Combined method of phytoremediation and electrical treatment for cleaning contaminated areas of the oil complex
- 1 — Ph.D., Dr.Sci. Professor Ufa State Petroleum Technological University ▪ Orcid
- 2 — Ph.D. Associate Professor Ufa State Petroleum Technological University ▪ Orcid
- 3 — Ph.D. Associate Professor Ufa State Petroleum Technological University ▪ Orcid
Abstract
The scale of land pollution with oil waste necessitates the use of economical and effective methods of recultivation. Phytoremediation is one of the simplest methods, but it has a number of limitations, so additional preparation of the territory is often required before it is carried out. Preliminary electrical preparation and subsequent seeding of special phytoremediants are of interest. Passing a constant electric current through the soil volume under a low voltage removes toxicants from deep soil layers even with flooding. In addition, it reduces pollutant content in the upper layer, where the plants root system is located, which creates more favorable conditions for phytoremediants. Adequately selected types of plants will ensure additional soil cleaning, improve its structure and air exchange. The results of two research directions are presented. Experiments on the study of plant resistance to oil-contaminated soil substrate allowed establishing contamination thresholds at which it is advisable to sow a particular species, and to choose optimal phytoremediants. The study of the oil-containing soil cleaning in a monocathodocentric electrochemical installation with the fixation of main characteristics (oil products concentration, soil temperature, volt-ampere characteristics) allows us to develop technical measures to prepare territories for phytoremediation taking into account the relief features.
Introduction
Anthropogenic pollution and degradation of soils are one of the most acute environmental problems. The total area of disturbed lands in the Russian Federation reached 1084.6 thousand hectares and continues to grow (an annual increase is to 7.7 thousand hectares) according to the official report of the Ministry of natural resources and ecology in 2020. Most of the disturbed territories belong to the category “industrial and other purpose lands”. The list of the main soils pollutants varies depending on the prevailing industries (heavy metals and oil products, residual pesticides and salts). The priority pollutants of oil-producing and oil-refining regions are oil products and various accompanying salt solutions. An accurate assessment of the oil-contaminated area is not possible due to the difficulty of identifying relatively small local leaks and spills [1]. The negative effects of the oil products on the environment are water-air imbalance, gluing, soil structural elements enlargement, violation of the soils bearing capacity, micro- and mesofauna suppression [2-4]. There is a wide range of soil-cleaning and remediation technologies with different characteristics. The combination of such methods taking into account the specificity of the practical tasks is the most effective [5-9].
Soil phytoremediation (i.e. the use of plants to remove pollutants and improve soil properties) is one of the most cost-effective technical directions [10-12]. However, it does not always provide sufficiently fast and effective cleaning. The intensity of phytoremediation significantly depends on the temperature of the soil-air environment, the light regime, the presence of biogenic elements, the form in which the toxicants are in the ground, etc. [13-16]. It should be taken into account that the ability of plants to absorb chemicals changes under phytogeochemical stress [17]. The combination of phytoremediation with preliminary preparation of the territory by other technologies allows improving the quality and speed of degradated lands remediation [18-22].
The preliminary preparation of the contaminated area by passing direct current through the soil massif to remove toxicants and further phytoremediant sowing is very interesting [23-26].
The electro-induced processes prepare the surface layer for sensitive phytoremediant plants and reduce the toxic components content at depth. So special wells are prepared in the soil by automatic or manual drilling, taking into account the degree of oil products vertical distribution. Cathodes and anodes connected to the power supply are mounted into wells. Small currents stimulate electro-oxidative [27-30] and electrokinetic reactions [31-34], promote stable complexes destruction and toxicants transformation into more bioavailable forms and cause other physicochemical phenomena [35]. The size, composition and texture of the electrodes are selected depending on soil properties, the characteristics of the horizontal and vertical toxicants distribution, the required treatment time and other conditions. The result of electrical remediation is the removal of various components (petroleum products, heavy metals, desalination). Periodic change of the electrodes polarity are practiced to avoid soil acidification during electrical treatment.
Objectives of this work are to establish the threshold values of oil pollution based on the analysis and synthesis of experimental data, at which seed germination and further growth of the studied plants are possible; to select tolerant plants species that are optimal for variable terrain and watering conditions; to study the specifics of energy-efficient soil preparation to species – phytoremediants due to preliminary electrochemical cleaning.
The presented research materials are aimed at developing the technology of complex recultivation of oil-contaminated soils. This technology includes higher flora species sowing with preliminary electrochemical soil preparation, taking into account the relief and the moisture degree.
The considered combined remediation method can be implemented on various soils. The type of plant (from the studied ones) that is optimal for specific conditions is selected at the phytoremediation stage of research. Also additional measures can be taken to improve the soil structure (mulching, organic fertilizers, claymation, etc.), if it is necessary. Electrochemical treatment is applicable on both sand, loam and clay.
The possibility of growing some types of plants on oil-contaminated soil was considered in the early works of the authors [36]. General patterns of electro-induced reduction of the oil products content in soils were established [37, 38]. This work continues and expands the cycle of research in these areas.
Methodology
The first stage of the experiments was studying the resistance of certain types of higher plants to oil pollution by germinating them on soil with different oil concentrations and determining the seeds germination percentage. The ultimate goal of these experiments was to select temperate zone plant species tolerant to oil pollution and to determine the threshold values of the toxicant content during their germination. The second stage included the electrical treatment of oil-contaminated soil at an experimental laboratory installation with an assessment of the cleaning degree and monitoring of the voltage, temperature, and acidity of the soil. These parameters allow defining the intensity and features of the cleaning process. This stage is aimed at developing effective measures for the preliminary preparation of heavily oil-contaminated soils for the subsequent selection of phytoremediants.
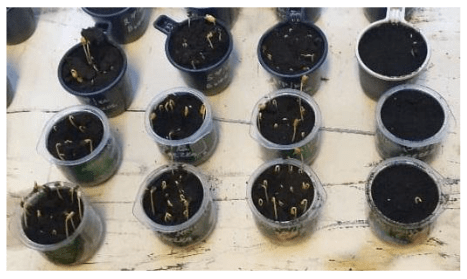
Fig.1. Containers with seedlings of tested Ipomoea purpurea (first row on the left) and Fagopýrum esculéntum (2-4 rows)
Experiments were carried out with a model soil based on leached chernozem. In all cases, oil with a density of 0.876 g/cm3 and a content (% by weight) of sulfur – 2.95, pa-raffins – 3.1, resins – 14.6, mechanical impurities – 0.880 was used to pollute the soil. Variations of the model soil were prepared for different series of experiments. The model soil composition corresponded to the contaminated soil taken from the spill sites at the oil field of the Republic of Bashkortostan. Research was carried out at an air temperature of 20 °C and atmospheric humidity of 67 %.
The first stage
Experiments to determine the resistance of higher plants species to soil oil pollution were carried out in spring and summer in the laboratory, and in autumn and winter with the use of the LCC-1000MP Diahan Labtech climate camera (to clarify the results). The illumination in the climate camera was maintained 4000 lux with the “day – night” photoperiod. The reaction of plants was studied by the method of seed germination in model soils with different concentrations of the toxicant with the calculation of the germination percent and further determination of the viable seedlings number.
First, the soil (leached chernozem) was prepared by removal of roots and small stones, sifting, weighing. 100 g of soil was poured into plastic containers. Different volume of crude oil (1; 2; 5; 10; 50; 100 ml) was put into each sample using a pipette, and then the soil was thoroughly rubbed and mixed to evenly contaminant distribution. Twenty seeds were sown in each container so that the soil layer covering them was about 1 cm. Then 60 ml of tap water was added. The control was soil without oil contamination. Three samples with each oil dose were prepared for experiments. The containers were kept at a temperature of 20 °C and the illumination mode corresponding to the daytime of spring-summer period. The number of seedlings was counted and the percentage of germination was determined after seven days in each container (Fig.1). The number of viable seedlines was recalculated to determine their survival after two weeks.
The results were used to identify the main effects exerted by different volumes of toxicant on the germination, with further detailed statistical processing.
The second stage
Electrotreatment of contaminated soil, which was carried out on a laboratory installation with a cylindrical cell, centric placement of the cathode and peripheral placement of anodes (Fig.2).
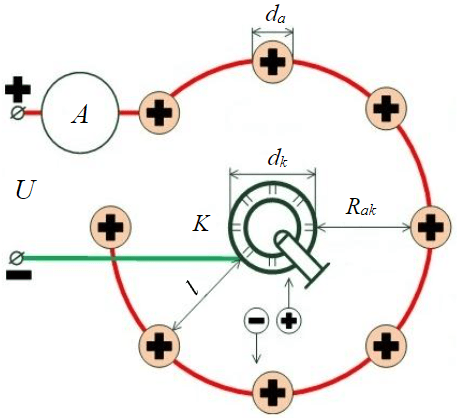
Fig.2. The diagram of the central cathode and peripheral anodes installation da – anodes diameter; dk – cathode diameter; l – interelectrode distance; Rak – distance between the centers of the cathode and anodes; K – central cathode; U – voltage between the cathode and anodes
It is necessary to remember that highly mineralised reservoir water become the limiting factor of plant development during the remediation of oil field spills. Therefore, it is important that the technology of preliminary preparation of the contaminated area for sowing phytoremediants not only reduces the oil products amount, but also desalinizes the soil. Therefore, the model soil variants containing oil (1 and 10 % by weight) and a solution of salts (40.8 % by weight) were prepared for the electrical treatment experiments. The composition of the salt solution corresponded to the reservoir waters of the deposit included (mg/kg) [39]: sodium chloride – 86000, calcium chloride – 10500, magnesium chloride – 5200, sodium sulfate – 4000, sodium bicarbonate – 400.
The central element of the electrochemical cell is a hollow cylindrical steel cathode with perforation on a surface (a drainage system for removing soil electrophoretic flow can be also connected, if it is necessary). Anodes are installed along the perimeter of the radial cell, at an equal distance from the cathode. All electrodes are connected to the corresponding pole of the power supply.
The installation used in the experiments had following characteristics: electrochemical cell diameter – 0.3 m; the soil layer height is 0.1 m; the cathode was made of a perforated steel tube, the anodes (with diameter 2.5 cm) are mesh cylinders (tubes) made of titanium with an oxide-ruthenium coating; the cell capacity reached 3 kg of model soil.
Several series of experiments on passing currents through chernozem containing different oil volumes were carried out. The constant amperage was maintained during the experiments: 0.8 A – in a series of experiments with 1 % soil contamination, 1 A in – a series with 10 % contamination. The use of these current values in each case is due to the fact that they are minimal, at which the voltage change between the electrodes is recorded, so we can talk about the appearance of electro-induced processes. The period of electric current passing was 360 min.
The oil products content in the soil was determined by IR spectrometry with the use of KN-2m. The parameters of voltage, temperature, humidity, and acidity were recorded every 30 minutes in the cathode, anode, and interelectrode zones. Multimeter, RGK thermal imager, digital soil moisture analyzer MC-7828SOIL, soil temperature and humidity analyzer HI9921 were used. Monitoring of these parameters allows determining the electrophysical reactions intensity.
Results and their discussion
Experiments on the plants reaction to soil pollution conducted over several years revealed some species resistant to the toxicants (Typha latifolia, Phragmites communis [36], Fagopýrum esculéntum and Ipomoea purpurea). These species have very different ecological niches, which allows them to be used at various territories.
Reliable inverse dependences of seed germination on the pollutants concentration were established in all cases after results statistical processing (Fig.3). The seeds of Ipomoea and Fagopýrum germinated more evenly, the seedlings condition was also more uniform in the same samples.
Experimentally obtained data on the toxicant level at which the seeds germination is equal to or exceeds the control values (i.e. a stimulating effect) are summarized in the Table. These values can be taken as a threshold level of pollution at which the use of a particular type of plants on oil-contaminated soils is effective. Moisture-loving Typha latifolia and Phragmites communis were additionally germinated with reservoir waters (the seedlings appeared in the sample with 5 ml content).
The main parameters of germination
Parameter |
Typha latifolia |
Phragmites communis |
Fagopýrum esculéntum |
Ipomoea purpurea |
Seed germination in the control, % |
52 |
43 |
65 |
75 |
Oil volume in the sample (germination is not less than control), ml |
10 |
5 |
10 |
2 |
Oil volume in the sample at zero germination, ml |
30 |
30 |
100 (single seedling) |
100 (single seedling) |
Regression equation, describing the dependence |
y = –2.642х2 + 12.35x + 42.8 |
y = –4x2 + 14.8x + 32.2 |
y = –6.964x2 + 37.17x + 33 |
y = –5.312x2 + 22.54x + 56.25 |
Approximation reliability |
r2 = 0.988 |
r2 = 0.999 |
r2 = 0.918 |
r2 = 0.955 |
The seedlings remained viable for 14 days after their appearance in all series of experiments (the period of monitoring their condition). They retain a green color without any damage to the leaf blades or yellowing. Thus, the data obtained indicate high resistance of all four species to oil pollution. These species can be used for seeding in polluted areas to improve soil properties both independently and during the final stage of complex remediation (for example, after the preliminary electrochemical treatment in our case).
Typha latifolia and Phragmites communis are moisture-loving wild-growing widely distributed plants and can be used for the recultivation of oil-contaminated lowlands and swampy areas. They can be sown at the territories of oil and reservoir water spills of oil deposits. It is necessary to create an irrigation system, if sowing is carried out during the dry summer period for normal germination and growth. These species are characterized by a decrease in the oil products content in the root zone, including due to the increased activity of hydrocarbon-oxidizing microorganisms [40].
The use of Amaranthus retroflexus L. after electrical treatment is also promising. It is a ubiquitous weed resistant to an increased content of oil products and mineral salts in the soil (experiments are planned).
Ipomoea purpurea is a climbing ornamental plant. It is suitable for use at urban territories with moderate moisture, for example, for sowing after electrical treatment of gas stations soils, areas along roads with fuel and lubricants spills, etc.
Fagopýrum esculéntum is a food and honey crops. However, it is recommended to use it exclusively as an agrotechnical culture in the context of studies, since it has a very intensive interchange of metabolic products between roots and leaves [41]. The issue of accumulation of harmful products of toxicants transformation in various parts of the plant requires study; therefore, it is rational not to eat the plant, when using it for final remediation.
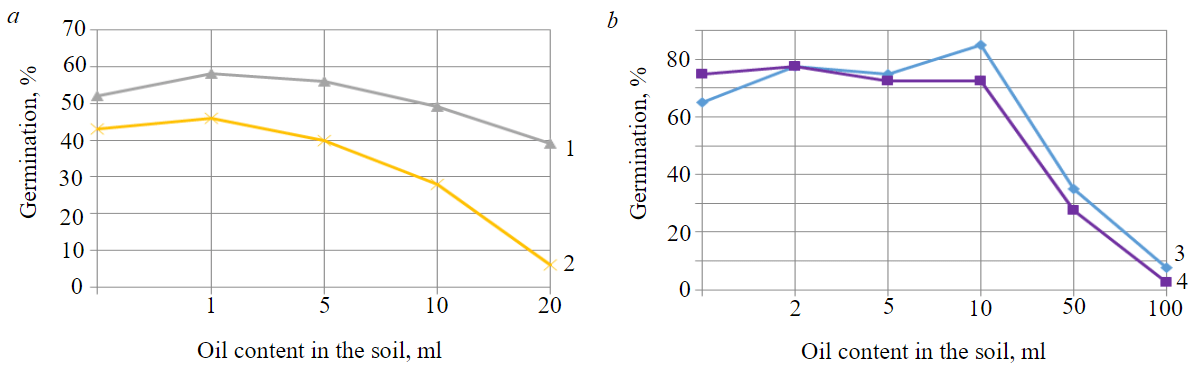
Fig.3. The dependence of the seeds germination on the oil content in the soil: а – experiments with Typha latifolia (1) and Phragmites communis (2); b – experiments with Fagopýrum esculéntum (3) and Ipomoea purpurea (4)
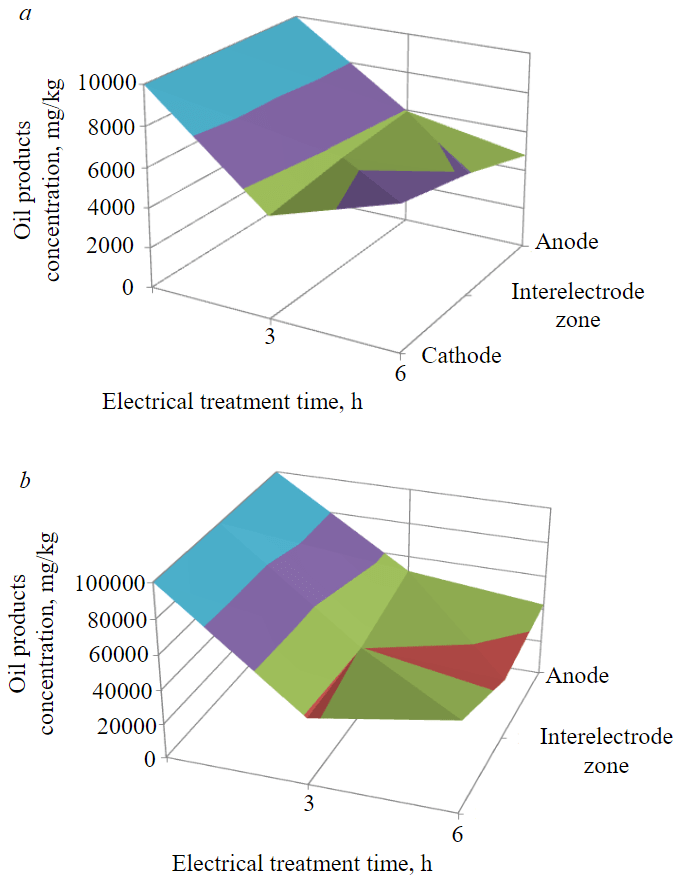
Fig.4. Spatio-temporal distribution of the oil products concentration in the soil in various series of experiments: а – the minimum level of pollution (1 %); b – the maximum level of pollution (10 %)
All the studied species are unpretentious to environmental conditions, they are often found in a temperate climate zone, seed material is easy to collect in the field (significant expenses for its purchase are not required).
Studies of oil-containing soil cleaning by low currents with cathodocentric electrode separation, which precede further phytoremediation, have shown significant efficiency. There was a decrease in the level of oil pollution in electrotreated soil (the cleaning degree is 47-65 %) in all cases. In addition, the oil products distribution over the interelectrode zones gradually occurs: there are an increase in their content at the cathode and a decrease at the anode (Fig.4).
The voltage increased from 8.9 to 9.4 V, when an electric current is passed through the soil with 1 % contamination. It varied in the range of 9.8-10.9 V in the soil with 10 % contamination. There is an increase in soil temperature in the cathode zone (temperature gradient is up to 8 °C), while the temperature in the anode and in the middle zones is almost the same (Fig.5). The use of a thermal imager makes it possible to analyze the soil temperature profile in the cell. The temperature zones highlighted in the images allow us to assess the electrical con ductivity of individual areas. Higher heating indicates the medium resistance increase and electrical conductivity decrease (the area with white-red coloring in Fig.5). This means that salts dissociation, migration and reduction of active ions (i.e. desalination occurs) is taking place at this areas. The pH distribution also confirms it. The alkaline medium is formed at the cathode as a result of the hydroxyl ions formation. The acidity increases in the rest of the electrochemical cell.
It is necessary to use special equations to estimate the energy consumption of this method on cathodocentric installations, taking into account the oil-contaminated soils parameters changes (in particular, resisti-vity) during the electrical process. The energy parameters of electrochemical cleaning (including in the real conditions) will be determined in this way. The total amperage between the anodes and the cathode is determined by the equation [37]
where N – is the number of anodes, pcs.; ρ – is the treating soil specific resistivity, Ω·m; l – is the interelectrode distance between the central cathode and the anodes, m.
The effective area of the electric current channel between the opposite charged electrodes are determined as follows:
where H – is the contamination depth, m.
The interelectrode distance is calculated by the equation
Specific electricity costs (J/kg of petroleum products) is determined by the equation
where V – is the oil-contaminated soil volume, m3; с0 – initial concentration of oil products, kg/m3.
The soil resistivity increases during the electrical treatment, as follows from experimental studies [39]. The specific resistance change in time can be estimated in the first approximation by the equation
where ρ0 – is the soil resistivity at the initial moment of time, Ω·m; ρk – is the final value of the soil resistivity at the last stage of electrical treatment, Ω·m; qsp – is the specific electric charge, which should ensure the oil-contaminated soil cleaning, С/kg, qsp values were established for clay, sandy soil and chernozem previously [39].
The specific energy consumption depending electrotheatment modes with constant value of amperage or voltage. So specific energy consumption for the constant voltage will be calculated with the use of equations (4) and (5):
specific energy consumption with a constant amperage is
The calculation of the energy efficiency of the laboratory installation using the above equations and the experimental data obtained showed that the specific energy consumption is 0.1139 MJ per 1 kg of soil containing 1 % of oil.
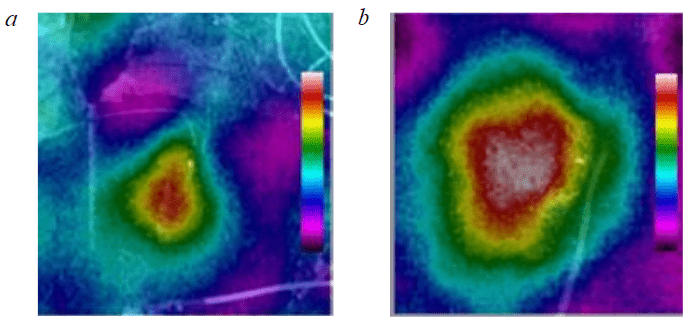
Fig.5. Thermal imaging of a radial cell with soil: initial (a) and final (b) treatment stages
The above methodology for determining energy consumption can be used in the design of specialized electrical cleaning installations for specific conditions, taking into account the properties of oil-contaminated areas and their contamination degree.
Conclusion
The conducted experimental and theoretical studies in two scientific fields allowed us to identify the main approaches for the implementation of a complex technology of oil-contaminated territories remediation.
Four types of plants – potential tolerant phytomeliorants suitable for the remediation of oil-contaminated soils of various territories have been selected. The thresholds values of the oil products content in the soil, which are recommended to phytoremediation were defined. Reliable dependences of seed germination on the oil products content have been established. It is proposed to use Typha latifolia and Phragmites communis species on highly moist soils, including hollows and ravines filled with water-oil emulsion and oil production brines (germination reaching and exceeding the control value with oil content up to 10 and 5 ml in the sample). It is advisable to use the Ipomoea purpurea in urban areas (the threshold volume of oil in the sample is 2 ml). Fagopýrum esculéntum is useful at the moderately moist agricultural areas exposed to oil spills (the threshold volume is 10 ml in the sample).
It is recommended to carry out electrochemical soils cleaning using a monocathode centric electrode placement scheme for preliminary preparation for phytoremediation and for oil products content reduction to the threshold values of specific species. This arrangement of the electrodes will allow to prepare the soil effectively even with a significantly rugged landscape, i.e. in the presence of lowlands and hills, in watered areas. The oil products cleaning degree in accordance with the conducted experiments reached 65 %. Temperature gradient during electric heating of soil was up to 8 °C. A method for determining some energy parameters of electroremediation process using a cathodocentric arrangement of electrodes, taking into account changes in soil resistance has been deve-loped.
The results obtained will contribute to the practical implementation of the technology of complex oil-contaminated soils cleaning based on phytoremediation with preliminary electrotreatment in real conditions.
References
- Pashkevich M.A., Bykova M.V. Improvability of measurement accuracy in determining the level of soil contamination with petroleum products. Mining Informational and Analytical Bulletin. 2022. N 4, p. 67-86 (in Russian). DOI: 10.25018/0236_1493_2022_4_0_67
- Pashkevich M.A., Antciferova T.A. Assessment of the impact of fuel and energy complex on the environment in the enlargement of production. Journal of Mining Institute. 2013. Vol. 203, p. 229-232 (in Russian).
- Dashko R.E., Lange I.Y. Forecasting bearing capacity change of sandy-argillaceous soils in course of their contamination by oil-products. Journal of Mining Institute. 2015. Vol. 211, p. 16-21 (in Russian).
- Pashkevich M.A., Bech J., Matveeva V.A., Alekseenko A.V. Biogeochemical assessment of soils and plants in industrial, residential and recreational areas of Saint Petersburg. Journal of Mining Institute. 2020. Vol. 241, p. 125-130. DOI: 10.31897/PMI.2020.1.125
- Wukui Zheng, Tian Cui, Hui Li. Combined technologies for the remediation of soils contaminated by organic pollutants. A review. Environmental Chemistry Letters. 2022. Vol. 20. Iss. 3, p. 2043-2062. DOI: 10.1007/s10311-022-01407-y
- Yan Xu, Yangjie Lu, Chang Tian, Jianqiang Yang. Application of combined remediation in heavy metal polluted soil. IOP Conference Series: Earth and Environmental Science. 2019. Vol. 300. N 032006. DOI: 10.1088/1755-1315/300/3/032006
- Guoxuan Fan, Xitao Liu, Xiaowan Li et al. Mechanochemical treatment with CaO-activated PDS of HCB contaminated soils. Chemosphere. 2020. Vol. 257. N 127207. DOI: 10.1016/j.chemosphere.2020.127207
- Ferreira M.B., Sales Solano A.M., Dos Santos E.V. et al. Coupling of Anodic Oxidation and Soil Remediation Processes: A Review. Materials. 2020. Vol. 13. Iss. 19. N 4309. DOI: 10.3390/ma13194309
- Dos Santos E.V., Sáez C., Cañizares P. et al.Treatment of ex-situ soil-washing fluids polluted with petroleum by anodic oxidation, photolysis, sonolysis and combined approaches. Chemical Engineering Journal. 2017. Vol. 310, p. 581-588. DOI: 10.1016/j.cej.2016.05.015
- Shehata S.M., Badawy R.K., Aboulsoud Y.I.E.Phytoremediation of some heavy metals in contaminated soil. Bulletin of the National Research Centre. 2019. Vol. 43. N 189. DOI: 10.1186/s42269-019-0214-7
- Tripathi S., Kumar Singh V., Srivastava P. et al. Phytoremediation of organic pollutants: current status and future directions. Abatement of Environmental Pollutants. 2020, p. 81-105. DOI: 10.1016/B978-0-12-818095-2.00004-7
- Krasnoperova S. The morphological analysis and resistance of plants recommended for phytoremediation of the oil-polluted soils. Modern High Technologies. Regional Application. 2015. N 4 (44), p. 184-188 (in Russian).
- Mader A.E., Holtman G.A., Welz P.J. Treatment wetlands and phyto-technologies for remediation of winery effluent: Challenges and opportunities. Science of the Total Environment. 2022. Vol. 807. Part 1. N 150544, p. 1-17. DOI: 10.1016/j.scitotenv.2021.150544
- Mamirova A., Baubekova A., Pidlisnyuk V. et al. Phytoremediation of Soil Contaminated by Organochlorine Pesticides and Toxic Trace Elements: Prospects and Limitations of Paulownia tomentosa. Toxics. 2022. Vol. 10. Iss. 8. N 465. DOI: 10.3390/toxics10080465
- Hauptvogl M., Kotrla M., Prčík M. et al.Phytoremediation Potential of Fast-Growing Energy Plants: Challenges and Perspectives – a Review. Polish Journal of Environmental Studies. 2020. Vol. 29. N 1, p. 505-516. DOI: 10.15244/pjoes/101621
- Macci C., Peruzzi E., Doni S., Masciandaro G. Monitoring of a long term phytoremediation process of a soil contaminated by heavy metals and hydrocarbons in Tuscany. Environmental Science and Pollution Research. 2020. Vol. 27, p. 424-437. DOI: 10.1007/s11356-019-06836-x
- Alekseenko V.A., Shvydkaya N.V., Alekseenko A.V. et al. Element Accumulation Patterns of Native Plant Species under the Natural Geochemical Stress. Plants. 2021. Vol. 10. Iss. 1. N 33. DOI: 10.3390/plants10010033
- Prabakaran K., Li Jian, Anandkumar A. et al.Managing environmental contamination through phytoremediation by invasive plants: A review. Ecological Engineering. 2019. Vol. 138, p. 28-37. DOI: 10.1016/j.ecoleng.2019.07.002
- Bajagain R., Lee S., Jeong S.-W. Application of persulfate-oxidation foam spraying as a bioremediation pretreatment for diesel oil-contaminated soil. Chemosphere. 2018. Vol. 207, p. 565-572. DOI: 10.1016/j.chemosphere.2018.05.081
- Kafle A., Timilsina A., Gautam A. et al. Phytoremediation: Mechanisms, plant selection and enhancement by natural and synthetic agents. Environmental Advances. 2022. Vol. 8. N 100203. DOI: 10.1016/j.envadv.2022.100203
- Bykova M.V., Alekseenko A.V., Pashkevich M.A., Drebenstedt C. Thermal desorption treatment of petroleum hydrocarbon-contaminated soils of tundra, taiga, and forest steppe landscapes. Environmental Geochemistry and Health. 2021. Vol. 43, p. 2331-2346. DOI: 10.1007/s10653-020-00802-0
- Strizhenok A.V., Korelskiy D.S., Choi Y. Assessment of the Efficiency of Using Organic Waste from the Brewing Industry for Bioremediation of Oil-Contaminated Soils. Journal of Ecological Engineering. 2021. Vol. 22. Iss. 4, p. 66-77. DOI: 10.12911/22998993/133966
- Rada E.C., Andreottola G., Istrate I.A. et al. Remediation of Soil Polluted by Organic Compounds Through Chemical Oxidation and Phytoremediation Combined with DCT. International Journal of Environmental Research and Public Health. 2019. Vol. 16. Iss. 17. N 3179. DOI: 10.3390/ijerph16173179
- Chirakkara R.A., Reddy K.R., Cameselle C. Electrokinetic Amendment in Phytoremediation of Mixed Contaminated Soil. Electrochimica Acta. 2015. Vol. 181, p. 179-191. DOI: 10.1016/j.electacta.2015.01.025
- 25. Cameselle C., Gouveia S. Phytoremediation of mixed contaminated soil enhanced with electric current. Journal of Hazardous Materials. 2019. Vol. 361, p. 95-102. DOI: 10.1016/j.jhazmat.2018.08.062
- Crognale S., Cocarta D.M., Streche C., D’Annibale A. Development of laboratory-scale sequential electrokinetic and biological treatment of chronically hydrocarbon-impacted soils. New Biotechnology. 2020. Vol. 58, p. 38-44. DOI: 10.1016/j.nbt.2020.04.002
- Rada E.C., Istrate I.A., Ragazzi M. et al.Analysis of Electro-Oxidation Suitability for Landfill Leachate Treatment through an Experimental Study. Sewage and Landfill Leachate. Assessment and Remediation of Environmental Hazards. 2016, p. 149-172. DOI: 10.1201/b20005-13
- Zhou W., Rajic L., Chen L. et al. Activated carbon as effective cathode material in iron-free Electro-Fenton process: Integrated H2O2 electrogeneration, activation, and pollutants adsorption. Electrochimica Acta. 2019. Vol. 296, p. 317-326. DOI: 10.1016/j.electacta.2018.11.052
- Ganiyu S.O., Gamal El-Din M. Insight into in-situ radical and non-radical oxidative degradation of organic compounds in complex real matrix during electrooxidation with boron doped diamond electrode: A case study of oil sands process water treatment. Applied Catalysis B: Environmental. 2020. Vol. 279. N 119366. DOI: 10.1016/j.apcatb.2020.119366
- Lawrence M.Z., Kenneth J.W., Pamukcu S. Case study: Electrochemical Geo-Oxidation (ECGO) treatment of Massachusetts New Bedford Harbor sediment PCBs. Electrochimica Acta. 2020. Vol. 354. N 136690. DOI: 10.1016/j.electacta.2020.136690
- Vocciante M., Dovì V.G., Ferro S. Sustainability in ElectroKinetic Remediation Processes: A Critical Analysis. Sustainability. 2021. Vol. 13. Iss. 2. N 770. DOI: 10.3390/su13020770
- Shabanov E.A., Prostov S.M., Gerasimov O.V. In vitro studies of electrochemical purification of foundation soil from oil products. Journal of Construction and Architecture. 2019. Vol. 21. N 4, p. 168-180. DOI: 10.31675/1607-1859-2019-21-4-168-180
- Ganiyu S.O., Martínez-Huitle C.A., Rodrigo M.A. Renewable energies driven electrochemical wastewater/soil decontamination technologies: A critical review of fundamental concepts and applications. Applied Catalysis B: Environmental. 2020. Vol. 270. N 118857. DOI: 10.1016/j.apcatb.2020.118857
- Gingine V., Shah R., Venkata Koteswara Rao P., Hari Krishna P. A review on study of Electrokinetic stabilization of Expansive soil. International Journal of Earth Sciences and Engineering. 2013. Vol. 6. N 2 (1), p. 176-181. DOI: 10.13140/2.1.2809.4086
- Streche C., Cocârţă D.M., Istrate I.A., Badea A.A. Decontamination of Petroleum-Contaminated Soils Using The Electrochemical Technique: Remediation Degree and Energy Consumption. Scientific Reports. 2018. Vol. 8. N 3272. DOI: 10.1038/s41598-018-21606-4
- Shulaev N.S., Pryanichnikova V.V., Kadyrov R.R., Bykovsky N.A. The phytoremediation of oil-contaminated soil. Butlerov Communications. 2016. Vol. 47. N 8, p. 133-138.
- Shulaev N.S., Meshalkin V.P., Pryanichnikova V.V. et al. Electrochemical Cleaning of Oil-Contaminated Soils, Taking into Account the Terrain. Ecology and Industry of Russia. 2022. Vol. 26. Iss. 2, p. 9-13 (in Russian). DOI: 10.18412/1816-0395-2022-2-9-13
- Shulaev N.S., Pryanichnikova V.V., Kadyrov R.R. Regularities of electrochemical cleaning of oil-contaminated soils. Journal of Mining Institute. 2021. Vol. 252, p. 937-946. DOI: 10.31897/PMI.2021.6.15
- Meshalkin V.P., Shulaev N.S., Chelnokov V.V. et al. Determination of electrical parameters for the electrochemical treatment of soils contaminated with oil. IOP Conference Series: Materials Science and Engineering. 2019. Vol. 537. N 062069 (in Russian). DOI: 10.1088/1757-899X/537/6/062069
- Fahid M., Arslan M., Shabir G. et al. Phragmites australisin combination with hydrocarbons degrading bacteria is a suitable option for remediation of diesel-contaminated water in floating wetlands. Chemosphere. 2020. Vol. 240. N 124890. DOI: 10.1016/j.chemosphere.2019.124890
- Shaikhiev I.G., Sverguzova S.V., Galimova R.Z., Grechina A.S.Using wastes of buckwheat processing as sorption materials for the removal of pollutants from aqueous media: a review. IOP Conference Series: Materials Science and Engineering. 2020. Vol. 945. N 012044. DOI: 10.1088/1757-899X/945/1/012044