Evaluation of the effectiveness of neutralization and purification of acidic waters from metals with ash when using alternative fuels from municipal waste
Abstract
The problem of pollution of natural water objects with heavy metals is extremely relevant for the areas where industrial enterprises are located. Unauthorized discharge of contaminated wastewater, inefficient operation of sewage treatment plants, as well as leakage of drainage waters from man-made massifs lead to changes in the hydrological system affecting living objects. The article studies the composition of ash from the combustion of alternative fuels from municipal waste, and also considers the possibility of using it to neutralize sulfuric acid drainage waters and extract metal ions (Cu, Cd, Fe, Mn, Zn) from them. It has been established that the efficiency of water purification from metals depends on the pH value achieved during the purification process. The pH value is regulated by the dose of the introduced ash, the contact time and depends on the initial concentration of metal ions and sulfates in the solution. Studies on the neutralization and purification of a model solution of sulfuric acid drainage waters of a tailings farm of known composition have shown that in order to achieve a pH of 8-9, optimal for precipitation of metal hydroxides Cu, Cd, Fe, Mn, Zn and Al washed out of ash, and water purification with an efficiency of 96.60 to 99.99 %, it is necessary to add 15 g/l of ash and stir the suspension continuously for 35 minutes. It was revealed that exposure to ash with sulfuric acid waters leads to the transition of water-soluble forms of metals into insoluble ones and their “cementation” with calcium sulfate. The amount of Zn and Fe ions washed out of the ash decreases by 82 and 77 %, Al, Cd, Cu, Mn – by 25 %. This reduces the toxicity of ash, which is proved by a decrease in the toxic multiplicity of dilution of the water extract by 14 times.
Funding The work was carried out within the framework of the state assignment of the Ministry of Science and Higher Education of the Russian Federation (FSRW-2024-0005).
Introduction
Pollution of natural water objects with heavy metals remains an urgent environmental problem to this day. According to the Ministry of Natural Resources of the Russian Federation the share of heavy metal pollution of surface fresh waters in 2022 increased by 8 % compared to 2021, which accounted for 54 % of the total number of cases of high and extremely high pollution. Half of the accidents at surface freshwater facilities are related to unauthorized discharge of contaminated wastewater or inefficient operation of wastewater treatment plants.
The production process of mining enterprises, starting from the stage of drilling and blasting operations and ending with the disposal of waste from processing and overburden, is inevitably accompanied by negative effects on the components of the natural environment and humans [1, 2]. One of the main sources of large-scale intake of heavy metals into natural waters are bulk and alluvial man-made massifs of enterprises engaged in the extraction and processing of mineral raw materials [3, 4]. In the process of interaction of sulfide ore processing waste with atmospheric precipitation, surface and underground flows, acidic highly mineralized multicomponent waters with metal concentrations exceeding background and maximum permissible values by several orders of magnitude are formed [5]. Drainage waters enter surface waters through catchment areas, as well as a result of leaks of drainage systems [6]. Heavy metals in natural waters can change their location, thereby leading to changes in the hydrological system affecting the ecosystem of water objects [7]. Bottom sediments, accumulating heavy metals, become man-made sediments – a source of secondary pollution of water objects [8, 9].
Currently, there is a high interest in environmental issues of the Arctic zone of the Russian Fede-ration (AZRF). Due to its geopolitical position and huge resource potential, the Russian Arctic is a region of strategic interests not only for Russia, but also for many countries of the world. According to the State Report, the Russian Arctic is recognized as the most sensitive region of Russia to environmental pollution due to the “high vulnerability of geosystems and low ability to restore landscapes characteristic of the region”. Since the XXI century, a huge amount of minerals has been mined in the Russian Arctic at Ni-Cu-MPG, Pb-Zn-Ag and copper-porphyry deposits, deposits of apatites, titanium, etc. More than 60 mineral deposits have been discovered only in the Kola Peninsula. Problem areas related to water pollution are located in the areas where ore mining and processing facilities are located.
The total pollution of AZRF surface waters increased by 35 % over the period 2014-2022. The main pollutants are compounds of iron, copper, manganese, zinc, aluminum, nickel, molybdenum and mercury.
The condition of the Severnaya Dvina River in the Arkhangelsk region is influenced by pulp and paper enterprises [10, 11]. The average content of metals (Fe, Cu, Zn, Al, Mn) in the river water exceeds the maximum permissible concentration (MPC) by 1.5-4.7 times [11].
In the Krasnoyarsk Region, the main source of pollution of natural water objects is wastewater and drainage waters of copper-nickel production enterprises, they contain ions Cu, Zn, Ni and Cd. The maximum pollution with copper ions occurs in the Nizhnyaya Tunguska River and the Boguchansk reservoir (maximum MPC exceeding by 83 and 55 times, respectively), zinc ions – in the Nizhnyaya Tunguska River (14.3 MPC) [12].
The largest number of cases of high and extremely high pollution occur in the water bodies of the Murmansk region. Here, the main sources of heavy metals are wastewater from mining enterprises (Kirov branch of AO Apatit, OOO Lovozerskii GOK, AO Kovdorskii GOK), iron ore production (AO Olkon) and metallurgical industry (AO Kol’skaya GMK, OAO Severonikel). In the area where enterprises are located, water objects are characterized by high salinity (up to 3,300 mg/l), they can be both alkaline (pH up to 10) and acidic (pH up to 3.7). The maximum permissible concentration in the water objects of the Kola Peninsula reaches the values: 2,350 (Cu), 342 (Ni), 94.7 (Mn), 50 (Al), 39.7 (Fe), 7.7 (Sr), 5.3 (Co) and 1.5 (Pb) [13]. As a result of pollution of the surface waters of the region, there is an increase in the content of Ni (1.2 MPC) and Cu (12.1 MPC), etc., and a deterioration in the water quality of Lake Imandra, which is a source of drinking water supply [14].
Purification of industrial wastewater, as well as acidic mine and drainage waters from metals, is carried out using various physical, chemical and biological processes [15-17]. The main methods are reagent (precipitation), sorption, ion exchange and electrochemical [16, 17]. The latter two expensive methods are used to refine water with low salinity. When using natural and artificial sorbents, the cost of cleaning highly concentrated wastewater also arises [18, 19]. The first and perhaps the only economically justified method of cleaning wastewater and drainage waters of mining enterprises from high metal content is the method using reagents.
The essence of the reagent method is the precipitation of metals in the form of various compounds (hydroxides, carbonates, sulfates) by changing the pH of acidic waters to the alkaline side and the course of redox reactions. In industry, sodium, potassium, and calcium hydroxides (in the form of lime milk) and calcium and magnesium carbonates act as reagents [20]. To accelerate the deposition of metal hydroxides by more than 20 times, coagulants (for example, Al2(SO4)3 and NaAlO2) are added during the reagent purification process and flocculants (e.g. polyacrylamide) [20].
Currently, the field of research devoted to the study of the composition and properties of waste from various industries and the possibility of their involvement in the circulation of material resources as raw materials for various purposes is intensively developing. A large number of studies are aimed at studying the effectiveness of using slags and ashes of industries to purify water from various pollutants, especially metals. The high dispersion makes it possible to use them without grinding, and the low cost compared, for example, with lime, determines the economic feasibility of use [21].
In the article [22], a multi-stage purification of sulfuric acid subsurface waters using a heat-treated magnesia-silicate reagent in the form of granules based on the overburden of the magnesite deposit is proposed. In [23], the mechanism of extraction of molybdenum (VI) ions from model solutions by iron-containing metalworking waste was studied. As a result of the experiments, it was determined that the efficiency of wastewater treatment from the studied ions ensures a decrease in their content to standard values. In the study [24], iron-magnesium waste was evaluated as a reagent for removing metals from wastewater. It was found that the initial concentration of metal ions in water, the amount of waste introduced per unit volume of treated water, as well as the contact time of the waste–water system affect the cleaning efficiency. An organic waste recycling product (bio coal from chicken manure) was investigated [25] as a sorbent for removing heavy metals from polluted waters. In [26], the authors studied the fly ash of various coals and evaluated its ability to neutralize. It was revealed that the use of one of the ash samples makes it possible to achieve 56.4-74.3 % efficiency of removing metals from the solution. There are studies on the synthesis of a mesoporous sieve from the ash of a coal residue, the properties of which make it possible to remove Pb, Cu and Cd from water solutions with an efficiency of up to 99.4; 41.66 and 43.98 %, respectively [27].
Both in Russia and in the world, the issue of recycling municipal solid waste (MSW) is acute. Every year in our country, the number of MSW generated increases by 3 %, of which 93-95 % are disposed of at landfills, and only 5-7 % are recycled [28]. Issues related to the handling of MSW and their impact on the environment are among the main environmental problems of the Arctic zone of the Russian Federation and have been considered in many scientific papers [29-31].
One of the directions of MSW processing is the production of alternative fuels by pre-sorting, drying, grinding, magnetic separation and granulation. The composition of the fuel depends on the place, area and conditions of origin [32]. Consumers of this fuel are cement plants and fuel and energy complex.
On the one hand, the creation of alternative fuels from MSW has positive prospects: obtaining cheap energy, reducing the volume of MSW, and, as a result, the areas for their placement. Investors, industrialists and the state are interested in the development of this area. On the other hand, fuel combustion is inevitably accompanied by the formation of ash and fly ash (10-30 % of the initial mass), which contain toxic inorganic and organic compounds. In particular, the heavy metals contained in MSW do not decompose during combustion, but pass into combustion waste and fly ash [32, 33]. Untreated ash in landfill burial without pretreatment poses a great threat to the environment and human health due to the potential leaching of heavy metals [33, 34]. Thus, the question arises of the safe use of ash, as well as the development of methods for processing ash in order to reduce its toxicity for subsequent landfill placement.
Currently, the direction of using ash to produce a new product is developing. There are studies devoted to the study of the possibility of ash utilization by glazing it with glass waste and further use of the resulting material [35, 36]. In [37], the process of solidification and stabilization of the tailings of an abandoned lead-zinc mine was studied when using ash from the combustion of MSW as a binder, resulting in a building material in the form of unfired bricks. This technology makes it possible to immobilize the heavy metals that make up the tailings and ash.
Like the fly ash of various coals, the ash from the combustion of MSW has an alkaline reaction (pH 11-13), which indicates the possibility of considering it as a reagent for neutralizing acidic waters. Previously, this area of ash use was not considered, which confirms the relevance of the research carried out in the work and determines the novelty of the results obtained.
Methods
As part of the study, experiments were conducted aimed at studying the neutralization of a sulfuric acid model solution and the removal of heavy metals (Cu, Cd, Fe, Mn, Zn) from it with a material representing ash remaining after burning alternative fuels from municipal waste (hereinafter referred to as ash). For the model solution, the following composition of drainage waters of the tailings of sulfide waste was selected at a pH of the model solution of 3.4-3.6 [38]: Cu2+ – 17.5; Cd2+ – 0.2; Fetotal – 31.1; Mn2+ – 35.5; Zn2+ – 121.0 mg/dm3.
Investigation of the composition of ash samples
Ash samples formed as a result of burning fuel from solid municipal waste consisting of paper (about 40 %), polymers (about 40 %), tetrapack (about 5 %), textiles (about 10 %), wood (about 5 %) at a temperature of 1110 °C in gasifier boilers are connected and averaged by quartering.
All studies and analyses of samples were carried out in the laboratory of environmental modeling on the basis of the scientific center “Assessment of technogenic transformation of ecosystems” of Saint Petersburg Mining University. The elemental composition of the average ash sample was determined on an XRF-1800 X-ray fluorescence spectrometer by X-ray fluorescence spectrometry according to fundamental parameters without normalization with preliminary sample preparation according to GOST 55879-2013.
The mass fraction of the elements was determined using the atomic absorption spectrometry method on AA-7000 (Shimadzu) according to MVI-80-2008 with preliminary sample preparation (acid decomposition using a microwave system).
The determination of the water-soluble forms of metals contained in the ash was carried out using the atomic absorption spectrometry method on AAC-7000 (Shimadzu), according to M-02-1109-08. Sample preparation was carried out by preparing a water extract: a sample of ash (1 g) was mixed with distilled water (100 ml) for 3 min. After mixing, the extract was filtered for further analysis.
The toxicity of the Water extract from the ash was determined according to the method using a multi-cuvette cultivator KVM-05 and an optical suspension meter for algae IPS-03. The value of the toxic dilution multiplicity was calculated using the formula
where Рb – the largest dilution value, at which the deviation index was lower than the toxicity criterion; Рs – the smallest dilution value, at which the deviation index was higher than the toxicity criterion; Ib and Is – the values of the indices corresponding to these dilutions I, fractions.
The determination of active calcium oxide in ash was carried out by the saccharate method according to GOST 22688-2018:
where V – volume of hydrochloric acid (1 H) consumed for titration, ml; TCaO – titer of hydrochloric acid, g CaO; m – weight of the ash sample, g.
Experiments to determine the amount of ash and the time to achieve an equilibrium pH value; evaluation of the effectiveness of purification of the model solution from metals
The equilibrium pH value for a multicomponent model drainage water solution was determined empirically earlier. During the experiment [38], the optimal pH range (8-9) was established, at which metal hydroxides (Cu, Cd, Fe, Mn, Zn) are formed and precipitated from multicomponent acidic drainage waters. To establish the minimum amount of ash required for Purifying 100 ml of a model solution of tailings drainage waters, ash samples (1.0-1.6 g in 0.1 g increments) were mixed with a model solution of sulfuric acid waters (100 ml for each of the samples) until the pH was stabilized. The pH was monitored using a laboratory pH meter WTW inoLab pH 740 with a combined electrode. According to the results of the experiment, the pH dependences on the time and weight of the suspension were built.
The resulting suspensions “ash – model solution” were filtered through “a blue ribbon” filter for further analysis by atomic absorption spectrometry for the residual metal content in the filtrate.
The evaluation of the efficiency of removing metals from the model solution was determined by the formula
where Сin is the initial concentration of metal ion in solution, mg/dm3; Сres – is the residual concentration of metal ion in solution after contact with ash, mg/dm3.
Determination of the effect of the initial concentration of metal ions on neutralization and purification efficiency
The preparation of model solutions of specified metal concentrations was carried out using a state standard reference sample and reagents, the pH of the solution in the range of 3.4-3.6 was achieved by adding a dilute solution of sulfuric acid. The concentration of metal ions in the initial solution was 1,000 mg/l for each of the ions. Next, the initial solution was diluted 2, 4, 10, 20, 40 and 100 times. The selected ash samples were mixed with the initial and dilute water solutions during the optimal contact time of the ash with the model solution, after which the “ash – model solution” system was filtered for further analysis by atomic absorption spectrometry. The evaluation of the purifying efficiency was determined by the formula (3).
Assessment of the degree of metals leaching from the sediment after purification of the model drainage solution and determination of the toxicity of the extract
After purification of a multicomponent model drainage water solution from metals, the precipitate was dried in a drying cabinet at a temperature of 105±1 °C to a constant mass and crushed in a porcelain mortar. Then the water-soluble forms of metals were determined in a water extract prepared similarly to a water extract from the initial ash. The value of the toxic dilution multiplicity of the water extract from the sediment was determined similarly to the extraction from the initial ash.
Discussion of the results
The results of the conducted studies showed that the main components of ash are calcium and silicon (in terms of oxides – 39.7 and 29.9 %, respectively). A high content of aluminum and iron was noted (in terms of oxides – 9.2 and 4.1 %, respectively). According to the results of the analysis of the gross content of elements, the ash contains significant concentrations of metals studied in drainage waters (Table 1): Cd, Cu, Fe, Mn, Zn. Metals belonging to various hazard classes were also found in the ash: As, Be, Se, Pb (high degree of hazard); Co, Cr, Mo, Ni, Sb (medium degree of hazard); V, Ba, Sr (low degree of hazard).
Table 1
Ash composition
Indicator |
Al |
Cd |
Cu |
Fe |
Mn |
Zn |
Ca |
Mg |
Gross content, mg/kg |
70,566 |
5 |
3,575 |
76,952 |
1,275 |
3,873 |
328,855 |
22,054 |
Water-soluble form, % of the gross content |
2.00 |
2.56 |
0.03 |
0.01 |
0.05 |
0.02 |
2.09 |
0.21 |
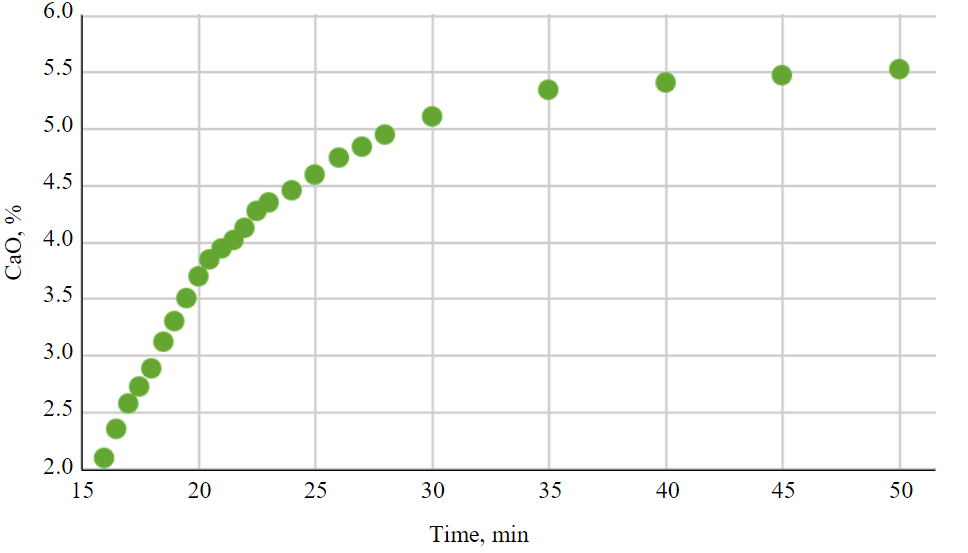
Fig.1. The content of active calcium oxide
Due to the fact that the initial purpose of the study was wastewater purification from Cd, Cu, Fe, Mn, Zn ions, the water-soluble forms of only the listed ions and Al were determined in the ash due to its high gross content in the ash. When exposed to 1 m3 of water per 1 kg of ash, passes into the solution, mg: Cd – 0.14; Cu – 1.00; Fe – 10.6; Mn – 0.65; Zn – 0.79, as well as about 1.4 g of Al.
The value of the toxic multiplicity of dilution of the water extract, necessary to reduce the toxic effect that causes pathological changes or death of organisms, was 645, which corresponds to the III hazard class. Thus, the need for pretreatment of ash before storage in landfills is confirmed.
The main characteristic necessary to assess the potential use of ash as a neutralization reagent is the content of active calcium oxide. According to the graph of the dependence of the content of active calcium oxide on time (Fig.1), it was found that its release is prolonged. It should be noted that the maximum release occurs up to 35 min, after which the content of active calcium oxide changes insignificantly. Thus, the content of active calcium oxide in the studied ash is on average 5.5 %.
For comparison, it should be noted that when purifying sulfuric acid waters in industry, lime milk is often added, where the content of active calcium oxide is 5-10 %. In order for the neutralization reaction to proceed completely, it is required to ensure the contact time of the ash – contaminated water system for 15 min for slightly polluted wastewater and more than 30 min for highly polluted waters (SNiP 2.04.03-85).
Thus, it can be assumed that the content of 5.5 % active calcium oxide in the ash will allow it to be used in its original form as a reagent for neutralizing sulfuric acid waters contaminated with metal ions, while ensuring a cleaning time of about 35 min.
According to previous studies [28], the optimal pH range for the formation of metal hydroxides (Cu, Cd, Fe, Mn, Zn) and their parallel deposition in a multicomponent solution is pH 8-9. In this range, the formation of Al hydroxides is also noted, which will allow to precipitate its water-soluble form, which is washed out of the ash during the purification process.
To achieve the required pH range, it is necessary to determine the optimal ash dose per unit volume of the model solution of acidic drainage waters, as well as the time required to reach pH 9 as the upper point of the range. Based on the graph of the pH dependence on the contact time (Fig.2), it can be noted that the samples weighing 1.0 and 1.1 g are not enough to achieve pH 9. When using a 1.6 g samples, pH 9 is reached as early as the 25th min. At the same time, according to the previously established release time of active calcium oxide (35 min), the use of ash for 25 min is impractical. Thus, the optimal dose was 1.3-1.5 g.
To evaluate the effectiveness of cleaning the model solution from metals with different doses of ash (Table 2) a repeated experiment was conducted, which was stopped after the set 35 min. The residual Al content in the obtained filtrates was also estimated relative to the initial concentration in the water extract.
For Cd, Mn, Zn, a decrease in concentration is observed with an increase in the pH of the system, i.e. with an increase in the ash dose. For Al, the opposite situation is observed – to achieve the maximum degree of purification (over 99 %), the pH should not exceed 8.7. Cu and Fe ions are deposited with an efficiency of over 99.9 % over the entire pH range.
Table 2
Efficiency of purification of the model solution from metals
Ash weight, g |
Initial pH of the solution |
Al |
Cd |
Cu |
Fe |
Mn |
Zn |
Final pH of the solution |
1.6 |
3.54 |
96.12 |
99.99 |
99.95 |
99.97 |
99.83 |
99.99 |
9.25 |
1.5 |
3.46 |
96.60 |
99.88 |
99.95 |
99.97 |
99.24 |
99.99 |
8.95 |
1.4 |
3.51 |
96.79 |
99.25 |
99.95 |
99.97 |
89.30 |
99.99 |
8.91 |
1.3 |
3.49 |
97.21 |
98.70 |
99.95 |
99.97 |
81.79 |
99.99 |
8.89 |
1.2 |
3.58 |
98.56 |
97.18 |
99.95 |
99.97 |
66.08 |
99.97 |
8.86 |
1.1 |
3.52 |
99.64 |
90.06 |
99.95 |
99.97 |
44.20 |
99.88 |
8.70 |
1.0 |
3.50 |
99.64 |
67.56 |
99.95 |
99.97 |
31.95 |
96.71 |
8.20 |
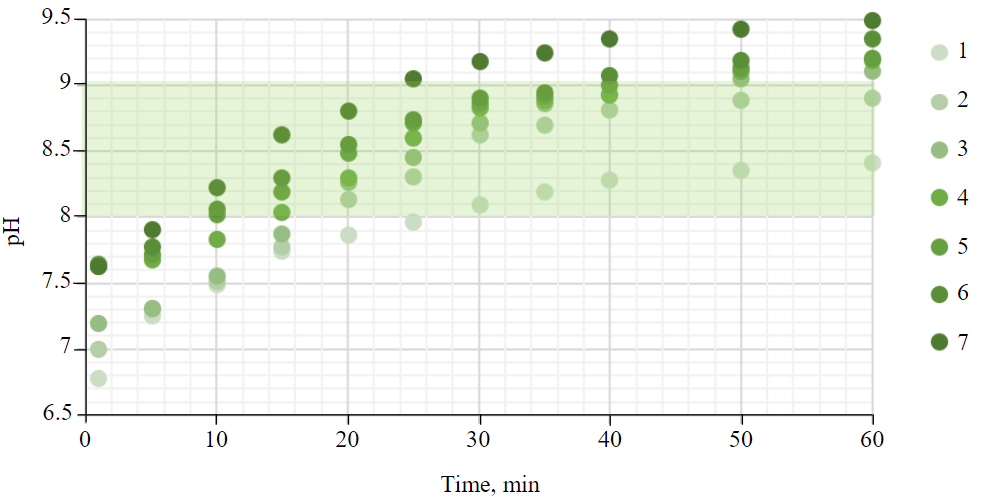
Fig.2. Dependence of pH on contact time at different ash doses
1 – 1.0; 2 – 1.1; 3 – 1.2; 4 – 1.3; 5 – 1.4; 6 – 1.5; 7 – 1.6 g
Thus, in order to achieve a purification efficiency of over 99 % for the studied ions (Cu, Cd, Fe, Mn, Zn), it is necessary to apply a dose of ash in the amount of 1.5 g per 100 ml of a model drainage water solution, while the purification efficiency from the Al ion will be 96.6 %.
When sulfuric acid waters interact with an alkaline reagent, in this case ash with active calcium oxide, a reaction of neutralization of sulfuric acid occurs with the formation of poorly soluble calcium sulfate and water. When the pH changes to the alkaline side, metals form hydroxides, precipitate and co-precipitate with calcium sulfate. The neutralization of acidic waters, as has been experimentally proven, is directly affected by the dosage of the introduced alkaline reagent, and, accordingly, the initial concentration of sulfates and metals in the solution.
According to the results of the study of the effect of the initial concentration of metal ions in solution on the purification efficiency (Table 3) it was found that the achievement of an optimal pH (8.75) with the addition of an ash sample of 1.5 g occurs at an initial concentration of each metal in a solution of 100 mg/l. At the same time, insufficient deposition efficiency of Cd and Mn ions is noted (82.06 and 22.14 %). Further, with a decrease in the concentration of each metal by half (50 mg/l), there is an increase in the efficiency of purification from Cd and Mn ions by 16 and 55 %, respectively. This may indicate that when cleaning multicomponent solutions, it is necessary to select the ash dosage empirically, based on the concentrations of each of the metal ions. Step-by-step purification of waters from various groups of metals may also be provided, depending on the pH of precipitation of metal hydroxides and the initial concentration of ions in solution.
An assessment of the degree of leaching of metals from the sediment after purification showed that the number of metal ions passing from ash to solution decreased by 1.3-5.6 times (Table 4). This may be due to the fact that the formed calcium sulfate “cements” metal hydroxides into an insoluble precipitate, which helps to reduce the transition of metal ions back into solution when exposed to water on the precipitate. Zn and Fe ions bind most well, and their leachability decreases by 82 and 77 %, respectively. The transition of Al, Cd, Cu and Mn ions into solution decreases by an average of 25 %.
Table 3
The efficiency of water purification from metals depending on the concentration of metal ions in the solution
The dilution multiplicity |
Initial pH of the solution |
Cd |
Cu |
Fe |
Mn |
Zn |
Final pH of the solution |
1 |
3.52 |
4.38 |
71.35 |
41.39 |
2.29 |
4.24 |
5.5 |
2 |
3.48 |
7.88 |
97.91 |
78.89 |
3.21 |
11.19 |
6.25 |
4 |
3.56 |
20.00 |
99.86 |
99.91 |
1.98 |
38.31 |
7.3 |
10 |
3.51 |
82.06 |
99.98 |
99.99 |
22.14 |
99.53 |
8.75 |
20 |
3.57 |
98.81 |
99.95 |
99.87 |
77.10 |
99.76 |
9.45 |
40 |
3.49 |
99.94 |
99.98 |
99.89 |
99.79 |
99.97 |
10.5 |
100 |
3.50 |
99.97 |
99.95 |
99.89 |
99.92 |
99.92 |
10.8 |
Table 4
Reducing the degree of leaching of metal ions from ash
Indicator |
Al |
Cd |
Cu |
Fe |
Mn |
Zn |
The amount of metal passing into 1 liter of solution, mg Initial ash |
14.1 |
0.0014 |
0.01 |
0.106 |
0.0065 |
0.0079 |
Sediment after purification |
10.7 |
0.0011 |
0.0072 |
0.0244 |
0.0050 |
0.0014 |
Reducing the degree of leachability, % |
24.11 |
21.43 |
28.00 |
76.98 |
23.08 |
82.53 |
The reduction of water-soluble forms of metals in the ash after using it as a reagent for cleaning the model solution affects the toxicity of the water extract. The value of the toxic dilution multiplicity of the water extract from the sediment was 46, which is 14 times lower than the value of the toxic dilution multiplicity of the water extract from the source water. The sediment after cleaning corresponds to hazard class IV. At least two bioindicators must be used to accurately determine the hazard class of ash and sediment.
Conclusion
The storage of waste from mining activities in the form of man-made massifs and the impact of atmospheric precipitation, surface and groundwater on them lead to the formation of highly mineralized multicomponent acid drainage streams, which, entering natural water objects, pollute them with metals. The need to neutralize and purify such effluents remains an urgent problem worldwide, especially for the Arctic zone, due to its particular vulnerability.
Ash from the combustion of alternative fuels from MSW was considered in the study as a reagent for neutralization and purification of water from metals. The studied average ash sample contains about 5.5 % of active calcium oxide. The process of interaction of the model solution with ash showed that ash effectively neutralizes and removes metal ions (Al, Cd, Cu, Fe, Mn, Zn) from the solution. The effectiveness of water purification primarily depends on the pH value achieved during the neutralization process; The pH of the system is regulated by the ash dose per volume of water, the contact time and depends on the initial concentration of metal ions and sulfates in the solution. At the initial concentration of the model solution of acidic drainage waters, 15 g/l of ash must be added for neutralization to pH 8-9 and purification from metal ions with an efficiency of 96.60 to 99.99 %, contact time 35 min.
As a result of the interaction of ash containing active calcium oxide and sulfuric acid waters, a poorly soluble calcium sulfate is formed, which “cements” the metal hydroxides formed during neutralization. The degree of leachability of metal ions from ash decreases by 1.3-5.6 times, as a result of which the toxic dilution multiplicity of water extract from ash decreases by 14 times. This suggests that the effect of sulfuric acid waters on ash helps to reduce the toxicity of ash.
The conducted studies confirm the possibility of using ash from the combustion of alternative fuels from MSW as a reagent for neutralization and metal purification of acidic drainage waters of tailings farms. This will solve two urgent problems: pollution of natural waters with metal ions in the area of the location of man-made massifs of mining enterprises and reducing the toxicity of ash before its landfill placement. In further studies, it is planned to consider water purification from a larger number of metals, including using multi-stage purification from metal groups; determination of the content of water-soluble forms of all metal ions found in ash and the degree of reduction in washout of each of them; assessment of ash toxicity by two bioindicators (before and after neutralization with acidic waters).
References
- Strizhenok A.V., Bykova M.V., Korotaeva A.E. Extractive Industries as a Source of Greenhouse Gas Emissions and the Possibility of its Natural Sequestration under the Climatic Conditions of Central and Northern Eurasia. Journal of Ecological Engineering. 2024. Vol. 25. Iss. 5, p. 43-69. DOI: 10.12911/22998993/185585
- Mysin A.V., Kovalevskiy V.N., Kirkin A.P. Ensuring environmental safety of massive explosions in the combined development of coal deposits in Kuzbass. IOP Conference Series: Earth and Environmental Science. 2021. Vol. 848. N 012139. DOI: 10.1088/1755-1315/848/1/012139
- Pashkevich M.A., Kulikova Yu.A. Monitoring and assessment of the negative impact of technogenic massives of the mineral and raw complex. Mining Informational and Analytical Bulletin. 2023. N 9-1, p. 231-247 (in Russian). DOI: 10.25018/0236_1493_2023_91_0_231
- Matveeva V.A., Lukyanova A.V. Evaluation of the effectiveness of the use of fluxing limestone of Sokol-Sitov deposit as a ameliorant for the neutralization of acidic soils. Geology and Geophysics of Russian South. 2023. Vol. 13. N 3, p. 155-168 (in Russian). DOI: 10.46698/VNC.2023.26.57.012
- Smolyakov B.S., Yermolaeva N.I., Romanov R.E., Sagidullin A.K. Response of plankton communities to the remediation of reservoirs contaminated with heavy metals: a field experiment. Water and Ecology: Problems and Solutions. 2020. N 2 (82), p. 104-113 (in Russian). DOI: 10.23968/2305-3488.2020.25.2.104-113
- Petrova T.A., Epishina A.D. Anti-corrosion protection of pipelines at mining and processing enterprises. Obogashchenie Rud. 2023. N 6, p. 52-58 (in Russian). DOI: 10.17580/or.2023.06.09
- Haque A., Jewel A.S., Hasan J. et al. Seasonal variation and ecological risk assessment of heavy metal contamination in surface waters of the Ganges river (Northwestern Bangladesh). Malaysian Journal of Analytical Sciences. 2019. Vol. 23. N 2, p. 300-311. DOI: 10.17576/mjas-2019-2302-14
- Slukovskii Z.I., Guzeva A.V., Dauvalter V.A. Rare earth elements in surface lake sediments of Russian arctic: Natural and potential anthropogenic impact to their accumulation. Applied Geochemistry. 2022. Vol. 142. N 105325. DOI: 10.1016/j.apgeochem.2022.105325
- Legostaeva Ya.B., Gololobova A.G. Bottom Sediments as an Indicator of the Geoecological State of Natural Water Currents. Ecology and Industry of Russia. 2022. Vol. 26. N 11, p. 66-71 (in Russian). DOI: 10.18412/1816-0395-2022-11-66-71
- Kolokolchikova R.S. Anthropogenic impact of pulp and paper enterprises on the environment as a factor leading to the ecological crisis in the European North of Russia in the late Soviet period (the case study of the Kotlas pulp and paper mill). Bulletin of Moscow Region State University. Series: History and Political Sciences. 2021. N 4, p. 123-133 (in Russian). DOI: 10.18384/2310-676X-2021-4-123-133
- Kotova E.I., Korobov V.B., Shevchenko V.P., Iglin S.M. Environmental situation in the Severnaya Dvina mouth zone (the White Sea). Advances in current natural sciences. 2020. N 5, p. 121-129 (in Russian). DOI: 10.17513/use.37402
- Alekseeva P.V., Kurilina T.A., Zhuravlev A.S. Assessment of the current state of surface waters of the Krasnoyarsk territory by their content of heavy metal ions of copper, zinc and nickel. Globus: Technical sciences. 2021. Vol. 7. N 2 (38), p. 52-57 (in Russian). DOI: 10.52013/2713-3079-38-2-8
- Yakovlev E.Yu., Druzhinina A.S., Druzhinin S.V., Ivanchenko N.L. Assessment of the impact of industrial discharges on surface water quality in the Kola Peninsula. Transactions of the Karelian Research Centre of the Russian Academy of Sciences. 2022. N 6, p. 88-101 (in Russian). DOI: 10.17076/lim1617
- Moiseenko T.I., Morgunov B.A., Gashkina N.A. et al. Ecosystem and human health assessment in relation to aquatic environment pollution by heavy metals: case study of the Murmansk region, northwest of the Kola Peninsula, Russia. Environmental Research Letters. 2018. Vol. 13. N 6. N 065005. DOI: 10.1088/1748-9326/aab5d2
- Sudarno J., Santoso R. Physical and Chemical Treatability Study in Wastewater Treatment Plant Design (Case Study: Leather Tanning Industry). IOP Conference Series: Earth and Environmental Science. 2023. Vol. 1268. N 012025. DOI: 10.1088/1755-1315/1268/1/012025
- Filatova E.G. Wastewater treatment from heavy metal ions, based on the physico-chemical processes. Review. Proceedings of Universities. Applied Chemistry and Biotechnology. 2015. N 2 (13), p. 97-109 (in Russian).
- Kulikova A.A., Sergeeva Yu.A., Ovchinnikova T.I., Khabarova E.I. Formation of mine water composition and analysis of treatment methods. Mining Informational and Analytical Bulletin. 2020. N 7, p. 135-145 (in Russian). DOI: 10.25018/0236-1493-2020-7-0-135-145
- Tatarintseva E., Olshanskaya L., Bukharova E. Wastewater purification from heavy metal ions and technologies for utilization of metal-containing electroplating sludge. PNRPU Bulletin, Applied ecology. Urban development. 2021. N 3 (43), p. 53-64 (in Russian). DOI: 10.15593/2409-5125/2021.03.05
- Semenov A.F., Liberman E.Yu., Kolesnikov V.A. Review of modern methods of wastewater treatment of electroplating plants from heavy metal ions. Uspekhi v khimii i khimicheskoy tekhnologii. 2020. Vol. 34. N 4 (227), p. 83-85.
- Smirnova V.S., Hudorozhkova S.A., Ruchkinova O.I. Substantiation of optimal conditions for the reagent purification of wash water from heavy non-ferrous metal ions. Bulletin of PNRPU. Construction and Architecture. 2019. Vol. 10. N 2, p. 106-118. DOI: 10.15593/2224-9826/2019.1.09
- Borowski G., Ozga M. Comparison of the processing conditions and the properties of granules made from fly ash of lignite and coal. Waste Management. 2020. Vol. 104, p. 192-197. DOI: 10.1016/j.wasman.2020.01.024
- 22. Kremenetskaya I.P., Ivanova T.K., Gurevich B.I. et al. Separate deposition of metals from highly concentrated solutions with granulated magnesia-silicate reagent. Vestnik of MSTU. Vol. 24. N 1, p. 118-130 (in Russian). DOI: 10.21443/1560-9278-2021-24-1-118-130
- Chukaeva M.A., Povarov V.G., Sverchkov I.P. Iron-Containing Metalworking Wastes as a Chemosorbent for Wastewater Treatment from Molybdenum Ions. Moscow University Chemistry Bulletin. 2020. Vol. 75. N 1, p. 36-42. DOI: 10.3103/S0027131420010058
- Antoninova N.Yu., Sobenin A.V., Usmanov A.I., Shepel K.V. Assessment of the possibility of using iron-magnesium production waste for wastewater treatment from heavy metals (Cd2+, Zn2+, Co2+, Cu2+). Journal of Mining Institute. 2023. Vol. 260, p. 257-265. DOI: 10.31897/PMI.2023.34
- Cheremisina O., Litvinova T., Sergeev V. et al. Application of the Organic Waste-Based Sorbent for the Purification of Aqueous Solutions. Water. 2021. Vol. 13. Iss. 21. N 3101. DOI: 10.3390/w13213101
- Shishelova T.I., Samuseva T.I. The use of fly ash from the thermal power plant of OAO Irkutskenergo for chemical reclamation of acidic soils. The successes of modern natural science. 2004. N 12, p. 85-86.
- Smirnov Yu.D., Matveeva V.A., Yakovlev N.M., Sakhabutdinova E.R. Modern wastewater treatment technologies in galvanic production: analysis and evaluation. Gornyi zhurnal. 2023. N 9, p. 55-60 (in Russian). DOI: 10.17580/gzh.2023.09.08
- Smirnov Y.D., Penezeva D.V. Experimental justification for converting paper, cardboard and plant waste into biomats. Environmental Geochemistry and Health. 2023. Vol. 45. Iss. 1, p. 215-225. DOI: 10.1007/s10653-022-01305-w
- Nedoseka E.V., Kozlovskiy V.V. Regional Specifics of Municipal Solid Waste Management in Arctic Regions of the Russian Federation. Arctic and North. 2021. N 42, p. 223-241 (in Russian). DOI: 10.37482/issn2221-2698.2021.42.223
- Grigorieva O.V., Markov A.V., Ivanets M.O., Terentyeva V.V. The integrated estimation methodology of the environmental in the Arctic using aerospace image situation. Arctic: ecology and economy. 2018. N 1 (29), p. 37-47 (in Russian). DOI: 10.25283/2223-4594-2018-1-37-47
- Grebenets V.I., Tolmanov V.A., Khairedinova A.G., Yurov F.D. Issues of waste disposal in the Russian Artic regions. Regional Environmental Issues. 2019. N 3, p. 63-67 (in Russian). DOI: 10.24411/1728-323X-2019-13063
- Sverchkov I.P.,Gembitskaya I.M., Povarov V.G., Chukaeva M.A. Method of reference samples preparation for X-ray fluorescence analysis. Talanta. 2023. Vol. 252. N 123820. DOI: 10.1016/j.talanta.2022.123820
- Wenchao Ma, Lingyu Tai, Zhi Qiao et al. Contamination source apportionment and health risk assessment of heavy metals in soil around municipal solid waste incinerator: A case study in North China. Science of the Total Environment. 2018. Vol. 631-632, p. 348-357. DOI: 10.1016/j.scitotenv.2018.03.011
- Beikmohammadi M., Yaghmaeian K., Nabizadeh R., Mahvi A.H. Analysis of heavy metal, rare, precious, and metallic element content in bottom ash from municipal solid waste incineration in Tehran based on particle size. Scientific Reports. 2023. Vol. 13. N 16044. DOI: 10.1038/s41598-023-43139-1
- Yuying Zhang, Lei Wang, Liang Chen et al. Treatment of municipal solid waste incineration fly ash: State-of-the-art technologies and future perspectives. Journal of Hazardous Materials. 2021. Vol. 411. N 125132. DOI: 10.1016/j.jhazmat.2021.125132
- Kicheol Kim, Kidong Kim, Miae Kim. Characterization of municipal solid-waste incinerator fly ash, vitrified using only end-waste glass. Journal of Cleaner Production. 2021. Vol. 318. N 128557. DOI: 10.1016/j.jclepro.2021.128557
- Ping Wang, Jiangshan Li, Yuanan Hu, Hefa Cheng. Solidification and stabilization of Pb–Zn mine tailing with municipal solid waste incineration fly ash and ground granulated blast-furnace slag for unfired brick fabrication. Environmental Pollution. 2023. Vol. 321. N 121135. DOI: 10.1016/j.envpol.2023.121135
- Pashkevich M.A., Kharko P.A. The use of a composite mix to remove metals from acidic drainage waters at tailings facilities. Obogashchenie rud. 2022. N 4, p. 40-47 (in Russian). DOI: 10.17580/or.2022.04.07