A new insight into recording the mineral composition of carbonate reservoirs at well killing: experimental studies
- 1 — Leading Engineer Branch of OOO “LUKOIL-Engineering” “PermNIPIneft” in Perm ▪ Orcid
- 2 — Ph.D., Dr.Sci. Associate Professor Perm National Research Polytechnic University ▪ Orcid ▪ Scopus ▪ ResearcherID
- 3 — Ph.D., Dr.Sci. Professor Perm National Research Polytechnic University ▪ Orcid ▪ Scopus ▪ ResearcherID
Abstract
Well killing operation remains an important technological stage before well workover or servicing, during which filtrate penetrates the bottomhole area of the formation. The impact of process fluids and their filtrate on rock has a significant influence on permeability and porosity of carbonate reservoirs, which decrease due to fines migration. There are few known scientific studies of the interaction of killing fluid filtrate with carbonate rock and fines migration. In our experiments, an aqueous phase was used which is the basis for well killing in pure form, for the preparation of blocking agents and is used in reservoir pressure maintenance system. Core samples taken from the pay of the reservoir were used to simulate the well killing process with generation of reservoir thermobaric conditions. Killing fluid filtrate was kept for seven days, which characterizes the average workover time at flowing wells in the fields of the Perm Territory. Using micro-X-ray tomography and scanning electron microscope, images were obtained before and after the experiment, which allowed confirming a decrease in total number of voids due to fines migration and, as a consequence, a decreasing permeability of samples. Measurement of pH and fines concentration in the aqueous phase was performed before and after the experiment and pointed to mineral reactions occurring as a result of rock dissolution. The results of experiments made it possible to record a decrease in permeability of carbonate samples by an average of 50 % due to clogging of void space and migration of fines (clayey and non-clayey).
Funding
The research was conducted with support of the Ministry of Science and Higher Education of the Russian Federation (project NFSNM-2024-0005).
Introduction
Killing fluids are used in well workover to prevent the formation fluids from penetrating into the well-bore [1, 2]. As a rule, in this case, the killing fluid filtrate penetrates the bot-tomhole area of the formation, which can lead to deterioration of reservoir properties or “damage of the formation” [3-5]. The influence of process fluid filtrate on rock properties is more significant in reservoirs characterized by a high permeability and a network of cracks and cavities [6, 7]. The main mechanisms causing formation damage include incompatibility of filtrate with formation fluid, rock and filtrate, penetration and deposition of solid particles in the void space of rock, chemical adsorption, swelling of clay minerals, migration of fines and biological activity [8]. Well capacity after workover will largely depend on the interaction degree between the filtrate of process fluids and rock in the bottomhole area [9, 10]. The main and urgent task when working with the pay is to minimize the negative impact of filtrate of process fluids, including those used for well killing, on reservoir properties.
The technologies using aqueous solutions of inorganic salts or blocking agents in aqueous phase are applied to kill wells in oil fields of the Perm Territory. Back pressure on the formation is created, due to which the killing fluid (or its filtrate) penetrates the reservoir. Interaction of killing fluid filtrate with rock-forming minerals and formation fluids leads to a deterioration in reservoir properties of the formation in the penetration zone. As a consequence, the process of bringing the well to stable produc-tion becomes more complicated, and well capacity decreases [11].
Specific features of interaction of drilling fluid filtrate and killing fluids with terrigenous and shale rocks are well studied [12-14]. The investigations showed that the content of aqueous phase and its chemical composition had a major impact on petrophysical properties of rock. The content of aqueous phase can influence the structure and the integrity of rock as well as the swelling of clay minerals, which leads to a change in the void space [15]. For example, the authors of [16] studied the effect of duration of interaction between process fluid filtrate and the rock, and found that after five days of exposure, the porosity of the Berea Buff field reservoir decreased by 41 %, which is associated with serious changes in rock pore structure.
However, for carbonate reservoirs there are few similar studies related to the assessment of changes in their properties under the influence of process fluids including killing fluids. Carbonate rocks, like sandstones, can have a complex mineralogical composition [17]. Silicate minerals have a significant impact on physical and chemical properties of carbonate rock due to their small particle size, large surface area and cation-exchange capacity [18]. In study [19], the authors investigated the effect of injected “smart” water on clay minerals in carbonate rocks. To evaluate the swelling and migration of clays, they used microfluidic tests with the presence of kaolinite and montmorillonite clays. As a result of the study, it was ascertained that the injected water with salt concentration below critical led to swelling and migration of clay minerals and, as a consequence, a decrease in the number of voids and rock permeability.
Analysis of scientific literature shows that the influence of mineralogy or the initial internal struc-ture of rock on the specific features of well killing is considered in few studies. In papers [20, 21] it was concluded that a heterogeneous pore size or pore shape distribution will affect the dissolution mechanisms and lead to different dissolution patterns within the rock. Other studies [22, 23] determined new dissolution patterns related to the internal structure of rock. On a large scale, major structural heterogeneities, such as cracks, affect the rate and form of rock dissolution [24].
This study is aimed at investigating the influence of killing fluid filtrate on reservoir properties of carbonate formations. The study makes a new contribution to analysing the interactions between the filtrate of process fluids (fluid for well killing) and carbonate sediments in two fields in the Perm Territory (Sukharev and Vinnikovskoe), a detailed geological description of which is presented in [17]. Chemical composition of fluid filtrate, mineralogical composition of carbonate rocks, formation pressure and temperature, and extent of the “filtrate – rock” contact are considered in this work as the key factors influencing the reservoir properties.
Methodology
To study the impact of process fluids on carbonate reservoirs (Tournaisian-Famennian), 10 samples were prepared from the pay of reservoir in the Sukharev and Vinnikovskoe fields. The choice of these formations was due to different structure of the void space and mineral composition of inclusions and new growths, which were assessed using X-ray phase and X-ray fluo-rescence analyses, scanning electron microscopy and micro-X-ray tomography [17].
Microscopic studies of core samples with diagnostics of minerals based on microprobe analysis and the description of specific features of microstructure and microtexture as well as the construction of 3D models of the structure of core samples with histograms of void distribution by size were con-ducted before and after the filtration experiments on assessment of the impact of killing fluid filtrate on rock.
Then, core samples were prepared for filtration experiments, while residual water saturation was produced using formation water specially taken from the well in the reservoir. Filtration tests were conducted on core testing unit UIK-5VG and AFS-300 under thermobaric formation conditions: pore pressure 18 MPa, hydraulic compression pressure 30 MPa, temperature 30±1 °C. Reservoir oil with viscosity of 4.9 mPa×s was used in experiments. Holding time of killing fluid filtrate is seven days, it characterizes average duration of flowing wells workover in the fields under consideration. Filtration experiments were accomplished according to the following scheme [25]:
- a sample saturated with kerosene is placed in a filtration unit;
- thermodynamic conditions are created;
- kerosene is filtered through the sample in a direction simulating the “formation – well” operation, permeability to kerosene is determined;
- kerosene in the sample is replaced by oil through its filtration in the amount of at least three pore volumes;
- oil flow from the formation into the well is simulated by filtering oil through the sample in the corresponding direction; permeability of sample to oil is determined (Ko1);
- well killing is simulated with penetration of process water into formation, for which it is pumped through the sample in the “well – formation” direction and maintained under specified thermodynamic conditions for seven days (average workover duration);
- well completion after workover is simulated, oil is filtered through the sample in the “formation-well” direction, permeability to oil (Ko2) and porosity of samples to helium are determined.
Thus, a series of experiments allowed determining the porosity and permeability of core samples to oil before and after well killing simulation with process water. In the course of experiments, the quantitative chemical analysis of the aqueous phase (filtrate) of well killing fluid was performed.
Results
Assessment of chemical analysis of killing fluid filtrate is presented below: pH value – 6.99; permanganate oxidability – 3.3 mg/dm3; hydrocarbonate ion – 131.15 mg/dm3; chloride ion – 76.33 mg/dm3; sulphate ion – 68.33 mg/dm3; nitrite nitrogen – 0.0 mg/dm3; nitrate ion – 0.34 mg/dm3; carbonate ion – 0.0 mg/dm3; calcium – 60.14 mg/dm3; total hardness – 3.64 °dH; total iron – 0.14 mg/dm3; iron (3+) – 0.0 mg/dm3; ammonium ion – 0.0 mg/dm3; free carbon dioxide – 17.60 mg/dm3; dry residue – 313 mg/dm3; magnesium (calculation) – 7.7 mg/dm3; total mass concentration of sodium and potassium ions (calculation) – 50.12 mg/dm3.
Based on results of chemical analysis, it was ascertained that the aqueous phase corresponded to the first category according to SanPin 2.1.4.1074-01 and was characterized as low-mineralized.
The results of filtration experiments on simulation of well killing operation are given in Table 1 and Fig.1. Table 1 also presents the results of assessing the open porosity of core samples before and after filtration experiments. Table 1 shows the most informative results of filtration studies.
Table 1
Determination of core samples permeability to oil and porosity before and after well killing simulation [25]
Field (sample number) |
Permeability, 10–3 µm2 / Porosity, % |
|
Before filtrate injection Ko1 |
After filtrate injection Ko2 |
|
Sukharev field (1) |
78.10/8.28 |
37.83/8.04 |
Sukharev field (2) |
41.70/5.80 |
11.8/5.25 |
Sukharev field (3) |
1.62/8.07 |
0.76/8.04 |
Sukharev field (4) |
4.70/6.72 |
2.65/6.57 |
Vinnikovskoe field (5) |
7.51/14.20 |
6.53/14.19 |

Fig.1. Dynamics of performance indicators for filtration experiments on well killing simulation on core sample N 1 from Sukharev field
Fig.2 shows the results of X-ray tomographic studies of core sample N 1 before and after well killing simulation. In Fig.2, a, colour shows the accumulated pore opening in accordance with the scale for the sample depth: brown and yellow hues are high values, blue and purple hues are low ones.
It was ascertained that as a result of the impact of killing fluid filtrate on rock, total number of voids decreased by 32.4 % (mainly due to voids in the range of 46-69 µm), and the volume of voids by 13.1 %.
The results of scanning electron microscopy before well killing simulation made it possible to determine that the main part of the void space was represented by microcavities and intergranular pores. They have different shapes and sizes, are filled with newly formed calcite crystals, between which there are intergranular pores, usually communicating. An extensive distribution of cracks of considerable extent is especially worth noting; crack opening reaches 0.2 mm. Based on relative orientation in rock, two systems of cracks can be distinguished approximately perpendicular to each other. Both the rock matrix and the newly formed substance have a calcite composition with microimpurities of silica, alumina and alkali metals, which can be associated with appearance of clayey matter (Table 2 and Fig.3).
Table 2
Chemical composition of limestone components, wt. %
Oxide |
Before simulation |
After simulation |
||
Matrix |
Inclusions in cavities and cracks |
Matrix |
Inclusions in cavities and cracks |
|
CaO |
56.32 |
54.59 |
55.08 |
53.53 |
MgO |
0.21 |
0.31 |
0.20 |
0.39 |
FeO |
0.14 |
0.07 |
0.08 |
0.73 |
SiO2 |
0.15 |
0.51 |
0.09 |
0.45 |
TiO2 |
|
0.07 |
|
0.01 |
Al2O3 |
|
0.10 |
|
0.14 |
K2O |
|
0.04 |
|
0.02 |
Na2O |
|
0.25 |
|
0.46 |

Fig.2. Results of X-ray tomographic studies of core sample N 1 before (A) and after (B) well killing simulation: a – void map; b – histogram of void size distribution
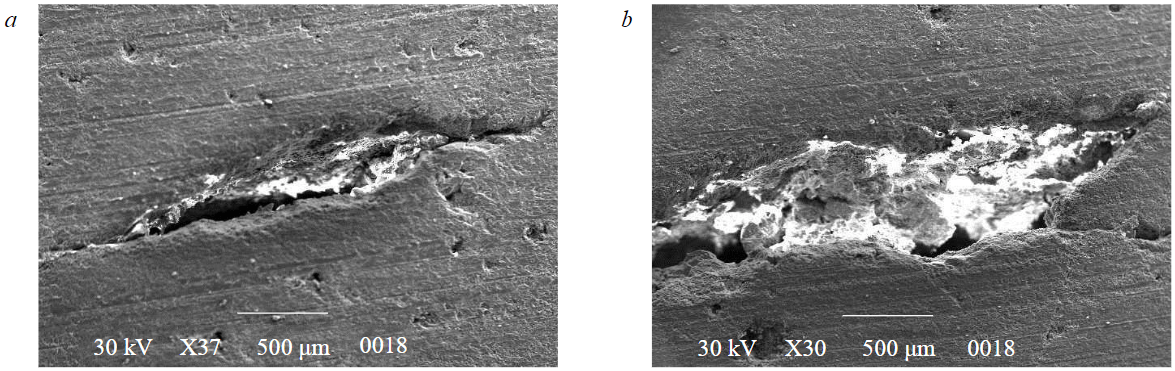
Fig.3. Scanning electron microscopy results before (a) and after (b) well killing simulation [25]

Fig.4. Scanning electron microscopy results before and after well killing simulation: a – newly formed calcite crystals in microcavities; b – amoeba-like carbonate secretions; c – pseudomorph after spicule; d – inclusions of ferruginous minerals
Scanning electron microscopy studies after filtration experiments showed that the void space was partly filled with fines. There are two types: clayey (montmorillonite and illite) and non-clayey (quartz, feldspar and carbonates). Newly formed calcite crystals to 0.1 mm in size also occur, especially in microcavities (Fig.4, a). The formation of amoeba-shaped films and aggregates recorded in certain areas of the sample should be noted (Fig.4, b).
Recrystallization phenomena with formation of larger calcite precipitates were recorded. These new formations often do not have a clear crystallographic faceting and are often round. During recrystallization fossils are replaced by calcite (Fig.4, c), ferrugination occurs showing up in the formation of films on the carbonate matrix surface and the appearance of newly formed calcite crystals (Fig.4, d).
Features of microstructure illustrated in Fig.4 were not recorded in the course of similar studies before the filtration experiments, which points to their technogenic nature. Scanning electron microscopy images show the expansion of voids caused by dissolution and their blockage due to fines migration, grain redistribution, and mineral precipitation. Mineral reactions dissolve the surface of grains and intergranular cement releasing rock grains, which leads to a decrease in permeability and corresponds to the conclusions given in [26]. The results presented in [23] also showed that the presence of oil in rock voids significantly slowed down rock creep under pressure. Oil covers calcite crystals and inhibits this process preventing the contact between the rock and aqueous phase, which is necessary for dissolution, transfer and precipitation of calcite [27, 28]. However, sticking of hydrocarbons to the surface of calcite can change its wetting properties and, thus, prevent diagenetic reactions (generation of secondary porosity). In relation to the described experiments, the formation of secondary voids is not recorded in the scanning electron microscope images. Interaction of killing fluid filtrate and clay minerals leads to swelling and migration and, further, to a decrease in permeability [29, 30].
Thus, comprehensive tomography and scanning electron microscopy results show that the penetration of killing fluid filtrate into rock disturbs the geochemical equilibrium between the formation fluids and rock minerals, thereby causing dissolution of carbonate minerals.
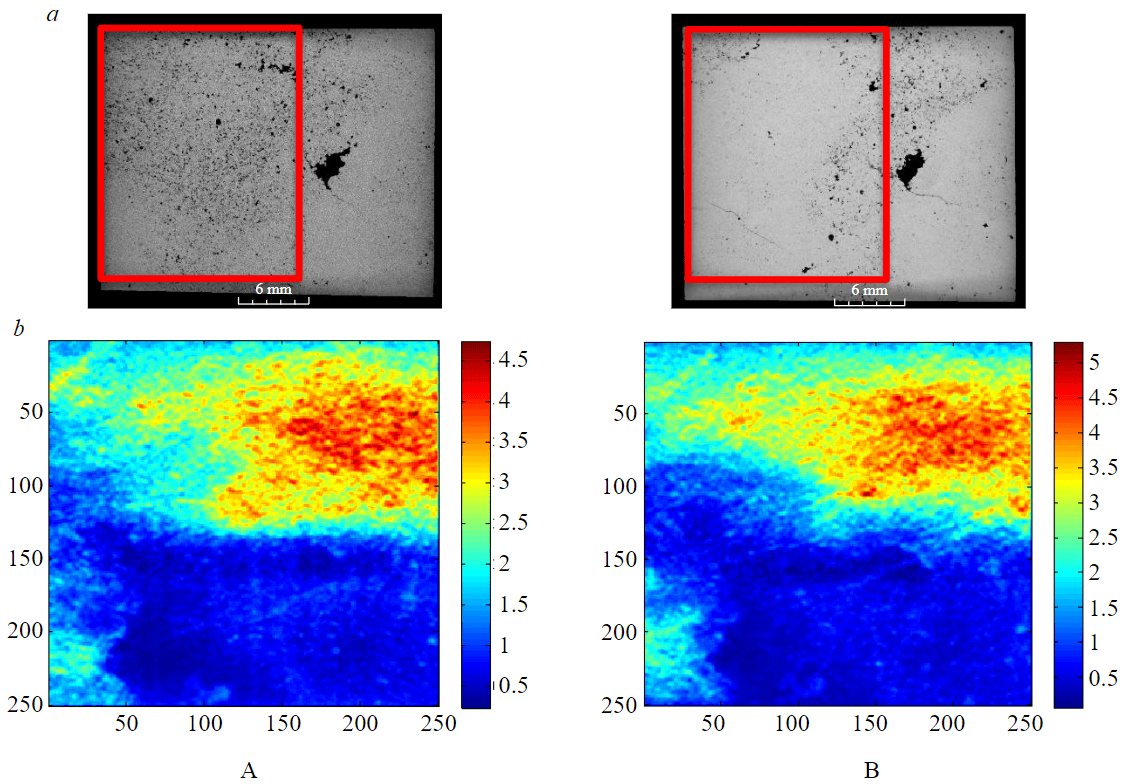
Fig.5. Results of X-ray tomographic studies of core sample N 2 before (A) and after (B) well killing simulation: a – section of tomogram of samples, density is shown as a grey gradient; b – void map
Fig.5 shows the results of X-ray tomographic studies of core sample N 2 before and after well killing simulation, which confirm the previously described decrease in reservoir properties (Table 1).
Comparison of tomography results before and after well killing simulation shows that there were changes in the structure of the void space of rock. This conclusion is confirmed by the quantitative distribution of voids – before the impact, the proportion of voids less than 100 μm in size in the sample was about 60 %, and after it, only about 40 %.
Contrasting results were obtained at well killing simulation on core samples from the Vinnikovskoe field. When comparing the results of tomographic studies and scanning electron microscopy, no major changes in the structure of the void space were recorded. This conclusion is clearly illustrated by the histogram of void distribution (Fig.6), from which it follows that the number of voids decreased by only 2 %.
The analysis of pH assessment of killing fluid filtrate indicates its change as well as an increase in chemical elements in the sample when simulating on core samples from the Sukharev field, which points to mineral reactions [31-33] occurring as a result of rock dissolution. A change in pH after well killing simulation was 6.99 before holding at the Sukharev and Vinnikovskoe fields, and after holding 7.27 at the Sukharev field and 7.01 at the Vinnikovskoe field.
Discussion
Currently, there are virtually no publications presenting the results of detailed studies on the impact of low-mineralized water on carbonate rocks, in contrast to terrigenous rocks. This area of research was not touched upon by scientists, since it is commonly presumed that carbonate reservoirs do not contain clay minerals that can, upon contact with low-mineralized water, change the wettability and, as a consequence, the permeability of rock [34].
At the same time, dissolution of carbonates is one of the main mineral reactions that can cause fines to break off and thereby change the rock permeability. In the course of the described experiments, when killing fluid filtrate was injected into carbonate rock, an increase in injection pressure was recorded (see Fig.1), which is, probably, due to fines migration, clogging of voids and is confirmed by the results of research [35, 36]. Due to dissolution of carbonates, clay minerals are also separated and can partly clog the void space, which is confirmed by scanning electron microscopy (see Fig.3).
There are scientific publications describing the processes of interaction of water with different salinity with carbonate rocks, but mostly in relation to waterflooding processes in order to increase the recovery of hydrocarbon reserves. We present several results that show the effect of injecting water of different salinity on the properties of carbonate reservoirs. It is known that a decrease in water salinity causes swelling of dispersed clay due to osmotic pressure and cation exchange depending on minerals and their crystal structure [37, 38]. It was shown in [39] that when mineralized water comes into contact with clay minerals, and the surface charge of cations is greater than that of salt ions, water will migrate into the space between clay layers and, therefore, cause their swelling. Montmorillonite clay has a higher swelling ability due to its very high cation exchange capacity compared to kaolinite. Article [40] states that montmorillonite clay became swollen from ~3.9 to 5.1 μm in diameter (~30 %) after brine salinity (NaCl) decreased from 5,000 mg/l to 0 (fresh water). The authors of [41] found that after replacing the injected fluid from formation water (9,662.9 mg/l) with fresh water, the permeability of core samples decreased by an average of 38 %. Thus, clay swelling can lead to a major decrease in reservoir properties of the formation since the exfoliated clays increase in size and are suspended until they re-precipitate in voids. The initial size of clay particles is the main factor determining their behaviour at break-off. Articles [27, 36] show that salinity decrease of injected water below the critical concentration causes swelling and migration of clay minerals and a subsequent decrease in permeability. When studying clay migration in the presence and absence of oil, it was found in [42] that at salinity below 4,000 ppm, clay migration occurs in both cases.

Fig.6. Results of X-ray tomographic studies of core sample from Vinnikovskoe field before (A) and after (B) well killing simulation: a – results of scanning electron microscopy; b – void map; c – histogram of void size distribution
It is possible that the mechanisms of the investigated processes (waterflooding and well killing operations) are in many ways similar, however, the duration of interaction of the “water – rock” system and the dynamics of the process proper are different, which will determine the degree of reservoir clogging. Therefore, it can be concluded that our study presents a new insight into the interaction processes of water-based killing fluids with carbonate rocks. The published works indicated that the migration of fines is possible only in sandy rocks due to a higher concentration of potentially transported material. The research presented in this article points to manifestation of the described effect in carbonates also, which leads to a significant decrease in reservoir properties to be taken into account when using the aqueous phase as various types of process fluids.
A number of articles [43-45] indicate that a conventional acid attack rather efficiently eliminates clogging of voids with fines and increases rock permeability, however, planning of acid treatment requires a separate study.
Conclusion
This article focuses on the influence of killing fluid filtrate on reservoir properties of carbonate formations in oil fields in the Perm Territory. Using core samples from the Sukharev and Vinnikovskoe oil fields, the experimental studies with simulation of actual conditions of well killing operations were conducted. The use of micro-X-ray tomography and a scanning electron microscope before and after the simulation experiments allowed recording a decrease in total number of voids caused by migration and clogging by fines and, as a consequence, a decrease in permeability of core samples. Thus, the use of low-salinity water as a base for killing fluids or an agent for the formation pressure maintenance system for the carbonate reservoir at the Sukharev field will involve a high risk of void space clogging due to dissolution and precipitation of carbonate rock minerals. Promising trends are: changing water salinity or the use of nanoparticles to maintain the geochemical equilibrium and reduce mineral dissolution of rocks.
References
- Mardashov D.V. Development of blocking compositions with a bridging agent for oil well killing in conditions of abnormally low formation pressure and carbonate reservoir rocks. Journal of Mining Institute. 2021. Vol. 251, p. 667-677. DOI: 10.31897/PMI.2021.5.6
- 2. Zhi Zhang, Baojiang Sun, Zhiyuan Wang et al. Intelligent well killing control method driven by coupling multiphase flow simulation and real-time data. Journal of Petroleum Science and Engineering. 2022. Vol. 213. N 110337. DOI: 10.1016/j.petrol.2022.110337
- Ebrahimi M.A., Sanati A. On the potential of alyssum as an herbal fiber to improve the filtration and rheological characteristics of water-based drilling muds. Petroleum. 2022. Vol. 8. Iss. 4, p. 509-515. DOI: 10.1016/j.petlm.2021.04.005
- Mardashov D.V. Improving the efficiency of oil well killing at the fields of the Volga-Ural oil and gas province with abnormally low reservoir pressure. Bulletin of the Tomsk Polytechnic University. Geo Аssets Engineering. 2022. Vol. 333. N 7, p. 185-194 (in Russian). DOI: 10.18799/24131830/2022/7/3707
- Mardashov D.V., Bondarenko А.V., Raupov I.R. Technique for calculating technological parameters of non-Newtonian liquids injection into oil well during workover. Journal of Mining Institute. 2022. Vol. 258, p. 881-894. DOI: 10.31897/PMI.2022.16
- Krishna S., Ridha S., Vasant P. et al. Conventional and intelligent models for detection and prediction of fluid loss events during drilling operations: A comprehensive review. Journal of Petroleum Science and Engineering. 2020. Vol. 195. N 107818. DOI: 10.1016/j.petrol.2020.107818
- Dokhani V., Ma Y., Geng T. et al. Transient analysis of mud loss in fractured formations. Journal of Petroleum Science and Engineering. 2020. Vol. 195. N 107722. DOI: 10.1016/j.petrol.2020.107722
- de Azevedo Novaes A.M., de Faria R.M.B., da Silva M.A.P., Peçanha R.P. Evaluation of rock-fluid interaction in a carbonate reservoir: Backflow test and reactive transport simulations. Geoenergy Science and Engineering. 2023. Vol. 230. N 212158. DOI: 10.1016/j.geoen.2023.212158
- Jia H., Wu X. Killing fluid loss mechanism and productivity recovery in a gas condensate reservoir considering the phase behavior change. Petroleum Exploration and Development. 2017. Vol. 44. Iss. 4, p. 659-666. DOI: 10.1016/S1876-3804(17)30075-7
- Civan F. Chapter 1 – Overview of formation damage. Reservoir Formation Damage (Fourth Edition). Fundamentals, Modeling, Assessment, and Mitigation. 2023, p. 1-12. DOI: 10.1016/B978-0-323-90228-1.00023-6
- Martyushev D.A., Govindarajan S.K. Development and study of a Visco-Elastic Gel with controlled destruction times for killing oil wells. Journal of King University – Engineering Sciences. 2022. Vol. 34. Iss. 7, p. 408-415. DOI: 10.1016/j.jksues.2021.06.007
- Zhang Lufeng, Zhou Fujian, Zhang Shicheng et al. Evaluation of permeability damage caused by drilling and fracturing fluids in tight low permeability sandstone reservoirs. Journal of Petroleum Science and Engineering. 2019. Vol. 175, p. 1122-1135. DOI: 10.1016/j.petrol.2019.01.031
- Klungtvedt K.R., Saasen A. A method for assessing drilling fluid induced formation damage in permeable formations using ceramic discs. Journal of Petroleum Science and Engineering. 2022. Vol. 213. N 110324. DOI: 10.1016/j.petrol.2022.110324
- Chukwuemeka A.O., Amede G., Alfazazi U. A review of wellbore instability during well construction: Types, causes, prevention and control. Petroleum and Coal. 2017. Vol. 59. Iss. 5, p. 590-610.
- Han Cao, Zheng Zhang, Ting Bao et al. Experimental Investigation of the Effects of Drilling Fluid Activity on the Hydration Behavior of Shale Reservoirs in Northwestern Hunan, China. Energies. 2019. Vol. 12. Iss. 16. N 3151. DOI: 10.3390/en12163151
- Gamal H., Elkatatny S., Adebayo A., Bageri B. Effect of exposure time on the compressive strength and formation damage of sandstone while drilling horizontal wells. Journal of Petroleum Science and Engineering. 2020. Vol. 195. N 107590. DOI: 10.1016/j.petrol.2020.107590
- Martyushev D.A., Ponomareva I.N., Chukhlov A.S. et al. Study of void space structure and its influence on carbonate reservoir properties: X-ray microtomography, electron microscopy, and well testing. Marine and Petroleum Geology. 2023. Vol. 151. N 106192. DOI: 10.1016/j.marpetgeo.2023.106192
- Almutairi A., Wang Y., Le-Hussain F. Effect of type of ion and temperature on fines migration induced by mineral reactions during water injection into carbonate rocks. Journal of Environmental Management. 2023. Vol. 342. N 118193. DOI: 10.1016/j.jenvman.2023.118193
- Karami M., Sedaee B., Nakhaee A. Effect of different injection fluids scenarios on swelling and migration of common clays in case of permeability variations: a micromodel study. Journal of Petroleum Exploration and Production Technology. 2023. Vol. 13. Iss. 8, p. 1761-1787. DOI: 10.1007/s13202-023-01628-z
- Sentemov A.A., Dorfman M.B. Percolation approach in reservoir simulation of well treatment methods. Bulletin of the Tomsk Polytechnic University. Geo Аssets Engineering. 2022. Vol. 333. N 7, p. 157-165 (in Russian). DOI: 10.18799/24131830/2022/7/3612
- Dianshi Xiao, Shu Jiang, David Thul et al. Impacts of clay on pore structure, storage and percolation of tight sandstones from the Songliao Basin, China: Implications for genetic classification of tight sandstone reservoirs. Fuel. 2018. Vol. 211, p. 390-404. DOI: 10.1016/j.fuel.2017.09.084
- Qian Li, Jing Li, Baolong Zhu. Experimental investigation of the influence of sequential water-rock reactions on the mineral alterations and porosity evolution of shale. Construction and Building Materials. 2022. Vol. 317. N 125859. DOI: 10.1016/j.conbuildmat.2021.125859
- Neveux L., Grgic D., Carpentier C. et al. Influence of hydrocarbon injection on the compaction by pressure-solution of a carbonate rock: An experimental study under triaxial stresses. Marine and Petroleum Geology. 2014. Vol. 55, p. 282-294. DOI: 10.1016/j.marpetgeo.2014.01.012
- Leger M., Luquot L., Roubinet D. Role of mineralogical, structural and hydrodynamic rock properties in conduits formation in three distinct carbonate rock types. Chemical Geology. 2022. Vol. 607. N 121008. DOI: 10.1016/j.chemgeo.2022.121008
- Chernykh V.I. Experimental simulation of process liquids impact on complexly composed carbonate reservoirs. Oilfield engineering. 2023. N 8 (656), p. 30-34 (in Russian). DOI: 10.33285/0207-2351-2023-8(656)-30-34
- Wang Y., Almutairi A.L.Z., Bedrikovetsky P. et al. In-situ fines migration and grains redistribution induced by mineral reactions – Implications for clogging during water injection in carbonate aquifers. Journal of Hydrology. 2022. Vol. 614. Part A. N 128533. DOI: 10.1016/j.jhydrol.2022.128533
- Barnaji M.J., Pourafshary P., Rasaie M.R. Visual investigation of the effects of clay minerals on enhancement of oil recovery by low salinity water flooding. Fuel. 2016. Vol. 184, p. 826-835. DOI: 10.1016/j.fuel.2016.07.076
- Qiqiang Ren, Qiang Jin, Jianwei Feng et al. Mineral filling mechanism in complex carbonate reservoir fracture system: Enlightenment from numerical simulation of water-rock interaction. Journal of Petroleum Science and Engineering. 2020. Vol. 195. N 107769. DOI: 10.1016/j.petrol.2020.107769
- Khabibullin M.Y. Research of a reservoir bottom zone destruction by filtering flow of the formation liquid and prevention of call formation in the well. Bulletin of the Tomsk Polytechnic University. Geo Аssets Engineering. 2021. Vol. 332. N 10, p. 86-94 (in Russian). DOI: 10.18799/24131830/2021/10/3397
- Ghasemi M., Shafiei A. Influence of brine compositions on wetting preference of montmorillonite in rock/brine/oil system: An in silico study. Applied Surface Science. 2022. Vol. 606. N 154882. DOI: 10.1016/j.apsusc.2022.154882
- Zhichao Yu, Zhizhang Wang, Caspar Daniel Adenutsi. Genesis of authigenic clay minerals and their impacts on reservoir quality in tight conglomerate reservoirs of the Triassic Baikouquan formation in the Mahu Sag, Junggar Basin, Western China. Marine and Petroleum Geology. 2023. Vol. 148. N 106041. DOI: 10.1016/j.marpetgeo.2022.106041
- Malki M.L., Saberi M.R., Kolawole O. et al. Underlying mechanisms and controlling factors of carbonate reservoir characterization from rock physics perspective: A comprehensive review. Geoenergy Science and Engineering. 2023. N 211793. DOI: 10.1016/j.geoen.2023.211793
- Hao J., Mohammadkhani S., Shahverdi H. et al. Mechanisms of smart waterflooding in carbonate oil reservoirs – A review. Journal of Petroleum Science and Engineering. 2019. Vol. 179, p. 276-291. DOI: 10.1016/j.petrol.2019.04.049
- Al Shalabi E.W., Sepehrnoori K., Delshad M. Mechanisms behind low salinity water injection in carbonate reservoirs. Fuel. 2014. Vol. 121, p. 11-19. DOI: 10.1016/j.fuel.2013.12.045
- Feyzulaev H.A., Agalarova S.V. Forecasting of the Technological Parameters of the Oil Displacement with the Various Mineral Content Water in the Clay Storage Collector. SOCAR Proceedings. 2020. N 3, p. 135-141 (in Russian). DOI: 10.5510/OGP20200300454
- Song W., Kovscek A.R. Direct visualization of pore-scale fines migration and formation damage during low-salinity waterflooding. Journal of Natural Gas Science and Engineering. 2016. Vol. 34, p. 1276-1283. DOI: 10.1016/j.jngse.2016.07.055
- Ghasemi M., Shafiei A. Atomistic insights into role of low salinity water on montmorillonite-brine interface: Implications for EOR from clay-bearing sandstone reservoirs. Journal of Molecular Liquids. 2022. Vol. 353. N 118803. DOI: 10.1016/j.molliq.2022.118803
- Yang Y., Jaber M., Michot L.J. et al. Analysis of the microstructure and morphology of disordered kaolinite based on the particle size distribution. Applied Clay Science. 2023. Vol. 232. N 106801. DOI: 10.1016/j.clay.2022.106801
- Roshan H., Masoumi H., Zhang Y. et al. Microstructural effects on mechanical properties of shaly sandstone. Journal of Geotechnical and Geoenvironmental Engineering. 2018. Vol. 144. Iss. 2. N 06017019. DOI: 10.1061/(ASCE)GT.1943-5606.0001831
- Yan Zhuang, Xiangjun Liu, Hanqiao Xiong, Lixi Liang. Microscopic Mechanism of Clay Minerals on Reservoir Damage during Steam Injection in Unconsolidated Sandstone. Energy & Fuels. 2018. Vol. 32. Iss. 4, p. 4671-4681. DOI: 10.1021/acs.energyfuels.7b03686
- Wenchao Fang, Hanqiao Jiang, Jie Li et al. Investigation of salt and alkali sensitivity damage mechanisms in clay-containing reservoirs using nuclear magnetic resonance. Particulate Science and Technology. 2017. Vol. 35. Iss. 5, p. 533-540. DOI: 10.1080/02726351.2016.1170082
- Molnár Z., Pekker P., Dódony I., Pósfai M. Clay minerals affect calcium (magnesium) carbonate precipitation and aging. Earth and Planetary Science Letters. 2021. Vol. 567. N 116971. DOI: 10.1016/j.epsl.2021.116971
- Karimi M., Ayatollahi S. A new wormhole mechanistic model for radial acid flow geometry using novel 3D flow correlations. Geoenergy Science and Engineering. 2023. Vol. 230. N 212176. DOI: 10.1016/j.geoen.2023.212176
- Martyushev D.A., Govindarajan S.K., Li Y., Yang Y. Experimental study of the influence of the content of calcite and dolomite in the rock on the efficiency of acid treatment. Journal of Petroleum Science and Engineering. 2022. Vol. 208. Part E. N 109770. DOI: 10.1016/j.petrol.2021.109770
- Al-Shargabi M., Davoodi S., Wood D.A. et al. A critical review of self-diverting acid treatments applied to carbonate oil and gas reservoirs. Petroleum Science. 2023. Vol. 20. Iss. 2, p. 922-950. DOI: 10.1016/j.petsci.2022.10.005