sluzhenikin S.F., Krivolutskaya N.A. Interaction Conditions of Norilsk Trap Intrusions with Their Host Rocks: Isotopic (O, H, and C) Evidence. Petrology. 2005. Vol. 13. N 1, p. 49-72.
- Naumov V.B., Dorofeeva V.A., Girnis A.V., Yarmolyuk V.V. Mean concentrations of volatile components, major and trace elements in magmatic melts in major geodynamic environments on Earth. I. Mafic melts. Geochemistry International. 2017. Vol. 55. N 7, p. 629-653. DOI: 10.1134/S0016702917070060
- Hofmann A.W. Chemical differentiation of the Earth: the relationship between mantle, continental crust, and oceanic crust. Earth and Planetary Science Letters. 1988. Vol. 90. Iss. 3, p. 297-314.
- Lightfoot P.С., Keays R.R. Siderophile and Chalcophile Metal Variations in Flood Basalts from the Siberian Trap, Norilsk Region: Implications for the Origin of the Ni-Cu-PGE Sulfide Ores. Economic Geology. 1995. Vol. 100. N 3, p. 439-462. DOI: 10.2113/gsecongeo.100.3.439
- Grinenko L.I. Sources of sulfur of the nickeliferous and barren gabbro-dolerite intrusions of the northwest Siberian platform. International Geology Revew. 1985. Vol. 27. Iss. 6, p. 695-708. DOI: 10.1080/00206818509466457
- Ripley E.M., Lightfoot P.C., Li C., Elswick E. Sulfur isotopic studies of continental flood basalts in the Noril’sk region: implications for the association between lavas and ore-bearing intrusions. Geochimica et Cosmochimica Acta. 2003. Vol. 67. Iss. 15, p. 2805-2817. DOI: 10.1016/S0016-7037(03)00102-9
- Zolotukhin V.V., Ryabov V.V., Vasiliev Yu.R., Shatkov V.A. Petrology of Talnakh ore-bearing differentiation trappean intrusion. Novosibirsk: Nauka, Siberian Branch, 1975. Iss. 186, p. 436 (in Russian).
- Ryabov V.V., Simonov O.N., Snisar S.G., Borovikov A.A. The source of sulfur in sulfide deposits in the Siberian Platform traps (from isotope data). Russian Geology and Geophysics. 2018. Vol. 59. N 8, p. 945-961. DOI: 10.1016/j.rgg.2018.07.015
- Li С., Ripley E.M., Naldrett A.J. et al. Magmatic anhydrite-sulfide assemblages in the plumbing system of the Siberian Traps. Geology. 2009. Vol. 37. N 3, p. 259-262. DOI: 10.1130/G25355A.1
- Polyanskii O.P., Reverdatto V.V. Role of fluid in heat and mass transfer during evolution of sedimentary basins with trap magmatism. Flyuidy i geodinamika: Materialy Vserossiiskogo simpoziuma “Glubinnye flyuidy i geodinamika”, 19-21 noyabrya 2003, Мoscow, Russia. Moscow: Nauka, 2006, p. 219-243 (in Russian).
- Reverdatto V.V., Babichev A.V., Korobeinikov S.N., Polyanskii O.P. Estimation of the Emplacement Depth of a Magmatic Intrusive Body Based on the Data for Distribution of Isogrades in the Surrounding Metamorphic Zoning (Model Approximation). Doklady Earth Sciences. 2010. Vol. 430. Part 2, p. 244-247. DOI: 10.1134/S1028334X10020212
- Krivolutskaya N.A. Mantle Origin of Heavy Isotopes of Sulfur in Ores of the Norilsk Deposits. Doklady Earth Sciences. 2014. Vol. 454. Part 1, p. 76-78. DOI: 10.1134/S1028334X14010206
- Zhuo-sen Yao, Mungall J.E. Linking the Siberian Flood Basalts and Giant Ni-Cu-PGE Sulfide Deposits at Norilsk. Journal of Geophysical Research: Solid Eart. 2021. Vol. 126. Iss. 3. N e2020JB020823. DOI: 10.1029/2020JB020823
- Sobolew W. Petrologie der trappen des Sibirischen tafellandes. Transactions of the Arctic institute. Leningrad: Izd-vo glavnogo upravleniya Severnogo morskogo puti, 1936. Vol. 43, p. 224 (in Russian).
- Dolgal A.S., Kostitsyn V.I., Novikova P.N. et al. Approximation of gravity anomalies in regional studies taking into account the spherical shape of the Earth. Geophysics. 2021. N 5, p. 35-43 (in Russian).
- Gritsenko Y.D., Kondrikova A.P., Gilbricht S. et al. Quantitative assessment of the relative roles of sulfide liquid collection, magmatic degassing and fluid-mediated concentration of PGE in low-sulfide ores of the Norilsk intrusions. Ore Geology Reviews. 2022. Vol. 148. N 105042. DOI: 10.1016/j.oregeorev.2022.105042
- 6 Aleksandrova T.N., O’Connor C. Processing of platinum group metal ores in Russia and South Africa: current state and prospects. Journal of Mining Institute. 2020. Vol. 244, p. 462-473. DOI: 10.31897/PMI.2020.4.9
- Aleksandrova T.N., Afanasova A.V., Kuznetsov V.V., Aburova V.A. Selection of copper–nickel sulfide ore flotation parameters based on floatability ranking of flotation components. Mining Informational and Analytical Bulletin. 2022. N 1, p. 131-147 (in Russian). DOI: 10.25018/0236_1493_2022_1_0_131