Modeling the efficiency of seasonal cooling devices when changing the statistical distribution of weather conditions
- 1 — Ph.D., Dr.Sci. Deputy Chief Engineer for Science OOO Gazprom Dobycha Nadym, Nadym
- 2 — General Director OOO Gazprom Dobycha Noyabrsk ▪ Orcid
- 3 — Ph.D., Dr.Sci. Head of the Department Gubkin Russian State University of Oil and Gas ▪ Orcid
- 4 — Ph.D. Associate Professor Gubkin Russian State University of Oil and Gas ▪ Orcid
Abstract
The climatic factor of warming, which is evidenced by a significant number of scientists and research teams in Russia and the world, has a significant impact on the cryogenic state of permafrost soil, the preservation of which is one of the most common principles of construction in the North. The influence of projected climatic changes in planning up to 2050 on the efficiency of seasonal cooling devices, the principle of operation of which is based on seasonal soil freezing, is considered. The conducted modeling has shown that in a situation of stable climate, the preservation of the cryogenic state of permafrost soil is realized without the use of additional measures. With warming with a trend of 0.1 °C per year, seasonal cooling devices do not ensure the preservation of the current level of the cryogenic state of the soil and additional measures are required to increase their efficiency in the summer. In the case of more extreme warming with a rate of 0.25 °C per year, the modeling results show that seasonal cooling devices do not significantly affect the rate of soil thawing and the solution to the problem should be its complete replacement with systems with year-round action.
Introduction
Promising areas for the development of the oil and gas industry in the Russian Federation tend to shift to the north and east. The development of the Yamal Gas production Center, the fields of the Gydan Peninsula, Yakutia and the Far East poses to oil and gas companies the most important task of high-quality and reliable operation of oil and gas sector facilities in conditions of continuous permafrost soil (PS).
The integrated development of deposits in the Arctic region of Russia involves the search for new methods of field exploration that would reduce the anthropogenic impact on PS by reducing the proportion of field stages of work [1, 2], new methods of field development with a reduction in thermal effects on frozen soil during drilling [3] or during construction and installation work [4], as well as new methods and technologies to ensure the reliability of the operation of fishing facilities in order to reduce the impact of emissions and leaks of extracted and transported products [5]. During the construction of natural gas production and transportation facilities in areas of continuous distribution of PS, their use is carried out according to the first principle – the preservation of permafrost during both construction [6, 7] and operation [8, 9]. Violation of the operating conditions of the soil in a cryogenic state can lead to catastrophic consequences of a natural and man-made nature [10, 11], which cause significant damage to the fragile ecosystem of the northern regions. The analysis of scientific works on Arctic exploration methods shows that at each stage of the life cycle of an industrial facility, the task of reducing the thermal impact on frozen soil is solved both through the use of new structures of grounds and foundations [4, 7] and optimization of technological processes [1, 3].
A typical technical solution for the implementation of this principle is the use of various designs of seasonal cooling devices (SCD). A review of such systems was carried out in [12], and in the article [13] it is shown how soil thermal stabilization systems during construction in the cryolithozone allow maintaining the required negative base temperature or restoring degraded permafrost. The efficiency of SCD operation directly depends on the climatic conditions of the environment, and stationary climate models based on statistical data of the climate of the construction site are usually used in their design [14].
According to estimates by a significant number of scientists [15, 16] and international climate organizations [17], active climate warming processes are underway in Russia, especially pronounced in the northern latitudes [18, 19] of the Russian North. It is shown [16] that over the past 20 years, the Yamal territory has undergone an abnormal decrease in average annual temperatures, as well as the number of days per year with low temperatures. Reports [17] of the well-known climate group Intergovernmental Panel on Climate Change record annual warming in the range from 0.03 to 0.1 °C, and data from Roshydromet [18] for the North of Russia demonstrate warming dynamics above 0.1 °C. Such climatic changes can cause significant damage to the cryogenic state of the soil and lead to its thawing, which will lead to a significant decrease in the bearing capacity of pile grounds and foundations [20, 21].
In the works [22, 23] devoted to the state of SCD, the issues of damage and destruction of these structures during construction and operation or issues of SCD efficiency depending on various anthropogenic and technogenic factors are considered [24]. The problems of the stability of engineering structures under climate warming have been studied [25, 26], some issues of modeling the operation of SCD [27, 28] or assessing the stability of piles under thermal influence [29], but the problem of reducing the efficiency of existing SCDs due to global climate warming is not considered.
The purpose of this study was to evaluate the behavior of SCD in various climate models based on the current understanding of climate change in Russia and the world. The object of the study was the frozen ground – climate – SCD system, and the subject of the study was the stability of this system to climate change.
Methods
The simulation of the joint work of frozen soil and SCD in various climatic conditions using the Borey 3D software package has been performed. The Borey 3D complex
is registered with Rospatent, N 2018660189 dated 08/17/2018. Certified and meets the requirements SP 25.13330.2012 (SNiP 2.02.04-88), RSN 67-87, STO Gazprom 2-2.1-435-2010, STO Gazprom 2-2.1-390-2009, GOST R ISO/m EXTRAVK 12119-2000, GOST R ISO 9127-94, GOST R ISO 9126-93, GOST R ISO 28806-90. Implements a mathematical apparatus for numerical simulation of the propagation of temperature fields in a soil mass, taking into account the phase transition of unfrozen water in the spectrum of negative temperatures. The software package has found practical application and was used by specialists in the framework of calculations of the use of SCD on PS [30, 31].
The main objective of the study is to assess the stability of PS to warming processes, to assess the rate of permafrost degradation in the presence of SCD, absence of SCD, as well as the application of methods to increase the effectiveness of SCD for the period from 2011 to 2051 in relation to the PS site, the parameters of which correspond to the typical geocryological picture of the Yamal Peninsula. The study included options for a stable climate, annual warming of 0.1 and 0.25 °C. At the same time, the possibility of changing the geocryological picture of PS sites in their natural state (without the use of SCD), with the use of SCD and the option of ensuring the operation of SCD in the summer was considered. The calculation model of the PS site is adopted in the form of a cube measuring 50×50×50 m, with specified soil conditions. As soils, the model uses plastically frozen loams typical of the Yamal Peninsula with the following parameters: density 1750-1840 kg/m3; total humidity 0.124-0.181 units; plasticity number 0.114; degree of salinity 0.065-0.081 %; freezing point –0.48 °C; without peat inclusions. A SCD with a depth of 10.5 m is installed in the center of the soil mass. A thermal well 1 is modeled in 0.5 m along the Y-axis, a thermal well 2 in 1 m, and a thermal well 3 in 2 m. The model cube displays the area that will be sampled to perform the calculation. A boundary condition of the third kind is assumed on the upper face of the model cube. The values for the calculation are presented in terms of wind speed, thickness and density of snow cover. The boundary conditions of zero heat flow are assumed on the side and bottom faces of the model cube. To solve the differential equation of heat propagation in the soil with phase transitions in the negative temperature spectrum, a method with a dynamuic division of the region into subdomains with an explicit and implicit computational scheme is used. A simple iteration method is used as an implicit computational scheme. To improve the convergence of the implicit method, the regularization method and dynamic time step selection are used. The website of the Borey 3D software package shows the results of positive verification of the applied computational models for problems with analytical solutions. Modeling of SCD operation is performed by setting boundary conditions of the second kind in the form of internal heat sources/sinks.
The prediction of the processes of PS state dynamics was carried out based on extrapolation of data from the Marresal climate station [32, 33]. Climatic indicators for a stable climate were obtained by processing statistical data (the average temperature of a five-day period and the average thickness of snow cover) for 2011-2021, which were repeated until 2051. The prediction of the parameters of the dynamics of climate data changes for the case of warming by 0.1 and 0.25 °C was carried out by increasing the temperature of a stable climate annually by the corresponding value. The 0.1 °C warming interval was adopted on the basis of the authors' approximation of real climate data from 1990-2020 [16], as well as estimates of the warming rate in the northern regions of Russia according to Roshydromet data for 1976-2016 [34], the 0.25 °C interval is based on the worst-case scenario of climate change in the northern territories of Russia by 10-12 °C at the end of 21 century according to the report [18]. The modeling period was adopted based on the calculation methodology of the Intergovernmental Panel on Climate Change (IPCC) [17].
Based on the wind speed range of 5-7 m/s typical for the Yamal Peninsula, the average wind speed of 6 m/s is assumed in the calculations. Data on the operating mode and characteristics of SCD TK32/12.M5-03 (FSA) coolant R-744 (carbon dioxide) presented in PC Borey 3D were used for modeling. A separate climate data model for the SCD capacitor has been developed for the option of switching the SCD operating mode in the summer. In this model, in the period from May 15 to October 15, the SCD capacitor is in a temperature field of –5 °C, and during the rest of the year, the climatic data of the SCD capacitor correspond to the climatic parameters of the temperature regime of the climate variant that is being modeled. Additionally, based on the analysis of climate dynamics with a warming trend of 0.25 °C, due to a significant increase in the summer period (the temperature is consistently above 0 °C) it was decided to consider the following mode of operation: from 01.01.2024, the mode is set from 01.05 to 31.10, and starting from 01.01.2025 – from 01.04 to 31.10.
Results
Under the condition of a stable climate, the simulation showed the preservation of the cryogenic state of the soil regardless of the use of SCD. Figure 1 shows the obtained data on the cryogenic state of the soil for 2050 in the absence of SCD (Fig.1, a), the presence of SCD for well 1 (Fig.1, b), the presence of SCD and the option of transferring it to work in the warm season for well 1 (Fig.1, c). Figure 2 shows the average annual data on the cryogenic state of the soil in a stable climate. In the absence of SCD, the depth of seasonal soil thawing by 2050 will be 1.6 m, which corresponds to the average level of thawing depth of the Yamal Peninsula today. In the presence of SCD, the depth of soil thawing will also be 1.6 m. When used in the variant of transferring SCD to work in the warm season, the depth of seasonal warming will decrease to 1.4 m. In the absence of SCD, the depth of the soil, below which the temperature does not exceed –1 °C, will be 3.5 m, in the presence of SCD – 2.5 m, and when used in the translation version SCD for work in the warmer months is 2.25 m. Analysis of these temperature fields showed that the presence of SCD lowers the temperature of frozen soil by 1 °C at a distance of 0.5 m from SCD, 0.8 °C at a distance of 1 m and 0.5 °C at a distance of 2 m. The use of SCD in the option of switching to work in the warm season will lower the average annual temperature of frozen soil in the depth range from 0.8 to 8 m by 0.5 °C at a distance of 0.5 m from SCD, 0.1 °C at a distance of 1 m from SCD and 0.07 °C at a distance of 2 m from SCD. For a stable climate, these changes are not critical, since the cryogenic state of the soil, sufficient for the effective functioning of the base, is preserved when using only SCD. However, even in this case, when used in the variant of transferring SCD to work in the warm season, the efficiency of its application increases by 15-20 %, depending on the distance from the SCD axis.

Fig.1. The state of frozen soil for a stable climate model for 2050

Fig.2. Forecast of the average annual soil temperature for 2050 with a stable climate: a – thermal well 1; b – thermal well 2; c – thermal well 3
1 – without SCD; 2 – in the presence of SCD; 3 – in the presence of SCD and its transfer to work in the warm season

Fig.3. The state of frozen soil for the 0.1 °C warming model for 2050

Fig.4. Forecast of the average annual ground temperature for 2050 with a warming of 0.1 °C:
a – thermal well 1; b – thermal well 2; c – thermal well 3
See the legend in Fig.2
Figure 3 shows data on the cryogenic state of the soil for 2050 in the absence of SCD (Fig.3, a), the presence of SCD for well 1 (Fig.3, b), and the transfer of SCD to work in the warm season (Fig.3, c). Figure 4 shows the average annual data on the cryogenic state of the soil with a warming climate of 0.1 °C. In the absence of SCD, the depth of seasonal soil warming by 2050 it will be 5 m, which is significantly higher than the average depth of warming of the Yamal Peninsula at the moment (1.5-2.0 m). In the presence of SCD, the depth of soil thawing will be 4 m, when used in the variant of transferring SCD to work in the warm season, the depth of soil thawing will decrease to 3 m. In the absence of SCD, the depth of the soil, below which the temperature does not exceed –1 °C, will be 7 m by 2050, and in the presence of SCD – 5.5 m. When used in the variant of transferring SCD to work in the warm season, the permafrost layer with a temperature below –1 °C begins from a depth of 4 m, which practically corresponds to the natural state of the permafrost (3.5 m).
Analysis of these temperature fields showed that the presence of SCD lowers the temperature of frozen soil by 1.5 °C at a distance of 0.5 m from SCD, 1 °C at a distance of 1 m and 0.6 °C at a distance of 2 m. Using SCD in the option of switching to work in the warm season will lower the average annual temperature of frozen soil in the depth range from 0.8 to 8 m by 1.4 °C at a distance of 0.5 m from SCD, 1 °C at a distance of 1 m from SCD and 0.5 °C at a distance of 2 m from SCD. In such a climate model, the use of SCD in the variant of transferring to work in the warm season significantly affects the state of permafrost, increasing the efficiency of SCD application by 34-40 %. To estimate the rate of soil thawing, calculations and analysis of average annual temperatures for 2020-2050 were carried out. The average annual ground depth temperature in the model without SCD decreases by 1 °C from every 10 years and by 2050 it will be less than –1 °C, maintained from a depth of about 7 m. The presence of SCD reduced the rate of thawing by about 1 °C, and when transferring SCD to work in the warm season, it additionally lowered the temperature by 0.5 °C.
Figure 5 shows the temperature fields of the cryogenic state of the model soil with a warming of 0.1 °C. In the conditions of annual warming of 0.25 °C, modeling shows the degradation of the cryogenic state of the soil to a significant depth and large-scale thawing both in the absence of SCD and in its presence. The option of transferring SCD to work in the warm season does not have a significant positive effect for stabilizing the cryogenic state of the soil.
Data on the cryogenic state of the soil for 2050 are presented in the absence of SCD (Fig.6, a), the presence of SCD for well 1 (Fig.6, b) and the use of SCD in the option of transferring to work in the warm season (Fig.6, c). Figure 7 shows the average annual data on the cryogenic state of the soil with a climate warming of 0.25 °C. In the absence of SCD, the depth of seasonal soil thawing by 2050 will be 9 m, which is significantly higher than the average depth of thawing in the Yamal Peninsula now (1.5-2.0 m). In the presence of SCD, the depth of soil thawing will be 8 m. In the case of transferring SCD to work in the warm season, the depth of soil thawing will decrease to 6 m. In the absence of SCD, the depth of the soil, below which the temperature does not exceed –1 °C, by 2050 it will be 11 m, and in the presence of SCD – 10 m. The option of transferring SCD to work in the warm season did not affect this characteristic of the cryogenic state of the soil.
Data analysis of temperature fields shows that the presence of SCD will reduce the soil temperature by 0.5 °C at a distance of 0.5 m from SCD, 0.25 °C at a distance of 1 m, and 0.15 °C at a distance of 2 m. The option of switching SCD to work in the warm season will lower the soil temperature in the depth range from 0.8 to 8 m by 0.69 °C at a distance of 0.5 m from SCD, 0.44 °C at a distance of 1 m from SCD, and 0.23 °C at a distance of 2 m from SCD. At a warming rate of 0.25 °C, the presence of SCD did not actually have a significant effect on the cryogenic state of the soil. In this model, the option of transferring SCD to work in the warmer months has almost doubled the efficiency of using SCD. Nevertheless, this is not enough to prevent large-scale thawing of the soil and preserve the permafrost condition of the soils.
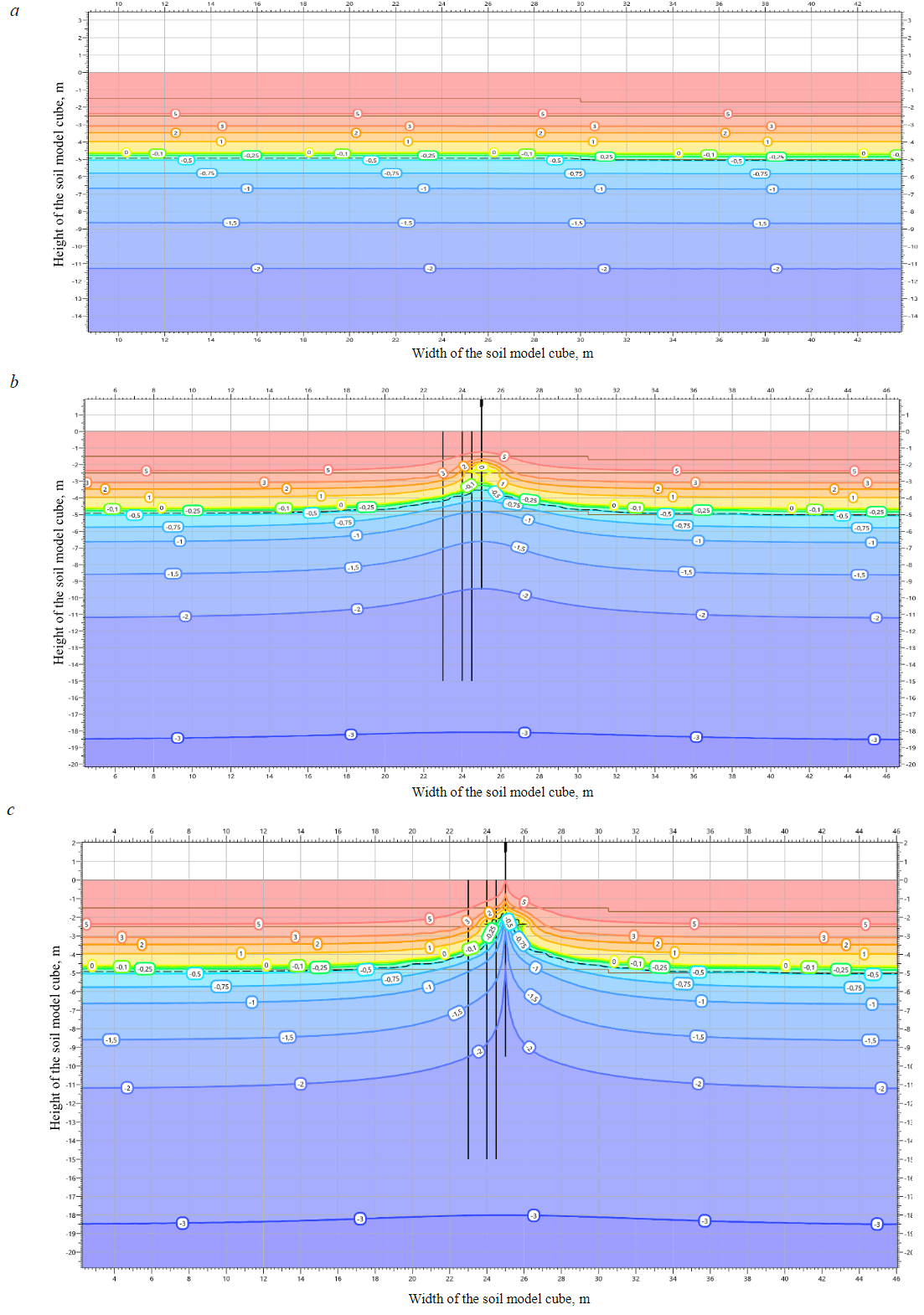
Fig.5. The temperature field of the model on 09.15.2050
The X-axis is indicated horizontally, the Z-axis is indicated vertically: a – in the absence of SCD; b – in the presence of SCD; c – when used in the variant of transferring SCD to work in the warm season

Fig.6. The state of the frozen ground for the 0.25 °C warming model for 2050

Fig.7. Forecast of the average annual ground temperature for 2050 with a warming of 0.25 °C:
a – thermal well 1; b – thermal well 2; c – thermal well 3
See the legend in Fig.2
To estimate the rate of soil thawing, calculations and estimates of average annual temperatures for 2020-2050 were carried out. The presence of SCD reduced the intensity of soil thawing by an average of 0.5-1 °C, which makes it possible in practice to operate the soil mass in a frozen state for longer. The rate of warming of 0.25 °C per year for 10 years by 2030 will lead to the fact that in the presence of SCD, the depth of seasonal thawing will increase from 1.4 to 2 m, and the soil mass with a constant temperature below –1 °C will start from a depth of 3.5 m instead of 2.75.
Conclusion
The computational models proposed in the work made it possible to assess the stability of PS to warming processes and to estimate the rate of permafrost degradation for three climate variants: in the presence of SCD, in the absence of SCD, as well as the use of methods to increase the effectiveness of SCD. The modeling results showed that the efficiency of SCD decreases at any values of climate warming. The model with a warming trend of 0.1 °C per year demonstrates a large-scale thawing of PS over 30 years of operation, which will have an extremely negative impact on the bearing capacity of any pile foundations. The presence of SCD slows down the thawing trend, allows to almost halving the scale of soil warming, lower the temperature of the PS mass by 1-1.5 °C, but does not stop this process. If it is possible to transfer the device to work in the summer, it will be possible to maintain the level of permafrost without significant degradation.
A model with a warming trend of 0.25 °C per year (to assess the worst-case scenario) demonstrates catastrophic thawing of PS by 2050, SCD slightly slows down such thawing, allowing to lower the temperature of the PS mass by 0.3-0.8 °C, but does not stop this process. By 2050, in such a catastrophic model, despite the use of SCD, the depth of seasonal soil thawing will increase to 8 m at a normal depth of 1.5 m. The possibility of switching the device to work in the summer period will increase the efficiency of SCD, but not stop the process of soil thawing.
Forced year-round cooling systems built on various industrial refrigerating machines will be an effective solution to save energy in a scenario with a warming of 0.25 °C per year. However, using them to modernize the existing SCD infrastructure is practically impossible due to the significant number of individually installed SCDs and the large area of their installation.
The paper models the SCD operating mode, taking into account the option of transferring to work in the warm season. The modeling results showed that such a solution could increase the efficiency of SCD by 35-40 % and prevent soil thawing with the most likely forecast of warming by 0.1 °C per year.
References
- Bondar K.M., Minaev V.А., Faddeev A.О. Cost Estimate for Exploration of Oil and Gas Fields in the Arctic Zone. IOP Conference Series: Materials Science and Engineering. 2021. Vol. 1079. N 062069. DOI: 10.1088/1757-899X/1079/6/062069
- Batin E.N., Dedov K.V., Kochegarova N.A. Arctic: the main directions of exploration and development, role in the oil and gas industry, transport system. Science Time. 2015. N 12 (24), p. 47-51.
- Eppelbaum L.V., Kutasov I.M. Well drilling in permafrost regions: dynamics of the thawed zone. Polar Research. 2019. Vol. 38, p. 1-9. DOI: 10.33265/polar.v38.3351
- Litvinenko V.S., Dvoynikov M.V., Trushko V.L. Elaboration of a conceptual solution for the development of the Artic shelf from seasonally flooded coastal areas. International Journal of Mining Science and Technology. 2021. Vol. 32. Iss. 1, p. 113-119. DOI: 10.1016/j.ijmst.2021.09.010
- Grebnev Y., Moskalev A. Modelling the accidental oil spills at potentially hazardous facilities in the Arctic zone of Krasnoyarsk Krai. IOP Conference Series: Earth and Environmental Science. 2021. Vol. 816. N 012007. DOI: 10.1088/1755-1315/816/1/012007
- Gilev N.G., Zenkov E.V., Poverenniy U.S. et al. Optimization of capital costs for pile foundations during construction of oil and gas production facilities on permafrost soil. Oil Industry. 2019. N 1, p. 46-49 (in Russian). DOI: 10.24887/0028-2448-2019-11-46-49
- Rzaev Ch.T. Choosing the type of foundation structures on permafrost soils. Nauka segodnya: globalnye vyzovy i mekhanizmy razvitiya: Materialy mezhdunarodnoi nauchno-prakticheskoi konferentsii: v 2 chastyakh, 26 aprelya 2017, Vologda, Rossiya. Vologda: OOO “Marker”, 2017. Part 1, p. 25-27 (in Russian).
- 8. Shepitko T.V., Lutsky S.Y., Nak G.I., Cherkasov A.M. Technological Features of Construction and Reconstruction of Geotechnical Structures in the Arctic Zone. Designs. 2022. Vol. 6. Iss. 2, p. 1-8. DOI: 10.3390/designs6020034
- Gendler S., Prokhorova E. Risk-Based Methodology for Determining Priority Directions for Improving Occupational Safety in the Mining Industry of the Arctic Zone. Resources. 2021. Vol. 10. Iss. 3, p. 1-14. DOI: 10.3390/resources10030020
- Bogoyavlensky V.I., Bogoyavlenskiy I.V., Kargina T.N. Catastrophic gas blowout in 2020 on the Yamal Peninsula in the Arctic. Results of comprehensive analysis of aerospace RS data. Arctic: Ecology and Economy. 2021. Vol. 11. N 3, p. 362-374 (in Russian). DOI: 10.25283/2223-4594-2021-3-362-374
- Yakushev V.S., Semenov A.P., Bogoyavlensky V.I. et al. Experimental modeling of methane release from intrapermafrost relic gas hydrates when sediment temperature change. Cold Regions Science and Technology. 2018. Vol. 149, p. 46-50. DOI: 10.1016/j.coldregions.2018.02.007
- Yarmak E. Permafrost Foundations Thermally Stabilized Using Thermosyphons. OTC Arctic Technology Conference, 23-25 March 2015, Copenhagen, Denmark. 2015. N OTC-25500-MS. DOI: 10.4043/25500-MS
- Koloskov G.V., Ibragimov E.V., Gamzaev R.G. On the issue of choosing optimal systems for thermal stabilization of soils during construction in the cryolithozone. Geotekhnika. 2015. N 6, p. 4-11 (in Russian).
- Abrosimov A., Zaletaev S. Soil coolers. Designs and calculation methods. London, United Kingdom: Palmarium Academic Publishing, 2012, p. 392 (in Russian).
- Khrustalev L.N., Emelyanova L.V. Determination of the Temperature Coefficient for Calculation of the Bearing Capacity of Permafrost Beds in a Changing Climate. Soil Mechanics and Foundation Engineering. 2013. Vol. 50. N 1, p. 19-23. DOI: 10.1007/s11204-013-9204-1
- Vasilev G.G., Dzhaljabov A.A., Leonovich I.A. Study of influence of climatic factor on dynamics of permafrost condition of soils beneath objects of oil and gas complex. Proceedings of Gubkin University. 2021. N 3 (304), p. 67-79 (in Russian). DOI: 10.33285/2073-9028-2021-3(304)-67-79
- IPCC, 2022: Climate Change 2022: Impacts, Adaptation, and Vulnerability. Contribution of Working Group II to the Sixth Assessment Report of the Intergovernmental Panel on Climate Change. Еd. by H.-O.Pörtner, D.C.Roberts, M.Tignor. Cambridge, UK and New York, NY, USA: Cambridge University Press, 2022, p. 3056. DOI: 10.1017/9781009325844
- Report on climate risks in the territory of the Russian Federation. Ed. bu V.M.Kattsova. St. Petersburg: Glavnaya geofizicheskaya observatoriya im. A.I.Voeikova, 2017, p. 106 (in Russian).
- Anisimov O., Kokorev V. Cities of the Russian North in the Context of Climate Change. Sustaining Russia's Arctic Cities: Resource Politics, Migration, and Climate Change. 2016. Vol. 2, p. 141-174. DOI: 10.2307/j.ctvswx6s0.12
- Nikiforova N.S., Konnov A.V. The bearing capacity of piles in permafrost soils under climate change. Construction and Geotechnics. 2021. Vol. 12. N 3, p. 14-24 (in Russian). DOI: 10.15593/2224-9826/2021.3.02
- Nikiforova N.S., Konnov A.B. Influence of permafrost degradation on piles bearing capacity. Journal of Physics: Conference Series. Deep Foundations and geotechnical problems of territories, 26-28 May 2021, Perm, Russian Federation. 2021. Vol. 1928. N 012046. DOI: 10.1088/1742-6596/1928/1/012046
- Strizhkov S.N. On the issue of the quality of operation of seasonally operating cooling devices. Geoinfo: nezavisimyi elektronnyi zhurnal. 2017. URL: http://www.geoinfo.ru/product/strizhkov-sergej-nikolaevich/k-voprosu-o-kachestve-raboty-sezonno-dejstvuyushchih-ohlazhdayushchih-ustrojstv-35150.shtml (accessed 15.08.2022) (in Russian).
- Presnov O.M., Melikhov V.P., Zaytsev S.A., Slivina D.M. Сonstruction of piles in permafrost conditions. International Research Journal. 2022. N 2 (116). Part 1, p. 41-43 (in Russian). DOI: 10.23670/IRJ.2022.116.2.006
- Vasiliev G.G., Dzhalyabov A.A., Leonovich I.A. Study of the permafrost temperature mode of oil and gas objects foundation equipped by seasonally operating cooling devices. Equipment and technologies for oil and gas complex. 2021. N 4 (124), p. 75-80. DOI: 10.33285/1999-6934-2021-4(124)-75-80
- Zhang A.A., Ashpiz E.S., Khrustalev L.N., Shesternev D.M. New way for thermal stabilization of permafrost under railway embankment. Earth’s cryosphere. 2018. Vol. 22. N 3, p. 67-71 (in Russian). DOI: 10.21782/KZ1560-7496-2018-3(67-71)
- Vasiliev G.G., Dzhaljabov А.А., Leonovich I.А. Analysis of the causes of engineering structures deformations at gas industry facilities in the permafrost zone. Journal of Mining Institute. 2021. Vol. 249, p. 377-385. DOI: 10.31897/PMI.2021.3.6
- Loli M., Tsatsis A., Kourkoulis R., Anastasopoulos I. A simplified numerical method to simulate the thawing of frozen soil. Proceedings of the Institution of Civil Engineers – Geotechnical Engineering. 2019. Vol. 173, p. 1-49. DOI: 10.1680/jgeen.18.00239
- Weismüller J., Wollschläger U., Boike J. et al. Modeling the thermal dynamics of the active layer at two contrasting permafrost sites on Svalbard and on the Tibetan Plateau. The Cryosphere. 2011. Vol. 5. Iss. 3, p. 741-757. DOI: 10.5194/tc-5-741-2011
- Buslaev G., Tsvetkov P., Lavrik A. et al. Ensuring the Sustainability of Arctic Industrial Facilities under Conditions of Global Climate Change. Resources. 2021. Vol. 10. N 12, p. 1-15. DOI: 10.3390/resources10120128
- Ryazanov A.V., Usachev A.A. Features of calculating the temperature regime of permafrost soils of the foundations of bored piles of bridge crossings. Fundamenty. 2021. N 4 (6), p. 49-51 (in Russian).
- Ryazanov A.V. Features of the design of foundations of structures on permafrost soils in a warming climate. Fundamenty. 2021. N 1 (3), p. 10-13 (in Russian).
- Bulygina O.N., Razuvaev V.N., Trofimenko L.T., Shvets N.V. Certificate of state registration of the database N 2014621485. Description of the data set of the average monthly air temperature at Russian stations. URL: http://meteo.ru/data/156-temperature#описание-массива-данных (accessed 06.09.2022) (in Russian).
- Bulygina O.N., Razuvaev V.N., Aleksandrova T.M. Certificate of state registration of the database N 2014621201. Description of the data set of snow cover characteristics at meteorological stations in Russia and the former USSR. URL: http://meteo.ru/data/165-snow-cover#описание-массива-данных (accessed 06.09.2022) (in Russian).
- Gruza G.V., Bardin M.Yu., Platova T.V. et al. The report on the peculiarities of the climate in the territory of the Russian Federation for 2016. Moscow: Rosgidromet, 2017, p. 70 (in Russian).