Increasing the quality of zeolite-bearing rocks from Eastern Transbaikalia by applying directed energy
- 1 — Ph.D., Dr.Sci. Vice-Rector Transbaikal State University, Chita ▪ Orcid
- 2 — Ph.D., Dr.Sci. Vice-Rector Transbaikal State University ▪ Orcid
- 3 — Ph.D., Dr.Sci. Professor Transbaikal State University ▪ Orcid
Abstract
This paper presents the use of accelerated electrons to treat zeolite-bearing rocks from Eastern Transbaikalia to increase the efficiency of separating zeolites from rock-forming minerals via electromagnetic separation. The effectiveness of the liberation of zeolite minerals using accelerated electrons was analyzed. The results of dry electromagnetic separation of zeolite-bearing rocks are presented. The dependence of the extraction of iron-bearing minerals from zeolite-bearing rocks by electromagnetic separation on the magnetic field intensity for different particle sizes has been established. The main methods of zeolite-bearing rock enrichment and ore preparation were determined. A technological scheme for processing zeolite-bearing rocks, based on the use of accelerated electron treatment at the ore preparation stage, is presented, significantly improving the zeolite production quality.
Introduction
The intense development of the mining industry is accompanied by technologies that generate a considerable amount of industrial waste that is not subject to disposal and processing [1, 2]. The accumulation of substantial volumes of waste rock and beneficiation tailings leads to serious economic and environmental problems in the areas where they are located [3, 4]. Most waste rock dumps and tailings storage facilities, which are a legacy of the 1990s and 2000s, lack responsible management, and combined with newly generated waste from the mining industry, they inflict significant harm on the environment [5, 6].
The solution to the problem of the negative impact of mining and processing waste on the environment is to use effective tools for managing industrial materials [7, 8]. This includes the adoption of best available technologies and materials capable of reducing the contents of toxic and radioactive elements in waste, preventing or significantly reducing dust and gas emissions from them and restoring land disturbed by anthropogenic activities while adhering to principles of conserving mi-neral resources, rationality, and complexity in their utilization [9, 10].
One of the most versatile tools in implementing environmental protection measures is sorbents, which possess the required properties, primarily adsorption properties [11, 12]. Among naturally occurring sorbents, zeolite-bearing rocks (ZCR) are highly effective, widely available, and cost-effective [13, 14]. The unique sorption and ion exchange properties of natural zeolites can be effectively utilized in waste management systems of mining production [15].
Currently, ZCR are primarily used in backfilling mined-out spaces (mines and quarries) in combination with waste rock and tailings from mineral resource storage and in road construction for technical purposes [14, 15]. Considering their unique properties, the potential of natural zeolites is in the identification of effective approaches for their application in waste management systems of mining production, significantly minimizing and preventing the negative impact of anthropogenic formations on the environment [16, 17]. These tasks include the purification of exhaust gases, se-wage, and circulating water, land reclamation, the preservation of tailings and waste rock, and the burial of toxic and radioactive waste [18, 19].
The main limitation of ZCR application is the relatively low quality of mineral resources (zeolite mineral content ranging from 35 to 60 %), despite significant reserves. Additionally, industry requirements for the quality of natural zeolites, including the adherence to international standards owing to Russia's membership in various trade organizations, stipulate that impurities in zeolite products cannot exceed 1-3 % [20, 21]. The vast majority of Russia's ZCR reserves consist of medium- and low-quality common rocks containing a high percentage of rock-forming minerals (mainly quartz, feldspars, and iron oxides), limiting the widespread use of this raw material [22, 23]. This necessitates the development of efficient beneficiation and modification technologies for ZCR to obtain zeolite products. On the one hand, these products will allow us to rationally solve the complex issue of comprehensive utilization, disposal, neutralization, and processing of mining and production waste. On the other hand, they will allow the creation of technologies used to control the physicochemical properties of natural zeolites to produce high-quality products [24, 25].
Previous studies [20, 26] have shown that utilizing accelerated electrons for pretreating raw mineral materials is an effective approach to modify their physicochemical and mechanical properties selectively. This alteration results in reduced ore strength characteristics, increased selective disintegration, and a considerable reduction in the duration of subsequent ore preparation operations, such as milling. The impact of accelerated electrons promotes the selective liberation of mineral intergrowths and enhances the efficiency of subsequent ore enrichment [20, 27].
The enrichment of ZCR depends on the similarities and differences in the technological properties of zeolites and rock-forming minerals, their textures and structures, and the mineral forms of iron and silica in rocks as isomorphic inclusions and native minerals [20, 28]. The necessity of using accelerated electron exposure and determining the optimal radiation treatment parameters lies in achieving selective modification of the properties of Eastern Transbaikalia ZCR. Such alterations will enhance the efficiency of their purification from rock-forming minerals, particularly through magnetic separation, and increase the adsorption capacity of zeolite minerals such as clinoptilolite, mordenite, and chabazite [20, 29].
The methodological and theoretical foundations of research in this field have been laid by well-known domestic scientists [26, 30]. This study aimed to explore the feasibility of applying energy-based treatments to significantly improve the quality of ZCR and obtain zeolite products with high sorption properties.
Methods
Montmorillonite-clinoptilolite-bearing rocks from the Shivyrtuy, clinoptilolite-bearing rocks from the Holinsky, and chabazite-bearing rocks from Talan-Gozagorsk deposits were used as the research objects. The research methodology included exposing the ZCR to accelerated electrons using an ILU electron accelerator developed at the Institute of Nuclear Physics of the Siberian Branch of the Russian Academy of Sciences.
The ZCR samples were fed through a dosing hopper and conveyor into the accelerator, where they were exposed to a beam of accelerated electrons. The irradiation dose ranged from 2 to 5 kGy. Mössbauer spectroscopy measurements were performed using an Ms-1104Em spectrometer. The particle size of the studied rocks in the samples was 0.05-0.07 mm, and the mass of each sample was 50-70 mg. The data processing of Mössbauer spectra was performed using the Univem MS program. The thermal studies of Eastern Transbaikalia ZCR after accelerated electron treatment were conducted in an argon atmosphere at 20-1400 °C using an STA 449C instrument (Germany). The heat capacity was determined to be 40-400 °C based on the baseline, standard (sapphire), and sample.
The liberation coefficient of zeolite minerals was determined using optical microscopy after milling zeolite minerals in a laboratory ball mill (MSHL, Russia) in two modes: conventional ZCR milling and milling after pretreatment with accelerated electrons. The obtained data were mathema-tically processed, and the corresponding functions and graphs were created. The electromagnetic separation of ZCR was performed using a 138-CE electromagnetic separator and a high-intensity isodynamic field separator SIM-1 (particle size of 0.1 mm). The research involved granulometric, mineralogical, chemical, and X-ray phase analyses, infrared spectroscopy, scanning electron microscopy, and optical-geometric image analysis.
The ZCR were studied using a JEOL-5300 analytical electron microscope with a Link-ISIS energy-dispersive attachment, which allows to determine the chemical composition of the minerals in the ZCR. The mineral composition of the ZCR was determined through quantitative X-ray phase analysis using an ADRON-1 diffractometer. The study of the mineralogical characteristics of the ZCR included determining the mineral composition and identifying the peculiarities of zeolite mineral inclusions and their association with rock-forming minerals. The properties of minerals extracted through directional ore preparation in combination with classical beneficiation methods (gravity, magnetic, and electrical) were also investigated.
Optical microscopy was employed to determine the textural and structural characteristics of Eastern Transbaikalia ZCR. Their phase composition was identified, and the properties and forms of the minerals were studied. An ADP-1.5 diffractometer was used for the X-ray diffraction analysis. The technological assessment of ZCR and the study of the distribution of minerals within them and in the processing products were conducted through image analysis based on the optical-geometric research methodology using the “Video-Master” complex.
Iron-bearing minerals in the Eastern Transbaikalia ZCR were present as mechanical microinclusions and isomorphic impurities; therefore, Mössbauer (nuclear gamma resonance) spectroscopy was employed for this analysis. The sorption characteristics of ZCR were studied at 77 K via the nitrogen adsorption method using an ASAP-2400 instrument (USA).
Discussion
The chemical analysis data of the ZCR from the Eastern Transbaikalia deposits are presented in Table 1. The main components of ZCR were SiO2, Al2O3, Fe2O3, and magnesium, sodium, and potassium oxides. The liberation coefficients of zeolite minerals during the milling of ZCR from the investigated deposits, both without and with radiation exposure, are shown in Fig.1. The rock size after milling is represented by curves 1 and 2, the size of zeolite minerals is shown in curves 3 and 4, and the liberation coefficient was determined as the ratio of the areas under the curves.
The use of low-dose (2-4 kGy) directional electron beam irradiation before milling increased the liberation coefficient of clinoptilolite minerals from the Shivyrtuy deposit from 38 to 54 % and from 46 to 62 % for clinoptilolite minerals from the Talan-Gozagorsk deposit. This confirmed the effectiveness of this ore preparation method. Functions describing the relationship between extraction and particle size during the milling of Eastern Transbaikalia ZCR were obtained, allowing to determine the increase in the liberation coefficient values of zeolite minerals after the electron beam pretreatment:
Table 1
Chemical composition of Eastern Transbaikalia ZCR
Components |
Average component content in deposits, % |
||
Shivyrtuy |
Holinsky |
Talan-Gozagorsk |
|
SiO2 |
62.90 |
65.62 |
53.12 |
P2O5 |
0.08 |
0.0004 |
0.33 |
Al2O3 |
13.61 |
12.21 |
16.63 |
TiO2 |
0.34 |
0.07 |
1.50 |
Fe2O3 |
3.00 |
1.25 |
11.40 |
FeO |
0.14 |
0.06 |
0.32 |
CaO |
0.61 |
2.07 |
5.82 |
MgO |
1.51 |
0.64 |
1.97 |
Na2O |
1.36 |
1.90 |
3.45 |
K2O |
4.04 |
4.14 |
1.78 |
Stotal |
0.007 |
0.016 |
0.041 |
MnO |
0.11 |
0.14 |
0.08 |
H2O |
3.88 |
3.82 |
1.08 |
Others |
8.173 |
8.0636 |
2.479 |
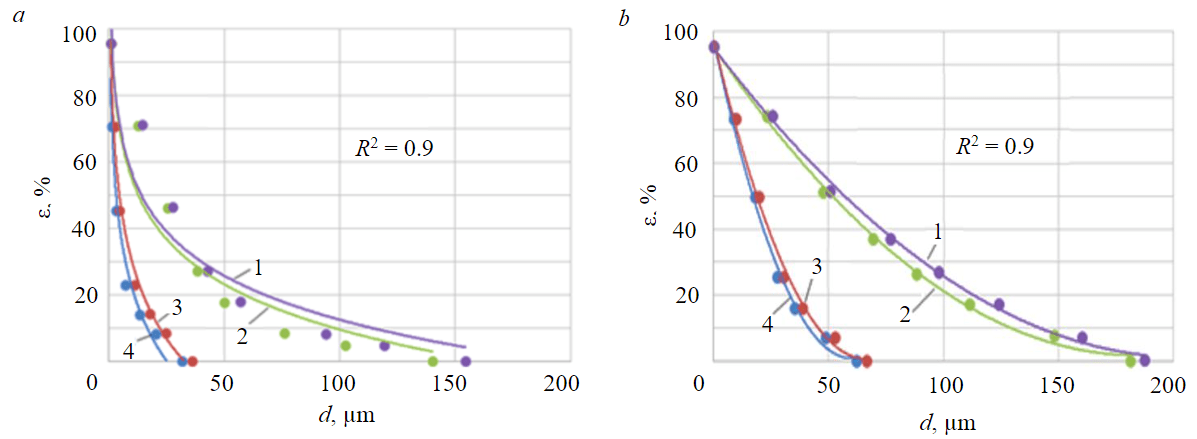
Fig.1. Influence of accelerated electron irradiation on the grain size distribution of ZCR (1, 2), zeolite minerals within the ZCR (3, 4), and the liberation coefficient of zeolites (clinoptilolite and chabazite): a – grinding without accelerated electron treatment; b – grinding with accelerated electron treatment at a dose of 2 kGy
- milling without accelerated electron treatment: ZCR from the Shivyrtuy deposit – the size of the milled rock is y= –24.08ln(x) + 77.6; the size of zeolite in the milled rock is y = –19.67ln(x) + 100.1; ZCR from the Talan-Gozagorsk deposit – the size of the milled rock is y = –19.13ln(x) + 100.5; the size of zeolite in the milled rock is y = –25.46ln(x) + 88.7;
- milling after accelerated electron treatment: ZCR from the Shivyrtuy deposit – the size of the milled rock is y = 0.027x2 – 3.2307x + 97.4; the size of zeolite in the milled rock is y = 0.0223x2 – 2.9383x + 97.5; ZCR from the Talan-Gozagorsk deposit – the size of the milled rock is y = 0.0028x2 − 1.0157x + 95.2; the size of zeolite in the milled rock is y = 0.0022x2 – 0.9164x + 95.
Figure 2 shows the temperature dependence of the heat capacity of the original and treated ZCR from the Talan-Gozagorsk deposit. Treating ZCR with accelerated electrons considerably affects this parameter.
The results of measuring the heat capacity of the original and accelerated electron-treated ZCR from the Shivyrtuy deposit showed that the initial heat capacities of both materials were identical (1.6 J/g) with a maximum value recorded at 130 °C. The maximum absolute value of the heat capacity of the ZCR from the Shivyrtuy deposit was considerably lower than that of the ZCR from the Talan-Gozagorsk deposit, which was 2.3 J/g. The higher heat capacity is attributed to the higher hematite content in the ZCR from the Talan-Gozagorsk deposit.
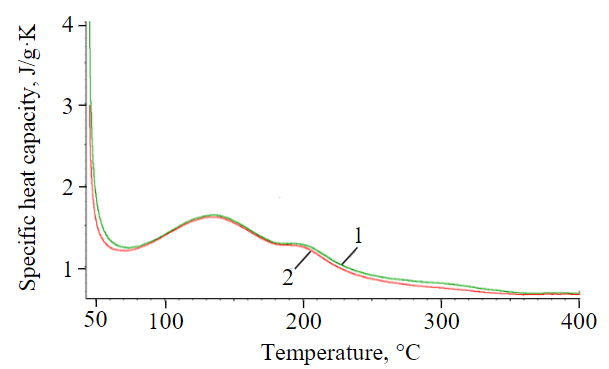
Fig.2. Temperature dependence of the specific heat capacity of ZCR from the Talan-Gozagorsk deposit
1 – before exposure to accelerated electrons; 2 – after exposure to accelerated electrons
The analysis of the Mössbauer spectroscopy results of the initial and accelerated electron-treated ZCR from the Talan-Gozagorsk deposit revealed that the sextets correspond to hematite, while the doublets correspond to montmorillonite (Table 2).
Treating ZCR with accelerated electrons considerably reduces the fine-dispersed hematite fraction and increases the coarse crystalline fraction. The accelerated electrons increased the iron content in the ZCR by removing volatile components and altering the magnitude of the resonance effect associated with the total iron content.
Table 2
Results of Mössbauer studies of ZCR from Eastern Transbaikalia
ZCR |
Spectrum component |
Isomeric shift δ, mm/s |
Magnetic fields on Fe57H nuclei, kOe |
Componentarea S, % |
Effect magnitude ε, % |
Interpretation |
Talan-Gozagorsk field,original |
C1(Fe3+) |
0.37 |
512 |
55.9 |
3.185 |
Hematite |
C2(Fe3+) |
0.39 |
491 |
18.4 |
|||
C3(Fe3+) |
0.36 |
362 |
0.6 |
Goethite hydrogoethite |
||
D1(Fe3+) |
0.38 |
0 |
10.1 |
Montmorillonite, hydromicas |
||
D2(Fe3+) |
0.38 |
0 |
15.0 |
|||
Talan-Gozagorsk field, processed |
C1(Fe3+) |
0.37 |
512 |
51.4 |
3.262 |
Hematite |
C2(Fe3+) |
0.37 |
492 |
24.5 |
|||
C3(Fe3+) |
0.36 |
362 |
1.5 |
Goethite hydrogoethite |
||
D1(Fe3+) |
0.38 |
0 |
9.4 |
Montmorillonite, hydromicas |
||
D2(Fe3+) |
0.36 |
0 |
13.2 |
|
||
Shivyrtuy field, original |
C1(Fe3+) |
037 |
513 |
6.8 |
1.069 |
Hematite |
D1(Fe2+) |
1.16 |
0 |
1.8 |
Olivine |
||
D2(Fe2+) |
1.07 |
0 |
4.5 |
Chlorite |
||
D3(Fe3+) |
0.43 |
0 |
7.7 |
Montmorillonite, hydromicas |
||
D4(Fe3+) |
0.37 |
0 |
12.8 |
|||
D5(Fe3+) |
0.37 |
0 |
66.4 |
|||
Shivyrtuy field, processed |
C1(Fe3+) |
0.37 |
512 |
5.5 |
1.250 |
Hematite |
D1(Fe2+) |
1.16 |
0 |
1.7 |
Olivine |
||
D2(Fe2+) |
1.07 |
0 |
4.4 |
Chlorite |
||
D3(Fe3+) |
0.41 |
0 |
9.3 |
Montmorillonite, hydromicas |
||
D4(Fe3+) |
0.37 |
0 |
7.6 |
|||
D5(Fe3+) |
0.37 |
0 |
71.5 |
The impact of accelerated electrons on ZCR intensifies the process of liberating intergrowths and separating zeolite minerals from rock-forming ones. The most significant effect on altering the technological properties of ZCR from the Shivyrtuy and Talan-Gozagorsk deposits was observed at low doses of accelerated electron exposure, specifically 2-4 kGy. When processing ZCR with a beam of accelerated electrons at low current densities, defects form in them. With a further increase in the absorbed energy (from 1 to 10 J/g), charge accumulates, leading to electrical breakdown in the ZCR. In this case, the discharge has a pulsating character, resulting in the formation of microcracks [21, 26], which considerably weaken the rocks, particularly along the boundaries of intergrowth between zeolite minerals and rock-forming minerals, determining the efficiency of subsequent selective disintegration [30, 31].
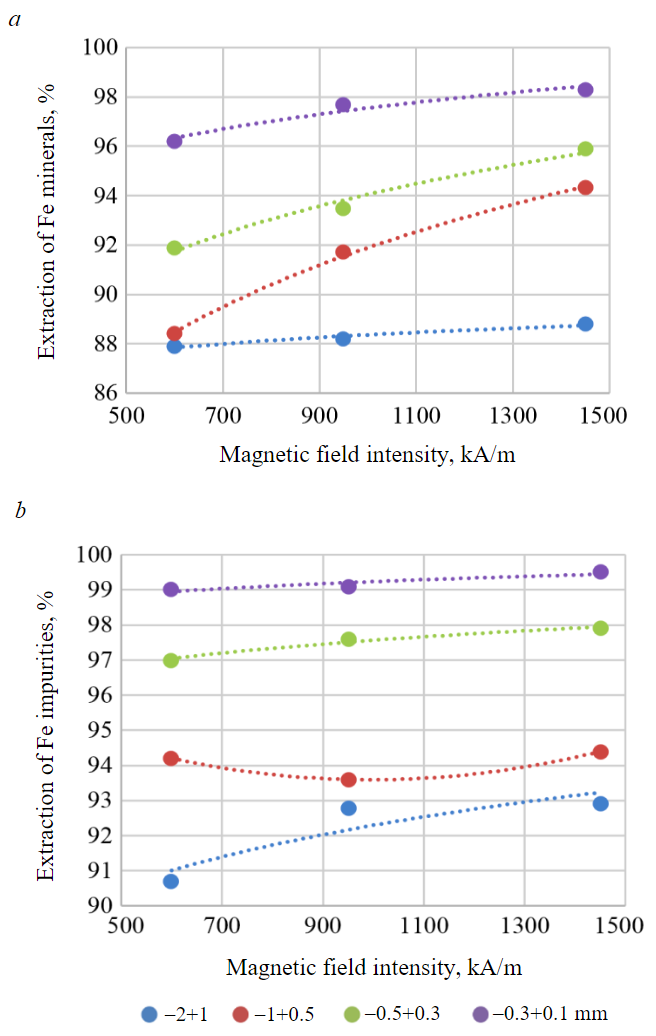
Fig.3. Dependence of the extraction of iron-containing minerals from ZCR from Shivyrtuy (a) and Talan-Gozagorsk (b) deposits on the magnetic field intensity for various particle sizes
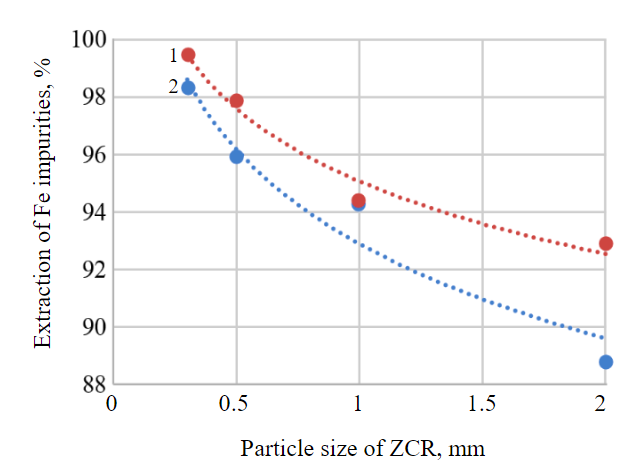
Fig.4. Dependence of the extraction of iron-containing minerals by electromagnetic separation on the particle size of ZCR
1 – Talan-Gozagorsk deposit; 2 – Shivyrtuy deposit
When ZCR from the Shivyrtuy and Talan-Gozagorsk deposits were exposed to accelerated electrons, it significantly altered their granulometric composition during milling and allowed for a more selective liberation of zeolite mine-rals (clinoptilolite and chabazite), improving subsequent magnetic and electrostatic separation processes. The electricity consumption was 0.6 kWh/t. The use of radiation treatment as a part of ore preparation operations, in combination with electromagnetic and electrostatic separation methods, reduced iron-containing minerals in the ZCR concentrates from the Shivyrtuy deposit from 3.14 to 0.36 % and from 11.2 to 0.12 % from the Talan-Gozagorsk deposit. Radiation treatment increased the content of coarse-grained hematite and the magnetic susceptibility of hematite from 14-25 · 10−8 to 460-660 · 10−8 m3/kg, indicating the effective application of electromagnetic separation in the enrichment of ZCR.
When the results from the treatment of Eastern Transbaikalia ZCR with accelerated electrons were analyzed, we established technological dependencies, as presented in Fig.3, 4. The coefficient of determination for these dependencies was 0.9. Figure 3, a shows the relationship between the extraction of iron-containing minerals from ZCR from the Shivyrtuy deposit and the magnetic field intensity for different particle sizes. The relationship between the extraction of iron-containing minerals from ZCR from the Talan-Gozagorsk deposit and the magnetic field intensity for different particle sizes is shown in Fig.3, b.
Figure 4 illustrates the relationship bet-ween the extraction of iron-containing minerals by electromagnetic separation and the particle size of ZCR during treatment with accelerated electrons.
The following functions are used to show the dependence of the extraction of iron-containing minerals from ZCR subjected to accelerated electron treatment on the magnetic field intensity of the separator for different particle sizes (see Fig.3). For ZCR from the Shivyrtuy deposit: y = 1.015ln(x) + 81.35 (particle size −2+1 mm), y = 6.693ln(x) + 45.65 (particle size −1+0.5 mm), y = 4.518ln(x) + 62.84 (particle size −0.5+0.3 mm), y = 2.393ln(x) + 81.02 (particle size −0.3+0.1 mm). For ZCR from the Talan-Gozagorsk deposit: y = 2.5232ln(x) + 74.86 (particle size −2+1 mm), y = 4E − 06x2 − 0.01x + 97.45 (particle size −1+0.5 mm), y = 1.0241ln(x) + 90.49 (particle size −0.5+0.3 mm), y = 0.561ln(x) + 95.35 (particle size −0.3+0.1 mm).
The study of the dependence of iron-containing mineral content on the particle size of ZCR treated with accelerated electrons (after magnetic separation) showed that as the rock particle size increased from 0.07 to 0.1 mm, the iron content increased as follows. For the ZCR from the Shivyrtuy deposit, the iron content increased from 0.35 to 0.85 %. For the ZCR from the Talan-Gozagorsk deposit, the iron content increased from 0.1 to 1.1 %. The dependence of the extraction of iron-containing minerals from ZCR on the particle size (Fig.4) (after treatment with accelerated electrons) is described by the following functions: y = −3.647ln(x) + 95.07 (for ZCR from the Shivyrtuy deposit) and y = −4.74ln(x) + 92.89 (for ZCR from the Talan-Gozagorsk deposit).
The study on the possibility of extracting iron-containing minerals from fine-dispersed accele-rated electron-treated ZCR from Eastern Transbaikalia with a particle size of −0.1+0.074 mm using magnetic separation in an isodynamic field allowed to determine the dependence of iron impurity content in zeolite (nonmagnetic) products on their particle size. The corresponding functions cha-racterizing the parameters of this dependence were determined: y = 6.539x + 1.3262 (for ZCR from the Shivyrtuy deposit) and y = 30.385x − 0.2685 (for ZCR from the Talan-Gozagorsk deposit).
The radiation treatment with accelerated electrons of ZCR from the Shivyrtuy and Talan-Gozagorsk deposits increased the extraction of iron-containing minerals by electromagnetic separation to 98.3 and 99.5 %, respectively. This ensures the production of high-quality zeolite products that can be used in various industrial sectors and in waste management technologies in mining. The greatest effect was achieved at a magnetic field intensity of 1450 kA/m and a particle size of −0.3+0.1 mm (Table 3). The enrichment of ZCR in an isodynamic field is characterized by high iron impurity extraction rates at a particle size of 0.1 mm (Table 4).
Table 3
Results of dry electromagnetic separation of ZCR from Eastern Transbaikalia with a particle size of −2+0.1 mm
Deposit |
Particle size, mm |
Average Fe impurity content in raw material, % |
Zeolite contentin product, % |
Fe minerals content in zeolite product, % |
Other impurities content, % |
Yield, % |
Shivyrtuy |
−2+1−1+0.5−0.5+0.3−0.3+0.1 |
3.14 |
73.5084.2085.9088.90 |
1.781.110.880.36 |
24.7214.6913.2210.74 |
9.418.427.744.5 |
Talan-Gozagorsk |
−2+1−1+0.5−0.5+0.3−0.3+0.1 |
11.2 |
70.1075.2084.9089.60 |
8.173.741.080.12 |
21.7321.0614.0210.28 |
8.817.123.250.9 |
Table 4
Results of dry electromagnetic separation of fine-dispersed ZCR in an isodynamic field
Deposit |
Magnetic field intensity, kA/m |
Current strength, A |
Particle size, mm |
Extraction of Fe minerals from magnetic product, % |
Mass fraction of Fe in magnetic product, % |
Yield, % |
Shivyrtuy |
600 |
0.50 |
−0.1+0.074−0.074+0.05 |
89.796.4 |
92.494.4 |
44.755.3 |
950 |
1.0 |
−0.1+0.074−0.074+0.05 |
93.397.9 |
95.696.2 |
47.552.5 |
|
1450 |
1.57 |
−0.1+0.074−0.074+0.05 |
95.498.2 |
96.797.1 |
49.850.2 |
|
Talan-Gozagorsk |
600 |
0.50 |
−0.1+0.074−0.074+0.05 |
98.799.1 |
96.396.8 |
49.650.4 |
950 |
1.0 |
−0.1+0.074−0.074+0.05 |
98.599.2 |
96.298.1 |
47.452.6 |
|
1450 |
1.57 |
−0.1+0.074−0.074+0.05 |
98.399.6 |
97.498.7 |
46.153.9 |
The application of directional methods, such as treatment with accelerated electrons, in the beneficiation schemes of ZCR considerably enhances the adsorption capacity of natural zeolites (from 147 to 164 mg/g for ZCR from the Shivyrtuy deposit and from 246 to 287 mg/g for ZCR from the Talan-Gozagorsk deposit) [28]. The increase in the sorption capacity of ZCR is achieved via the extraction of iron-bearing minerals and the purification of the pore space of natural zeolites from organic compounds, which is achieved through preprocessing using directional methods in combination with magnetic and electrostatic separations [32-34].
One crucial factor in developing beneficiation and processing technologies for ZCR is the ability to comprehensively process ZCR and separate them from rock-forming minerals [30, 31]. The obtained dependencies in the processing and beneficiation of ZCR from Eastern Transbaikalia allowed to determine a rational sequence of operations in the technological scheme: crushing; enhancing contrast and altering physicochemical properties through directional treatment (treatment with accelerated electrons); milling; and magnetic and electrostatic separation to obtain high-quality zeolite concentrate, iron-bearing (hematite, hydrogoethite, olivine, and pyroxene), and polymineral (quartz, plagioclases, calcite, montmorillonite) products, which are sent for further processing or practical use. In particular, the iron-bearing product can serve as a source of iron, while the polymineral product can be used as a binder for backfilling mined-out areas [35-37].
Creating beneficiation technologies for ZCR involves the following: directed impact before crushing and milling of the material used for disintegration and altering the contrast properties of minerals; maximum extraction of Fe-bearing minerals by electromagnetic separation using separators with an isodynamic field; and extraction of nonmagnetic impurities through electrostatic separation.
Theoretical and experimental studies allowed us to identify the main methods of beneficiation and ore preparation for ZCR, which are crucial for developing the technology selection algorithm for the beneficiation of ZCR from Eastern Transbaikalia (Table 5).
Table 5
Separation methods for ZCR depending on the mineral composition
Separated mineral |
Main associated minerals |
Concentration methods |
Zeolite |
Feldspars, micas > 50 µm |
Crushing in rotary crushers and milling in ball mills |
Directed treatments (accelerated electrons) |
||
Electromagnetic separation (for fine-dispersed ZCR using an electromagnetic separator with an isodynamic field) |
||
Electrostatic separation |
||
Clay minerals < 50 µm |
Directed treatments (accelerated electrons) |
|
Electromagnetic separation using an electromagnetic separator with an isodynamic field |
||
Electrostatic separation with heating and electrification |
||
Minerals of the quartz group, clay minerals |
Crushing in rotary crushers and milling in ball mills |
|
Accelerated electrons |
||
Electrostatic separation |
The proposed systematization allows us to determine the number of stages in ore preparation, select the necessary type of impact on ZCR, and develop rational processes for their enrichment and processing, which determines the efficiency of separating zeolite minerals from rock-forming mine-rals, taking into account technological properties (electrical conductivity and magnetic susceptibility) and technological performance indicators. The experimental studies on the enrichment of ZCR serve as the basis for the development of technological schemes for their enrichment.
The developed scientific and methodological foundations for the processing and enrichment of ZCR have been tested under industrial conditions. The possibility of implementing the developed technology for the enrichment of ZCR from the Talan-Gozagorsk and Shivyrtuy deposits in the production complex of OOO “Scientific and Production Entrepreneurship Society “Zeolite” (Krasnokamensk) was studied, resulting in the production of high-quality zeolite products. To achieve the required quality parameters of zeolite products, which meet the technical specifications, the following processes should be introduced into the technological scheme:
- directed impact on ZCR before milling;
- electromagnetic separation in an isodynamic field to achieve the maximum extraction of iron-containing minerals;
- electrostatic separation;
- electrification of ZCR with salicylic acid vapor to enhance the contrast of minerals separated by electrostatic separation.
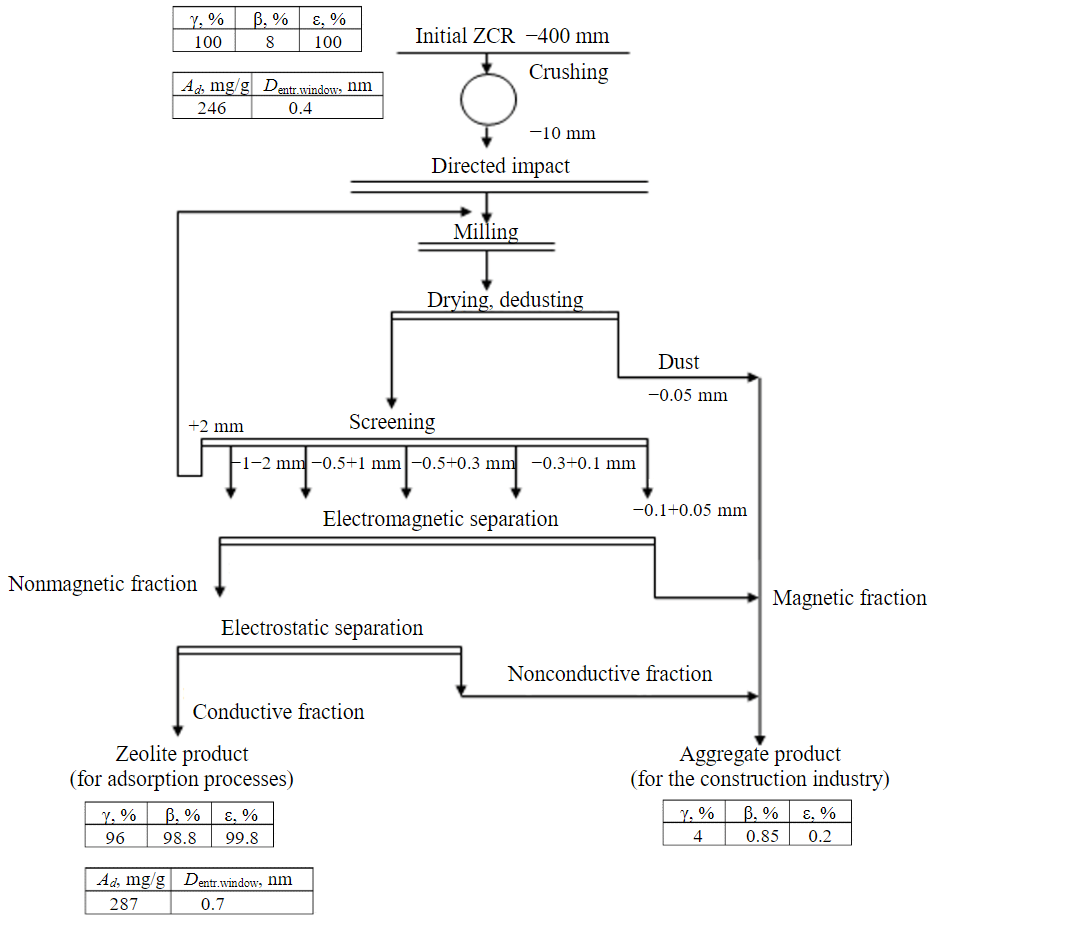
Fig.5. Technological scheme for processing ZCR from Eastern Transbaikalia [21]
The technological scheme for the enrichment of ZCR is shown in Fig.5. The practical significance of the results was confirmed by implementing the developed technology for ZCR enrichment in the techno-economic calculations of OOO “Scientific and Production Entrepreneurship Society “Zeolite”.
The assessment of the economic efficiency of implementing the technology for processing natural ZCR at the beneficiation plant of OOO “Scientific and Production Entrepreneurship Society “Zeolite” showed that the cost for obtaining high-quality zeolite product with a monomineral content of up to 99.1 % will be approximately 10,000 rubles per ton, and the market price of the pro-duct of the appropriate quality will be up to 100,000 rubles per ton. The calculations justify the environmental and economic feasibility of using directed interventions during the preparation stage of ZCR from Eastern Transbaikalia. Thus, the implementation of the proposed technologies for the beneficiation and processing of ZCR from Eastern Transbaikalia is sufficiently effective, with a discounted payback period of one year.
Conclusion
In this study, it was determined that accelerated electron treatment of ZCR from Shivyrtuy and Talan-Gozagorsk deposits significantly altered the granulometric composition during milling and provided a more selective liberation of zeolite minerals (clinoptilolite and chabazite), thus improving the subsequent processes of magnetic and electrostatic separation. The use of radiation treatment as an ore preparation operation in combination with the electromagnetic and electrostatic separation methods contributes to the reduction of the Fe-containing mineral content in concentrates from 3.14 to 0.36 % and from 11.2 to 0.12 % for ZCR from Shivyrtuy and Talan-Gozagorsk deposits, respectively. The radiation treatment increased the content of coarse-crystalline hematite and the magnetic susceptibility parameter of hematite from 14-25 · 10−8 to 460-660 · 10−8 m3/kg. This enables the effective use of electromagnetic separation during ZCR beneficiation and the production of high-quality zeolite products, which can be used in various industries and in waste management technologies in the mining industry.
References
- Gorlova O.E., Shadrunova I.V., Zhilina V.A. Development of deep and comprehensive processing processes of technogenic mineral raw materials in a view of sustainable development strategy. IMPC 2018 – 29th International Mineral Processing Congress, 17-21 September 2018, Moscow, Russian Federation. Canadian Institute of Mining, Metallurgy and Petroleum, 2019, p. 3279-3287.
- Solodukhina M.A., Yurgenson G.A. Arsenic in the landscapes of the Sherlovogorsk Ore District (Eastern Transbaikalia). Chita: Zabaikalskii gosudarstvennyi universitet, 2018, p. 176 (in Russian).
- Kudеlkо J. Еffеctivеnеss оf minеrаl wаstе mаnаgеmеnt. Intеrnаtiоnаl Jоurnаl оf Mining, Rеclаmаtiоn аnd Еnvirоnmеnt. 2018. Vol. 32. N 6, p. 440-448. DOI: 10.1080/17480930.2018.1438036
- Zaharyan S.V., Gedgagov E.I., Yun A.B. Increase in an Ecological Safety at the Entities of Non-Ferrous Metallurgy Due to Use of Sorption Processes. Ecology and Industry of Russia. 2018. Vol. 22. N 1, p. 26-32 (in Russian). DOI: 10.18412/1816-0395-2018-1-26-32
- Shadrunova I.V., Gorlova O.E., Zhilina V.A. The new paradigm of an environmentally-driven resourcesaving technologies for processing of mining. IOP Conference Series: Materials Science and Engineering. 2019. Vol. 687. Iss. 6. N 066048. DOI: 10.1088/1757-899X/687/6/066048
- Kauppila P., Ryaisyanen M.L., Myullyuoya S. Best environmental practices in the mining industry. Khelsinki: Tsentr okruzhayushchei sredy Finlyandii, 2013, p. 241 (in Russian).
- Abdrakhmanova R.N., Orehkova N.N., Gorlova O.E.Adaptation of the SAVMIN process for mine water treatment. IOP Conference Series: Materials Science and Engineering. 2019. Vol. 687. Iss. 6. N 066077. DOI: 10.1088/1757-899X/687/6/066077
- Zakondyrin A.E. Best Available Techniques in mining industry: topical issues and solution methods. Mining Informational and Analytical Bulletin. 2020. N 6-1, p. 55-64 (in Russian). DOI: 10.25018/0236-1493-2020-61-0-55-64
- Skobelev D.O. Information and methodological support to the implementation of Best Available Techniques in the Russian Federation. Upravlenie. 2019. Vol. 7. N 4, p. 5-15 (in Russian). DOI: 10.26425/2309-3633-2019-4-5-15
- Savon D.Yu. Improvement of system of paid environmental management. Mining Informational and Analytical Bulletin. 2014. N 6, p. 314-320 (in Russian).
- Alam R., Ahmed Z., Howladar M.F. Evaluation of heavy metal contamination in water, soil and plant around the open landfill site Mogla Bazar in Sylhet, Bangladesh. Groundwater for Sustainable Development. 2020. Vol. 10. N 100311. DOI: 10.1016/j.gsd.2019.100311
- Shushkov D.A., Shuktomova I.I. Sorption of radioactive elements by zeolite-bearing rocks. Proceedings of the Komi Science Centre of the Ural Division of the Russian Academy of Sciences. 2013. Iss. 1 (13), p. 69-73 (in Russian).
- Sivashankari L., Rajkishore S.K., Lakshmanan A., Subramanian K.S. Optimization of dry milling process for synthesizing nano zeolites. International Journal of Chemical Studies. 2019. Vol. 7. N 4, p. 328-333.
- Tyaglov S.G., Voskresova G.N. Features of determination of technology as NDT: Russian and foreign experience. Journal of Economic Regulation. 2019. Vol. 10. N 2, p. 96-112 (in Russian). DOI: 10.17835/2078-5429.2019.10.2.096-112
- Kotova O.B., Shushkov D.A. A process of zeolites production from fly ash. Obogashchenie rud. 2015. N 5, p. 60-63 (in Russian). DOI: 10.17580/or.2015.05.10
- Manchuk J.G., Birks J.S., McClain C.N. et al. Estimating Stable Measured Values and Detecting Anomalies in Groundwater Geochemistry Time Series Data Across the Athabasca Oil Sands Area, Canada. Natural Resources Research. 2021. Vol. 30. N 2, p. 1755-1779. DOI: 10.1007/s11053-020-09801-5
- Cecinati F., Matthews T., Natarajan S. et al.Mining Social Media to Identify Heat Waves. International Journal of Environmental Research and Public Health. 2019. Vol. 16. Iss. 5. N 762. DOI: 10.3390/ijerph16050762
- Li H., Watson J., Zhang Y. et al. Environment-enhancing process for algal wastewater treatment, heavy metal control and hydrothermal biofuel production: A critical review. Bioresource Technology. 2020. Vol. 298. N 122421. DOI: 10.1016/j.biortech.2019.122421
- Uçkun Ş., Sarıkaya M., Top S., Timür İ. Removal of Heavy Metals from Wastewater Solution Using a Mechanically Activated Novel Zeolitic Material. Journal of Mining Science. 2020. Vol. 56. N 6, p. 1010-1023. DOI: 10.1134/S1062739120060137
- Khatkova A.N., Rostovtsev V.I., Razmakhnin K.K., Emelyanov V.N. Effect of Accelerated Electrons on Zeolite-Containing Rocks of the East Transbaikalia. Journal of Mining Science. 2013. Vol. 49. N 6, p. 1004-1010. DOI: 10.1134/S1062739149060221
- Razmakhnin K.K. Development and justification of treatment and modification technology for East Transbaikalia zeolite rocks. Journal of Mining Science. 2021. Vol. 57. N 3, p. 493-501. DOI: 10.15372/FTPRPI20210314
- Ping Wang, Zehang Sun, Yuanan Hu, Hefa Cheng. Leaching of heavy metals from abandoned mine tailings brought by precipitation and the associated environmental impact. Science of the Total Environment. 2019. Vol. 695. N 133893. DOI: 10.1016/j.scitotenv.2019.133893
- Xueyan Zou, Yanbao Zhao, Zhijun Zhang. Preparation of hydroxyapatite nanostructures with different morphologies and adsorption behavior on seven heavy metals ions. Journal of Contaminant Hydrology. 2019. Vol. 226. N 103538. DOI: 10.1016/j.jconhyd.2019.103538
- Mei Hong, Lingyun Yu, Yanding Wang et al. Heavy metal adsorption with zeolites: The role of hierarchical pore architecture. Chemical Engineering Journal. 2019. Vol. 359, p. 363-372. DOI: 10.1016/j.cej.2018.11.087
- Yiping Su, Jing Wang, Shun Li et al. Self-templated microwave-assisted hydrothermal synthesis of two-dimensional holey hydroxyapatite nanosheets for efficient heavy metal removal. Environmental Science and Pollution Research. 2019. Vol. 26. Iss. 29, p. 30076-30086. DOI: 10.1007/s11356-019-06160-4
- Rostovtsev V.I. Theoretical fundamentals and practice of electrochemical and radiation effects application in ore preparation and mineral processing. Vestnik Chitinskogo gosudarstvennogo universiteta. 2010. N 8 (65), p. 91-99 (in Russian).
- Yusupov T.S., Baksheeva I.I., Rostovtsev V.I. Analysis of Different-Type Mechanical Effects on Selectivity of Mineral Dissociation. Journal of Mining Science. 2015. Vol. 51. N 6, p. 1248-1253. DOI: 10.1134/S1062739115060552
- Milyutin V.V., Nekrasova N.A., Razmakhnin K.K., Khatkova A.N.Natural Zeolites of Eastern Transbaikalia in Technologies for Mining Enterprises Wastewater Treatment. Journal of Environmental Research, Engineering and Management. 2020. Vol. 76. N 3, p. 62-70. DOI: 10.5755/j01.erem.76.3.24220
- Litvinenko V.G., Razmakhnin K.K. Natural zeolites in technologies to ensure environmental safety of mining industries. Fundamentalnye i prikladnye voprosy gornykh nauk. 2021. Vol. 8. N 2, p. 158-162 (in Russian). DOI: 10.15372/FPVGN2021080224
- Chanturia V.A., Shadrunova I.V., Zhilina V.A. Recovery of mining waste in the complex development of mineral resources. Moscow: Publishing house Sputnik+, 2019, p. 120.
- Chanturia V.A., Shadrunova I.V., Zhilina V.A. et al. Environmentally focused processing of mining waste. Мoscow: ООО “Izd-vo “Sputnik+”, 2018, p. 200 (in Russian).
- Itzel-Hernández G., Hernández M.A., Portillo R. et al. Hierarchical structure of nanoporosity of Mexican natural zeolites of clinoptilolite type. Bulletin of the Tomsk Polytechnic University. Geo Assets Engineering. 2018. Vol. 329. N 10, p. 107-117 (in Russian). DOI: 10.18799/24131830/2018/10/2110
- Ying Wang, Yange Yu, Haiyan Li, Chanchan Shen.Comparison study of phosphorus adsorption on different waste solids: Fly ash, red mud and ferric-alum water treatment residues. Journal of Environmental Sciences. 2016. Vol. 50, p. 79-86. DOI: 10.1016/j.jes.2016.04.025
- Kotova O.B., Shabalin I.L., Kotova E.L. Phase transformations in synthesis technologies and sorption properties of zeolites from coal fly ash. Journal of Mining Institute. 2016. Vol. 220, p. 526-531. DOI: 10.18454/PMI.2016.4.526
- Sugonyako D.V., Zenitova L.A. Silicon dioxide as a reinforcing filler of polymeric materials. Herald of Technological University. 2015. Vol. 18. N 5, p. 94-100 (in Russian).
- Bogdanov A.V., Fedotov K.V., Kachor O.L. Development of scientific and practical foundations for the recuperative technology of ecoconcreting of arsenic-containing waste from the mining and processing industry. Irkutsk: Izd-vo IrGTU, 2014, p. 182 (in Russian).
- Aleksandrova T.N., Afanasova A.V., Nikolaeva N.V. On the Use of Microwave Treatment of Gold-Containing Concentrates for Its Efficient Processing. New Materials: Preparation, Properties and Applications in the Aspect of Nanotechnology. 2020, p. 179-188.